Abstract
Eukaryotic organisms rely on intricate signaling networks to connect recognition of microbes with the activation of efficient defense reactions. Accumulating evidence indicates that phospholipids are more than mere structural components of biological membranes. Indeed, phospholipid-based signal transduction is widely used in plant cells to relay perception of extracellular signals. Upon perception of the invading microbe, several phospholipid hydrolyzing enzymes are activated that contribute to the establishment of an appropriate defense response. Activation of phospholipases is at the origin of the production of important defense signaling molecules, such as oxylipins and jasmonates, as well as the potent second messenger phosphatidic acid (PA), which has been shown to modulate the activity of a variety of proteins involved in defense signaling. Here, we provide an overview of recent reports describing the different plant phospholipase pathways that are activated during the establishment of plant defense reactions in response to pathogen attack.
In plant cells, perception of pathogenic microbes largely relies on transmembrane pattern recognition receptors that specifically recognize highly conserved pathogen-derived molecules called PAMPs/MAMPs (pathogen-/microbial-associated molecular patterns), such as bacterial flagellin.Citation1 PAMP recognition by the plant leads to basal defense responses. A second layer of defense is based on the recognition of specific pathogen-derived molecules, called effectors, primarily by an additional class of plant cytoplasmic receptor proteins [nucleotide-binding leucine-rich repeat (NB-LRR) proteins] but also by protein receptors predicted to be located at the plasma membrane [receptor-like proteins (RLPs) and receptor-like kinases (RLKs)]. This recognition leads to the activation of plant immune responses that are frequently associated to the development of hypersensitive cell death (HR) at the inoculation site, which has been shown to contribute to plant resistance.Citation2
The activation of plant immunity involves a variety of early signaling events, including rapid accumulation of reactive oxygen species (ROS), changes in cellular ion fluxes, activation of protein kinase cascades, changes in gene expression and production of stress-related hormones.Citation3,Citation4 During recent years, a substantial number of reports have also shown the importance of lipids and lipid-related molecules, including glycerolipids, sphingolipids, fatty acids, oxylipins, jasmonates and sterols, in the regulation of plant defense responses.Citation5
Phospholipids are more than structural components in biological membranes. Indeed, evidence that phospholipases and phospholipid-derived molecules are involved in plant signaling, and more particularly in plant immunity, is rapidly accumulating.Citation6,Citation7 In plants, phosphatidic acid (PA) can be produced from phospholipids by phospholipase D (PLD) enzymes or from diacylyglycerol (DAG) by DAG kinases (DGKs) in the phospholipase C (PLC) pathway. PA is a potent secondary signal messenger molecule that modulates the activity of kinases, phosphatases, phospholipases and proteins involved in membrane-trafficking, Ca2+ signaling and the oxidative burst.Citation8,Citation9 In addition, a growing body of evidence indicates that phospholipase A (PLA) [and related molecules such as lysophospholipids (LPLs) and free fatty acids (FFAs)] and phospholipase C (PLC) (and its related molecules DAG and DGK) play important roles in the control of the plant defense response to the attack by invading pathogens.Citation7
Here, we review the recent advances in understanding phospholipase-mediated signaling and its importance in the control of plant immune responses.
Phospholipase A
Members of the phospholipase A (PLA) superfamily catalyze the hydrolysis of membrane phospholipids at the sn-1 (PLA1) or sn-2 position (PLA2) of the glycerol backbone to produce free fatty acids (FFAs) and lysophospholipids (LPLs) (). In contrast to the case of animal and yeast cells, the major components of plant cell membranes are not phospholipids but galactolipids. In agreement with this observation, plants encode enzymes that are able to hydrolyze galactolipids. However, most of these enzymes, which are called LAHs (Lipid Acyl Hydrolases), are also able to act on phospholipids, but not on triacylglycerol (TAG). In Arabidopsis, PLAs can be classified in three main groups: (1) the patatin-like proteins, which present non-specific LAH activity and are able to hydrolyze both at sn-1 and sn-2 positions of phospholipids and galactolipids, (2) the DAD (Defective in Anther Dehiscence)-like proteins with LAH and TAG lipase activity and (3) the secretory PLA2s that specifically hydrolyze phospholipids at their sn-2 position. For most plant PLAs, substrate specificities and cleavage positions are still poorly characterized.
