Abstract
Contributions of dietary miRNAs to circulating small RNA profiles would have profound implications for interpretation of miRNA biomarker studies: presumptive disease-specific markers might instead indicate responses to disease-associated quantitative or qualitative dietary alteration. This examination weighs the evidence for a 2-fold hypothesis: first, that ingested biological matter contributes directly to the miRNA complement of body compartments; and second, that these diet-derived exogenous miRNAs (or “xenomiRs”) affect total miRNA profiles as part of a circulating miRNA homeostasis that is altered in many diseases. Homeostasis of high-density lipoprotein (HDL), a known miRNA carrier—provides a model as a proposed component of broader miRNA homeostasis. Further research into the dietary xenomiR hypothesis is needed to ensure rigor in the search for truly disease-specific miRNA biomarkers.
Introduction
Interest in microRNAs has expanded rapidly during the past decade as these short, single-stranded RNA oligonucleotides, once considered a curiosity of the model organism C. elegans, have been identified as managers of mRNA stability and translation,Citation1,Citation2 and as biomarkers of disease.Citation3,Citation4 Presence in non-invasively obtained body fluids renders miRNAs particularly attractive as biomarkers, while the types and provenance of vehicles that protect circulating miRNAs from degradation may provide insight into disease processes. These vehicles include exosomes and microvesicles,Citation5 lipid-protein complexes such as high-density lipoproteins (HDL),Citation6 and protein complexesCitation7,Citation8 similar or identical to those that contain miRNA inside the cell.Citation9 miRNA biomarker studies in oncology have greatly outnumbered investigations of small RNA in all other diseases combinedCitation10—and for good reason, since the largely clonal nature of many cancers simplifies detection of altered profiles in this high-priority group of diseases—but miRNA associations have also been reported in other conditions, from brain and metabolic disorders to infectious diseases.Citation11
It may be premature, however, to conclude from existing biomarker studies that disease-associated miRNA profile changes are necessarily and in their entirety attributable directly to disease. Differential expression of miRNAs could instead be due to unrelated or indirect factors (), but analyses of potential confounders are not usually included in biomarker studies. Perhaps the largest pachyderm in the disease biomarker room, though, is diet, which may exert 3-fold modulation on circulating miRNA profiles:
Table 1. Potentially confounding factors in miRNA disease biomarker studies
1) The indirect influence of dietary substances on endogenous miRNA production;
2) The direct entry into the circulating miRNA population of dietary exogenous miRNAs, or “xenomiRs,” many of which would be largely or wholly indistinguishable, sequence- and function-wise, from endogenous miRNAs; and
3) The indirect influence of dietary xenomiRs and their vehicles on known and unknown homeostatic mechanisms that maintain the concentration of circulating miRNA-containing vehicles (lipoprotein particles, exosomes, microvesicles, and specific protein complexes) and could thus effect changes in the concentration of specific miRNAs in response to diet.
The first influence of diet is well established. From vitamin A to zinc, nutrients affect miRNA production in animalsCitation28-Citation30 and plants.Citation31 This examination will not review these studies but will instead focus on aspects of diet that distinguish it from other potential confounders of disease biomarker studies: food itself contains miRNAs that, if absorbed, would contribute directly to apparent circulating miRNA “expression,” and indirectly by affecting homeostasis of miRNA vehicles such as HDL. Because diet and disease are closely linked, these possibilities present wide-ranging implications for the investigation of miRNA disease biomarkers.
The diet-disease axis
The interrelatedness of disease and diet is evident, for example, in cancers, most cases of which are associated with one or more of the following related, often definitionally overlapping, conditions: anorexia, malnutrition, weight loss, cachexia, and wasting.Citation32-Citation34 Each is usually connected with reduced alimentary intake, often in step with metabolic changes and negative energy balance.Citation35-Citation37 Qualitative dietary changes may also accompany cancers, especially during advanced disease and physical blockages that necessitate enteral or parenteral nutrition with highly processed product that are less likely than fresh food to contain intact miRNAs. (Cooking reduces the amounts of measurable miRNA in vegetable foods by as much as 100-fold,Citation38 while mixing, storage, and drying reduce levels in milk products.Citation39)
Infectious diseases provide a second broad example of the diet-disease axis. Loss of appetite is part of the acute cytokine-induced “sickness behavior” accompanying innate immune responses.Citation40 Specific pathogens may also precipitate chronic nutrition-related conditions, e.g., wasting and other nutrition-related conditions that preceed or define AIDS.Citation41,Citation42 While these conditions may stem from metabolic alterations, alimentary intake is usually changed as well, and appetite stimulants may be necessary for cachexic patients.Citation43 A recent study of nutrition and HCV-infected individuals concluded that “(m)alnutrition occurs early…and progresses relentlessly throughout the spectrum of HCV disease.”Citation44 Finally, some infectious diseases disproportionately afflict individuals with particular socioeconomic backgrounds and attendant diets. Unless controls are carefully selected with attention to nutrition status, diet itself may distinguish patients from controls.
