Abstract
A glycosylated arginase acting as a fungal lectin from Peltigera canina is able to produce recruitment of cyanobiont Nostoc cells and their adhesion to the hyphal surface. This implies that the cyanobiont would develop organelles to motility towards the chemoattractant. However when visualized by transmission electron microscopy, Nostoc cells recently isolated from P. canina thallus do not reveal any motile, superficial organelles, although their surface was covered by small spindles and serrated layer related to gliding. The use of S-(3,4-dichlorobenzyl)isothiourea, blebbistatin, phalloidin and latrunculin A provide circumstantial evidence that actin microfilaments rather than MreB, the actin-like protein from prokaryota, and, probably, an ATPase which develops contractile function similar to that of myosin II, are involved in cell motility. These experimental facts, the absence of superficial elements (fimbriae, pili or flagellum) related to cell movement, and the appearance of sunken cells during of after movement verified by scanning electron microscopy, support the hypothesis that the motility of lichen cyanobionts could be achieved by contraction-relaxation episodes of the cytoskeleton induced by fungal lectin act as a chemoattractant.
Introduction
Lichens are intimate and long-term specific symbioses of photosynthetic algae or cyanobacteria and heterotrophic fungi joined to form a new biological entity different from its individual components.Citation1 Specificity required for the lichen association can be defined in this context as the preferential, but not exclusive, association of a biont with another.Citation2 Recognition of compatible algal cells is performed by specific lectins produced and secreted by the potential mycobiont.Citation3 Some lectins from phycolichens are glycosylated arginases which bind to an algal cell wall receptor identified as an α-1,4-polygalactosylated urease.Citation4 The binding is enhanced by Ca2+ and Mn2+, in a similar way to that described for legume lectins.Citation5 Since recognition must be achieved even for new algae produced inside the thallus after cell division, evolution of symbiotic relationships implies then the synchronization of cell division and lectin receptor production, probably as a consequence of the perception of environmental factors, such as light and temperature.Citation6,Citation7 The role of fungal lectins in the establishment of the lichen association requires two different locations for glycosylated arginase: in the cell wall of fungal hyphae as a particulated glycoprotein where it participates in cell-cell interactions between chloro- and mycobiont;Citation8 and near the surface of fungal hyphae, where it is secreted and produces recruitment of compatible algal cells.Citation5 In this way, eventual death of some cells during the first steps of association is balanced by increasing the number of cells near the surface of hyphae.
A similar pattern of recognition has been described for cyanolichens. Kardish et al.Citation9 described a lectin isolated from the mycobiont of Nephroma laevigatum that binds to Nostoc cells from different origins, and this lectin seems to be involved in the control of the symbiotic balance in the lichen thallus. Lectins have also been isolated from the mycobionts of some Peltigera species.Citation10,Citation11 In P. aphthosa, a similar lectin is required for the initiation of cephalodia in a highly specific manner.Citation12 Cyanobiont specificity was shown using inoculation of foreign Nostoc strains into cephalodia of P. aphthosa.Citation13 Recently, a glycosylated arginase acting as a fungal lectin has been reported to bind to Nostoc from P. caninaCitation14 and Leptogium corniculatumCitation15 to produce recruitment and adhesion of cyanobiont cells to the hyphal surface. To complete this last step of the process, lectin-recruited cells must move toward the hyphal surface to establish cell-to-cell adhesive contacts, which are mediated by binding of the particulated lectins to free binding sites in the cyanobiont cell wall surface. One hypothesis is that the fungal lectin acts as a chemoattractant to recruit cyanobiont cells. Although the knowledge of cyanobacterial chemotaxis in plant or lichen associations is scarce, there is some evidence supporting this hypothesis. Dick and StewartCitation16 described discrete fimbriae in Nostoc cyanobionts isolated from P. canina when grown on N2; these authors speculated that these non-flagellar appendages could be involved in cell motility.
On the other hand, Nostoc cells from the liverwort Blasia pusilla appear as small, undifferentiated, motile (gliding) cells (“hormogonia”).Citation17 These cellular morphologies may promote cellular dispersal. The reason for this morphological change remains unknown, although it can be hypothesized that the host produces a hormogonial-inducing-factor (HIF) that diffuses to the medium and interacts with Nostoc cells,Citation18 modulating their morphology and/or motile properties. In this regard, Vivas suggest that Nostoc hormogonia produce the lectin receptor in cyanobionts isolated from L. corniculatum. This is consistent with the putative role of hormogonia in the dispersal of Nostoc cells inside the thallus from the initial filament.Citation19 Several hormogonia separate from the pluricellular filament and migrate between fungal hyphae to establish in a new site inside the thallus. Due to the possibility of dispersion, hormogonia can hardly be visualized in recently isolated Nostoc from P. canina thalli, in agreement with our experimental results.Citation14 Mature, symbiotic cyanobacteria do not require continuous recognition by their fungal partner initially; however, recognition would be required to re-establish specific cell-to-cell contact if the initial contact with their mycobiont is lost. However, heterocysts do not contain the fungal lectin receptor in their cell walls.Citation14 Consistent with this, a high frequency of heterocysts follows increased hormogonia formation in free-living filaments in response to nitrogen-limited or starved Nostoc from Anthoceros punctatus.Citation20 This is likely to increase the frequency and rate of infection by Nostoc spp. because the gametophyte tissue requires a source of fixed nitrogen for continued growth. In addition to diffusible HIF, the host plant produces other chemoattractants. Chemoattraction toward exudates or extracts of natural host and non-host plants and some sugars has been reported in Nostoc cells;Citation21 in the same experiments, flavonoids and amino acids had no effect.
It is currently unknown whether formation of pili is required for cell gliding. Hormogonia from Calothrix express genes that promote pili differentiation during their formation.Citation22 Likewise, a type IV pilus system mediates pili formation on the surface of hormogonium of N. punctiforme; in this system, mutations in pili-like genes altered surface piliation and reduced symbiotic competency.Citation23 Similarly, mutation of genes pilB1 and pilT1 of Synechocystis result in a complete loss of motility and acquisition of non-piliated and hyperpiliated phenotypes, respectively;Citation24 pilB1 is involved in type IV pili assembly whereas pilT1 promotes pili disassembly.
However, cell motility without peripheral motile structures probably requires the assistance of cytoplasmic organelles. Although cytoskeleton has not been well studied so far in prokaryotic cells, the presence of actin-like proteins has been reported in both bacteria and cyanobacteria, developing functions related to chromosome segregation, cell motility in the absence of locomotive appendages and the maintenance the cell shape.Citation25,Citation26 Bacterial cell division requires microtubule protein FtsZ although perhaps not as a cytoskeletal protein. The shape of Escherichia coliCitation27,Citation28 and CaulobacterCitation29 is controlled by an actin-like protein, MreB, which also mediates segregation of Caulobacter chromosome.Citation30 Accordingly, the depletion of MreB causes defects in the cell shape of Bacillus subtilisCitation31 and E. coli. The occurrence of other actin-like proteins has also been reported in some cyanobacteria, such as Spirulina platensis.Citation32 This protein contains conserved epitopes corresponding to actin regions known to interacts with cross-linking proteins. They are also reported for Anabaena cylindrica and A. variabilis.Citation33 In a similar way to that found for bacteria, MreB depletion in Anabaena sp. drastically modifies the cell shape of this cyanobacteria.Citation34
In this study, we attempt to prove that fungal lectin is involved in the process as the host chemoattractant and to elucidate the mechanism, involving MreB or other actin-like proteins, through which Nostoc chemotaxis is developed.