In Arabidopsis, protein complexes containing EDS1 (Enhanced Disease Susceptibility1), PAD4 (Phytoalexin Deficient4) and SAG101 (Senescence-Associated Gene101) constitute an essential regulatory node that mediates both effector-triggered activation of several TIR-NB-LRR proteins and basal defense responses.Citation10 Interestingly, EDS1, PAD4 and, less convincingly, SAG101 have homology to LAHs. However, no enzymatic activity has been reported to date for any of these proteins and it has been thus suggested that, as described for other signaling proteins, they may play a structural rather than an enzymatic role during plant immunity.Citation10
LAHs have been mainly linked to plant immunity through their role in oxylipin and jasmonic acid (JA) biosynthesis, molecules with well-studied effects on plant defense responses.Citation11,Citation12 Oxylipins and jasmonates are synthesized from FFAs liberated from membrane phospholipids or galactolipids by deacylating enzymes, and oxidized by a lipoxygenase (LOX) or α-dioxygenase (α-DOX).Citation11,Citation13 Besides their role in oxylipin or JA biosynthesis, induction of PLA2 activity during pathogen defense has also been correlated with ROS production.Citation14 In addition, in elicitor-treated cells of Californian poppy, lysophosphatidylcholine (LPC), which is transiently generated by PLA2, was proposed to activate a vacuolar H+/Na+ antiporter to decrease the cytosolic pH and activate phytoalexin biosynthesis.Citation15,Citation16
Patatins.
Patatins were first described as vacuolar storage proteins in potato tubers.Citation17 Patatin-like proteins (pPLAs) are present in many plant species and have been implicated in plant defense signaling through JA or oxylipin accumulation. For example, Arabidopsis plants mutated in pPLAI, encoding a protein with a patatin-type catalytic domain, showed increased resistance to inoculation by the necrotrophic pathogen Botrytis cinerea. These plants also displayed reduced basal levels of JA, but the pathogen-induced production of JA was not modified.Citation18 How the decrease in JA basal levels is linked to enhanced resistance to Botrytis is still unclear.
The expression of an additional member of the Arabidopsis pPLA family, pPLA-IIα/PLP2, is induced after infection with B. cinerea or Pseudomonas syringae pv. tomato.Citation19 Altered expression of PLP2 in Arabidopsis modifies plant susceptibility to Botrytis and Pseudomonas and to treatment with paraquat, a cell death-promoting molecule. Indeed, PLP2 expression favored the development of necrotizing pathogens, and increased resistance to the obligate pathogen cucumber mosaic virus.Citation19,Citation20 In response to Botrytis infection, PLP2 expression induces late accumulation of oxylipins through the α-DOX pathway,Citation20 which are thought to limit HR spreading. PLP2 would thus participate to the execution of plant cell death through non-specific hydrolysis of membrane lipids followed by cell death spreading controlled by oxylipins. In this scenario, PLP2 activity may be exploited by pathogens with different lifestyles to facilitate host colonization.
The expression of three tobacco patatin-like proteins (NtPAT1–3) was rapidly induced, preceding JA accumulation, during the HR triggered in response to tobacco mosaic virus (TMV).Citation21 These enzymes display PLA2 activity and may contribute to FFA production for JA biosynthesis. In the elicitor-infiltrated zone, NtPAT PLA2 activity was rapidly induced before JA accumulation and cell death appearance.Citation22 Activation of NtPAT1–3 and 9-LOX was detected in cryptogein-elicited tobacco leavesCitation23 and a patatin-like and a 9-LOX protein were co-expressed in cotton leaves following infection with Xanthomonas campestris.Citation24 Taken together, these results suggest that patatins may contribute to oxylipin biosynthesis though their metabolic association with LOXs ().
DAD-like proteins.
DAD1 encodes a chloroplastic PLA1 that initiates JA synthesis in stamens after release of free linolenic acid from membrane phospholipids. The dad1 mutant is thus impaired in pollen development and anther dehiscence.Citation25 No clear evidence that DAD1-like proteins play a role in the regulation of plant immunity has been reported to date, although a recent report indicates that expression of the DAD-like LAHs PLA1-Iγ1 and PLA1-Iγ2 was induced after infection with B. cinerea and P. syringae, while PLA1-III was only induced after treatment with Botrytis.Citation26 However, following Botrytis or Pseudomonas infection, knock-out lines in these three DAD1-like genes exhibited similar resistance level and JA profiles, compared to wild-type plants.
Secretory PLA2s.
