Abstract
Salt tolerance is a complex trait involving the coordinated action of many gene families that perform a variety of functions such as control of water loss through stomata, ion sequestration, metabolic adjustment, osmotic adjustment and antioxidative defense. In spite of the large number of publications on the role of antioxidative defense under salt stress, the relative importance of this process to overall plant salt tolerance is still a matter of controversy. In this article, the generation and scavenging of reactive oxygen species (ROS) under normal and salt stress conditions in relation to the type of photosynthesis is discussed. The CO2 concentrating mechanism in C4 and CAM plants is expected to contribute to decreasing ROS generation. However, the available data supports this hypothesis in CAM but not in C4 plants. We discuss the specific roles of enzymatic and non enzymatic antioxidants in relation to the oxidative load in the context of whole plant salt tolerance. The possible preventive antioxidative mechanisms are also discussed.
Introduction
Soil salinity is a major threat to global food security. Up to 20% of the world's irrigated land, which produces one third of the world's food, is salt affected.Citation1 Salinity affects plant growth and development in two ways. First, it imposes osmotic stress by reducing the soil water potential leading to limiting the water uptake. Second, it causes excessive uptake of ions particularly Na+ and Cl− that ultimately interferes with various metabolic processes. Plant responses to the osmotic and ionic components of salt stress are complicated and involve many gene networks and metabolic processes. Such responses depend mainly on the inherent salt tolerance of the plant, the severity of salt stress (the concentration of salt in the soil solution) and the duration of exposure of the plant roots to the salt (reviewed in refs. Citation2–Citation4). Consequently, plant responses to salt stress could lead to varying degrees of adaptation ranging from adaptation for growth continuation, though at a modified rate, to adaptation for survival where the growth is almost suspended but the plant stays alive until the stress is relieved. Contrarily, sensitive responses could result in accelerated senescence and eventually death of the plant.
The essential processes leading to plant adaption to salt stress include control of water loss through stomata, metabolic adjustment, toxic ion homeostasis, and osmotic adjustment.Citation2,Citation4 However, the significance of an additional process that is detoxification of (ROS) is still a matter of debate. On the one hand, it is considered as an essential component of salt tolerance based on studies on mutant and transgenic plants with enhanced capacities to scavenge ROS that showed higher salt tolerance (reviewed in ref. Citation2). On the other hand, it has been suggested that genetic differences in salt tolerance are not necessarily due to differences in the ability to detoxify ROS.Citation4 This approach is supported by the finding that Arabidopsis mutants lacking cytosolic and/or chloroplastic ascorbate peroxidase (APX) were more salt tolerant than the wild type plants.Citation5 Careful examination of the available information particularly recent findings on the production and scavenging of ROS under salt stress in relation to plant growth (adaptation) could resolve this paradox. Other aspects of ROS under salt stress such as their role in signaling have been reviewed elsewhere.Citation6
ROS and their Production Under Normal Conditions
Molecular oxygen in its ground state (triplet oxygen) is essential to life on earth. It is a relatively stable molecule that does not directly cause damage to living cells. However, when triplet oxygen receives extra energy or electrons, it generates a variety of ROS that will cause oxidative damage to various components of living cells including lipids, proteins and nucleic acids. The most common ROS are singlet oxygen (1O2), hydrogen peroxide (H2O2), superoxide anions (O-2) and hydroxyl radicals (OH•). Triplet oxygen has two unpaired electrons with parallel spin located in different orbitals. Upon receiving extra energy from a photosensitizer such as chlorophyll, these two electrons show anti-parallel spin, a change that substantially increases the oxidizing power of oxygen (singlet oxygen) (reviewed in ref. Citation7). When triplet oxygen receives an electron, it gives rise to O-2, which generates hydrogen peroxide and hydroxyl radicals through a series of chemical conversions (reviewed in ref. Citation8). In photosynthesis, light energy is captured by Photosystem II and I and used to excite electrons which go through a series of electron transport reactions and end up in NADPH. It is suggested that about 10% of the photosynthetic electrons leak from the photosynthetic electron transport chains to oxygen as a final electron acceptor (Mehler reaction) resulting in the formation of O-2.Citation9
Plants produce ROS under normal conditions essentially from photosynthesis, photorespiration and respiration. Additional sources include NADPH oxidases, amine oxidases and cell wallbound peroxidases (reviewed in ref. Citation10). The most common ROS generated under normal conditions are O-2 and H2O2 perhaps as a result of electron leakage from the photosynthetic and respiratory electron transport chains to oxygen. Another source of ROS (H2O2) is photorespiration resulting from the oxygenase activity of Rubisco. Rates of photorespiration are basically controlled by the ratio of [CO2] to [O2]and temperature. In C3 plants, photorespiration constitutes about 20–30% of photosynthesis under the current atmospheric conditions at 25°C.Citation11 Contrarily, C4 plants show lower rates of photorespiration (3.5–6% of photosynthesis) under various environmental conditions due to their CO2 concentrating mechanism.Citation12,Citation13 However, it seems that the levels of ROS under normal conditions do not vary largely between C3 and C4 plants. In a comparative study involving two wheat (C3) and two maize (C4) varieties, similar levels of lipid peroxidation (resulting from the activity of ROS) were observed.Citation14 This could imply that either the actual contribution of photorespiration to generation of ROS under normal conditions in C3 plants is less than what is expected or C3 plants have higher CAT activity than C4 ones, which immediately detoxify H2O2. It is not possible to verify this suggestion because CAT activity was not measured in that study.