Results
Nostoc cyanobacteria undergo chemotaxis toward a secreted arginase lectin from Peltigena canina
To study the chemotaxis of the Nostoc cyanobacteria, cells isolated from P. canina thalli were attracted toward a capillary containing 10 µL of a solution of 1.0 mg mL−1 fungal lectin (0.16 units of arginase activity), purified as described in Material and Methods, and cells within the capillary quantified at different time points. A peak of cell accumulation was observed after 8 h of incubation, decreasing thereafter (). We thus selected this time point to perform a dose-response analysis. We found a semi-Gaussian response to different doses of the chemoattractant, with a peak at 0.75 mg mL−1 of purified arginase (), which is in agreement with classical models of chemotactic responses to diffusion-controlled chemical gradients. We then investigated whether chemotaxis induced morphological modifications in the migrating cells, e.g., formation of fimbriae, since, initially, Nostoc cells recently isolated from P. canina thalli did not exhibit any superficial motile structures (). Thus, specialized motile filaments known as hormogonia were not visualized in these cyanobacterial preparations.
To do this, we used SEM to visualize cells recovered from the lectin-containing capillary (migratory) and freshly isolated cells not exposed to the chemoattractant (non-migratory). We observed that non-migratory cells did not exhibit pili or fimbriae, although their surface was covered by small spindles (). Conversely, migratory cyanobacteria associated in multicellular groups and were covered by an exocellular, mucilaginous sheath that seemed to keep the multicellular aggregates together ( and D). In some cases, very small protrusions were observed, but they were small and did not display the features of fully formed fimbriae (). Cytoplasmic protrusions have also been described for plant cells, i.e., in tobacco cell suspensions, as a consequence of force changes generated after actin filament depolimerization.Citation35 In summary, chemotaxis toward the lectin does not induce robust fimbriae formation although it is accompanied by cell association in nascent filaments.
Nostoc displacement does not involve MereB protein.
Some actin-like proteins or MreB have been described in free-living cyanobacteria such as SpirulinaCitation32 and Anabaena.Citation34 Thus, assay of Nostoc motility toward Peltigera lectin was performed by including in the medium two different concentrations of S-(3,4-dichlorobenzyl)isothiourea, A22, a well-known MreB inhibitor.Citation36 Contrary to that expected, as shown in , A22 at a concentration of 20 µM did not prevent the movement of cells Nostoc toward the source of the lectin. Identical results were obtained by using a concentration of A22 five times higher that the above used, 100 µM (data not shown). However, MreB occurrence and distribution was studied by incubating the cells recovered from the capillary containing the lectin with FITC-phalloidin. Most of these cells exhibited very low intensity of fluorescence emission suggesting that MreB has not been polymerized after the reception of lectin signaling. Even some of these cells recovered from the capillary exhibited red fluorescence due to chlorophylls ().
Nostoc chemotaxis toward arginase requires actinand myosin II-like proteins.
Actin and actin-organizing proteins such as α-actinin have been described in cyanobacteria.Citation32 To gain insight in their role of in chemotaxis to the lectin, Nostoc cells were incubated with the actin inhibitor phalloidin. Two different concentrations of phalloidin, 0.095 µM and 0.19 µM, inhibited chemotaxis by 50% (). Interestingly, when phalloidin (0.19 µM) was combined with the eukaryotic myosin II inhibitor blebbistatin (25 µM), the inhibition was more acute (78%), suggesting that blebbistatin may target a chemotaxis-related molecular target in cyanobacteria (). This was further suggested by independent experiments in which concentrations of blebbistatin from 10 µM to 25 µM produced a 30% inhibition of chemotaxis in 8 h of incubation (). No significant differences of motility inhibition were found for these two blebbistatin concentrations at 8 h treatment although, at the start of the experiment, 25 µM blebbistatin produced 40% of chemotaxis inhibition whereas no effect was found for 10 µM drug ().
To further study the possible involvement of polymerization of actin-like proteins in this process, the cells were incubated with 10 µM latrunculin A, which sequesters eukaryotic G-actin and prevents polymerization. Latrunculin A inhibited chemotaxis by 44%, suggesting that dynamic polymerization/depolymerization of actin-like proteins was involved in the motility of Nostoc cells ().
Chemotactic arginase does not polarize its surface receptor in Nostoc cells, but promotes actin asymmetry.
To investigate whether chemotaxis induces a polarized redistribution of the ligand on the surface of the migrating Nostoc cells, purified arginase from P. canina was labeled with FITC, using it for the chemotaxis assay. After 4 h, vegetative and individualized Nostoc cells were found inside the capillary that showed intense and homogeneous emission of green fluorescence from their surface, suggesting that the chemoattractant binds to a receptor that is evenly distributed on the surface of Nostoc. Furthermore, many of these cells formed filaments, although neither heterocysts nor hormogonia could be observed (). Addition of 25 µM blebbistatin significantly inhibited the movement of cells toward the lectin but did not impair binding of the FITC-labeled lectin. Since chemotaxis seemed to be the basis of motility, it could be concluded that blebbistatin significantly impeded motility induced by chemotaxis. However, lower number of filaments, absent from the recently isolated cells population, were observed (). Finally, combination of 25 µM blebbistatin and 0.19 µM phalloidin in the incubation buffer, motility of cells toward the lectin is drastically inhibited and few, but always individual cells can be recovered from the capillary ().
The actin-like targets of phalloidin were then visualized by incubating the cells recovered from the capillary with FITC-phalloidin. Most of these cells exhibited asymmetric staining (red arrows in ), suggesting that the actin-like backbone of these cells distributes asymmetrically. Also in this case, inhibitors significantly reduced the number of cells showing an actin asymmetric distribution (). Actin, or an actin-like protein, was really involved in cyanobacterial movement since 10 µM latrunculin A impeded in some extent the binding of phalloidin to the lectin-chemoattracted Nostoc cells ().
