Abstract
Uniquely among the plant glutathione transferases, two classes possess a catalytic cysteine capable of performing glutathione-dependent reductions. These are the dehydroascorbate reductases (DHARs) and the lambda-class glutathione transferases (GSTLs). Using immobilized GSTLs probed with crude plant extracts we have identified flavonols as high affinity ligands and subsequently demonstrated a novel glutathione-dependent role for these enzymes in recycling oxidized quercetin. By comparing the activities of DHARs and GSTLs we now propose a unified catalytic mechanism that suggests oxidized anthocyanidins and tocopherols may be alternative polyphenolic substrates of GSTLs.
All plants examined to date contain a superfamily of glutathione transferases (GSTs), which have the common features of being soluble proteins, with a shared overall structural topology and an ability to bind the tripeptide glutathione. In terms of phylogeny, this GST superfamily is remarkably varied and can be subdivided into classes reflecting sequence, structural and presumably functional diversification. GSTs consistently turn up in proteomic and transcriptomic data-sets due to their abundance and strong inducibility by chemical, biological and environmental perturbations.Citation1 Indeed, this ubiquity and strong association with stress responses has consistently linked GSTs with important functions, though in most cases these remain unknown. One approach to divine function for these proteins is to study their active site chemistry, which gives important clues as to the likely reactions catalyzed. The active sites of GSTs retain a highly conserved set of residues involved in docking glutathione, termed the G-site, and a hydrophobic binding domain, or H-site, composed of less conserved residues which co-ordinates the binding of co-substrates.Citation2 Within the G-site, GSTs possess a single amino acid residue that interacts with the sulfhydryl group of glutathione to catalyze a range of chemical reactions. For most classes of plant GST this residue is a serine, which acts to abstract the sulfhydryl proton, thereby stabilizing the highly reactive thiolate anion of GSH. In this activated state the GSH can take part in nucleophilic substitution and nucleophilic addition reactions with co-substrates, along with mechanistically-related isomerization and peroxidase activities.Citation2
In addition to this common active site chemistry, in 2002 we described a further two classes of plant GST which contain a cysteine residue in place of the conserved serine.Citation3 These two classes of “cysteinyl” GSTs are the dehydroascorbate reductases (DHARs) and the lambda class GSTs (GSTLs).Citation3 The simple substitution of these active site residues completely changes the reactivity profile of these enzymes. In particular, rather than promoting the formation of the reactive thiolate anion of GSH, this cysteine can reversibly form mixed disulfides with the thiol. This is an oxidative reaction that in turn provides the capability to reduce substrates. As their name suggests, the DHARs already had a well-characterized enzymic role, though their phylogenetic relationship to the GST family had not previously been recognized. In contrast, the functions of the GSTLs were unknown. The GSTLs demonstrate several behaviors which make them important targets for functional characterization. Importantly, specific GSTLs are strongly upregulated in response to exposure to xenobiotic compounds including herbicides, herbicide safeners and pharmaceuticals.Citation3–Citation5 As with other GST classes, this correlation provides circumstantial evidence for a role in stress tolerance. However, unlike other family members the active site chemistry suggests a glutathione-dependent reductase, rather than detoxification activity. To better understand the function of these enzymes, their catalytic role needs to be determined. We have approached this challenge firstly by applying knowledge from the related DHARs, and secondly by searching for small metabolite ligands for GSTLs.
In the case of DHAR, its substrate is derived from the major plant antioxidant, ascorbic acid (vitamin C). On oxidation, ascorbic acid is readily converted to monodehydroascorbate and then, if not rapidly re-reduced, dismutates to dehydroascorbate (DHA). Acting to maintain the ascorbate pool, DHARs efficiently reduce DHA back to ascorbic acid by concomitantly oxidizing glutathione to glutathione disulfide, as part of the ascorbate-glutathione cycle.Citation6 Importantly, the subcellular distribution of DHARs correlates well with this function. Thus, these enzymes are present or predicted to be present, in the cytosol, chloroplast and peroxisome, all locations having an active ascorbate-glutathione cycle, at least in Arabidopsis.Citation3,Citation7 Similarly, the regulation of these enzymes also functionally links them to maintaining redox homeostasis, with DHAR1 in Arabidopsis strongly induced (at the transcriptional level) by a number of stresses expected to enhance the turnover of ascorbate.Citation3 Drawing analogies with the DHARs, members of the GSTL family are also differentially responsive to treatments likely to impose oxidative stress and are expressed in both plastid and the cytosol, suggesting any associated protective roles are likely to apply to ubiquitous cellular metabolites.