Scavenging of ROS Under Normal Conditions
Plants control the concentrations of ROS under normal conditions by an array of enzymatic and non enzymatic antioxidants. Constitutive (under non stressful conditions) activity and expression of various antioxidant enzymes have been reported in C3 and C4 plants. Stepien and KlobusCitation14 reported constitutive activities of the antioxidant enzymes superoxide dismutase (SOD), ascorbate peroxidase (APX) and glutathione reductase (GR) in wheat and maize varieties. The constitutive activities of these enzymes were higher (up to two-fold) in maize than in wheat although levels of lipid peroxidation were the same in both plants. In another study on wheat and maize, the constitutive activities of SOD, APX, GR, dehydroascorbate reductase (DHAR) and catalase (CAT) as well as the contents of H2O2 and rates of lipid peroxidation were largely the same in both plants.Citation15 This difference in the measured constitutive activity is obviously due to the genetic background of the used varieties and/or experimental setup. Conversely, the constitutive activities of SOD, guaiacol peroxidase (POD), GR and CAT, have been reported to be higher in the leaves of sunflower (C3) than in those of maize (C4), but no consistent trend was observed for the activity of these enzymes in the roots. Furthermore, the enzyme activity depended largely on the plant organ and nitrogen source.Citation16 The available data does not support the hypothesis that C4 photosynthesis reduces the generation of ROS under normal conditions. Data on the production of ROS in obligate CAM plants as compared to C3 or C4 ones is rare if at all. Thus, more broad-spectrum comparative studies involving C3, C4 and CAM plants are needed in order to evaluate the effect of the type of photosynthesis on the production and scavenging of ROS under normal conditions (if any) and consequently under salt stress.
The constitutive expression of antioxidant enzymes has been reported in C3 and C4 plants at mRNA and protein levels.Citation17–Citation21 The consistent existence of constitutive activities and expression of antioxidant enzymes indicates that plants inevitably experience oxidative stress even under what is believed to be normal environmental conditions and they are fully equipped to cope with it. Most of the information on antioxidative defense comes from plants grown in controlled environments in which all conditions (including relative humidity, temperature, wind speed and light intensity) are kept more or less unchanged during the day/night periods. Under these conditions, the antioxidative defense may seem relatively unnecessary. However, plants grown in the field are subject to rapidly changing environmental conditions even in the absence of salt (or drought) stress. A simple example for this is the appearance of the sun from behind a cloud that imposes dramatic change in the light intensity resulting in sudden and massive increase in energy capture.Citation4 Simova-Stoilova et al.Citation22 studied the antioxidative activities of wheat subjected to field drought and reported well expressed SOD, CAT and non specific peroxidase (GPX) in the unstressed control plants. In some cases, the expression of these enzymes in the control plants was comparable to that in the droughted ones, indicating that the antioxidative defense is an integral part of the basic metabolism, enabling the plant to cope instantaneously with rapid environmental changes. In this context, the steady state levels of ROS are supposed to be used by the plant to monitor the intracellular levels of oxidative stress.Citation10
Generation and Scavenging of ROS Under Salt Stress in Relation to the Type of Photosynthesis
Three types of photosynthesis are recognized in green plants namely: C3, C4 and CAM (Crassulacean Acid Metabolism). In C3 plants, atmospheric CO2 is fixed directly by the carboxylase activity of Rubisco (Ribulose 1,5-bisphosphate carboxylase oxygenase) into a 5-carbon sugar to produce a 3-carbon compound. In C4 and CAM plants, atmospheric CO2 is first fixed into a 3-crabon compound by phosphenolpyruvate carboxylase (PEPC) to produce a 4-carbon compound, which is then decarboxylated to release CO2 in the vicinity of Rubisco, thereby concentrating CO2 and decreasing the chance of oxygenase reaction. In C4 plants, fixation of atmospheric CO2 and its subsequent release into the action of Rubisco are spatially separated (leaf mesophyll and bundle sheath cells respectively) whereas these two events are temporally separated in CAM plants (night and day respectively).