Discussion
Activation and chemotaxis of lichen photobionts induced by fungal lectins cause cell aggregation. The glycoprotein is able to recruit both algal cells (chlorobionts) and cyanobacteria (cyanobionts), forming high affinity bonds with different galactose units in the poly-α-1,4-galactoside side chain of the lectin receptor on the cell wall of different photobionts.Citation5,Citation15 This implies that these lectins must be able to attract different algae or cyanobacteria in the corresponding cell suspension. In this study, we have identified a chemotropic lectin from P. canina. The motile behavior induced by this lectin is chemotropic because: (1) Nostoc differentiate motile cells which enter in the capillary containing the lectin, according to that described for Nostoc cells from the lichen P. canina,Citation16 the liverwort Blasia pusilla,Citation17 the hornwort Leiosporoceros dussiiCitation37 or the angiosperm Gunnera manicata,Citation21 although neither fimbriae or pili have been observed nor filaments are formed before chemotropic movement; (2) the motile response follows a Gaussian distribution ( and ), reaching a peak at intermediate concentrations and declining thereafter. This behavior is thought to involve either receptor or signaling desensitization.Citation38
In migrating eukaryotic cells, the chemotactic response elicits the formation of specialized, actin-driven structures, such as filopodia, lamellipodia, etc., which drive cell motility.Citation39 Similarly, Dick and Stewart described thin protrusions or fimbriae, on the surface of motile cells when they are growing in medium containing gaseous N2 as the sole source of nitrogen. Then, the absence of pili when cyanobionts are included in the lichen thallus could be deduced from this assertion, but authors do not bring any experimental probe about this. Based on this, the hypothesis that vegetative Nostoc cells and heterocysts inside the lichen thallus do not generate motile organelles has been observed ( and ).
Some free-living algal cells, such as Porphyra pulchellaCitation40 emit pseudopodia and display actin- and myosin-dependent amoeboid and gliding motility. Such motion is hypothesized to be tension- and contraction-dependent. One mechanism by which these cells generate tension is through the linkage of the end-actin microfilament to their binding proteins immobilized the cell wall, which causes actin microfilaments to generate the physical forces required for cell motility.Citation41 The other mechanism is through molecular motors. Myosin II generates contractile forces through ATPase-dependent sliding of actin filaments. In mammalian cells, it is central in the control of cell adhesion, cell migration and tissue architecture.Citation42 Myosin II is also implicated in several types of morphogenetic movements in plant cells, such as polarization of the pollen tubes.Citation43 However, actin is absent from prokaryotic cells.Citation44 One of its prokaryotic ancestor, MreB, a major component of the bacterial cytoskeleton, exhibits high structural homology to its eukaryotic counterpart actin. Live cell microscopy studies suggest that MreB molecules organize into large filamentous spirals that support the cell membrane and play a key shape-determining function. MreB exhibited rates of ATP hydrolysis and phosphate release similar to that of F-actin, however, with a critical concentration of approximately 3 nM, which is approximately 100-fold lower than that of actin.Citation45 A22 binds MreB with at least micromolar affinity in its nucleotide-binding pocket in a manner that is sterically incompatible with simultaneous ATP binding. Nevertheless, MreB is not involved in Nostoc movement effected by the lectin. A22, an inhibitor which negatively affects both the time course and extent of MreB polymerization in vitro in the presence of ATP,Citation46 prevents the assembly of MreB polymers but it does not inhibit the entry of Nostoc cells in the capillary containing Peltigera arginase (). Consequently, changes of cell shape, such as polar cell invagination, which accompanied motility () cannot be dependent on MreB polymerization. If polymeric MreB was formed during the overall process, these polymers must be revealed by using phalloidin as marker.Citation47
The lack of peripheral, motile organelles in mature, vegetative cells of Nostoc recently isolated from the lichen thallus suggests that their movement can be catalogs as chemotropism induced by the lichen lectinCitation17 since chemotaxis necessarily implies motile organelles. Filamentous cyanobacteria, including short, broken filaments and Nostoc hormogonia, move by gliding on a surfaceCitation48,Citation49 being this gliding generated for forces of slime extrusion and surface waves.Citation50 Movement occurs in a direction parallel to the cell's long axis, is associated with the production of polysaccharide slime and requires attachment of the cell to a surface. The outer membrane is important to the process.
In the absence of fimbriae, cells displacement can also be achieved by gliding, since hair-like fiber, serrated external layer and peptidoglycanCitation49 are also visualized as components of the Nostoc cell wall () but the invagination shown as associated to the movement cannot be explained on this basis. Cell invagination would require successive contraction-relaxation episodes of the cellular cytoskeleton to generate the shape changes observed during chemotropic displacement. This probably implicates the generation of an intracellular force, different from slime extrusion and surface waves, by the mechanisms described above. The surprising result that both blebbistatin ( and ) and phalloidin/latrunculin A () inhibit Nostoc chemotropism suggests two very exciting facts. First, the actin-like proteins present in Nostoc cells are sensitive to drugs that target actin polymerization/depolymerization in eukaryotic cells, suggesting a very similar rearrangement of the cellular cytoskeleton. In this way, the actin-like protein from symbiotic Nostoc involved in cell displacement must be considered closest to the eukaryotic actin than that to MreB protein of prokaryotes. In this regard, the bacterial actin MreB of Anabaeba sp. is required for cell shape determination and heterocyst formation. Similar proteins could be the targets of phalloidin and latrunculin A in Nostoc cells. Second, the effect of blebbistatin is harder to interpret. Its high specificity for the ATPase pocket of myosin II suggests the presence of an ATPase with homology to the motor domain of myosin II involved in Nostoc chemotropic behavior. This will be vigorously pursued in our lab.
However, it is tempting to speculate that the interaction between contractile ATPases and actin-like proteins could underlie the invagination of initially spherical cells. In this sense, it is useful to state that sunken Nostoc cells have never been observed inside of the thallus. I could not think that invagination is an effect of dehydration, since many cells within the capillary have regained its spherical shape. This suggests a cycle of sinking-rounding in response to chemoattractant. Repeated cycles of invagination/rounding (similar to the dynamics of swimming jellyfish) would generate the propulsive force required to promote cell displacement. In this regard, contractile deformation is neither observed in non-stimulated cells in the absence of the lectin, nor in cells that have reached the center of the gradient (defined by the capillary that creates the radial gradient of chemoattractant). These data are consistent with a model proposed in , in which initially spherical cells “sense” the chemoattractant gradient and generate a contractile invagination opposing the highest concentration of the gradient ( and ). The cycle would be repeated until the cells reach an area with high concentration of the gradient, which would cause desensitization and inhibit the contraction/relaxation cycle, stopping motility (). A similar mechanism has been proposed for the social amoeba Dictyostelium discoideum, which exhibits a robust contractile actomyosin apparatus at the trailing edge to propel contractile motion toward the gradient,Citation39 although, in this case, chlorinated alkylphenones, instead of a glycosylated protein, showing activity as differentiation-inducing factor-1 act as chemotaxis effectors through specific receptor different from that related to cell differentiation.Citation51
A main difference between that previously observed for L. corniculatum and the results described herein consists in the presence of Nostoc filaments, including heterocysts and hormogonia, in L. corniculatumCitation15,Citation52 and the absence of these filaments of Nostoc in P. canina thalli. Hormogonia are not only motile cellsCitation23 but also cells specialized in vegetative reproduction.Citation11,Citation53 Their differentiation is induced by several factors, such as changes in the chemical environment of the cells, e.g., host lectins and changes in illumination.Citation54 On this basis, hormogonia are the cells that only induce lectin receptor in filaments from L. corniculatumCitation15 whereas all Nostoc cells isolated from P. canina are reactive against Peltigera lectin. This is, non-motile cells of the cyanobacterium bind the lectin, are recruited and move toward the lectin source. Non-stimulated cell (phase I in ), the binding of the first lectin molecules to cell wall receptors that produce an invagination at the opposite pole of the cell (phase II). This is followed by depolymerization of actin at the opposite pole (phase III), which probably releases the tension from the actin-like cable bound to the membrane, and induces recovery of the spherical cell shape and displacement of the cell. Finally, upon reaching the maximal concentration of the gradient, asymmetry disappears and the cell becomes desensitized (phase IV). At this stage, lectin binding promotes cell aggregation.