Dietary miRNA uptake and function in animals
Because miRNAs are found in animals and plants, and miRNA-like species are present in fungi,Citation45 almost all fresh foods contain small RNAs that could contribute to the circulating miRNA population. Even processed foods—e.g., cooked rice, potatoes, cabbage,Citation38 and baby milk formulaCitation39—contain miRNAs, albeit at reduced concentrations. That these miRNAs could be delivered to the blood is supported by oral delivery of pharmacological preparations of siRNA.Citation46-Citation48 When protected from the acidic and enzymatic environment of the digestive tract by lipids, proteins, or polysaccharides, ingested small RNA molecules may enter into circulation through the gut. Protection is achieved by artificial shells for therapeutic siRNAs, but multiple protective means are available for food miRNAs, including natural lipid vesiclesCitation49 and protein complexes.Citation38 This process is thought to involve transcytosis across the gut epithelium, particularly by M cells of the Peyer’s patches. Macrophages and T-cells of the gut-associated lymphoid tissue (GALT) have been implicated in subsequent distribution of RNA-containing complexes throughout the body,Citation47 and these cells contribute to the miRNA-containing circulating vesicle population.Citation50,Citation51 It is possible that there are additional, uncharacterized mechanisms for uptake from the GI tract of unprotected small RNAs. Although unshielded miRNAs are degraded much more rapidly in an acidic environment than are miRNAs in fresh food, even these exposed miRNAs may survive for several hours,Citation38 long enough for uptake via receptor-mediated endocytosis or, speculatively, by uncharacterized transporters. (Interestingly, the first miRNA receptor was recently described.Citation52)
Direct support for uptake of dietary xenomiRs was lacking, however, until a recent report that miRNAs from dietary plant matter circulate in the blood, enter multiple tissues, including the liver, and even regulate genes in mammalsCitation38 with rice-based diets. The authors specifically reported that the LDL receptor associated protein LDLRAP1 contained a plant miRNA target site in its 3′ untranslated region and could be regulated by dietary miRNAs. (In the future, further genetic analyses will be useful to identify additional plant miRNA target sites in animal transcripts.) Several reviews of these findings appeared in the popular and scientific press,Citation53-Citation55 often in the context of “cross-kingdom regulation” and speculated implications for herbal medicine and genetic engineering. It is important to emphasize that the data from this study do not suggest that all ingested miRNAs end up in the bloodstream in potentially functional quantities. While the investigators found 25 plant miRNAs by sequencing pools of human serum, only four were identified in all pools. Also, absolute numbers of specific miRNA sequence reads as well as relative proportions were quite variable (), even for the consistently detected miR156 and miR168a, which are among the most conserved plant miRNAs and the most abundant in nutritionally useful parts of plants and in pollen.Citation56 No low-abundance, species-specific miRNAs were reported. While it is thus unlikely that some of the speculative claims of Zhang, et al.’s reviewers are plausible—for example, that miRNAs specific to Chinese folk remedies contribute to their effects,Citation53 or that engineering of plants could expose humans to dangerous miRNAsCitation53—the central conclusion of Zhang et al., stands: abundant dietary xenomiRs enter the mammalian bloodstream and have functional consequences in the liver.Citation38
Table 2. Plant miRNAs detected in human serum (adapted from Zhang, et al.Citation38)
Despite the noted limitations, these findings raise important questions for biomarker research that have not yet been carefully reviewed, first and foremost the possibility that some proportion of miRNA biomarkers in disease studies may be xenomiRs of plant and animal origin. The authors showed that serum and liver plant miRNA concentrations were upregulated 2-fold or more following a dietary switch from processed to fresh food. This magnitude of regulation is consistent both with functional consequences, as underlined by the authors,Citation38,Citation57,Citation58 and with biomarker changes that have been reported in the literature.Citation59-Citation61 If plant miRNAs—with plant-specific chemical modifications and surrounded by proteins foreign to the ingesting animal—can enter the bloodstream, it is probable that dietary