Theoretically, salt stress is expected to encourage the generation of ROS in plants. First: plants respond to salt stress by decreasing stomatal conductance to avoid excessive water loss. This in turn decreases the internal CO2 concentrations (Ci) and slows down the reduction of CO2 by Calvin cycle. This response leads to depletion of the oxidized NADP+, which acts as a final acceptor of electrons in PSI, and alternatively increases the leakage of electrons to O2 forming O-2.Citation23 Furthermore, Na+/Cl− toxicity resulting from salt stress could disrupt the photosynthetic electron transport and provoke electron leakage to O2.Citation24–Citation26 Second: the decrease in Ci slows down the reactions of Calvin cycle and induces photorespiration particularly in C3 plants, resulting in generation of more H2O2 in the peroxisome.Citation27–Citation29 Third: the cell membrane-bound NADPH oxidase and the apoplastic diamine oxidase are supposed to be activated during salt stress and therefore contribute to generation of ROS.Citation30–Citation34 Fourth: salt stress increases the rates of respiration with the consequence of respiratory electron leakage to O2.Citation35–Citation37
Practically, it is not possible to determine the contribution of each of the above sources to the generation of ROS under salt stress. However, photorespiration is strongly induced by salt stress in C3 but not in C4 and CAM plants,Citation38 (see above). It has been estimated that photorespiration accounts for over 70% of the H2O2 produced under osmotic stress.Citation39 This means that photorespiration is expected to be the major source of ROS under salt stress. Furthermore, the day-time regeneration of CO2 by malate decarboxylation in CAM plants has been suggested to limit the over-reduction of the photosynthetic machinery under water stress and therefore reduce the generation of ROS.Citation40 If this is true, then C4 and CAM plants should produce less ROS under salt stress as compared to C3 plants.
Experimental data reported by Stepien and Klobus14 on the tolerance to oxidative stress in wheat (C3) and maize (C4) indicated that maize suffered less oxidative stress as indicated by the levels of lipid peroxidation (H2O2 contents were not shown) under salt stress. In that study, varietal differences in the sensitivity to oxidative stress were observed in wheat but not in maize and no data was shown on the inherent salt tolerance of the tested varieties (growth under salt stress). Moreover, the constitutive and salt stress-inducible activities of SOD, APX and GR were higher in maize. It is thus unclear whether the higher tolerance of maize to oxidative stress is essentially due to lower production of ROS (perhaps due to C4 photosynthesis) or due to higher activities of the antioxidative enzymes. There is no evidence to suggest that the higher activities of antioxidant enzymes in maize are intrinsically related to C4 photosynthesis. In their comparative study on sunflower (C3) and maize (C4), Rios-Gonzalez et al.Citation16 reported that the activities of GR, SOD, POD and CAT were higher in the leaves of sunflower than in these of maize under salt stress with 100 mM NaCl. However, such high activities did not seem to be essentially due to salt stress response but rather were due to the higher constitutive activities and the nitrogen source. No consistent responses of the root antioxidant enzymes were observed in either plant. Growth parameters showed that sunflower was more salt tolerant than maize. Although higher activities of antioxidant enzymes in sunflower correlated with higher salt tolerance, it is unclear how much these activities have contributed to salt tolerance because no data on the production of ROS under salt stress was presented. It is evident that the available data on the production and scavenging of ROS in C3 and C4 plants is insufficient to deliver an unequivocal conclusion on the role of antioxidative defense under salt stress in both groups of plants. Consequently, the contribution of photorespiration to generation of ROS in C3 and C4 plants under salts stress should be re-examined more thoroughly.
CAM photosynthesis has been correlated with relief of oxidative stress in the salt-stressed ice plant (Mesembryanthemum crystallinum) by comparing the SOD activities in CAM-deficient mutant and the wild type after the development of CAM. The development of CAM in the wild type (but not in the mutant) was accompanied by lower SOD activities in the wild type. The conclusion was that the lack of CAM in the mutant imposed higher oxidative stress that required higher SOD activities.Citation41 However, neither growth parameters nor levels of ROS were shown and therefore the adaptive significance of CAM shift at whole plant level is unclear. Recently, Slesak et al.Citation42 reported that salt stressdependent CAM shift was followed by reduction in ROS contents in the leaves of ice plant although there was an increase in ROS contents during the C3-CAM transition. The authors suggested that the increase in ROS contents during the C3-CAM transition is not related to CAM but rather is the product of salt stress. The reduction in ROS content after the full development of CAM may indicate that CAM produces less ROS compared to C3 metabolism,Citation40 although the possibility of CAM-dependent increase in antioxidative efficiency by upregulation of SOD cannot be excluded.Citation43
It seems that CAM may be more effective in reducing oxidative stress compared to C3 and C4 metabolism. This could be because CAM is basically and adaptation to low water availability as well as low cellular CO2 concentrations in contrast to C4 metabolism in which the plant may benefit from the CO2 concentrating mechanism but at the same time continues losing water until it eventually closes the stomata. At this point, C4 may not represent an advantage over C3 metabolism in terms of ROS generation.