In summary, the results presented here suggest a cytoskeletal-driven mode of cyanobacteria chemotropism similar to those present in eukaryotic cells responding to a chemoattractant gradient. Further research will be aimed to elucidate the molecular players involved in this process and unravel the mechanism of force generation and chemotropism.
Material and Methods
Plant material.
Peltigera canina (Ach.) Schrad. is a cyanolichen from Peltigerales, containing Nostoc as cyanobiont. Thalli were colleted at El Escorial (Madrid, Spain), and used throughout this work. Thalli were dried in air and stored in the dark, at room temperature, no longer than two weeks.
Isolation of lichen cyanobionts.
Cyanobionts were isolated from thalli of P. canina according to a protocol modified from reference Citation55. Samples of dry thalli (1.0 g) were rinsed distilled water to remove superficial contaminations. Samples were then macerated in a mortar with 15 mL of an extraction buffer (50 mM HEPES-NaOH buffer, pH 7.0, containing 0.25 M sorbitol with the addition of 1% polyvinylpirrolidone 40,000 and 0.25% bovine serum albumin). To sediment larger fragments, the homogenate was brought to 700x g, and then the centrifuge was turned off. Grinding in new buffer and centrifugation were repeated four times. All supernatants were pooled and centrifuged (1,200x g, 10 min). The pellet obtained contained only few traces of fungal hyphae.
Arginase secretion.
Samples of lichen thallus of 1.0 g dry weight were floated on 20 mL 40 mM arginine in 0.1 M TRIS-HCl buffer, pH 9.15, for 8 h at 25°C maintained in the dark. Media were filtered through Whatman paper 3 MM and dialyzed against distilled water for 24 h at 4°C in the dark.Citation56 Protein estimated according to reference Citation57. Peltigera arginase was pre-purified by affinity chromatography using cyanogen bromide-activated agarose bearing urease secreted from Evernia prunastri thalli, according to reference 5. About 2.0 g of agarose were fully hydrated with MiliQ water and mixed with 20 mL of a solution of purified urease containing 0.5 mg protein mL−1 in 0.1 M phosphate buffer (pH 7.4) for 16 h at 4°C. Glycine (0.6 g) was added to the mixture and stored at room temperature for 8 h.Citation58 Beads of activated agarose containing bound urease were packed in columns (4.0 cm × 1.0 cm i.d.) and washed with 300 mL 0.1 M phosphate buffer (pH 7.4) at a flux rate of 1.0 mL min−1. Samples of 2.0 ml of media after incubation of P. canina thalli on 40 mM arginine were loaded onto the bead and kept for 2 h at room temperature. Protein was eluted with 100 mM α-D-galactose in buffer, collecting fractions of 3.0 mL volume and monitored by absorbance at 280 nm. Eluted protein from agarose beads was assayed for arginase activity.
Assay of arginase activity.
Both dialyzed media and cell-free extracts were assayed for arginase activity according to reference Citation59, including crystalline urease in the reaction mixtures. These contained 10 µmol TRIS-HCl buffer (pH 9.1), 7.5 µmol maleic acid, 5.0 µmol Mn2+ (as manganese sulfate), 0.4 µmol arginine, 8.1 mg crystalline urease and 10.0 µg purified protein in a final volume of 3.0 mL. The reaction was performed at 37°C for 30 min and stopped by addition of 0.5 mL of a saturated potassium carbonate solution. Ammonia produced was measured by the microdiffusion method.Citation60 A unit of specific activity was defined as 1.0 µmol ammonia produced per mg protein per min.
Labeling of lichen arginases with fluorescein isothiocynate and binding to cyanobiont cells.
Purified arginase was labeled with fluorescein isothiocyanate (FITC) according Molina et al. A buffer solution of arginase, containing 10 µg protein mL−1, was mixed with 100 µg FITC and maintained in the dark, for 1 h at 30°C with continuous shaking. This mixture was dialyzed with distilled water to remove free fluorophore.
Cyanobionts cells isolated from samples of 1.0 g of P. canina thalli were incubated with fluorescent, secreted arginase (10 µg protein mL−1) in a final volume of 2.0 mL for 2 h at 30°C in the dark and continuous shaking. Cells were then collected by centrifugation at 10,000x g for 5 min at 2°C, resuspended in 1 mL extraction buffer (50 mM HEPES-NaOH buffer, pH 7.0, containing 0.25 M sorbitol with the addition of 1% polyvinylpirrolidone 40,000 and 0.25% bovine serum albumin).
Samples of collected cells were immobilized on a slide that had been coated with 30 µL poly-L-lysine (1.0 µM in MiliQ water, Milipore) for 12 h, mounted in Mowiol-DABCO (Calbiochem, La Jolla, CA USA) to avoid fluorescence decay, and examined using a Zeiss Axioplan 2 fluorescence microscope (Jena, Germany) equipped with CCD. Images were captured with Viewfinder Lite program (Zeiss).
Chemotaxis assay.
Cyanobacterial chemotaxis was studied according to the method described by Sjoblad et al. with modifications. Petri dishes containing 4.0 mL distilled water were used. Initially, assay was performed in absence of in the presence of 20 µM or 100 µM S-(3,4-dichlorobenzyl)isothiourea, A22 a MreB inhibitor.Citation29,Citation30 Alternatively, 10 µM or 25 µM blebbistatin, 0.095 µM or 0.19 µM phalloidin or both 25 µM blebbistatin and 0.19 µM phallodin, and 10 µM latrunculin A were added and homogeneously distributed after shaking to reach the indicated final concentrations, when indicated. Then, 100 µL of a cell suspension, immediately after isolation and containing about 300 ± 20 cells were deposited into water in the Petri dish and the open end of a capillary containing 20 µL of 1.0 mg L−1 lichen lectin was submerged in the water at 4 mm of cyanobacterial suspension. Plates were covered with black boxes to avoid lighting and air movement and to minimize evaporation. Plates were incubated at 22°C ± 1°C for variable time period. Since all the inhibitors were simultaneously assayed, an unique control without chemicals was performed.
After incubation, capillaries were removed from the Petri dishes and their outside surfaces were carefully dried. The content of each capillary was deposited on a glass slide, observed under a light microscope using a Zeiss 60 invertoscope fitted with a CCD camera for capturing images using a Viewfinder Lite program. Ten different fields by every slide were selected at random, counted the cells that appear in them and calculated the average. The procedure was repeated in triplicate, using three different capillaries for each assay.
