Abstract
Chloroplast function is largely dependent on its resident proteins, most of which are encoded by the nuclear genome and are synthesized in cytosol. Almost all of these are imported through the translocons located in the outer and inner chloroplast envelope membranes. The motor protein that provides the driving force for protein import has been proposed to be Hsp93, a member of the Hsp100 family of chaperones residing in the stroma. Combining in vivo and in vitro approaches, recent publications have provided multiple lines of evidence demonstrating that a stromal Hsp70 system is also involved in protein import into this organelle. Thus it appears that protein import into chloroplasts is driven by two motor proteins, Hsp93 and Hsp70. A perspective on collaboration between these two chaperones is discussed.
Chloroplasts are plant and algal specific organelles where photosynthesis and many other cellular processes take place. Chloroplasts contain ∼3,000 proteins,Citation1,Citation2 with about 100 encoded by the chloroplast genome. In other words, more than 90% of chloroplast proteins are encoded by nuclear genes, synthesized in the cytosol and post-translationally imported into plastids. Most imported proteins are synthesized as precursors with a cleavable N-terminal signal, called a transit peptide. Such precursors are recognized by receptors in the outer envelope membrane, translocated through translocons in the outer and inner envelope membranes of chloroplasts (Toc and Tic), and processed to either their mature- or intermediate-sized forms in the chloroplast stroma.Citation3–Citation8 Thylakoid proteins are further transported to their final destinations via one of four pathways, the cpSec, cpSRP, cpTAT and spontaneous pathways.Citation9–Citation11 It is believed that the precursors are translocated across the envelope membranes in at least partially unfolded conformations and that the import machinery possesses some degree of unfolding activity.Citation12
Three proteins make up the core Toc complex, Toc159, Toc34 and Toc75. The Toc159 and Toc34 proteins are receptors possessing GTPase activities and recognizing transit peptides. Toc75 is a ß-barrel protein that forms the protein-translocating channel across the outer envelope membrane.Citation13 The Tic complex is also formed from multiple subunits. Tic110, Tic21 and Tic20 have each been suggested to function as the channel of the Tic complex.Citation14–Citation16 A ternary complex containing the stroma-facing domain of Tic110, Tic40 and a stromal factor, Hsp93 (a member of the Hsp100 family, possessing two ATPase domains), interacts with incoming precursor proteins.Citation17–Citation26 Hsp93 has been proposed to serve as the import motor.Citation27 Other Tic components include regulatory subunits Tic62, Tic55 and Tic32 that are purported to facilitate redox- and calcium/calmodulin-dependent precursor translocation across the inner envelope membrane (reviewed in ref. Citation3). Tic22 is a peripheral membrane protein associated with the inner envelope and exposed to the intermembrane space.Citation28 It is suggested that Tic22 connects the Toc and Tic translocons during protein import.
A Role for Hsp70 in Chloroplast Protein Import
A central issue concerning protein translocation across biological membranes is the nature of the required energy expenditure.Citation29 In mitochondria and the ER, the driving force for post-translational protein import is thought to be derived from ATP hydrolysis by Hsp70s chaperones that are located in mitochondrial matrix and ER lumen, respectively. Members of the highly conserved Hsp70 family of molecular chaperones are localized in several additional cellular compartments and play crucial roles in protein folding and transport processes. They operate with specific co-chaperones; J-domain proteins (JDPs) which stimulate Hsp70s' ATPase activity, thereby altering the affinity of the chaperone for its substrates, and nucleotide exchange factors (NEFs, GrpE in bacteria, CGE in plastids) which facilitate the exchange of ATP for ADP. Three chloroplast-localized Hsp70s were identified in pea two decades ago,Citation30 one associated with outer envelope membrane and two in stroma.