The Arabidopsis secretory PLA2 family (AtsPLA2) consists of four isoforms denoted AtsPLA2-α, -β, -γ and -δ.Citation27 Although animal sPLA2s have been previously implicated in host defense,Citation28 evidence of the role of sPLA2s in plant immunity is more limited. AtsPLA2-α, which controls auxin transport protein trafficking to the plasma membrane,Citation29 has been recently involved in non-enzymatic control of plant defense. Consistent with the presence of a signal peptide in its N-terminus, AtsPLA2-α was localized intracellularly in Golgi-associated vesicles and later secreted to the extracellular space.Citation29,Citation30 However, in the presence of the transcription factor AtMYB30, a positive regulator of Arabidopsis defense reactions, AtsPLA2-α is partially relocalized to the nucleus, where both proteins interact. This protein interaction leads to repression of the AtMYB30-mediated transcriptional activity and negative regulation of plant HR and defense responses ().Citation30 The regulation of plant defense mediated by AtsPLA2-α is independent of its enzymatic activity, suggesting that PLA functions may extend beyond their lipid hydrolyzing activity, as previously described for several animal sPLAs.Citation28
Phospholipase D
Phospholipases D (PLDs) catalyze the hydrolysis of structural phospholipids, such as phosphatidylcholine (PC) and phosphatidylethanolamine (PE), to produce phosphatidic acid (PA) and the respective headgroup (). PA, which can also be produced via the PLC pathway (see below), has emerged as a crucial second messenger in the regulation of numerous cellular functions, including the signaling pathways associated to plant defense ().Citation7 While humans present two PLD enzymes, and yeast only one, PLDs are overrepresented in plants. For example, Arabidopsis and rice encode 12 and 17 PLDs, respectively.Citation31,Citation32 Plant PLDs have been classified in 6 classes (α, β, γ, δ, ε and ζ), depending on their sequence homology and in vitro enzymatic activity.Citation31
PLD activity has been associated to a variety of stress responses in plants.Citation6 Activation of PLD activity during plant defense was first described in rice, after infection by Xanthomonas oryzae.Citation33 Interestingly, PLD was recruited to the plasma membrane at the point where bacteria attack the cell. Elicitor treatment of tomato,Citation34,Citation35 tobaccoCitation36 or rice cellsCitation37 were also found to induce PLD activity. In Arabidopsis, expression of the α, β and γ class of PLD genes is induced after infiltration by both virulent and avirulent strains of P. syringae.Citation38 After recognition of the P. syringae avirulence proteins AvrRpm1 and AvrRpt2 in Arabidopsis, a biphasic accumulation of PA was observed. The first wave of PA was attributed to the PLC/DGK pathway (see below) whereas a later and higher peak of PA was found to be due to PLD activity.Citation39
The effects of PLD activation during plant-pathogen interactions are varied. Indeed, PA has been shown to induce ROS productionCitation40,Citation41 and activate defense-relatedCitation37 or ethylene-responsive genes.Citation42 PLDs also participate in salicylic acid-dependent signaling.Citation43 In contrast, LePLDβ1 is induced after elicitor treatment in tomato and is a negative regulator of ROS production.Citation44 Similarly, OsPLDβ1-knockdown plants spontaneously activate defense responses, suggesting that OsPLDβ1 would function as a negative regulator of disease resistance.Citation45 Several hypotheses have been formulated to explain the differential regulation of plant defense displayed by distinct PLD family members. PLDs may be localized in different subcellular compartmentsCitation46,Citation47 and, depending on their subcellular localization, they may generate different molecular species of PA,Citation48 probably modifying the interactions between PA and its target proteins. In addition, PLDs display different biochemical properties with regard to their requirement for Ca2+ or substrate phospholipids, for instance.Citation49
An interesting link has been made in Arabidopsis between PLA2 and PLD activities, during the regulation of plant defense. In Arabidopsis plants of the Pi-0 ecotype infected with P. syringae expressing the AvrBst effector, PA is produced through the PLD pathway, which is necessary for the establishment of resistance and HR.Citation50 This resistance phenotype displayed by Pi-0 plants is due to a loss of function mutation in SOBER1, a conserved α/β hydrolase with PLA2 activity.Citation51 Conversely, in the susceptible Col-0 Arabidopsis ecotype, SOBER1 PLA2 activity would compete with PLD for substrate availability, thereby reducing PA accumulation in response to AvrBst elicitation. These findings underline the importance of PA in the regulation of plant defense signaling.
Phospholipase C
Plant PLC is a plasma membrane protein and its activity requires calcium. Depending on the calcium concentration, PLC is able to use different substrates. At low (micromolar) Ca2+ concentrations, both phosphatidylinositol-4-phosphate (PI4P) and phosphatidylinositol4,5-bisphosphate (PIP2) are hydrolyzed, whereas at higher (millimolar) Ca2+ concentrations, PLC preferentially uses phosphatidylinositol (PI). As PLC was first reported to hydrolyze PIP2 both in vitro and in vivo, it was assumed that PLC uses predominantly PIP2 as substrate. However, plant cell membranes have no or very little PIP2. Conversely, there is plenty of PI4P in the plasma membrane, with at least one additional pool occurring in the Golgi. Therefore, it has been proposed that PI4P is very likely the in vivo substrate of PLC.Citation6 Hydrolysis of PLC substrates leads to the production of inositol 1,4,5-trisphosphate (IP3) and DAG ( and ). While DAG remains in the plasma membrane, IP3 diffuses into the cytosol, where it induces the release of Ca2+ from intracellular store(s), most probably from the vacuole.Citation7 IP3 may be rapidly converted into IP6, which is also able to trigger an increase in cytosolic Ca2+ levels. In animal cells, DAG activates members of the protein kinase C (PKC) superfamily. However, in plants, which lack PKC, DAG is rapidly phosphorylated to PA through the action of the DGK ().Citation34,Citation52 One of the main features of a second messenger signal is its transient nature. PA may thus be phosphorylated into diacylglycerol pyrophosphate (DGPP) by the PA kinase (PAK). Whether the formation of DGPP is just a mechanism for attenuating PA signaling or whether it also acts as a signaling molecule remains to be elucidated.Citation6 Although eukaryotic PLCs have been classified in six subfamilies, all plant PLCs belong to the PLCζ class. The Arabidopsis genome contains nine PLCs, three of which have been characterized,Citation53–Citation55 and seven DGK genes.