Alternatively, fluorescein isothiocyanate-conjugated phalloidin was used for visualization of actin cytoskeleton by fluorescence microscopy.Citation63 Fluorescent phalloidin treatment was done after recovering the cells from the capillaries. Stained cells were observed using an Olympus DP50 fluorescence microscope adapted with an Olympus DP72 camera. A last probe to demonstrate that actin is involved in motility consist of the recovering of cells after moving toward the lichen lectin, to treat these cells with 10 µM latrunculin A to depolymerize F-actin and to reveal after this with 0.19 µM phalloidin. If latrunculin A promotes depolymerization, no phalloidin-related fluorescence emission might be observed from the cells.
Transmission electron microscopy.
Ultrastructural TEM analysis was performed by softening recently isolated Nostoc cells in phenol:acetic acid (1:1 v/v) mixture for three days and then the material was dehydrated in an alcohol series and fixed with glutaraldehyde, including after dehydration a step during which cells were treated with uranyl acetate. Embedding was done in propylene oxide-araldite II resin (standard mixture) for three days at 70°C. Ultrathin sections (600 a) obtained with anOmU-2Reichert-Jung ultratome (Wien, Austria) were examined on a Jeol 1010 electron microscope (Tokyo, Japan).
Scanning electron microscopy.
For conventional scanning electron microscopy (SEM), samples were collected from the corresdponding capillaries after chemotaxis assay, fixed in 2% glutaraldehyde (v/v) in 0.1 M phosphate buffer, pH 7.2, post-fixed with osmium tetroxide, washed, dehydrated in acetone, critical-point dried, sputter-coated with gold/palladium and scanned at 20 kV by SEM using a JEOL JSM 6400 (Japan). Digital images were obtained by using an INCA (Oxford) program incorporated to the equipment.
Disclosure of Potential Conflicts of Interest
No potential conflicts of interest were disclosed.
Figures and Tables
Figure 1 Time course of cell motility effected by 20 ng of Peltigera lectin and estimated as the total number of single cells that entry the capillary after different time periods. Values are the mean of three replicates. Vertical bars give the standard error where larger than the symbols.
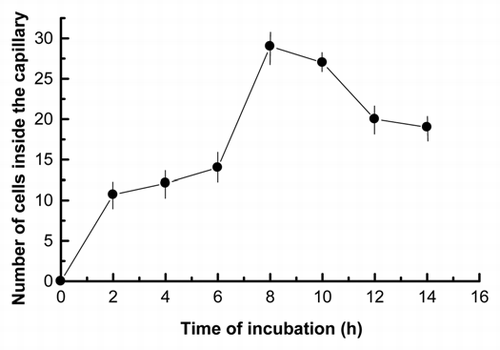
Figure 2 Dose-response curve of Nostoc motility after 8 h of chemotactic stimulation effected by variable concentrations of the lichen lectin, done as single cells inside the capillary. Values are the mean of three replicates. Vertical bars give the standard error where larger than the symbols.
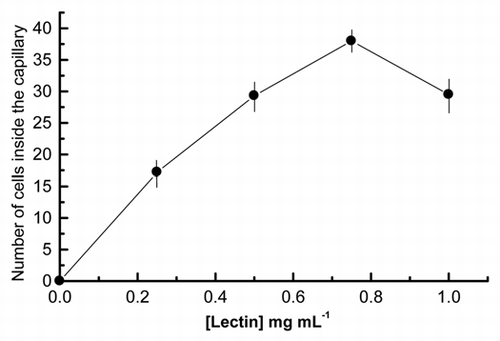
Figure 3 Transmission electron micrographs of Nostoc recently isolated from Peltigera canina showing the complex external layer with its serrated substructure. EL, serrated external layer; F, hair-like fibers; OM, outer membrane; P, peptidoglycan.
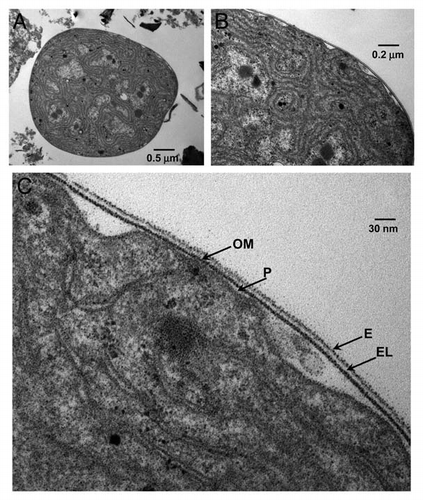
Figure 4 SEM micrographs of Nostoc cells isolated from Peltigera canina thalli after 4 h of contact the capillary containing the lichen lectin (20 ng) and the buffer containing cyanobacteria at 4 mm from the open capillary tip. (A) shows Nostoc cells remaining in the external buffer whereas (B–D) show cyanobacteria after their entry inside the capillary. Before the entry, several cells show the absence of external motile structures (pili) and a clear invagination (arrows in A) whereas after the entry, Nostoc cells are grouped (B) forming incipient filaments (C and D) and they are covered by a mucilaginous substance.
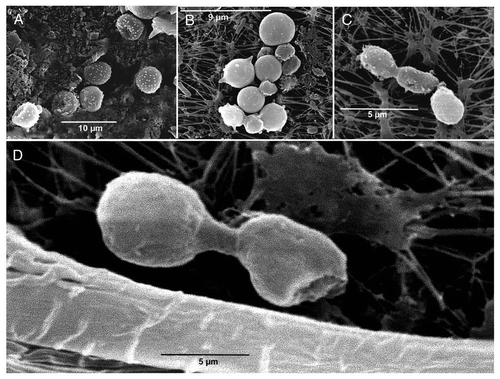
Figure 5 (A) Effect of A22 on cyanobacteria cell motility effected by 20 ng of lichen lectin in terms of the number of single cells inside the capillary. Two different concentrations of phalloidin were included in the buffer, 20 µM (triangles) and 100 µM (data not shown) whereas the buffer of the control assay (circles) did not contain the inhibitor. (B) Fluorescence micrographs of Nostoc cells inside the capillary containing 20 ng of FITC-labeled lichen lectin in the presence of 20 µM A22 revealed with 0.19 µM FITC-phalloidin.
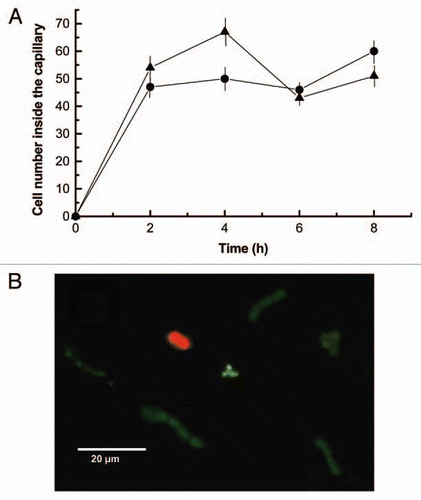
Figure 6 (A) Effect of phalloidin on cyanobacteria cell motility effected by 20 ng of lichen lectin in terms of the number of single cells inside the capillary. Two different concentrations of phalloidin were included in the buffer, 0.19 µM (squares) and 0.095 µM (triangles) whereas the buffer of the control assay (circles) did not contain the inhibitor. (B) Effect of blebbistatin on cyanobacteria cell motility effected by 20 ng of lichen lectin. Two different concentrations of blebbistatin were included in the buffer, 10 µM (squares) and 25 µM (triangles) whereas the buffer of the control assay (circles) did not contain the inhibitor. (C) Combined effect of 0.19 µM phalloidin and 25 µM blebbistatin (triangles) on Nostoc motility. Values are the mean of three replicates. Vertical bars give the standard error where larger than the symbols.