Types of Antioxidant Enzymes Activities Required for Proper Antioxidative Defense
The activities of antioxidant enzymes have been studied intensively and yet the significance of these enzymes in salt tolerance is still a matter of controversy, because high antioxidant enzymes activities have been associated with salt tolerance as well as salt sensitivity. This led to the suggestion that genetic differences in salt tolerance among plants are not necessarily due to differences in the ability to detoxify ROSCitation4 despite the large number of studies that correlate efficient antioxidative defense to salt tolerance. This paradox has probably resulted from, first: the fact that is hard to measure ROS contents in plant tissues and in many publications, enzyme activity measurements were not accompanied by ROS contents. In these cases, high antioxidant activities could be interpreted as symptoms of oxidative stress or damage (the plant upregulates the antioxidant enzymes because it is producing more ROS). Conversely, high antioxidant activity could be interpreted as higher tolerance to oxidative stress (the plant suffers less oxidative stress because it has higher antioxidant activity). Second: efficient antioxidative defense has often been viewed as upregulation of a full set of antioxidant enzymes (SOD, CAT, POD, GR, etc.) although each of these enzymes performs a specific function and its activity should be assigned to a specific role in ROS detoxification i.e., efficient antioxidative activity does not necessarily mean the strong upregulation of the full set of antioxidant enzymes and vice versa.
Many comparative studies using salt tolerant and sensitive genotypes have correlated the salt tolerance to an increase in the activity of antioxidant enzymes.Citation44–Citation48 Moreover, overexpression of some antioxidant enzymes has been reported to improve the salt tolerance.Citation49,Citation50 Contrarily, salt tolerance did not consistently depend on higher antioxidant activities.Citation51 Furthermore, a mutant of Arabidopsis lacking cytosolic and/or chloroplastic APX isoforms has been found to be more salt tolerant than the wild type.Citation5 This discrepancy concerning the role of ROS detoxification under salt stress may have resulted at least in part from (1), the technical inability to determine the major sources(s) of ROS under salt stress and therefore the appropriate antioxidant enzyme required and (2), the expectation that ROS scavenging enzymes particularly catalases and peroxidases perform similar functions.
The most common targets of antioxidant enzymes under salt stress are O-2 and H2O2.Citation10 O-2 is converted to H2O2 by SOD in the chloroplast, mitochondrion, cytoplasm, apoplast and peroxisome.Citation52 H2O2 is detoxified by two groups of enzymes namely: catalases and peroxidases. H2O2 at low concentrations is suggested to play a role in signaling under stress.Citation6,Citation8 Therefore, the role of H2O2-detoxifying enzymes is to impose tight control on its cellular concentrations rather than to remove it completely (). CAT is known to have lower affinity to H2O2 than POD (mM and µM range respectively). Thus, CAT is suggested to be involved in mass scavenging of H2O2 whereas POD is suggested to be involved in fine regulation of H2O2.Citation10,Citation53–Citation55 Recently, we have shown that salt tolerance in barnyard grass (Echinochloa crusgalli) mutants depended on higher activities of POD, APX and GR, whereas loss of activities of these enzymes in the sensitive plants led to induction of CAT that coincided with accumulation of ROS and growth cessation. Our conclusion was that POD, APX and GR are involved in fine regulation of ROS and loss of their activities resulted in buildup of ROS to high levels that resulted in CAT induction. However, because CAT has low affinity to H2O2 and is unable to bring it down to the physiological levels, the plants suffered oxidative stress even in the presence of high CAT activity.Citation20 In this case, lower CAT activities correlated to salt tolerance simply because large increase in CAT activity was not essential as long as POD, APX and GR were imposing tight control on H2O2 concentration. SOD activity was lowest in the most salt tolerant mutant that also showed low levels of ROS indicating that high SOD activity was not needed.
In their study on cow peas, Cavalcanti et al.Citation56 showed that the plants accumulated higher levels of malondialdehyde (MDA, indicator of lipid peroxidation) concomitantly with growth retardation under salt stress. POD was induced by salt stress in contrast to CAT and SOD that were either inhibited or unchanged respectively. The authors concluded that salt sensitivity could be due to failure of SOD and CAT to detoxify O-2 and H2O2 produced from photosynthesis and photorespiration respectively. In this case, and contrarily to our findings, lower SOD and CAT activities correlated to salt sensitivity. In general, the significance of particular activities of ROS scavenging enzymes should be interpreted based on the overall plant response to salt stress, the cellular levels of ROS and the activities of other enzymes involved in ROS scavenging in order to make a precise correlation between enzyme activity and plant adaptation.
It appears that induction of ROS generation under salt stress is unavoidable in many plants and the scavenging process requires a concerted action of ROS detoxifying enzymes. However, the relative importance of ROS scavenging process in relation to other processes involved in salt tolerance (see introduction) as well as the relative importance of each type of activity (SOD, CAT, POD, etc.) required for ROS scavenging vary from a plant to the other according to the rates of ROS generation and the major sources of ROS and consequently the type of the required antioxidative activity. Thus, ROS scavenging as a whole may be relatively unimportant for plants that generate less ROS under salt stress.Citation57 Moreover, salt sensitivity related to oxidative stress results from “breaks” in the “weak links” of the ROS detoxifying chains.