To identify putative GSTL substrates, we recently identified a series of metabolites that bind tightly to wheat GSTLs, and from that deduced a likely enzyme substrate and catalytic cycle that was subsequently experimentally confirmed.Citation8 By immobilizing Strep-tagged recombinant wheat GSTLs on an affinity matrix, the enzyme was incubated with crude plant extracts. Using this “panning” method, tight-binding ligands were retained while other molecules were washed away (). After affinity elution of the tagged GSTLs, the bound ligands were then solvent extracted and identified by HPLC-MS. The major xenobiotic-inducible GSTL in wheat, termed TaGSTL1, was found to tightly bind a series of tobacco flavonols and their derivatives, including rutin, kaempferol-3-rutinoside, taxifolin and dihydrokaempferol. Rutin and kaempferol-3-rutinoside were also pulled down from wheat extracts, which was surprising since these flavonols had not previously been described in wheat, showing the high sensitivity of the technique. It was determined that this binding was strongly dependent on the state of the enzyme active-site cysteine. Ligand binding was weak when the active site cysteine was in a reduced state, but very strong when oxidized to form a mixed disulfide with GSH. By comparison with the proposed catalytic cycle for DHARs,Citation3 we were able to postulate that the enzyme-glutathione mixed disulfide was a catalytic intermediate, with the most likely precursor being a glutathione-conjugated flavonol. Synthesis of glutathione conjugates of the model flavonol quercetin allowed this to be tested. In addition to TaGSTL1 actively catalyzing the reductive deglutathionylation of these conjugates, similar activities could be demonstrated with other GSTLs isolated from wheat and Arabidopsis, even though these proteins had not pulled down flavonols in similar ligand fishing experiments. At first glance, the DHAR and flavonol conjugate reduction activities appear quite different. For example, unlike the DHAR reaction, flavonol reduction acts on a glutathione conjugate rather than directly on an oxidized cofactor. However, a common reaction mechanism based on that proposed for DHARCitation3 can be postulated, involving single electron transfer to a vicinal tricarbonyl substrate (). In the case of flavonol conjugate reduction, such a substrate can be formed by spontaneous or enzyme-catalyzed elimination of the GSH.
From these studies, we now have good biochemical evidence that glutathione adducts of oxidized flavonols can be recycled back to the parent flavonols by GSTLs in vitro, a potentially important step in maintaining antioxidant pools. However, in wheat the flavonols are very minor substrates, posing the question is this the only function of GSTLs in vivo, or can they catalyze other important endogenous reduction activities? In Arabidopsis, the distribution and expression of GSTLs quite closely mirrors that observed for DHARs. For example, AtGSTL1 is apparently cytosolic and strongly inducible, while AtGSTL2 has a likely chloroplast targeting peptide (and may also localize to the peroxisome) and is expressed constitutively, and AtGSTL3 appears to be a constitutive, cytosolic protein.Citation3,Citation9 Similarly, the GSTLs in wheat show a similar distribution, with TaGSTL1 and TaGSTL3 being cytosolic, while TaGSTL2 has a putative chloroplast targeting peptide. Of these, only TaGSTL1 is stress-inducible,Citation5 with its QPCR-measured transcript levels increasing 30-fold when wheat seedlings were treated with the herbicide safener cloquintocet-mexyl. In contrast, transcripts for TaGSTL2 and TaGSTL3 were not significantly affected by safener treatment (unpublished data). In addition to these three isoenzymes, two further clades of GSTLs were determined in wheat,Citation8 though based on expression data, these additional family members appear to be poorly expressed and limited to anther-derived EST populations. The diversity in expression patterns for essentially similar enzymes points to divergent spatiotemporal roles. However, conservation in function cannot be wholly assumed. For example, while the stress-inducible, cytosolic GSTLs (AtGSTL1, TaGSTL1) tightly bound flavonols, the constitutively expressed GSTLs did not. Further, these two GSTLs were the only GSTLs to show catalytic discrimination between the two isomeric glutathionyl-quercetin substrates. This suggests that while all GSTLs have essentially conserved functions when acting on ubiquitous natural products, specific family members have been recruited to perform related protective functions with specific stress inducible metabolites. Intriguingly, phylogenetic analysis of GSTL polypeptide sequences from the monocotyledonous species wheat and rice, and from the dicotyledonous species Arabidopsis and poplar show a phylogeny compatible with the monocot GSTLs having evolved and diversified separately from the eudicot GSTLs (). Therefore, the selective flavonol-binding characteristics of AtGSTL1 and TaGSTL1 appear to have arisen independently.