The PLC pathway responds to a variety of stress-induced signals from specific membrane receptors, which perceive signaling molecules such as hormones or pathogen elicitors. In particular, PAMP recognition triggers the activation of the PLC pathway, supporting the idea that PLC-mediated signaling is involved in basal defense responses.Citation34,Citation56 Expression of the rice gene OsPLC1 was shown to be induced by diverse chemical and biological inducers of plant defense pathways and during the incompatible interaction between rice and the pathogen fungus Magnaporthe grisea, suggesting that OsPLC1 might be involved in the activation of the signaling pathway leading to disease resistance in rice.Citation57 As mentioned above, recognition of the Pseudomonas avirulence proteins AvrRpm1 or AvrRpt2 induced a biphasic accumulation of PA, with the first wave attributed to PLC/DGK activities.Citation39 In tomato (Solanum lycopersicum) cell cultures expressing the cognate Cf-4 resistance gene, the race-specific elicitor Avr4 from the pathogenic fungus Cladosporium fulvum rapidly induces accumulation of PA, via the sequential activity of PLC and DGK.Citation52 Intriguingly, accumulation of PA is associated with ROS production, potentially via direct activation of an NADPH oxidase, contributing to the defense response.Citation52,Citation58 More recently, the tomato PLC isoform, SlPLC4 was shown to be required for full Cf-4/Avr4 recognition.Citation59 Interestingly, an additional tomato PLC isoform, SlPLC6, is not involved in the establishment of the Cf-4/Avr4-mediated HR but rather in general plant defense responses against various pathogens. Therefore, a differential requirement of PLC isoforms in plant immune responses has been proposed.Citation59 In agreement with this hypothesis, Arabidopsis PLC proteins display differential organ expression and it has been reported that most of the PLC genes are induced in response to diverse environmental stimuli and, particularly, during plant defense responses.Citation60 Similarly, expression of DGKs is induced during different environmental stresses including microbial elicitation, suggesting that DGK, as a generator of PA, has an important role in plant basal resistance.Citation61,Citation62
It is noteworthy that, beyond the previously described effect of PA in inducing ROS production,Citation40,Citation41,Citation52 PA also mediates the induction of additional pathogen-induced signaling components (). For example, CTR1, a protein kinase known to repress plant ethylene responses triggered by biotic and abiotic stress stimuli, is negatively regulated by PA in vitro. Therefore, stress-induced PA formation might trigger downstream ethylene responses via its interaction with CTR1. Accumulation of stressinduced PA would thus represent an alternative way to induce ethylene-mediating resistance responses in the absence of ethylene.Citation63 Additionally, the Arabidopsis protein kinase AtPDK1 is activated through PA binding, triggering the subsequent activation of the kinase AtAGC2-1.Citation64 AtAGC2-1 is also known as OXI1, a kinase that mediates oxidative burst signaling and acts upstream of a MAP kinase cascade involved in basal resistance against Hyaloperonospora arabidopsidis.Citation65 This observation strongly suggests that PA may contribute to the activation of plant basal defense through MAP kinase signaling.
Conclusions and Perspectives
Significant advances have been made during the last decade in elucidating phospholipid-based signaling in plants. Molecular, biochemical and genetic studies have allowed uncovering the important role played by lipids in the intricate signaling network triggered in plant cells after pathogen recognition. More particularly, a growing body of data indicates that phospholipases and phospholipid-derived molecules play crucial roles in the modulation of plant defense responses through their action on the generation of ROS, activation of protein kinases, Ca2+ signaling, hormone production and defense-related gene activation, for example.
Despite the technical difficulties associated to measuring and manipulating transient second messenger signaling components, the role of some phospholipase-derived molecules in plant defense, in particular PA, is now well established. The reported identification of PA targetsCitation66 should allow a better understanding of its function during plant-pathogen interactions. In addition, the identification of LAHs with non-enzymatic functionsCitation10,Citation30 and their importance for the regulation of plant defense are also intriguing findings that need to be better understood in the future.
What are the upstream signal(s) that trigger activation of phospholipases following pathogen recognition? How is phospholipase activity regulated? What are the cellular targets that are activated by phospholipases and phospholipid-derived molecules? What are the molecular mechanisms that allow integrating phospholipase activities in the complex signaling pathways that lead to the establishment of plant defense? Are pathogens able to manipulate host phospholipid signaling for their own benefit and, if so, what are the mechanisms involved? Providing answers to these and other questions remains an important challenge for future research.