Non Enzymatic Antioxidants
Scavenging of ROS depends on both enzymatic and non enzymatic components. Among the important non enzymatic antioxidants are ascorbate (which acts as a reductant for peroxidases), tocopherol and carotenoids. An ascorbate deficient Arabidopsis mutant showed higher sensitivity to the oxidative component of salt stress compared to the wild type. The mutant had lower content of reduced ascorbate, lower activities of monodehydroascorbate reductase (MDAR) and dehydroascorbate reductase (DHAR), and higher levels of H2O2, indicating that ascorbate can limit ROS scavenging under salt stress.Citation58 Athar et al.Citation59 used exogenous ascorbic acid to alleviate the oxidative stress in wheat subjected to salinity stress. This indicates that steady state contents of ascorbate may not be enough for efficient antioxidative defense under salt stress and its de novo synthesis may be necessary. Tocopherol is located in cell membranes and plays an important role in the scavenging of lipid peroxyl radicals. It has been shown to accumulate in plants subjected to oxidative stress.Citation60,Citation61 Carotenoids are known to quench singlet oxygen and minimize its formation by receiving excess energy from the excited chlorophyll.Citation62 However, the contribution of carotenoids to ROS scavenging under salt stress is unclear since it has been reported that their contents generally decrease in response to salt stress.Citation63
Preventive Antioxidative Defense
It is surprising that plants vary in their ability to produce ROS under salt stress although they share the mechanisms of ROS generation. One reason for this variation may be the deployment of physiological preventive antioxidative mechanisms that could minimize the generation of ROS (). Among these mechanisms is the shift from C3 to CAM that has been shown to reduce the oxidative load under salt stress (see above). Based on their properties, carotenoids could play a role in preventive antioxidative defense, but more investigation is needed to verify this suggestion.
Alternative oxidases (AOXs) are a group of enzymes that can divert electrons from electron transport chains in the mitochondria and chloroplasts and use them to reduce O2 into water. By doing so, AOXs exhaust the intracellular oxygen as well excess electrons and thereby contributes to reducing the generation of O-2 (reviewed in ref. Citation9). AOXs are expressed under normal conditions but can be further induced by stress treatments (reviewed in ref. Citation64). The lower capacity of plant mitochondria to produce ROS compared to the chloroplasts has been attributed to AOX activity.Citation8,Citation65 Giraud et al.Citation64 reported that Arabidopsis mutant lacking alternative oxidase1a (aox1a) showed acute sensitivity to drought and light stress. The authors showed that the effect of the mutation was not limited to the mitochondria where AOX is located but rather involved the regulation of many other genes involved in the stress response, indicating that AOX plays a role in controlling the steady state level of ROS in the cell. However, the expression of different AOX genes at mRNA and protein levels has been studied in the roots of Vigna unguiculata cultivars with contrasting salt and drought tolerance. The expression of two AOX genes was not detectable under control or salt stress, while that of a third gene (VuAox2b) showed no consistent response to salt stress at mRNA and protein levels as related to whole plant salt tolerance.Citation66 Although AOX is expected to play a role in preventing ROS generation, the relationship between its activity and stress response is unclear presumably because it is under transcriptional and post transcriptional regulation involving other factors than oxidative stress.Citation67 More information is needed to understand the role of AOX in controlling the generation of ROS under salt stress.
Another preventive strategy involves modifying the capacity of Photosystem II and I to capture light energy. Malkin and NiyogiCitation68 suggested a model for regulation of energy capture and distribution between photosystem II and I. In this model, part of the light harvesting complex II (LHC-II), which works as auxiliary antenna for photosystem II, is phosphorylated when the plastoquinone, which works as a mobile electron carrier between PSII and I, pool is over-reduced (a situation that is also likely to occur under salt stress). Upon phosphorylation, LHC-II migrates away from the stacked granal membranes (where PSII is located) thereby decreasing the energy capture by PSII. Dephosphorylation and back migration of LHC-II occurs when the plastoquinone pool is not over-reduced. However, the validity of this model and its significance under salt stress await evidence.
Concluding Remarks and Future Prospects
Generation of ROS seems to be unavoidable under normal conditions as evidenced by the constitutive expression and activity of antioxidant enzymes in almost all studied plants so far. C3, C4 and CAM plants are supposed to vary in terms of ROS generation under salt stress. However, the available data indicates that C3 and C4, but not CAM, plants are more or less equally subject to salt stress-induced oxidative stress. More broad-spectrum investigations using C3, C4 and CAM plants with contrasting salt tolerance are required to verify the role of photosynthesis versus the salt tolerance background of the plant in controlling ROS generation under salt stress. Although green plants share the mechanisms of ROS generation under salt stress, some plants are known to produce less ROS. Intensive studies are also needed to reveal the underlying mechanisms of this phenomenon particularly the role of AOX.
Figures and Tables
Figure 1 Main intracellular sites of ROS generation under salt stress. The possible preventive antioxidative mechanisms are shown in italic in each cell organelle. Question marks mean the mechanism needs more evidence. The generated ROS are regulated by the action of different enzymes as indicated. The ROS concentrations in excess of normal steady state level work as signal to induce the defense mechanisms. Other sites of ROS generation (cell wall, etc.) are not shown for the sake of simplicity.