From these observations we can conclude that the reduction of glutathionylated-flavonols is unlikely to be the primary function for GSTLs in plants, which begs the question as to their primary physiological function. An initial assumption is that any function will involve a GSH-dependent reduction activity, most likely directed toward a hydrophobic ligand. Based on the DHAR analogy, GSTLs are most likely to act on oxidized products of ubiquitous metabolites that act as antioxidants, or that are otherwise sensitive to oxidation. Based on the known enzyme chemistry of DHARs and the GSTL ligand fishing experiments, compounds which share characteristics with dehydroascorbate and glutathionylquercetin would be excellent candidate substrates. Intriguingly, oxidized anthocyanidins fulfill several of these characteristics. Anthocyanidins, such as the widely encountered cyanidin, are oxidation-sensitive precursors of anthocyanins (anthocyanidin glycosides) which are well known to accumulate in response to oxidative stress.Citation10 Using in vitro approaches,Citation8 the oxidation of cyanidin in the presence of glutathione generates glutathionylcyanidin derivatives. Preliminary mass spectrometry evidence has shown that these conjugates have similar properties to quercetin conjugates, suggesting a similar structure and therefore a likely ability to undergo a GSTL-catalyzed reduction.
A second class of potential GSTL substrates are the oxidized derivatives of tocopherols (vitamin E), which are major lipophilic antioxidants sharing analogous functions to the more hydrophilic ascorbic acid. Tocopherols act to quench lipid peroxy radicals, and in doing so are initially oxidized to the tocopheroxyl radical. This in turn, can be re-reduced by ascorbate. However, in a secondary reaction the tocopheroxyl radical can undergo further oxidation to form a ring-opened tocopheryl quinone species (). Although it is known in vivo that this quinone is recycled, reformation of tocopherol is a multi-step pathway, and the nature of the reductive step remains unclear.Citation11
Following from these reasoned arguments as to the functions of GSTLs, it will now be of interest to test their reducing activities toward tocopherols and anthocyanidins in vitro and in planta. The continuous formation of oxidized metabolites and cofactors as a consequence of photosynthesis and oxidative stress offers up several putative roles for novel reducing and recycling activities. Without guiding hypotheses, many of these functions may be intrinsically difficult to determine due to the instability of the metabolites concerned and the likely functional redundancy of individual GSTLs masked by the multiple representatives of the family. In a further twist to this enzyme chemistry, while GSTLs are unique to plants, many organisms contain related GSTs with active-site cysteines. In particular, animals contain omega-class GSTs which again have reductase activity but no well-characterized endogenous substrates.Citation12 Based on progress being made with the plant enzymes, it is certainly possible that knowledge of the functions of GSTLs will help deduce the function of such related enzymes in animals.
The ligand fishing methodology described in reference Citation8, has proved to be a novel and hugely powerful means to discover protein ligands. We have described its use to identify binding partners for GSTLs and here it directly lead to discovery of a role for these enzymes in flavonol recycling. The application of these methods to other plant proteins is likely to be similarly beneficial in deducing natural ligands and enzyme function.
Abbreviations
D | = | dehydroascorba |
DH | = | dehydroascorbate reducta |
G | = | glutathio |
G | = | glutathione transfera |
GS | = | lambda class glutathione transfera |
Figures and Tables
Figure 1 Schematic illustrating the in vitro (left) and in vivo (right) ligand fishing methods, with ligands shown by triangles and protein of interest shown by filled circles.
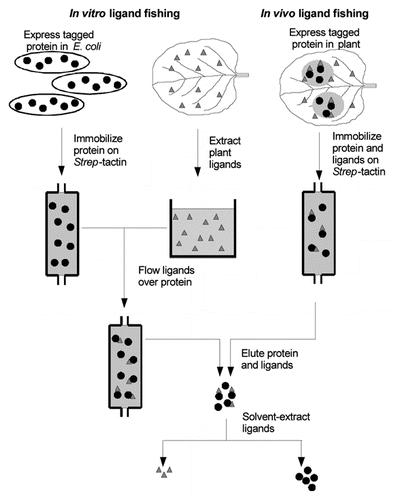
Figure 2 Model for the oxidation of quercetin and subsequent GSTL -catalyzed recycling. The tricarbonyl species of oxidized quercetin is acted on by the thiolate anion of the enzyme active site cysteine (E-S−) in a single electron transfer reaction. Glutathione (GSH) is then used to further reduce the substrate and oxidize the enzyme, giving an enzyme-GSH mixed disulfide. Disulfide exchange with a second GSH molecule allows regeneration of the reduced enzyme (not shown). The proposed related mechanism for dehydroascorbate reduction to ascorbate is shown inset for comparison.