animal miRNAs would follow a similar path. Indeed, artificial mammalian miR-150 was delivered to the blood by the oral route.Citation62 Albeit currently without experimental support, animal miRNAs could well undergo preferential uptake due to the “familiar” nature of their sequence and packaging. At the same time, sequence similarity greatly complicates analytical separation of xenomiRs from endogenous animal miRNAs. While the human genome does not contain sequences homologous to abundant plant miRNAs, abundant animal miRNAs are often 100% identical from fish to ruminants to humans. In existing biomarker reports, then, xenomiRs that are present have been conflated with endogenous miRNAs, the apparent concentration of which might thus differ based on diet quantity and quality. Experiments with carefully controlled diet or with food sources containing in vivo labeled miRNAs will be needed to identify these xenomiRs and their contributions to disease profiles.
When disease results in reduced food intake, as is often the case, we might exclusively expect a deficit of certain diet-derived miRNAs. Such a prediction might be overly simplistic, however, because it assumes that the direct effects of dietary miRNAs are the only effects or the predominant effects. However, miRNA profiling studies rarely identify uniform downmodulation of circulating miRNA concentrations, even with diseases such as cancers that can greatly affect food intake. This observation leads us to the next part of the dietary xenomiR hypothesis, proposing a mechanistically multipartite system of circulating miRNA homeostasis that is closely related to hunger impulses and metabolism.
Indirect effects of diet and xenomiRs on circulating miRNA homeostasis—the HDL example
A system of circulating miRNA homeostasis would include sensors to monitor miRNA concentration in the blood and mechanisms of miRNA release or uptake to replenish or deplete the extracellular pool of miRNAs. However, the lipids and proteins that protect extracellular miRNAs from degradation would also presumably preclude recognition by cell-surface receptors (note, though, the recent characterization of TLR8 as a miRNA sensorCitation52). Plausibly, miRNA homeostasis would involve recognition of surface features of the various small vehicles that transport miRNAs. Maintenance of relatively constant levels of miRNA in circulation would then depend on homeostasis of miRNA vehicles. If inclusion of miRNA in these systems has evolutionary consequences and is not merely a bystander effect, each such system would likely involve:
• Feedback controls linking miRNAs and elements of vehicle-specific regulatory pathways
• Incorporation of specific miRNAs into specific vehicles
• A mechanism for sensing concentration of extracellular miRNA vehicles and release or uptake of the vehicles;
• Changes in appetite and/or metabolism that are direct or bystander effects of sensing.
Importantly, although not yet assembled into a whole from the standpoint of miRNA, it appears that each individual piece has already been characterized for at least one known extracellular miRNA vehicle: lipoprotein particles. We will now review this evidence, with a focus on HDL.
miRNAs regulate the machinery of cholesterol homeostasis
Recent advances have highlighted the role of miRNA in cholesterol homeostasis (). miR-33Citation64,Citation65 and miR-26Citation66 family members, as well as miRs-106b,Citation67 -122,Citation68,Citation69 -335,Citation70 -613,Citation71 and -758,Citation72 are reported to modulate governors of cholesterol efflux, uptake, synthesis, and HDL metabolism.Citation73 miRNA precursor transcription, in turn, is inhibited by cholesterol metabolism-related liver X receptors (LXRs).Citation66 Inhibition of miR-33 allows cholesterol efflux to increase, along with HDL particle concentrations, and miR-33 targets include members of additional metabolic pathwaysCitation74 (note, as well, further evidence of reciprocal influence of miRNA networks and metabolism.Citation75,Citation76) In a mouse model of atherosclerosis in which both copies of the LDL receptor gene are knocked out, miR-33 inhibition prompted increases in HDL levels, shrinkage of sclerotic plaques, and decreased inflammatory signaling.Citation77 Higher HDL and concomitantly reduced VLDL were also observed in primates.Citation78 While miRNA-mediated modulation of cholesterol homeostasis would not strictly be required to establish HDL as a regulated carrier of miRNA, the existence of these mechanisms supports the hypothesis that miRNA homeostasis is an evolutionarily conserved corollary of HDL homeostasis.
