Abstract
Leaf senescence is an organized process, which requires fine tuning between nuclear gene expression, activity of proteases and the maintenance of primary metabolism. Recently, we reported that leaf senescence was accompanied by an early degradation of the microtubule cytoskeleton in Arabidopsis thaliana. As the cytoskeleton is essential for cell stability, vesicle shuttling and organelle mobility, it might be asked how the regulation of these cell functions occurs with such drastic modifications of the cytoskeleton. Based on confocal laser microscopy observations and a micro-array analysis, the following addendum shows that mitochondrial mobility is conserved until the late stages of leaf senescence and provides evidences that the actin-cytoskeleton is maintained longer than the microtubule network. This conservation of actin-filaments is discussed with regards to energy metabolism as well as calcium signaling during programmed cell death.
Early Degradation of Microtubules during Leaf Senescence
Etymologically, senescence means “to grow old”. However, in plants, leaf senescence can be the result of natural aging as well as abiotic/biotic stresses. Therefore, its understanding represents a fundamental scientific challenge, notably in the present conjuncture, when improving crop yields becomes crucial for feeding our fast growing population. The primary function of this complex and organized process is the retrieval and reallocation of valuable nutrients towards other organs of the plant. In order to make such a process possible, an accurate regulation of the metabolism is needed to readjust fluxes and to fulfill the energetic demand of the cell after the loss of photosynthetically active chloroplasts. However, although most cells from the senescing leaf are in an actively remodeling environment, we recently reported an intriguing event: the early degradation of the microtubule (MT) lattice in the epidermis and mesophyll cells of Arabidopsis thaliana.Citation1 This discovery raised two main questions (1) what are the molecular mechanisms responsible for such degradation and in a longer term (2) how do cells fulfill their metabolic functions with such a reduced MT network. To address the first question, we primarily assumed that although MT assembly is energy dependent, the lack of ATP/GTP was not responsible for the disorganization of the MT lattice as we previously showed mitochondria to provide sufficient ATP to maintain cellular functions until the end of the process of senescence.Citation2,Citation3 Instead, we showed that disruption of the MT network was concomitant with the repression of both genes encoding the tubulin subunits and genes encoding nine bundling/stabilizing Microtubule-Associated Proteins MAP65 (MAP65-1 to MAP65-9). Yet, we also noticed a strong induction of the gene encoding the severing microtubule-associated protein MAP18.Citation1 MAP18 has been shown to depolymerize microtubules in vitroCitation4 but its function remains unclear as a recent study concluded that MAP18 was a plasma membrane associated Ca2+-binding protein that strongly interacts with several phosphatidylinositol phosphates, in a calcium-calmodul-independent manner.Citation5 Interestingly, a strong induction of MAP18 was similarly observed in individually-darkened leaves and in leaves from entirely darkened plants. However, while a disruption of the MT network was observed in the epidermis and mesophyll cells from leaves undergoing dark-induced senescence, the MT network was rather well conserved in leaves from entirely darkened plant.Citation1 Therefore, although MAP18/PCaP2 (as renamed by Kato et al.Citation5) is very likely to be involved in the regulation of cellular functions including stress response and cytoskeletal reorganization, we concluded that its high induction during leaf senescence was not sufficient to trigger the complete degradation of the MT network. We consequently proposed that early degradation of the MT network during leaf senescence resulted from a combined repression of the genes encoding tubulin subunits and bundling/stabilizing MAP65 proteins, as well as induction of the gene encoding the destabilizing protein MAP18. In addition, p60 katanin, a MT severing protein,Citation6 and two of its potential targeting subunits (p80s) did not seem to be involved in the degradation of the MT network during leaf senescence and were instead proposed to contribute to the reorganization of the MTs in response to prolonged darkness.Citation1
Mitochondrial Mobility is Conserved Until the Latest Stages of Leaf Senescence
In the light of these new findings and since the dynamic assembly and disassembly of the MTs is essential for cell stability and survival, we are currently questioning how vesicle trafficking and organelle mobility, for instance, are achieved in cells having a drastically reduced MT network. In an attempt to elucidate this question, we monitored the mobility of very active organelles, mitochondria, in epidermis cells from leaves undergoing natural senescence. As shown in and B, the number of mitochondria (GFP-labeled, Logan et al.Citation7) was drastically reduced towards the late stages of natural leaf senescence. This is in line with our previous work, where an important diminution of the number of mitochondria was observed in leaves subjected to dark-induced senescence.Citation3 However, although the MT network was highly altered in leaves having lost 75% to 85% of their chlorophyll content,Citation1 a distinct motility of mitochondria could still be observed (estimated during 40 seconds as shown in and D), suggesting a conservation of cytoplasmic streaming until the last stage of leaf senescence.