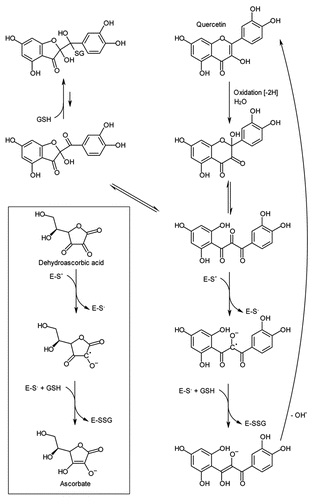
Figure 3 Deduced phylogenetic tree for GSTL polypeptide sequences from monocot (Os, Oryza sativa; Ta, Triticum aestivum) and eudicot (At, Arabidopsis thaliana; Pt, Populus trichocarpa) lineages showing that this distribution is compatible with monophyletic origins for monocot and eudicot GSTLs as indicated by the dotted line. Except for T. aestivum, sequences are taken from the genome databases. For T. aestivum, TaGSTL 1, TaGSTL 2 and TaGSTL 3 are as described in reference Citation8. TaGSTL 4 and TaGSTL 5 are derived from ESTs representative of GSTL clades 4 and 5 respectively.Citation8
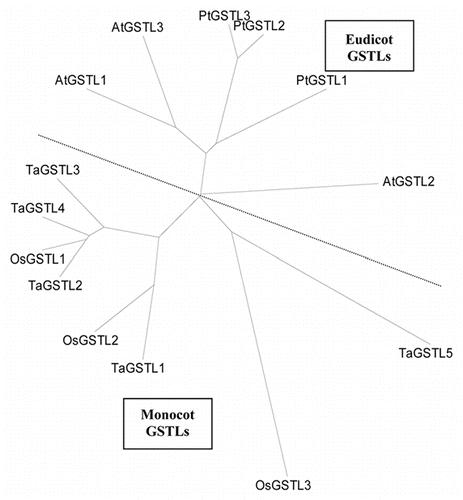
Figure 4 Putative pathway for the oxidation and re-reduction of α-tocopherol, adapted from Kobayashi and DellaPenna (2008).Citation11 The reduction from quinone to quinol is a possible function of GSTLs.
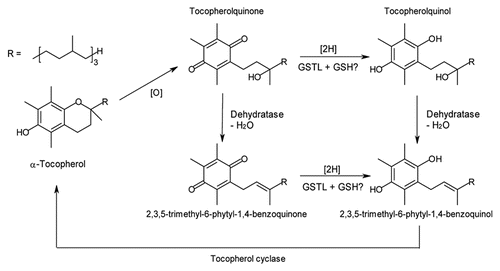
Acknowledgments
This work was supported by the Biotechnology and Biological Sciences Research Council (grant number BBG0017661).
Addendum to:
References
- Dixon DP, Lapthorn A, Edwards R. Plant glutathione transferases. Genome Biol 2002; 3:1 - 10
- Cummins I, Dixon DP, Freitag-Pohl S, Skipsey M, Edwards R. Multiple roles for plant glutathione transferases in xenobiotic detoxification. Drug Metab Rev 2011; 43:266 - 280
- Dixon DP, Davis BG, Edwards R. Functional divergence in the glutathione transferase superfamily in plants—identification of two classes with putative functions in redox homeostasis in Arabidopsis thaliana. J Biol Chem 2002; 277:30859 - 30869
- Hershey HP, Stoner TD. Isolation and characterization of cDNA clones for RNA species induced by substituted benzenesulfonamides in corn. Plant Mol Biol 1991; 17:679 - 690
- Theodoulou FL, Clark IM, He XL, Pallett KE, Cole DJ, Hallahan DL. Co-induction of glutathione-S-transferases and multidrug resistance associated protein by xenobiotics in wheat. Pest Manage Sci 2003; 59:202 - 214
- Foyer CH, Noctor G. Redox regulation in photosynthetic organisms: signaling, acclimation and practical implications. Antiox Redox Signal 2009; 11:861 - 905
- Reumann S, Quan S, Aung K, Yang P, Manandhar-Shrestha K, Holbrook D, et al. In-depth proteome analysis of Arabidopsis leaf peroxisomes combined with in vivo subcellular targeting verification indicates novel metabolic and regulatory functions of peroxisomes. Plant Physiol 2009; 150:125
- Dixon DP, Edwards R. Roles for stress-inducible lambda glutathione transferases in flavonoid metabolism in plants as identified by ligand fishing. J Biol Chem 2010; 285:36322 - 36329
- Dixon DP, Hawkins T, Hussey PJ, Edwards R. Enzyme activities and subcellular localization of members of the Arabidopsis glutathione transferase superfamily. J Exp Bot 2009; 60:1207 - 1218
- Winkel-Shirley B. Biosynthesis of flavonoids and effects of stress. Curr Opin Plant Biol 2002; 5:218 - 223
- Kobayashi N, DellaPenna D. Tocopherol metabolism, oxidation and recycling under high light stress in Arabidopsis. Plant J 2008; 55:607 - 618
- Board PG, Coggan M, Cappello J, Zhou H, Oakley AJ, Anders MW. S-(4-Nitrophenacyl)glutathione is a specific substrate for glutathione transferase omega 1-1. Anal Biochem 2008; 374:25 - 30