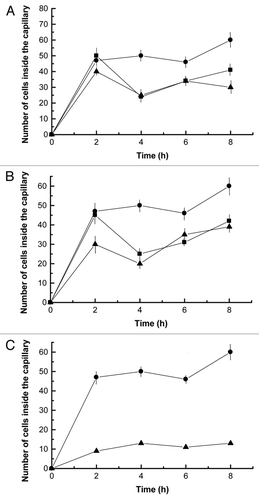
Figure 7 (A) Effect of 10 µM latrunculin A (triangles) and combined effect of 10 µM latrunculin A and 25 µM blebbistatin (squares) on cyanobacteria cell motility, in terms of the number of single cells inside the capillary, effected by 20 ng of lichen lectin against untreated (circles) control cells. Values are the mean of three replicates. Vertical bars give the standard error where larger than the symbols.
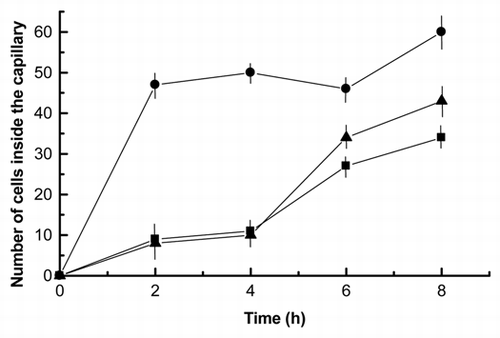
Figure 8 Fluorescence micrographs of Nostoc cells inside the capillary containing 20 ng of FITC-labeled lichen lectin in absence of inhibitors revealed with 0.19 µM FITC-phalloidin (A), on 25 µM blebbistatin (B) and on 0.19 µM phalloidin and 25 µM blebbistatin (C). White arrows indicate the start of cell aggregation, since no filaments have been seen in the intact thallus, whereas red arrows indicate polar points of probable depolymerization of F-actin, since no fluorescence has been detected at this zone.
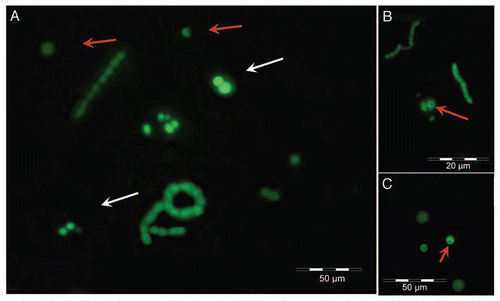
Figure 9 (A) Fluorescence micrographs of Nostoc cells inside the capillary containing the lichen lectin in absence of inhibitors revealed with FITC-phalloidin. (B) Cells from the same sample as (A) treated for 4 h with 10 µM latrunculin A before addition of FITC-phalloidin. Red fluorescence emission in (B) is due to Nostoc chlorophylls, which is not masked when FITC-phalloidin is unable to bind to latrunculin-depolymerized actin.
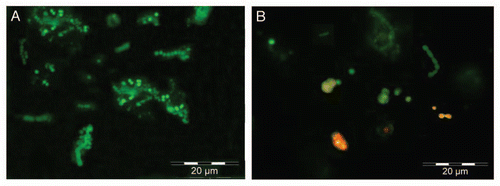
Figure 10 Hypothetical scheme of Nostoc cells motility through a gradient of lichen lectin. The first binding of the lectin to its receptors induces polar cell invagination produced by interaction of an ATPase with contractile ability, sensitive to blebbistatin, with F-actin cytoskeleton. After this, depolymerization of F-actin is achieved at the opposite pole, re-polymerization of which produces the cell advancement.
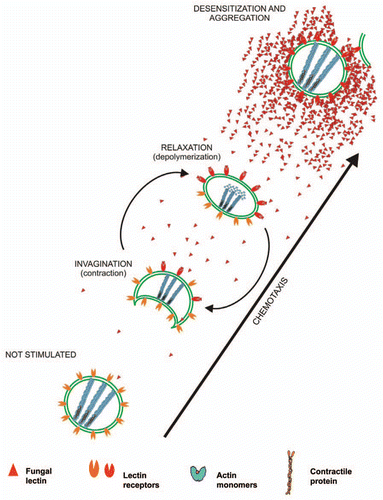
Table 1 Quantitation of the number cells showing asymmetrical distribution of F-actin
Acknowledgments
This work was supported by a Grant from the Dirección General de Investigación Científica y Tecnológica (Ministerio de Ciencia e Innovación, Spain) BFU2009-11983.