Differential Regulation of the Cytoskeletal Components
The plant cytoskeleton is made of two main components: the microtubules and the actin filaments. In plants, the positioning of mitochondria has been associated with both actin and tubulin cytoskeleton.Citation8–Citation10 However, plant mitochondria are thought to move predominantly on actin filaments, using myosin as motor proteins.Citation11–Citation13 This process is often referred to as cytoplasmic streaming.Citation14 Since we found mitochondrial motility to be conserved during leaf senescence, it was hypothesized that genes encoding different components of the cytoskeleton could be differentially regulated. Using publicly available micro-array data performed with leaves undergoing natural senescence,Citation15 the expression values were extracted for the genes encoding tubulin, kinesin motor-proteins and MAPs for microtubules as well as the genes encoding actin lato sensu, myosin motor-proteins and actin-binding proteins (profilin, villin and fimbrin) for actin filaments. These data were expressed as the log2 of the ratio between t25 t50 or t75 and the expression value of the gene at t0 (t0 being a 6 week-old leaf and t25, t50 and t75 being leaves with a loss of 25%, 50% and 75% of their chlorophyll content, respectively). Shown in , genes encoding tubulins and kinesins were under represented in the cluster of positively regulated genes (2C) during natural senescence while genes encoding actin, villin and myosin were under-represented in the cluster of negatively regulated genes (2A). This clearly indicates that the actin filaments, their myosin motor-proteins and the villin/profilin actin-binding proteins are more prone to stability and upregulation than their microtubule-related counterparts. Taken together, these results highlight the differential regulation of genes encoding different components of the cytoskeleton and provide convincing evidence for a more conserved and active actin-cytoskeleton than MT-cytoskeleton during leaf senescence.
In 2003, Smertenko et al.Citation16 suggested the F-actin to be crucial for the embryonic programmed cell death in the gymnosperm Picea abies as actin-filaments disappeared significantly later than the MTs. During leaf senescence, a conserved and functional actin-cytoskeleton would be essential to allow a proper metabolic efficiency of the remaining mitochondria, mainly for maintaining the primary metabolism until the complete arrest of metabolic activity prior to cell death. However, we may also question whether the interaction actin-filaments/mitochondria could act as signaling agents regulating the death of cells notably by modulating Ca2+ concentrations.Citation17
Figures and Tables
Figure 1 Representative mature rosette leaves undergoing natural senescence. t0 represents a 7 week-old leaf (control); t25, t50 and t75 represent leaves with 25%, 50% and 75% of their chlorophyll content degraded, respectively. The mobility of β-ATPase-GFP mitochondriaCitation7 was monitored with a Leica SP2 confocal laser microscope during 40 s in the epidermis of t0 leaf (A) and t75 leaf (B) and the estimated mitochondrial mobility of t0 and t75 is represented in (C and D), respectively.
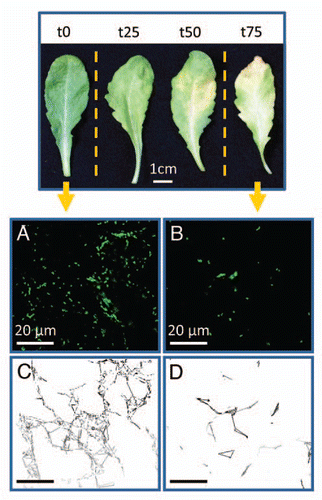
Figure 2 Analysis of transcript abundance of genes encoding cytoskeleton-related proteins during natural senescence. Fold changes (from extracted dataCitation15) are expressed as the log2 of the ratio between t25 t50 or t75 and t0. 207 genes were hierarchically clustered and 2 subclusters were defined with genes that were consistently down (red) or up (blue) regulated. The pie diagrams represent the percentage distribution of gene families within the subclusters of downregulated genes (A), of upregulated genes (C) and within the entire subset of genes covered by this study (B).