Figure 1. Cholesterol, HDL, and miRNAs. Mutual relationships of miRNAs and cholesterol transport components in the extracellular space, cytosol, lysosome, and nucleus (not to scale). Inhibitory and stimulatory effects are depicted in red and green, respectively. The mechanism(s) that impart specificity to miRNA HDL loading are unknown. Because of seemingly conflicting results concerning the effects of neutral sphyngomyelinase 2 on miRNA export,Citation6,Citation63 nSMase2 is not depicted here.
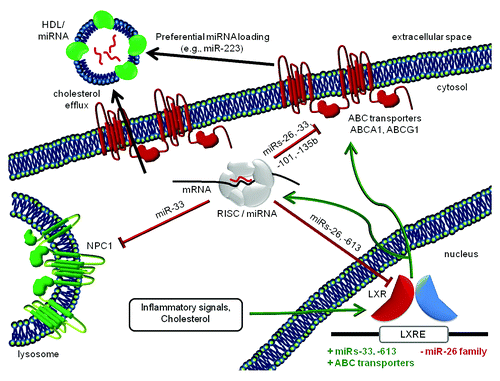
Cholesterol-containing lipoprotein particles carry miRNA
The involvement of the ceramide pathway in miRNA export from cellsCitation79 and the finding that some exported particles were cholesterol rich and exosome or sub-exosome-sizedCitation63,Citation80 prompted an investigation of lipoproteins as potential miRNA carriers.Citation6 In this study by the Remaley group, HDL particles were confirmed as miRNA vehicles, and they were found to harbor a unique miRNA profile: specific miRNAs, for example miR-223, were enriched in HDL RNA in comparison with RNA from the cells of origin (see ).Citation6 Moreover, RNA-free reconstituted HDL particles were capable of miRNA incorporation.Citation6,Citation81 miRNAs were also discovered in low-density lipoproteins, although the miRNA profile of LDL more closely resembled that of exosomes.Citation6
Based upon these studies, it is entirely possible that diet- or disease-related changes in HDL contents or concentration could affect overall circulating miRNA profiles sufficiently to confound biomarker studies. When recombinant HDL were recovered from the blood of mice that received high-fat or regular diets, concentrations of specific miRNAs—including commonly disease-associated miRNAs such as miRs-16, -92, -223, and members of the miR-27, -29, and -30 families—differed by as much as 64-fold or more (Fig. S1), while the human genetic disease hypercholesterolemia was associated with miRNA fold changes in the hundreds to thousands range.Citation6 It is not uncommon for proposed circulating miRNA biomarkers of cancer (for example, esophageal squamous cell carcinoma) to be differentially regulated by 3-fold or less in cancer patients vs. controls, or in before-and-after assessments of resection or chemotherapy.Citation59-Citation61 Thus, although further studies are needed to analyze rigorously and in parallel HDL/LDL concentrations, the miRNA content of these lipoproteins, and overall circulating miRNA concentrations in health and specific diseases, there is ample reason to suspect that some proposed miRNA biomarkers could be significantly affected by HDL miRNAs.