Figures and Tables
Figure 1 Schematic representation of phospholipid structure and the sites of cleavage by phospholipases. (A) General structure of a phospholipid, with two fatty acyl chains linked to a glycerol backbone, at the sn-1 and sn-2 positions, and a phosphate group creating the «phosphatidyl» moiety to which a variable head group is attached. The sites of phospholipase activity are indicated by arrows. Lipid acyl hydrolases (LAHs) are able to hydrolyze phospholipids at sn-1 and sn-2 positions. (B) Possible head groups and resulting phospholipids are indicated: PLA, phospholipase A; PLC, phospholipase C; PLD, phospholipase D; Fatty Acid 1, fatty acid in sn-1 position; Fatty Acid 2, fatty acid in sn-2 position; P, phosphate group.
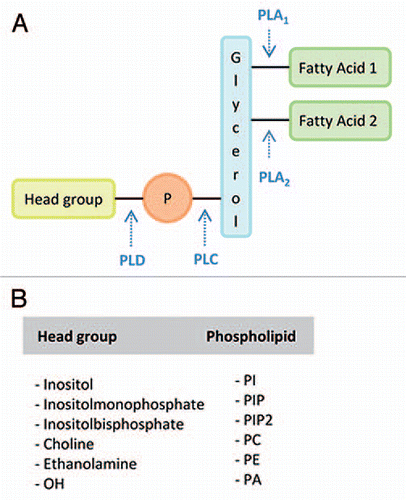
Figure 2 Model of phospholipase signaling in plant defense responses. Pathogen recognition induces the activation of phospholipase-mediated signaling pathways. Phospholipase A (PLA) hydrolyzes membrane phospholipids (PL) to produce free fatty acids (FFA) and lysophospholipids (LPL). PLAs are classified in three main groups: (1) the patatin-like proteins, responsible for the production of oxilipins and jasmonates, (2) the DAD (Defective in Anther Dehiscence)-like proteins with, to date, no well-established role in plant immunity and (3) the secretory PLA2s. Phospholipids from the plasma membrane, as well as from various intracellular membranes (mitochondria, Golgi, chloroplast…), are potential substrates for PLA activity. EDS1, PAD4 and SAG101 are essential immunity components that shuttle across the nuclear envelope to regulate defense responses. Despite their sequence homology to LAHs, the fact that no enzymatic activity has been demonstrated to date for these proteins suggests that their LAH domain may play a structural role rather than an enzymatic role. Similarly, a non-enzymatic function has been shown for AtsPLA2-α, a negative regulator of plant defense that is translocated into the nucleus in the presence of the transcription factor AtMYB30. Phospholipase C (PLC) is a plasma membrane protein that hydrolyzes phosphatidylinositol (PI), phosphatidylinositol bisphosphate (PIP2) or phosphatidylinositol phosphate (PI4P) to produce inositol trisphosphate (IP3) and diacylglycerol (DAG). IP3, which may be converted to IP6, diffuses in the cytosol where it releases Ca2+ from internal stores whereas DAG is rapidly converted to phosphatidic acid (PA) through the action of diacylglycerol kinase (DGK). Increased PA levels modulate additional signaling components, which are indicated in the figure. PA signaling may be attenuated via its phosphorylation to diacylglycerol pyrophosphate (DGPP) by a PA kinase (PAK). Finally, PLD also generates PA through the hydrolysis of structural phospholipids, such as phosphatidylcholine (PC) or phosphatidyl-ethanolamine (PE). PLDs present different subcellular localizations and may thus use phospholipids from the plasma membrane or from intracellular membranes.