Despite the initial expectation and computational analysesCitation31 that the stromal Hsp70s would be involved in chloroplast protein import, little evidence for such a role had been obtained until recently. Instead of Hsp70, the stromal Hsp93 was often found to be stably associated with the Tic translocon. This Hsp93 was therefore proposed to provide the driving force for protein import, playing a role in chloroplasts analogous to that of Hsp70s in mitochondria and ER.Citation27
We have recently worked to develop P. patens as a model system in which to investigate the function of the plastid stromal Hsp70s.Citation32 This moss is unique among green plants in performing homologous recombination in the nucleus with introduced DNA at relatively high rates, which makes it an attractive model plant for reverse genetic studies. In addition, we have shown previously that it imports proteins into its chloroplasts in a manner so far indistinguishable from that of flowering plants.Citation33 We demonstrated that one of the three stromal Hsp70s in moss, designated Hsp70-2, was an essential protein in the moss. Similarly, knock out of both stromal co-chaperones, CGE1 and CGE2, caused lethality. Null mutants of these proteins could be obtained only when moss protoplasts were co-transformed with the knockout DNA construct together with an Hsp70-2 or CGE cDNA rescue plasmid, respectively, in which the deletion of the chromosomal gene(s) was complemented by expression of a rescuing cDNA. These data indicated that a stromal Hsp70 system was essential for viability. To gain further insight into the functions of the stromal Hsp70 system, we generated conditional Hsp70-2 mutants based on amino acid substitutions in the yeast mitochondrial Hsp70 that rendered it unstable at elevated temperatures.Citation34,Citation35 When chloroplasts isolated from these putative temperature sensitive mutants were subjected to a brief heat shock, subsequent protein import was reduced compared to import reactions in wild type chloroplasts. In a second approach, we created a CGE1 null and CGE2 knock-down mutant (lcge), in which the total stromal CGE level was only 10–20% of that in wild type. Again, we found that lcge chloroplasts imported precursor proteins at significantly lower rates than did wild type chloroplasts. Lastly, we observed using the lcge mutant, which would be predicted to exhibit prolonged association between an Hsp70 and its substrates, that incoming precursors and the Tic components Tic40 and Hsp93 could be immunoprecipited with Hsp70-2-specific antibodies. These three lines of evidence all pointed to a role for an Hsp70 system in protein import into chloroplasts, most likely as an import motor.
Studies on the role of stromal Hsp70s in chloroplast protein import into chloroplasts in Arabidopsis were conducted independently in the Li laboratory during approximately the same period that we performed our work.Citation36 This group demonstrated that knocking out either of the two Arabidopsis stromal Hsp70s, cpHsc70-1 and cpHsc70-2, caused defects in chloroplast protein import provided that the plants were not too old. Such defects were more severe at early developmental stages, and both precursors of photosynthetic and non-photosynthetic proteins were affected. They found that Hsp70 influenced the translocation step in the import reaction sequence, while binding was not chaperone dependent, which is consistent with our findings in moss.Citation32 This again suggests that the stromal Hsp70 serves as a motor for chloroplast protein import. In addition, immunoprecipitation using a newly generated cpHsc70 antibody captured an incoming precursor protein, as well as stoichiometric amounts of Hsp93 and Tic110.
In an attempt to discern the relationship between the two stromal motor systems represented by Hsp70 and Hsp93, Su and LiCitation36 generated a double mutant of the dominant paralogs of Hsp70 and Hsp93 in Arabidopsis, CPHSC70-1 and HSP93-V.Citation20,Citation21,Citation36 Chloroplasts isolated from this mutant displayed a more severe protein import defect than that observed with each single mutant. The authors proposed from this that the two chaperone motor systems, Hsp70 and Hsp93, function independently. The lethality of another double mutant, cphsp70-1/tic40, seemingly supported this hypothesis because Tic40 is known to modulate the activity of Hsp93,Citation24 making a Tic40 knock out roughly equivalent to an Hsp93 knock out.
Taken together, the two papers by Su and LiCitation36 and by our groupCitation32 make a strong and convincing case that the Hsp70 chaperone system functions as part of the chloroplast protein import motor.
Interaction of the Two Chloroplast Import Motors
To date at least four proteins have been proposed to contribute to the driving force for chloroplast protein import. First, in addition to its role as a precursor receptor, Toc159 was shown to be a GTP-dependent motor capable of driving proteins across the outer envelope membrane.Citation5,Citation37 Second, an Hsp70 from pea was found to be tightly associated with the outer envelope membrane facing the intermembrane space.Citation30 Later, an integral outer membrane Hsp70, very likely the same chaperone mentioned above, was isolated with the import machinery.Citation38 Curiously, attempts to identity this protein in Arabidopsis have thus far not been successful.Citation39 Third, a stromal Hsp93 has been repeatedly shown to be important for chloroplast protein import.Citation17,Citation19–Citation22 Lastly, weCitation32 and the Li laboratoryCitation36 have demonstrated that a stromal HSP70 system is also involved in the protein import process, presumably providing additional force in cooperation with the Hsp93 chaperone. It seems likely that these two chaperones together are responsible for the stromal ATP requirement for the import reaction.Citation40,Citation41 Members of the Hsp100 family of chaperones are also known to influence protein import into mitochondria and the ER, but only when the Hsp70/BiP system is compromised.Citation42 What appears to be unique to the chloroplast situation is that plastids appear to utilize both of these chaperone motors in wild type plants. We would like to know the individual roles of the two chaperones. What are the roles of Hsp93 and Hsp70 in this import process, and do they function sequentially or simultaneously, independently or synergistically?