Cellular receptors govern sensing, uptake, and release of miRNA-containing HDL
Circulating HDL particles—and their cargo of enriched miRNAs—are sensed and taken up by liver cells via scavenger receptor SCARB1. Although most abundant in liver, SCARB1 is expressed in other tissues,Citation82,Citation83 suggesting that uptake of HDL miRNA throughout the body involves the HDL-SCARB1 interaction. It also appears that SCARB1 regulates release of HDL. SCARB1 initiates intracellular signalingCitation84 through a scaffold protein, PDZK1.Citation85 Both SCARB1 and PDZK1 knockout mice display increased cholesterol efflux and production of abnormal HDL particles,Citation86,Citation87 along with altered endocrine, GI, and cardiac physiology,Citation85 supporting involvement of this pathway in both uptake and release of HDL miRNA. Interestingly, cholesterol conjugation has been used to deliver therapeutic miRNAs successfully in a mouse model of hepatocellular carcinoma.Citation88
Changes in appetite and/or metabolism are associated with HDL sensing
If homeostases of miRNA and circulating particles are linked and dietary xenomiRs contribute to the extracellular miRNA pool, influence of miRNA vehicle concentration on nutrition intake (i.e., through appetite) would be expected. In cholesterol homeostasis, there is already ample evidence for this. In health, appetite is governed largely by hypothalamic responses to leptin and other hormones, in turn influenced by energy balance and tied to insulin signaling and cholesterol homeostasis. Consider two examples: exercise and treatment with atypical antipsychotic drugs. Endurance training has well-established effects on lipid profiles, decreasing LDL and increasing HDL;Citation89 exercise also suppresses appetite. However, the directionality of this relationship is often unclear. Weight gain and low HDL are also associated with atypical antipsychotic drugs (AAPDs),Citation90 but do appetite changes, caused by blockade of hypothalamic receptors (e.g., histamine H1 receptor), lead to obesity and thus induce dyslipidemias? Or, rather, do AAPDs affect HDL, precipitating appetite alteration and obesity by interfering with insulin resistance? Cases of either scenario may be found, and additional factors may contribute.Citation90 Perhaps the soundest conclusion is to assume a normal reciprocity between HDL concentration (or a state it represents) and appetite. As for HDL, do HDL levels directly affect appetite, or are they simply one of many manifestations of metabolic states that also govern production of hunger hormones? Much remains to be learned about the reciprocal relationships of diet, metabolism, and appetite, but the centrality of blood sensing by the brain is clear, as recently illustrated by the finding that hypothalamus tanycytes, which lie outside the blood-brain barrier in the median eminence, support neurogenesis and respond to high fat intake.Citation91
Conclusions and future studies
Taken together, the studies reviewed here provide the pieces necessary to trace mechanisms of dietary miRNA influence on homeostasis of circulating miRNA profiles. In the most complete model, HDL, a well characterized, diet- and metabolism-related homeostatic system (cholesterol transport) is regulated by miRNA and includes carriers of miRNAs that, when sensed by cellular receptors (e.g., SCARB1), initiate canonical intracellular signaling pathways. Depending on the level and type of signal, this may result in uptake of vehicular small RNA contents into recipient cells as a non-canonical form of signaling—with demonstrated functional consequences and partial depletion of the extracellular miRNA population—or provoke release of additional miRNA vehicles and supplementation of specific portions of the circulating miRNA complement. Furthermore, the HDL system is often deranged in disease. With differential miRNA concentration in HDL vs. cell of origin, disease-associated dietary influence on miRNA profiles could involve apparent up- or downregulation of specific miRNAs, changes that may be only ancillary to pathology. Such miRNAs would not be true disease biomarkers, and manipulating them therapeutically would likely be ineffective.
The findings and syntheses reported in this Point-of-View suggest research directions and precautions that should be taken in ongoing and future miRNA biomarker research ().
Table 3. Future directions and considerations in miRNA biomarker studies
It is hoped that further development and research of the xenomiR hypothesis will help to distinguish between directly disease-associated biomarkers and those that result from altered diet quality or quantity. This outcome will allow investigators to focus on specific biomarkers that are also most promising as targets of future small RNA-based therapeutics.Citation96
Abbreviations: | ||
HDL | = | high-density lipoprotein |
LDL | = | low-density lipoprotein |
HIV | = | human immunodeficiency virus |
HCV | = | hepatitis C virus |
siRNA | = | small interfering RNA |
xenomiR | = | exogenous miRNA |
Additional material
Download Zip (140.4 KB)Acknowledgments
I would like to thank Janice E. Clements for support and insightful comments on the manuscript, Kelly Meulendyke and Luna Alammar for important observations, and all members of the Molecular and Comparative Pathobiology Retrovirus Laboratory for helpful discussions. I declare no conflicts of interest. This work was supported by the Johns Hopkins Brain Sciences Institute and the National Institutes of Health (NS076357).