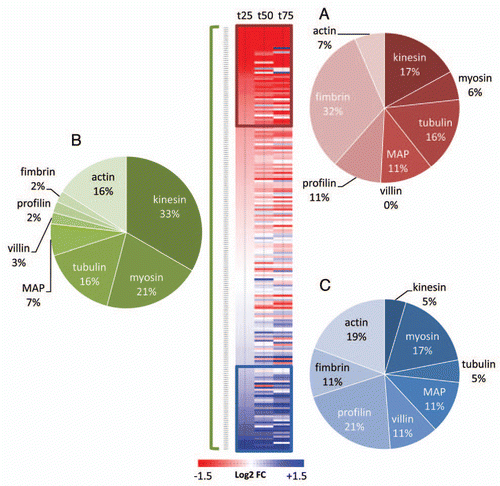
Addendum to:
References
- Keech O, Pesquet E, Gutierrez L, Ahad A, Bellini C, Smith SM, et al. Leaf senescence is accompanied by an early disruption of the microtubule network in Arabidopsis thaliana. Plant Physiol 2010; 110:163402
- Keskitalo J, Bergquist G, Gardeström P, Jansson S. A Cellular Timetable of Autumn Senescence. Plant Physiol 2005; 139:1635 - 1648
- Keech O, Pesquet E, Ahad A, Askne A, Nordvall DAG, Vodnala SM, et al. The different fates of mitochondria and chloroplasts during dark-induced senescence in Arabidopsis leaves. Plant, Cell Environ 2007; 30:1523 - 1534
- Wang X, Zhu L, Liu B, Wang C, Jin L, Zhao Q, et al. Arabidopsis MICROTUBULE-ASSOCIATED PROTEIN18 Functions in Directional Cell Growth by Destabilizing Cortical Microtubules. Plant Cell 2007; 19:877 - 889
- Kato M, Nagasaki-Takeuchi N, Ide Y, Maeshima M. An Arabidopsis Hydrophilic Ca2+-Binding Protein with a PEVK-Rich Domain, PCaP2, is Associated with the Plasma Membrane and Interacts with Calmodulin and Phosphatidylinositol Phosphates. Plant Cell Physiol 2010; 51:366 - 379
- Burk DH, Liu B, Zhong R, Morrison WH, Ye ZH. A Katanin-like Protein Regulates Normal Cell Wall Biosynthesis and Cell Elongation. Plant Cell 2001; 13:807 - 828
- Logan DC, Leaver CJ. Mitochondria-targeted GFP highlights the heterogeneity of mitochondrial shape, size and movement within living plant cells. J Exp Bot 2000; 51:865 - 871
- Van Gestel K, Kohler RH, Verbelen JP. Plant mitochondria move on F-actin, but their positioning in the cortical cytoplasm depends on both F-actin and microtubules. J of Exp Bot 2002; 53:659 - 667
- Logan DC. Mitochondrial fusion, division and positioning in plants. Biochem Soc T 2010; 038:789 - 795
- Romagnoli S, Cai G, Faleri C, Yokota E, Shimmen T, Cresti M. Microtubule- and Actin Filament-Dependent Motors are Distributed on Pollen Tube Mitochondria and Contribute Differently to Their Movement. Plant Cell Physiol 2007; 48:345 - 361
- Wada M, Suetsugu N. Plant organelle positioning. Curr Opin Plant Biol 2004; 7:626 - 631
- Peremyslov VV, Prokhnevsky AI, Avisar D, Dolja VV. Two class XI myosins function in organelle trafficking and root hair development in arabidopsis. Plant Physiol 2008; 146:1109 - 1116
- Zheng M, Beck M, Müller J, Chen T, Wang X, Wang F, et al. Actin Turnover Is Required for Myosin-Dependent Mitochondrial Movements in Arabidopsis Root Hairs. PLoS ONE 2009; 4:5961
- Shimmen T, Yokota E. Cytoplasmic streaming in plants. Curr Opin Cell Biol 2004; 16:68 - 72
- van der Graaff E, Schwacke R, Schneider A, Desimone M, Flugge UI, Kunze R. Transcription Analysis of Arabidopsis Membrane Transporters and Hormone Pathways during Developmental and Induced Leaf Senescence. Plant Physiol 2006; 141:776 - 792
- Smertenko AP, Bozhkov PV, Filonova LH, Arnold Sv, Hussey PJ. Re-organisation of the cytoskeleton during developmental programmed cell death in Picea abies embryos. Plant J 2003; 33:813 - 824
- Wang Y, Zhu Y, Ling Y, Zhang H, Liu P, Baluska F, et al. Disruption of actin filaments induces mitochondrial Ca2+ release to the cytoplasm and [Ca2+] changes in Arabidopsis root hairs. BMC Plant Biol 2010; 10:53