In order to better describe the relationship between the two chaperone systems based on in vivo studies, we summarized some of the genetic data in . When either chaperone system is completely knocked out (i.e., the Pp_hsp70-2 single and the Pp_cge1/cge2 double knock outs in the moss, and the hsp70-1/hsp70-2 and hsp93-V/hsp93-IIIa) double mutants in Arabidopsis,Citation16,Citation30 neither Arabidopsis nor moss are viable, indicating that both chaperone systems are essential. If one system is functionally minimized while the other system remains intact, as in the moss lcge mutant or the At_hsp70-1, At_hsp93-V and the At_hsp93-V/IIIb mutants in Arabidopsis, import rates in the mutant chloroplasts drop to 40–60% of that in wild type. If the function of both chaperone systems is reduced by genetic manipulation, a more serve defect in protein import is observed (At_hsp70-1/hsp93-V), or it even causes lethality (At_ hsp70-1/tic40). At first blush, these latter experiments would appear to support a model in which the chaperones act independently in parallel to drive protein import. Additional support for this model can be found in the observations by Soll and his colleaguesCitation43 that certain precursors, i.e., prOE33, appear to contact Hsp70 preferentially during import, while others, such as prSSU, preferentially interact with Hsp93. This model of parallel action is also attractive in that it offers a potential explanation for the apparent use of different Toc receptors by different precursors, with the so-called photosynthetic proteins going through the Toc159/Toc34 receptors and house-keeping proteins passing through the Toc130 (120/90)/Toc33 receptors.Citation44 It could be speculated that one of these complexes better feeds the Tic/Hsp93 translocon and the other prefers the Tic/Hsp70 translocon.
In opposition to predictions of a parallel model of chaperone motor function during protein import, both Su and LiCitation36 and weCitation32 found that Hsp70 and Hsp93 could be immunoprecipitated together, and with an incoming precursor, which suggests that they are all in the same complex. This was the case for prSSU, a photosynthetic (non-housekeeping) protein precursor which was observed in early studies to predominantly interact with Hsp93 alone. Here we can make a distinction between two models of chaperone action, depicted in . In one (), the chaperones are seen to act sequentially and rather independently, recruiting their own sets of cofactors and co-chaperones. In this model, the influence of each chaperone system on the activity of the other is not a requirement. shows a different scenario in which the chaperones act synergistically, interacting with each other and with (some of) the same sets of cofactors and co-chaperones. Such a mechanism was suggested for the bichaperone network involving Hsp70 and ClpB (an Hsp100 member) in protein disaggregation.Citation45
Experiments that will distinguish between these different models of chaperone action during chloroplast protein import are currently being pursued in our laboratory. We note that the genetics approach is confounded somewhat by the presence of multiple copies of the genes encoding the two chaperone motors, both in Arabidopsis and in moss. This might explain the approximate 50% reduction in import rates observed in both Hsp70 and Hsp93 mutants noted in . Reduction of their gene copy numbers, as well as the creation of better conditional mutants, might lead to a simplification of the genetics experiments and ultimate clarity on this issue. For these and other experiments, we anticipate that P. patens, with its facility for the isolation of sitedirected knock outs, will continue to be a useful model organism for the study of chloroplast protein import in the coming years.
Figures and Tables
Figure 1 Concurrent with or after translocating across outer envelope membrane, the precursor protein is engaged in the Tic transport channel. While the precursor is in the channel, it interacts with a stromal motor complex that is minimally composed of Tic110, Tic40, Hsp93 and Hsp70. The motor pulls the precursor into stromal at expense of AT P hydrolysis. The two AT Pases, Hsp93 and Hsp70, may cooperate in two different modes: (A) sequentially and independently or (B) simultaneously and synergistically (refer to text for details). Hsp70 associates with and dissociates from the motor complex under regulation of its two co-chaperones, CGE and JDP, to complete an AT P hydrolysis cycle. The transit peptide of the importing precursor is removed by the stromal processing peptidase (SPP).
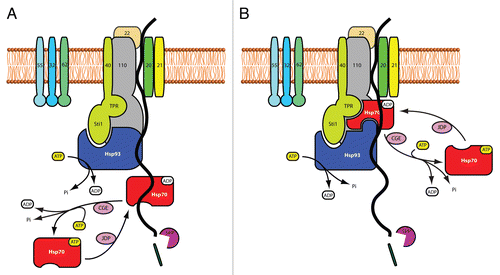
Table 1 Comparison of import defects of mutants from P. patens and Arabidopsis thaliana
Acknowledgments
The work on protein import in P. patens in the Theg laboratory has been supported by grants from the National Science Foundation.