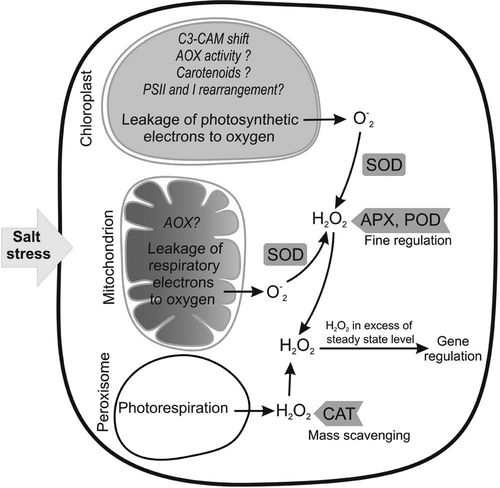
References
- FAO. FAO Land and Plant Nutrition Management Service 2007; http://www.fao.org/ag/agl/agll/spush
- Hasegawa PM, Bressan RA, Zhu J-K, Bohnert HJ. Plant cellular and molecular responses to high salinity. Ann Rev Plant Biol 2000; 51:463 - 499
- Zhu J-K. Salt and drought signal transduction in plants. Annu Rev Plant Biol 2002; 53:247 - 273
- Munns R, Tester M. Mechanisms of salinity tolerance. Ann Rev Plant Biol 2008; 59:651 - 681
- Miller G, Suzuki N, Rizhsky L, Hegie A, Koussevitzky S, Mittler R. Double mutants deficient in cytosolic and thylakoid acsorbate peroxidase reveal a complex mode of interaction between reactive oxygen species, plant development and a response to abiotic stress. Plant Physiol 2007; 144:1777 - 1785
- Hancock J, Desikan R, Harrison J, Bright J, Hooley R, Neill S. Doing the unexpected: proteins involved in hydrogen peroxide perception. J Exp Bot 2006; 57:1711 - 1718
- Krieger-Liszkay A. Singlet oxygen production in photosynthesis. J Exp Bot 2004; 56:337 - 346
- Apel K, Hirt H. Reactive oxygen species: metabolism, oxidative stress and signal transduction. Annu Rev Plant Biol 2004; 55:373 - 399
- Foyer CH, Noctor G. Oxygen processing in photosynthesis: Regulation and signalling. New Phytol 2000; 146:359 - 388
- Mittler R. Oxidative stress, antioxidants and stress tolerance. Trends Plant Sci 2002; 7:405 - 410
- Sage RF. The evolution of C4 photosynthesis. New Phytol 2004; 161:341 - 370
- Lacuesta M, Dever LV, Miñoz-Rueda A, Lea PJ. A study of photorespiratory ammonia production in the C4 plant Amaranthus edulis, using mutants with altered photosynthetic capacities. Physiol Plant 1997; 99:447 - 455
- Carmo-Silva AE, Powers SJ, Keys AJ, Arrabac MC, Parry MAJ. Photorespiration in C4 grasses remains slow under drought conditions. Plant Cell Environ 2008; 31:925 - 940
- Stepien P, Klobus G. Antioxidant defense in the leaves of C3 and C4 plants under salinity stress. Physiol Plant 2005; 125:31 - 40
- Nayyar H, Gupta D. Differential sensitivity of C3 and C4 plants to water deficit stress: Association with oxidative stress and antioxidants. Environ Exp Bot 2006; 58:106 - 113
- Rios-Gonzalez K, Erdei L, Lips SH. The activity of antioxidant enzymes in maize and sunflower seedlings as affected by salinity and different nitrogen sources. Plant Sci 2002; 162:923 - 930
- Bueno P, Piqueras A, Kurepa J, Savoure A, Verbruggen N, Montagu MV, Inze D. Expression of antioxidant enzymes in response to abscisic acid and high osmoticum in tobacco BY-2 cell cultures. Plant Sci 1998; 138:27 - 34
- Menezes-Benavente L, Teixeira FK, Kamei CLA, Margis-Pinheiro M. Salt stress induces altered expression of genes encoding antioxidant enzymes in seedlings of a Brazilian indica rice (Oryza sativa L.). Plant Sci 2004; 166:323 - 331
- Mittova V, Guy M, Tal M, Volokita M. Salinity upregulates the antioxidative system in root mitochondria and peroxisomes of the wild salt-tolerant tomato species Lycopersicon pennellii. J Exp Bot 2004; 55:1105 - 1113
- Abogadallah GM, Serag MM, Quick WP. Fine and coarse regulation of reactive oxygen species in the salt tolerant mutants of barnyard grass and their wildtype parents under salt stress. Physiol Plant 2009; 138:60 - 73
- Rubio MC, Bustos-Sanmamed P, Clemente MR, Becana M. Effects of salt stress on the expression of antioxidant genes and proteins in the model legume Lotus japonicus. New Phytol 2009; 181:851 - 859
- Simova-Stoilova L, Demirevska K, Petrova T, Tsenov N, Feller U. Antioxidative protection and proteolytic activity in tolerant and sensitive wheat (Triticum aestivum L.) varieties subjected to long-term field drought. Plant Growth Regul 2009; 58:107 - 117
- Hsu SY, Kao CH. Differential effect of sorbitol and polyethylene glycol on antioxidant enzymes in rice leaves. Plant Growth Regul 2003; 39:83 - 90
- Gossett DR, Millhollon EP, Lucas MC. Antioxidant response to NaCl stress in salt-tolerant and salt-sensitive cultivars of cotton. Crop Sci 1994; 34:706 - 714
- Borsani O, Valpuesta V, Botella MA. Evidence for a role of salicylic acid in the oxidative damage generated by NaCl and osmotic stress in Arabidopsis seedlings. Plant Physiol 2001; 126:1024 - 1030