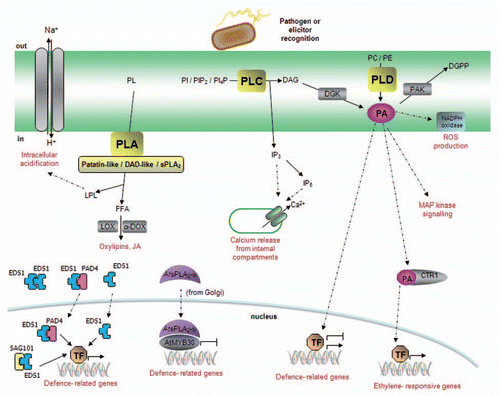
References
- Zipfel C. Pattern-recognition receptors in plant innate immunity. Curr Opin Immunol 2008; 20:10 - 16
- Jones JD, Dangl JL. The plant immune system. Nature 2006; 444:323 - 329
- Rivas S, Thomas CM. Molecular interactions between tomato and the leaf mold pathogen Cladosporium fulvum. Annu Rev Phytopathol 2005; 43:395 - 436
- Boller T, Felix G. A renaissance of elicitors: perception of microbe-associated molecular patterns and danger signals by pattern-recognition receptors. Annu Rev Plant Biol 2009; 60:379 - 406
- Shah J. Lipids, lipases and lipid-modifying enzymes in plant disease resistance. Annu Rev Phytopathol 2005; 43:229 - 260
- Munnik T, Testerink C. Plant phospholipid signaling: “in a nutshell”. J Lipid Res 2009; 50:260 - 265
- Laxalt A, Munnik T. Phospholipid signaling in plant defence. Curr Opin Plant Biol 2002; 5:332 - 338
- Munnik T. Phosphatidic acid: an emerging plant lipid second messenger. Trends Plant Sci 2001; 6:227 - 233
- Wang X. Lipid signaling. Curr Opin Plant Biol 2004; 7:329 - 336
- Feys BJ, Wiermer M, Bhat RA, Moisan LJ, Medina-Escobar N, Neu C, et al. Arabidopsis SENESCENCE-ASSOCIATED GENE101 stabilizes and signals within an ENHANCED DISEASE SUSCEPTIBILITY1 complex in plant innate immunity. Plant Cell 2005; 17:2601 - 2613
- Blee E. Impact of phyto-oxylipins in plant defense. Trends Plant Sci 2002; 7:315 - 322
- Turner JG, Ellis C, Devoto A. The jasmonate signal pathway. Plant Cell 2002; 14:153 - 164
- Feussner I, Wasternack C. The lipoxygenase pathway. Annu Rev Plant Biol 2002; 53:275 - 297
- Chandra S, Heinstein PF, Low PS. Activation of phospholipase A by plant defense elicitors. Plant Physiol 1996; 110:979 - 986
- Viehweger K, Dordschbal B, Roos W. Elicitor-activated phospholipase A(2) generates lysophosphatidylcholines that mobilize the vacuolar H(+) pool for pH signaling via the activation of Na(+)-dependent proton fluxes. Plant Cell 2002; 14:1509 - 1525
- Viehweger K, Schwartze W, Schumann B, Lein W, Roos W. The Galpha protein controls a pH-dependent signal path to the induction of phytoalexin biosynthesis in Eschscholzia californica. Plant Cell 2006; 18:1510 - 1523
- Hendriks T, Vreugdenhil D, Stiekema WJ. Patatin and four serine proteinase inhibitor genes are differentially expressed during potato tuber development. Plant Mol Biol 1991; 17:385 - 394
- Yang W, Devaiah SP, Pan X, Isaac G, Welti R, Wang X. AtPLAI is an acyl hydrolase involved in basal jasmonic acid production and Arabidopsis resistance to Botrytis cinerea. J Biol Chem 2007; 282:18116 - 18128
- La Camera S, Geoffroy P, Samaha H, Ndiaye A, Rahim G, Legrand M, Heitz T. A pathogen-inducible patatin-like lipid acyl hydrolase facilitates fungal and bacterial host colonization in Arabidopsis. Plant J 2005; 44:810 - 825
- La Camera S, Balague C, Gobel C, Geoffroy P, Legrand M, Feussner I, et al. The Arabidopsis patatin-like protein 2 (PLP2) plays an essential role in cell death execution and differentially affects biosynthesis of oxylipins and resistance to pathogens. Mol Plant Microbe Interact 2009; 22:469 - 481
- Dhondt S, Geoffroy P, Stelmach BA, Legrand M, Heitz T. Soluble phospholipase A2 activity is induced before oxylipin accumulation in tobacco mosaic virus-infected tobacco leaves and is contributed by patatin-like enzymes. Plant J 2000; 23:431 - 440
- Dhondt S, Gouzerh G, Muller A, Legrand M, Heitz T. Spatio-temporal expression of patatin-like lipid acyl hydrolases and accumulation of jasmonates in elicitor-treated tobacco leaves are not affected by endogenous levels of salicylic acid. Plant J 2002; 32:749 - 762
- Cacas JL, Vailleau F, Davoine C, Ennar N, Agnel JP, Tronchet M, et al. The combined action of 9 lipoxygenase and galactolipase is sufficient to bring about programmed cell death during tobacco hypersensitive response. Plant Cell Environm 2005; 28:1367 - 1378
- Cacas JL, Marmey P, Montillet JL, Sayegh-Alhamdia M, Jalloul A, Rojas-Mendoza A, et al. A novel patatin-like protein from cotton plant, GhPat1, is co-expressed with GhLox1 during Xanthomonas campestris-mediated hypersensitive cell death. Plant Cell Rep 2009; 28:155 - 164