Addendum to:
References
- Leister D. Chloroplast research in the genomic age. Trends Genet 2003; 19:47 - 56
- Lopez-Juez E, Pyke KA. Plastids unleashed: their development and their integration in plant development. Int J Dev Biol 2005; 49:557 - 577
- Kovacs-Bogdan E, Soll J, Bolter B. Protein import into chloroplasts: the Tic complex and its regulation. Biochim Biophys Acta 2010; 1803:740 - 747
- Li HM, Chiu CC. Protein transport into chloroplasts. Annu Rev Plant Biol 2010; 61:157 - 180
- Jarvis P. Targeting of nucleus-encoded proteins to chloroplasts in plants. New Phytol 2008; 179:257 - 285
- Andres C, Agne B, Kessler F. The TOC complex: preprotein gateway to the chloroplast. Biochim Biophys Acta 2010; 1803:715 - 723
- Kessler F, Schnell D. Chloroplast biogenesis: diversity and regulation of the protein import apparatus. Curr Opin Cell Biol 2009; 21:494 - 500
- Inaba T, Schnell DJ. Protein trafficking to plastids: one theme, many variations. Biochem J 2008; 413:15 - 28
- Keegstra K, Cline K. Protein import and routing systems of chloroplasts. Plant Cell 1999; 11:557 - 570
- Gutensohn M, Fan E, Frielingsdorf S, Hanner P, Hou B, Hust B, et al. Toc, Tic, Tat, et al. structure and function of protein transport machineries in chloroplasts. J Plant Physiol 2006; 163:333 - 347
- Cline K, Ettinger WF, Theg SM. Protein-specific energy requirements for protein transport across or into thylakoid membranes. Two lumenal proteins are transported in the absence of ATP. J Biol Chem 1992; 267:2688 - 2696
- Endo T, Kawakami M, Goto A, America T, Weisbeek P, Nakai M. Chloroplast protein import. Chloroplast envelopes and thylakoids have different abilities to unfold proteins. Eur J Biochem 1994; 225:403 - 409
- Hinnah SC, Wagner R, Sveshnikova N, Harrer R, Soll J. The chloroplast protein import channel Toc75: pore properties and interaction with transit peptides. Biophys J 2002; 83:899 - 911
- Heins L, Mehrle A, Hemmler R, Wagner R, Kuchler M, Hormann F, et al. The preprotein conducting channel at the inner envelope membrane of plastids. EMBO J 2002; 21:2616 - 2625
- Chen X, Smith MD, Fitzpatrick L, Schnell DJ. In vivo analysis of the role of atTic20 in protein import into chloroplasts. Plant Cell 2002; 14:641 - 654
- Teng YS, Su YS, Chen LJ, Lee YJ, Hwang I, Li HM. Tic21 is an essential translocon component for protein translocation across the chloroplast inner envelope membrane. Plant Cell 2006; 18:2247 - 2257
- Akita M, Nielsen E, Keegstra K. Identification of protein transport complexes in the chloroplastic envelope membranes via chemical cross-linking. J Cell Biol 1997; 136:983 - 994
- Inaba T, Li M, Alvarez-Huerta M, Kessler F, Schnell DJ. atTic110 functions as a scaffold for coordinating the stromal events of protein import into chloroplasts. J Biol Chem 2003; 278:38617 - 38627
- Nielsen E, Akita M, Davila-Aponte J, Keegstra K. Stable association of chloroplastic precursors with protein translocation complexes that contain proteins from both envelope membranes and a stromal Hsp100 molecular chaperone. EMBO J 1997; 16:935 - 946
- Constan D, Froehlich JE, Rangarajan S, Keegstra K. A stromal hsp100 protein is required for normal chloroplast development and function in Arabidopsis. Plant Physiol 2004; 136:3605 - 3615
- Kovacheva S, Bedard J, Patel R, Dudley P, Twell D, Rios G, et al. In vivo studies on the roles of Tic110, Tic40 and Hsp93 during chloroplast protein import. Plant J 2005; 41:412 - 428
- Kovacheva S, Bedard J, Wardle A, Patel R, Jarvis P. Further in vivo studies on the role of the molecular chaperone, Hsp93, in plastid protein import. Plant J 2007; 50:364 - 379
- Bedard J, Kubis S, Bimanadham S, Jarvis P. Functional similarity between the chloroplast translocon component, Tic40, and the human co-chaperone, Hsp70-interacting protein (Hip). J Biol Chem 2007; 282:21404 - 21414