- Slesak I, Miszalski Z, Karpinska B, Niewiadomska E, Ratajczak R, Karpinski S. Redox control of oxidative stress responses in the C-3-CAM intermediate plant Mesembryanthemum crystallinum. Plant Physiol Biochem 2002; 40:669 - 677
- Leegood RC, Lea PJ, Adcock MD, Hausler RE. The regulation and control of photorespiration. J Exp Bot 1995; 46:1397 - 1414
- Wingler A, Lea PJ, Quick WP, Leegood RC. Photorespiration: metabolic pathways and their role in stress protection. Philos Trans R Soc Lond B Biol Sci 2000; 355:1517 - 1529
- Ghannoum O. C4 photosynthesis and water stress. Ann Bot 2009; 103:635 - 644
- Cross AR, Erichson R, Ellis BA, Curnutte JT. Spontaneous activation of NADPH oxidase in cellfree system: unexpected multiple effects of magnesium ion concentrations. Biochem J 1993; 38:229 - 233
- Hernandez JA, Ferrer MA, Jimenez A, Barcelo AR, Sevilla F. Antioxidant systems and O2·-/H2O2 production in the apoplast of pea leaves. Its relation with salt-induced necrotic lesions in minor veins. Plant Physiol 2001; 127:817 - 831
- Lin CC, Kao CH. Cell wall peroxidase activity, hydrogen peroxide level and NaCl-inhibited root growth of rice seedlings. Plant Soil 2001; 230:135 - 143
- Mazel A, Leshem Y, Tiwari BS, Levine A. Induction of salt and osmotic stress tolerance by overexpression of an intracellular vesicle trafficking protein AtRab7 (AtRabG3e). Plant Physiol 2004; 134:118 - 128
- Tsai YC, Hong CY, Liu LF, Kao CH. Expression of ascorbate peroxidase and glutathione reductase in roots of rice seedlings in response to NaCl and H2O2. J Plant Physiol 2005; 162:291 - 299
- Fry IV, Huflejt M, Erber WWA, Peschek GA, Packer L. The role of respiration during adaptation of the freshwater cyanobacterium Synechococcus 6311 to salinity. Arch Biochem Biophys 1986; 244:686 - 691
- Moser D, Nicholls P, Wastyn M, Peschek GA. Acidic cytochrome c6 of unicellular cyanobacteria is an indispensable and kinetically competent electron donor to cytochrome oxidase in plasma and thylakoid membranes. Biochem Int 1991; 24:757 - 768
- Jeanjean R, Matthijs HCP, Onana B, Havaux M, Joset F. Exposure of the cyanobacterium Synechocystis PCC6803 to salt stress induces concerted changes in respiration and photosynthesis. Plant Cell Physiol 1993; 34:1073 - 1079
- Cushman JC, Bohnert HJ. Molecular genetics of Crassulacean acid metabolism. Plant Physiol 1997; 113:667 - 676
- Noctor G, Veljovic-Jovanovic S, Driscoll S, Novitskaya L, Foyer C. Drought and oxidative load in the leaves of C3 plants: A predominant role for photorespiration?. Ann Bot 2002; 89:841 - 850
- Griffiths H. Luttge U. Carbon dioxide concentrating mechanisms and the evolution of CAM in vascular epiphytes. Vascular plants as epiphytes 1989; Berlin Springer-Verlag 42 - 86
- Borland A, Elliott S, Patterson S, Taybi T, Cushman J, Pater B, Barnes J. Are the metabolic components of crassulacean acid metabolism upregulated in response to an increase in oxidative burden?. J Exp Bot 2006; 57:319 - 328
- Slesak I, Libik M, Miszalski Z. The foliar concentration of hydrogen peroxide during salt-induced C3-CAM transition in Mesembryanthemum crystallinum L. Plant Sci 2008; 174:221 - 226
- Miszalski Z, Slesak I, Niewiadomska E, Baczek-Kwinta R, Luttge U, Ratajczak R. Subcellular localization and stress responses of superoxide dismutase isoforms from leaves in the C3-CAM intermediate halophyte Mesembryanthemum crystallinum L. Plant Cell Environ 1998; 21:169 - 179
- Shalata A, Tal M. The effect of salt stress on lipid peroxidation and antioxidants in the leaf of the cultivated tomato and its wild salt-tolerant relative Lycopersicon pennellii. Physiol Plant 1998; 104:169 - 174
- Shalata A, Mittova V, Volokita M, Guy M, Tal M. Response of the cultivated tomato and its wild salt-tolerant relative Lycopersicon pennellii to salt-dependent oxidative stress: The root antioxidative system. Physiol Plant 2001; 112:487 - 494