References
- Galun M, Kardish N. Lectins as determinants of symbiotic specificity in lichens. Cryptogam Bot 1995; 5:144 - 148
- Bubrick P. Galun M. Effects of symbiosis on the photobiont. Handbook of Lichenology 1988; 2:Boca Raton CRC Press 133 - 148
- Lockhart CM, Rowell P, Stewart WDP. Phytohaemagglutinins from the nitrogen-fixing lichens Peltigera canina and P. polydactyla. FEMS Microbiol Lett 1978; 3:127 - 130; http://dx.doi.org/10.1111/j.1574-6968.1978.tb01899.x
- Sacristán M, Millanes AM, Legaz ME, Vicente C. A lichen lectin specifically binds to the α-1,4-polygalactoside moiety of urease located in the cell wall of homologous algae. Plant Signal Behav 2006; 1:23 - 27; PMID: 19521472; http://dx.doi.org/10.4161/psb.1.1.2276
- Legaz ME, Fontaniella B, Millanes AM, Vicente C. Secreted arginases from phylogenetically far-related lichens species act as cross-recognition factors for two different algal cells. Eur J Cell Biol 2004; 3:435 - 446; http://dx.doi.org/10.1078/0171-9335-00384
- Czeczuga B, Krukowska KR. Effect of habitat condition on phycobionts and the content of photosynthesizing pigments in five lichen species. J Hattori Bot Lab 2001; 90:293 - 305
- Sacristán M, Millanes AM, Vicente C, Legaz ME. Synchronic production of fungal lectin, phycobiont lectin receptors and algal division in Evernia prunastri. J Hattori Bot Lab 2006; 100:739 - 751
- Bubrick P, Galun M. Proteins from the lichen Xanthoria parietina which bind to phycobiont cell walls: correlation between binding patterns and cell wall cytochemistry. Protoplasma 1980; 104:167 - 173; http://dx.doi.org/10.1007/BF01279379
- Kardish N, Silberstein L, Flemminger G, Galun M. Lectin from the lichen Nephroma laevigatum. Localization and function. Symbiosis 1991; 11:47 - 62
- Lehr H, Fleminger G, Galun M. Lectin from the lichen Peltigera membranacea: Characterization and function. Symbiosis 1995; 18:1 - 13
- Rai AN, Söderbäck E, Bergman B. Cyanobacterium-plant symbioses. New Phytol 2000; 147:449 - 481; http://dx.doi.org/10.1046/j.1469-8137.2000.00720.x
- Lehr H, Galun M, Ott S, Jahns HM, Fleminger G. Cephalodia of the lichen Peltigera aphthosa. Specific recognition of the compatible photobiont. Symbiosis 2000; 29:357 - 365
- Paulsrud P, Rikkinen J, Lindblad P. Field experiments on cyanobacterial specificity in Peltigera aphthosa. New Phytol 2001; 152:117 - 123; http://dx.doi.org/10.1046/j.0028-646x.2001.00234.x
- Díaz EM, Sacristán M, Legaz ME, Vicente C. Isolation and characterization of a cyanobacterium-binding protein and its cell wall receptor in the lichen Peltigera canina. Plant Signal Behav 2009; 4:598 - 603; PMID: 19820309; http://dx.doi.org/10.4161/psb.4.7.9164
- Vivas M, Sacristán M, Legaz ME, Vicente C. The cell recognition model in chlorolichens involving a fungal lectin binding to an algal ligand can be extended to cyanolichens. Plant Biol 2010; 12:615 - 621; PMID: 20636904
- Dick H, Stewart WDP. The occurrence of fimbriae on a N22-fixing cyanobacterium which occurs in lichen symbiosis. Arch Microbiol 1980; 124:1907 - 1999; http://dx.doi.org/10.1007/BF00407037
- Knight CD, Adams DG. A method for studying chemotaxis in nitrogen fixing cyanobacterium-plant symbiosis. Physiol Mol Plant Pathol 1996; 49:73 - 77; http://dx.doi.org/10.1006/pmpp.1996.0040
- Rasmussen U, Johansson C, Bergman B. Early communication in the Gunnera-Nostoc symbiosis plant-induced cell differentiation and protein synthesis in the cyanobacterium. Mol Plant Microbe Interact 1994; 7:696 - 702; http://dx.doi.org/10.1094/MPMI-7-0696
- Rikkinen J. Rai AN, Bergman B, Rasmussen U. Cyanolichens: an evolutionary overview. Cyanobacteria in Symbiosis 2002; Amsterdam Kluwe Academic Publishig 31 - 72
- Campbell EL, Meeks JC. Characteristics of hormogonia formation by symbiotic Nostoc spp. in response to the presence of Anthoceros punctatus or its extracellular products. Appl Environ Microbiol 1989; 55:125 - 131; PMID: 16347816
- Nilsson M, Rasmussen U, Bergman B. Cyanobacterial chemotaxis to extracts of host and nonhost plants. FEMS Microbiol Ecol 2006; 55:382 - 390; PMID: 16466377; http://dx.doi.org/10.1111/j.1574-6941.2005.00043.x
- Damerval T, Guglielmi G, Houmard J, Tandeau de Marsac N. Hormogonium differentiation in the cyanobacterium Calothrix: a photoregulated developmental process. Plant Cell 1991; 3:191 - 201; PMID: 12324595
- Duggan PS, Gottardello P, Adams DG. Molecular analysis of genes in Nostoc punctiforme involved in pilus biogenesis and plant infection. J Bacteriol 2007; 189:4547 - 4551; PMID: 17416648; http://dx.doi.org/10.1128/JB.01927-06
- Yoshihara S, Ikeuchi M. Phototactic motility in the unicellular cyanobacterium Synechocystis sp. PCC 6803. Photochem Photobiol Sci 2004; 3:512 - 518; PMID: 15170479; http://dx.doi.org/10.1039/b402320j
- Erickson HP. Cytoskeleton: evolution in bacteria. Nature 2001; 413:30; PMID: 11544510; http://dx.doi.org/10.1038/35092655
- Vollmer W. The prokaryotic cytoskeleton: a putative target for inhibitors and antibiotics?. Appl Microbiol Biotechnol 2006; 73:37 - 47; PMID: 17024474; http://dx.doi.org/10.1007/s00253-006-0586-0
- Osborn MJ, Rothfield L. Cell shape determination in Escherichia coli. Curr Opin Microbiol 2007; 10:606 - 610; PMID: 17981077; http://dx.doi.org/10.1016/j.mib.2007.09.004
- Shiomi D, Sakai M, Niki H. Determination of bacterial rod shape by a novel cytoskeletal membrane protein. EMBO J 2008; 27:3081 - 3091; PMID: 19008860; http://dx.doi.org/10.1038/emboj.2008.234
- Dye NA, Pincus Z, Theriot JA, Shapiro L, Gitai Z. Two independent spiral structures control cell shape in Caulobacter. Proc Natl Acad Sci USA 2005; 102:18608 - 18613; PMID: 16344481; http://dx.doi.org/10.1073/pnas.0507708102
- Gitai Z, Dye NA, Reisenauer A, Wachi M, Shapiro L. MreB actin-mediated segregation of a specific region of bacterial chromosome. Cell 2005; 120:329 - 341; PMID: 15707892; http://dx.doi.org/10.1016/j.cell.2005.01.007
- Jones LJF, Carballido-Lopez R, Errington J. Control of cell shape in bacteria: helical, actin-like filaments in Bacillus subtilis. Cell 2001; 104:913 - 922; PMID: 11290328; http://dx.doi.org/10.1016/S0092-8674(01)00287-2
- Usmanova A, Astier C, Méjean C, Hubert F, Feinberg J, Benyamin Y, et al. Coevolution of actin and associated proteins: an α-actinin-like protein in a cyanobacterium (Spirulina platensis). Comp Biochem Physiol B Biochem Mol Biol 1998; 120:693 - 700; PMID: 9854817; http://dx.doi.org/10.1016/S0305-0491(98)10065-2