- Ishiguro S, Kawai-Oda A, Ueda J, Nishida I, Okada K. The DEFECTIVE IN ANTHER DEHISCIENCE gene encodes a novel phospholipase A1 catalyzing the initial step of jasmonic acid biosynthesis, which synchronizes pollen maturation, anther dehiscence and flower opening in Arabidopsis. Plant Cell 2001; 13:2191 - 2209
- Grienenberger E, Geoffroy P, Mutterer J, Legrand M, Heitz T. The interplay of lipid acyl hydrolases in inducible plant defense. Plant Signal Behav 2010; 5:1181 - 1186
- Lee HY, Bahn SC, Shin JS, Hwang I, Back K, Doelling JH, Ryu SB. Multiple forms of secretory phospholipase A2 in plants. Prog Lipid Res 2005; 44:52 - 67
- Boyanovsky BB, Webb NR. Biology of secretory phospholipase A2. Cardiovasc Drugs Ther 2009; 23:61 - 72
- Lee O, Kim S, Kim H, Hong J, Ryu S, Lee S, et al. Phospholipase A2 is required for PIN-FORMED protein trafficking to the plasma membrane in the Arabidopsis root. Plant Cell 2010; 6:1812 - 1825 DOI: tpc.110.074211v1-tpc.110
- Froidure S, Canonne J, Daniel X, Jauneau A, Briere C, Roby D, Rivas S. AtsPLA2-alpha nuclear relocalization by the Arabidopsis transcription factor AtMYB30 leads to repression of the plant defense response. Proc Natl Acad Sci USA 2010; 107:15281 - 15286
- Wang X. Regulatory functions of phospholipase D and phosphatidic acid in plant growth, development and stress responses. Plant Physiol 2005; 139:566 - 573
- Li G, Lin F, Xue HW. Genome-wide analysis of the phospholipase D family in Oryza sativa and functional characterization of PLD beta1 in seed germination. Cell Res 2007; 17:881 - 894
- Young SA, Wang X, Leach JE. Changes in the plasma membrane distribution of rice phospholipase D during resistant interactions with Xanthomonas oryzae pv. oryzae. Plant Cell 1996; 8:1079 - 1090
- van der Luit AH, Piatti T, van Doorn A, Musgrave A, Felix G, Boller T, Munnik T. Elicitation of suspension-cultured tomato cells triggers the formation of phosphatidic acid and diacylglycerol pyrophosphate. Plant Physiol 2000; 123:1507 - 1516
- Laxalt AM, ter Riet B, Verdonk JC, Parigi L, Tameling WI, Vossen J, Haring M, et al. Characterization of five tomato phospholipase D cDNAs: rapid and specific expression of LePLDbeta1 on elicitation with xylanase. Plant J 2001; 26:237 - 247
- Suzuki K, Yano A, Nishiuchi T, Nakano T, H K, Yamaguchi K, Shinshi H. Comprehensive analysis of early response genes to two different microbial elicitors in tobacco cells. Plant Sci 2007; 173:291 - 301
- Yamaguchi T, Minami E, Ueki J, Shibuya N. Elicitor-induced activation of phospholipases plays an important role for the induction of defense responses in suspension-cultured rice cells. Plant Cell Physiol 2005; 46:579 - 587
- de Torres Zabela M, Fernandez-Delmond I, Niittyla T, Sanchez P, Grant M. Differential expression of genes encoding Arabidopsis phospholipases after challenge with virulent or avirulent Pseudomonas isolates. Mol Plant Microbe Interact 2002; 15:808 - 816
- Andersson MX, Kourtchenko O, Dangl JL, Mackey D, Ellerstrom M. Phospholipase-dependent signaling during the AvrRpm1- and AvrRpt2-induced disease resistance responses in Arabidopsis thaliana. Plant J 2006; 47:947 - 959
- Sang Y, Cui D, Wang X. Phospholipase D and phosphatidic acid-mediated generation of superoxide in Arabidopsis. Plant Physiol 2001; 126:1449 - 1458
- Yamaguchi T, Minami E, Shibuya N. Activation of phospholipases by N-acetylchitooligosaccharide elicitor in suspension-cultured rice cells mediates reactive oxygen generation. Physiol Plant 2003; 118:361 - 370
- Testerink C, Larsen PB, McLoughlin F, van der Does D, van Himbergen JA, Munnik T. PA, a stress-induced short cut to switch-on ethylene signaling by switching-off CTR1?. Plant Signal Behav 2008; 3:681 - 683
- Krinke O, Flemr M, Vergnolle C, Collin S, Renou JP, Taconnat L, et al. Phospholipase D activation is an early component of the salicylic acid signaling pathway in Arabidopsis cell suspensions. Plant Physiol 2009; 150:424 - 436
- Bargmann BO, Munnik T. The role of phospholipase D in plant stress responses. Curr Opin Plant Biol 2006; 9:515 - 522