- Mittova V, Tal M, Volokita M, Guy M. Upregulation of the leaf mitochondrial and peroxisomal antioxidative systems in response to salt-induced oxidative stress in the wild salt-tolerant tomato species Lycopersicon pennellii. Plant Cell Environ 2003; 26:845 - 856
- Sumithra K, Jutur PP, Carmel BD, Reddy AR. Salinity-induced changes in two cultivars of Vigna radiata: responses of antioxidative and proline metabolism. Plant Growth Regul 2006; 50:11 - 22
- Sekmen AH, Turkan I, Takio S. Differential responses of antioxidative enzymes and lipid peroxidation to salt stress in salt-tolerant Plantago maritima and salt-sensitive Plantago media. Physiol Plant 2007; 131:399 - 411
- Eltayeb AE, Kawano N, Badawi GH, Kaminaka H, Sanekata T, Shibahara T, et al. Overexpression of monodehydroascorbate reductase in transgenic tobacco confers enhanced tolerance to ozone, salt and polyethylene glycol stresses. Planta 2007; 225:1255 - 1264
- Prashanth SR, Sadhasivam V, Parida A. Overexpression of cytosolic copper/zinc superoxide dismutase from a mangrove plant Avicennia marina in indica Rice var Pusa Basmati-1 confers abiotic stress tolerance. Transgenic Res 2008; 17:281 - 291
- Kim Y, Arihara J, Nakayama T, Nakayama N, Shimada S, Usui K. Antioxidative responses and their relation to salt tolerance in Echinochloa oryzicola Vasing and Setaria virdis L. Beauv. Plant Growth Regul 2004; 44:87 - 92
- Bowler C, Montagu MV, Inze D. Superoxide dismutases and stress tolerance. Annu Rev Plant Physiol Plant Mol Biol 1992; 43:83 - 116
- Asada K. Ascorbate peroxidase-a hydrogen peroxide scavenging enzyme in plants. Physiol Plant 1992; 85:235 - 241
- Willekens H, Chamnongpol S, Davey M, Schraudner M, Langebartels C, Van Montagu M, et al. Catalase is a sink for H2O2 and is indispensable for stress defense in C3 plants. EMBO J 1997; 10:1723 - 1732
- Noctor G, Foyer CH. Ascorbate and glutathione: Keeping active oxygen under control. Ann Rev Plant Physiol Plant Mol Biol 1998; 49:249 - 279
- Cavalcanti FR, Oliveira JTA, Martins-Miranda AS, Viegas RA, Silveira JAG. Superoxide dismutase, catalase and peroxidase activities do not confer protection against oxidative damage in salt-stressed cowpea leaves. New Phytol 2004; 163:563 - 571
- Bajji M, Kinet J-M, Lutts S. Salt stress effects on roots and leaves of Atriplex halimus L. and their corresponding callus cultures. Plant Sci 1998; 137:131 - 142
- Huang C, He W, Guo J, Chang X, Su P, Zhang L. Increased sensitivity to salt stress in an ascorbate-deficient Arabidopsis mutant. J Exp Bot 2005; 56:3041 - 3049
- Athar H, Khan A, Ashraf M. Exogenously applied ascorbic acid alleviates salt-induced oxidative stress in wheat. Environ Exp Bot 2008; 63:224 - 231
- Munné-Bosh S, Schwarz K, Alegre L. Enhanced formation of α-tocopherol and highly oxidized abietane diterpenes in water stressed rosemary plants. Plant Physiol 1999; 121:1047 - 1052
- Munné-Bosch S. The role of alpha-tocopherol in plant stress tolerance. J Plant Physiol 2005; 162:743 - 748
- Siefermann-Harms D. The light-harvesting and protective functions of carotenoids in photosynthetic membranes. Physiol Plant 1987; 69:561 - 568
- Parida AK, Das AB. Salt tolerance and salinity effects on plants: a review. Ecotoxicol Environ Safety 2005; 60:324 - 349
- Giraud E, Ho LHM, Clifton R, Carroll A, Estavillo G, Tan YF, et al. The absence of alternative oxidase1a in Arabidopsis results in acute sensitivity to combined light and drought stress. Plant Physiol 2008; 147:595 - 610
- Purvis AC. Role of the alternative oxidase in limiting superoxide production by plant mitochondria. Physiol Plant 1997; 100:165 - 170
- Costa JH, Jolivet Y, Hasenfratz-Sauder M-P, Orellano EG, Lima MS, Dizengremel P, de Melo DF. Alternative oxidase regulation in roots of Vigna unguiculata cultivars differing in drought/salt tolerance. J Plant Physiol 2007; 164:718 - 727
- Lutts S, Almansouri M, Kinet J-M. Salinity and water stress have contrasting effects on the relationship between growth and cell viability during and after stress exposure in durum wheat callus. Plant Sci 2004; 167:9 - 18
- Malkin R, Niyogi K. Buchanan B, Gruissem W, Jones R. Photosynthesis. Biochemistry and Molecular Biology of plant. Am Soc Plant physiol 2000; 591 - 592