- Chou ML, Chu CC, Chen LJ, Akita M, Li HM. Stimulation of transit-peptide release and ATP hydrolysis by a cochaperone during protein import into chloroplasts. J Cell Biol 2006; 175:893 - 900
- Chou ML, Fitzpatrick LM, Tu SL, Budziszewski G, Potter-Lewis S, Akita M, et al. Tic40, a membrane-anchored co-chaperone homolog in the chloroplast protein translocon. EMBO J 2003; 22:2970 - 2980
- Stahl T, Glockmann C, Soll J, Heins L. Tic40, a new “old” subunit of the chloroplast protein import translocon. J Biol Chem 1999; 274:37467 - 37472
- Jackson-Constan D, Akita M, Keegstra K. Molecular chaperones involved in chloroplast protein import. Biochim Biophys Acta 2001; 1541:102 - 113
- Kouranov A, Chen X, Fuks B, Schnell DJ. Tic20 and Tic22 are new components of the protein import apparatus at the chloroplast inner envelope membrane. J Cell Biol 1998; 143:991 - 1002
- Alder NN, Theg SM. Energy use by biological protein transport pathways. Trends Biochem Sci 2003; 28:442 - 451
- Marshall JS, DeRocher AE, Keegstra K, Vierling E. Identification of heat shock protein hsp70 homologues in chloroplasts. Proc Natl Acad Sci USA 1990; 87:374 - 378
- Zhang XP, Glaser E. Interaction of plant mitochondrial and chloroplast signal peptides with the Hsp70 molecular chaperone. Trends Plant Sci 2002; 7:14 - 21
- Shi LX, Theg SM. A stromal heat shock protein 70 system functions in protein import into chloroplasts in the moss Physcomitrella patens. Plant Cell 2010; 22:205 - 220
- Hofmann NR, Theg SM. Physcomitrella patens as a model for the study of chloroplast protein transport: conserved machineries between vascular and nonvascular plants. Plant Mol Biol 2003; 53:643 - 654
- Gambill BD, Voos W, Kang PJ, Miao B, Langer T, Craig EA, et al. A dual role for mitochondrial heat shock protein 70 in membrane translocation of preproteins. J Cell Biol 1993; 123:109 - 117
- Kang PJ, Ostermann J, Shilling J, Neupert W, Craig EA, Pfanner N. Requirement for hsp70 in the mitochondrial matrix for translocation and folding of precursor proteins. Nature 1990; 348:137 - 143
- Su PH, Li HM. Stromal Hsp70 is important for protein translocation into pea and Arabidopsis chloroplasts. Plant Cell 2010; 22:1516 - 1531
- Schleiff E, Jelic M, Soll J. A GTP-driven motor moves proteins across the outer envelope of chloroplasts. Proc Natl Acad Sci USA 2003; 100:4604 - 4609
- Schnell DJ, Kessler F, Blobel G. Isolation of components of the chloroplast protein import machinery. Science 1994; 266:1007 - 1012
- Ratnayake RM, Inoue H, Nonami H, Akita M. Alternative processing of Arabidopsis Hsp70 precursors during protein import into chloroplasts. Biosci Biotechnol Biochem 2008; 72:2926 - 2935
- Theg SM, Bauerle C, Olsen LJ, Selman BR, Keegstra K. Internal ATP is the only energy requirement for the translocation of precursor proteins across chloroplastic membranes. J Biol Chem 1989; 264:6730 - 6736
- Scott SV, Theg SM. A new chloroplast protein import intermediate reveals distinct translocation machineries in the two envelope membranes: energetics and mechanistic implications. J Cell Biol 1996; 132:63 - 75
- Schmitt M, Neupert W, Langer T. Hsp78, a Clp homologue within mitochondria, can substitute for chaperone functions of mt-hsp70. EMBO J 1995; 14:3434 - 3444
- Vojta L, Soll J, Bolter B. Requirements for a conservative protein translocation pathway in chloroplasts. FEBS Lett 2007; 581:2621 - 2624
- Kubis S, Patel R, Combe J, Bedard J, Kovacheva S, Lilley K, et al. Functional specialization amongst the Arabidopsis Toc159 family of chloroplast protein import receptors. Plant Cell 2004; 16:2059 - 2077
- Weibezahn J, Tessarz P, Schlieker C, Zahn R, Maglica Z, Lee S, et al. Thermotolerance requires refolding of aggregated proteins by substrate translocation through the central pore of ClpB. Cell 2004; 119:653 - 665