- Yamaguchi T, Kuroda M, Yamakawa H, Ashizawa T, Hirayae K, Kurimoto L, et al. Suppression of a phospholipase D gene, OsPLDbeta1, activates defense responses and increases disease resistance in rice. Plant Physiol 2009; 150:308 - 319
- Fan L, Zheng S, Cui D, Wang X. Subcellular distribution and tissue expression of phospholipase Dalpha, Dbeta and Dgamma in Arabidopsis. Plant Physiol 1999; 119:1371 - 1378
- Kusner DJ, Barton JA, Qin C, Wang X, Iyer SS. Evolutionary conservation of physical and functional interactions between phospholipase D and actin. Arch Biochem Biophys 2003; 412:231 - 241
- Hong Y, Zheng S, Wang X. Dual functions of phospholipase Dalpha1 in plant response to drought. Mol Plant 2008; 1:262 - 269
- Qin C, Wang C, Wang X. Kinetic analysis of Arabidopsis phospholipase Ddelta. Substrate preference and mechanism of activation by Ca2+ and phosphatidylinositol-4,5-biphosphate. J Biol Chem 2002; 277:49685 - 49690
- Kirik A, Mudgett MB. SOBER1 phospholipase activity suppresses phosphatidic acid accumulation and plant immunity in response to bacterial effector AvrBsT. Proc Natl Acad Sci USA 2009; 106:20532 - 20537
- Cunnac S, Wilson A, Nuwer J, Kirik A, Baranage G, Mudgett MB. A conserved carboxylesterase is a SUPPRESSOR OF AVRBST-ELICITED RESISTANCE in Arabidopsis. Plant Cell 2007; 19:688 - 705
- De Jong C, Laxalt A, Bargmann B, De Wit P, Joosten M, Munnik T. Phosphatidic acid accumulation is an early response in the Cf-4/Avr4 interaction. Plant J 2004; 39:1
- Hirayama T, Mitsukawa N, Shibata D, Shinozaki K. AtPLC2, a gene encoding phosphoinositide-specific phospholipase C, is constitutively expressed in vegetative and floral tissues in Arabidopsis thaliana. Plant Mol Biol 1997; 34:175 - 180
- Yamamoto YT, Conkling MA, Sussex IM, Irish VF. An Arabidopsis cDNA related to animal phosphoinositide-specific phospholipase C genes. Plant Physiol 1995; 107:1029 - 1030
- Hirayama T, Ohto C, Mizoguchi T, Shinozaki K. A gene encoding a phosphatidylinositol-specific phospholipase C is induced by dehydration and salt stress in Arabidopsis thaliana. Proc Natl Acad Sci USA 1995; 92:3903 - 3907
- Legendre L, Yueh YG, Crain R, Haddock N, Heinstein PF, Low PS. Phospholipase C activation during elicitation of the oxidative burst in cultured plant cells. J Biol Chem 1993; 268:24559 - 24563
- Song F, Goodman RM. Molecular cloning and chracterization of a rice phosphoinositide-specific phospholipase C gene, OsPI-PLC1, that is activated in systemic acquired resistance. Physiol Mol Plant Pathol 2002; 61:31 - 40
- Laxalt AM, Raho N, Have AT, Lamattina L. Nitric oxide is critical for inducing phosphatidic acid accumulation in xylanase-elicited tomato cells. J Biol Chem 2007; 282:21160 - 21168
- Vossen JH, Abd-El-Haliem A, Fradin EF, van den Berg GC, Ekengren SK, Meijer HJ, et al. Identification of tomato phosphatidylinositol-specific phospholipase-C (PI-PLC) family members and the role of PLC4 and PLC6 in HR and disease resistance. Plant J 2010; 62:224 - 239
- Meijer HJ, Munnik T. Phospholipid-based signaling in plants. Annu Rev Plant Biol 2003; 54:265 - 306
- Arisz SA, Testerink C, Munnik T. Plant PA signaling via diacylglycerol kinase. Biochim Biophys Acta 2009; 1791:869 - 875
- den Hartog M, Verhoef N, Munnik T. Nod Factor and elicitors activate different phospholipid signaling pathways in suspension-cultured alfalfa cells. Plant Physiol 2003; 132:311 - 317
- Testerink C, Larsen PB, van der Does D, van Himbergen JA, Munnik T. Phosphatidic acid binds to and inhibits the activity of Arabidopsis CTR1. J Exp Bot 2007; 58:3905 - 3914
- Anthony RG, Henriques R, Helfer A, Meszaros T, Rios G, Testerink C, et al. A protein kinase target of a PDK1 signaling pathway is involved in root hair growth in Arabidopsis. EMBO J 2004; 23:572 - 581
- Rentel MC, Lecourieux D, Ouaked F, Usher SL, Petersen L, Okamoto H, et al. OXI1 kinase is necessary for oxidative burst-mediated signaling in Arabidopsis. Nature 2004; 427:858 - 861
- Testerink C, Dekker HL, Lim ZY, Johns MK, Holmes AB, Koster CG, et al. Isolation and identification of phosphatidic acid targets from plants. Plant J 2004; 39:527 - 536