- Guerrero-Barrera AL, García-Cuéllar CM, Villalba JD, Segura-Nieto M, Gómez-Lojero C, Reyes ME, et al. Actin-related proteins in Anabaena spp. and Escherichia coli. Microbiology 1996; 142:1133 - 1140; PMID: 8704955; http://dx.doi.org/10.1099/13500872-142-5-1133
- Hu B, Yang G, Zhao W, Zhang Y, Zhao J. MreB is important for cell shape but not for chromosome segregation of the filamentous cyanobacterium Anabaena sp. PCC 7120. Mol Microbiol 2007; 63:1640 - 1652; PMID: 17367385; http://dx.doi.org/10.1111/j.1365-2958.2007.05618.x
- van der Honing HS, de Ruijter NCA, Emons AMC, Ketelaat T. Actin and myosin regulate cytoplasm stiffness in plant cells: a study using tweezers. New Phytol 2010; 185:90 - 102; PMID: 19761443; http://dx.doi.org/10.1111/j.1469-8137.2009.03017.x
- Iwai N, Nagai K, Wachi M. Novel S-Benzyisothiourea compound that inbduces spherical cells in Escherichia coli probably by acting on a rod-shape-detgermining protein(s) other than penicillin-binding protein 2. Biosci Biotechnol Biochem 2002; 66:2658 - 2662; PMID: 12596863; http://dx.doi.org/10.1271/bbb.66.2658
- Villarreal AJC, Renzaglia KS. Structure and development of Nostoc strands in Leiosporoceros dussii (Anthocerotophyta): a novel symbiosis in land plants. Am J Bot 2006; 93:693 - 705; PMID: 21642133; http://dx.doi.org/10.3732/ajb.93.5.693
- Rubik BA, Koshland DE. Potentiation, desensitization and inversion of response in bacterial sensing of chemical stimuli. Proc Natl Acad Sci USA 1978; 75:2820 - 2824; PMID: 351616; http://dx.doi.org/10.1073/pnas.75.6.2820
- Van Haastert PJ, Devreotes PN. Chemotaxis: signalling the way forward. Nat Rev Mol Cell Biol 2004; 5:626 - 634; PMID: 15366706; http://dx.doi.org/10.1038/nrm1435
- Ackland JC, West JA, Pickett-Heaps J. Actin and myosin regulate pseudopodia of Porphyra pulchella (Rhodophyta) archeospores. J Phycol 2007; 43:129 - 138; http://dx.doi.org/10.1111/j.1529-8817.2006.00311.x
- Higaki T, Sano T, Hasezawa S. Actin microfilament dynamics and actin side-binding proteins in plants. Curr Opin Plant Biol 2007; 10:549 - 556; PMID: 17936064; http://dx.doi.org/10.1016/j.pbi.2007.08.012
- Vicente-Manzanares M, Ma X, Adelstein R, Horwitz AR. Non-muscle myosin II takes centre stage in cell adhesion and migration. Nat Rev Mol Cell Biol 2009; 10:778 - 790; PMID: 19851336; http://dx.doi.org/10.1038/nrm2786
- Lenartowska M, Michalska A. Actin filament organization and polarity of pollen tubes revealed by myosin II subfragment 1 decoration. Planta 2008; 228:891 - 896; PMID: 18696106; http://dx.doi.org/10.1007/s00425-008-0802-5
- dos Remedios CG, Chhabra D, Kekic M, Dedova IV, Tsubakihara M, Berry DA, et al. Actin binding proteins: regulation of cytoskeletal microfilaments. Physiol Rev 2003; 83:433 - 473; PMID: 12663865
- Esue O, Cordero M, Wirtz D, Tseng Y. The assembly of MreB, a prokaryotic homolog of actin. J Biol Chem 2005; 280:2628 - 2635; PMID: 15548516; http://dx.doi.org/10.1074/jbc.M410298200
- Bean GJ, Flickinger ST, Westler WM, McCully ME, Sept D, Weibel DB, et al. A22 disrupts the bacterial actin cytoskeleton by directly binding and inducing a low-affinity state in MreB. Biochemistry 2009; 48:4852 - 4857; PMID: 19382805; http://dx.doi.org/10.1021/bi900014d
- Guerrero-Barrera AL, de la Garza M, Mondragón R, Garcia-Cuellar C, Segura Nieto M. Actin-related proteins in Actinobacillus pleuropneumoniae and their interactions with actin-binding proteins. Microbiology 1999; 145:3235 - 3244; PMID: 10589733
- Hoiczyk E. Gliding motility in cyanobacteria: observations and possible explanations. Arch Microbiol 2000; 174:11 - 17; PMID: 10985737; http://dx.doi.org/10.1007/s002030000187
- Hoiczyk E, Baumeister W. Envelope structure of four gliding filamentous cyanobacteria. J Bacteriol 1995; 177:2387 - 2395; PMID: 7730269
- Adams DG. How do cyanobacteria glide?. Microbiol Today 2001; 28:131 - 133
- Kuwayama H, Kikuchi H, Oshima Y, Kubohara Y. Artificial compounds differentially control Dictyostelium chemotaxis and cell differentiation. Cell Struct Funct 2011; 36:21 - 26; PMID: 21258165; http://dx.doi.org/10.1247/csf.10018
- Sacristán M, Vivas M, Millanes AM, Fontaniella B, Vicente C, Legaz ME. Méndez-Vilas A. The recognition pattern of green algae by lichenized fungi can be extended to lichens containing a cyanobacterium as photobiont. Communicating Current Research and Educational Topics and Trends in Applied Microbiology 2007; Badajoz Formatex 213 - 219
- Bergman B, Rai AN, Johanson C, Söderbäck E. Cyanobacterial-plant symbioses. Symbiosis 1993; 14:61 - 81
- Meeks JC, Elhai J. Regulation of cellular differentiation in filamentous cyanobacteria in free-living and plant-associated symbiotic growth states. Microbiol Mol Biol Rev 2002; 66:94 - 121; PMID: 11875129; http://dx.doi.org/10.1128/MMBR.66.1.94-121.2002
- Wastlhuber R, Loos E. Differences between cultured and freshly isolated cyanobiont from Peltigera is there symbiosis-specific regulation of glucose carrier. Lichenologist 1996; 28:67 - 68
- Planelles V, Legaz ME. Purification and some properties of the secreted arginase of the lichen Evernia prunastri and its regulation by usnic acid. Plant Sci 1987; 51:9 - 16; http://dx.doi.org/10.1016/0168-9452(87)90214-7
- Lowry OH, Rosebrough NJ, Farr AL, Randall RJ. Protein measurement with the Folin phenol reagent. J Biol Chem 1951; 193:265 - 275; PMID: 14907713
- Walters RR. Dean PDG, Johnson WS, Middle FA. Ligands for immobilization. Affinity Chromatography, a practical approach 1985; Oxford IRL Press 114 - 116
- Legaz ME, Vicente C. Two forms of arginase in Evernia prunastri. Biochem Biophys Res Commun 1982; 104:1441 - 1446; PMID: 7073752; http://dx.doi.org/10.1016/0006-291X(82)91411-5
- Conway EJ. Microdiffusion Analysis and Volumetric Error 1962; 5:London Crosby Loockwood 65 - 122
- Molina MC, Muñiz E, Vicente C. Enzymatic activities of algal-binding protein and its algal cell wall receptor in the lichen Xanthoria parietina. An approach to the parasitic basis of mutualism. Plant Physiol Biochem 1993; 31:131 - 142
- Sjoblad RD, Chet I, Mitchell R. Quantitative assay for algal chemotaxis. Appl Environ Microbiol 1978; 36:847 - 850; PMID: 32834
- Millanes AM, Vicente C, Legaz ME. Sugarcane glycoproteins bind to surface, specific ligands and modify cytoskeleton arrangement of Ustilago scitaminea teliospores. J Plant Interact 2008; 3:95 - 110; http://dx.doi.org/10.1080/17429140701861727