References
- Bartel DP. MicroRNAs: target recognition and regulatory functions. Cell 2009; 136:215 - 33; http://dx.doi.org/10.1016/j.cell.2009.01.002; PMID: 19167326
- Friedman RC, Farh KK, Burge CB, Bartel DP. Most mammalian mRNAs are conserved targets of microRNAs. Genome Res 2009; 19:92 - 105; http://dx.doi.org/10.1101/gr.082701.108; PMID: 18955434
- Mendell JT, Olson EN. MicroRNAs in stress signaling and human disease. Cell 2012; 148:1172 - 87; http://dx.doi.org/10.1016/j.cell.2012.02.005; PMID: 22424228
- Weber JA, Baxter DH, Zhang S, Huang DY, Huang KH, Lee MJ, et al. The microRNA spectrum in 12 body fluids. Clin Chem 2010; 56:1733 - 41; http://dx.doi.org/10.1373/clinchem.2010.147405; PMID: 20847327
- Kosaka N, Iguchi H, Ochiya T. Circulating microRNA in body fluid: a new potential biomarker for cancer diagnosis and prognosis. Cancer Sci 2010; 101:2087 - 92; http://dx.doi.org/10.1111/j.1349-7006.2010.01650.x; PMID: 20624164
- Vickers KC, Palmisano BT, Shoucri BM, Shamburek RD, Remaley AT. MicroRNAs are transported in plasma and delivered to recipient cells by high-density lipoproteins. Nat Cell Biol 2011; 13:423 - 33; http://dx.doi.org/10.1038/ncb2210; PMID: 21423178
- Turchinovich A, Weiz L, Langheinz A, Burwinkel B. Characterization of extracellular circulating microRNA. Nucleic Acids Res 2011; 39:7223 - 33; http://dx.doi.org/10.1093/nar/gkr254; PMID: 21609964
- Arroyo JD, Chevillet JR, Kroh EM, Ruf IK, Pritchard CC, Gibson DF, et al. Argonaute2 complexes carry a population of circulating microRNAs independent of vesicles in human plasma. Proc Natl Acad Sci U S A 2011; 108:5003 - 8; http://dx.doi.org/10.1073/pnas.1019055108; PMID: 21383194
- Winter J, Diederichs S. Argonaute proteins regulate microRNA stability: Increased microRNA abundance by Argonaute proteins is due to microRNA stabilization. RNA Biol 2011; 8:1149 - 57; http://dx.doi.org/10.4161/rna.8.6.17665; PMID: 21941127
- Oosta G, Razvi E. Analysis of miRNA market trends reveals hotspots of research activity. Epigenomics 2012; 4:237 - 40; http://dx.doi.org/10.2217/epi.12.14; PMID: 22449194
- Weiland M, Gao XH, Zhou L, Mi QS. Small RNAs have a large impact: Circulating microRNAs as biomarkers for human diseases. RNA Biol 2012; 9:850 - 9; http://dx.doi.org/10.4161/rna.20378; PMID: 22699556
- Soci UP, Fernandes T, Hashimoto NY, Mota GF, Amadeu MA, Rosa KT, et al. MicroRNAs 29 are involved in the improvement of ventricular compliance promoted by aerobic exercise training in rats. Physiol Genomics 2011; 43:665 - 73; http://dx.doi.org/10.1152/physiolgenomics.00145.2010; PMID: 21447748
- Liu G, Detloff MR, Miller KN, Santi L, Houlé JD. Exercise modulates microRNAs that affect the PTEN/mTOR pathway in rats after spinal cord injury. Exp Neurol 2012; 233:447 - 56; http://dx.doi.org/10.1016/j.expneurol.2011.11.018; PMID: 22123082
- Nielsen S, Scheele C, Yfanti C, Akerström T, Nielsen AR, Pedersen BK, et al. Muscle specific microRNAs are regulated by endurance exercise in human skeletal muscle. J Physiol 2010; 588:4029 - 37; http://dx.doi.org/10.1113/jphysiol.2010.189860; PMID: 20724368
- Radom-Aizik S, Zaldivar F Jr., Leu SY, Adams GR, Oliver S, Cooper DM. Effects of exercise on microRNA expression in young males peripheral blood mononuclear cells. Clin Transl Sci 2012; 5:32 - 8; http://dx.doi.org/10.1111/j.1752-8062.2011.00384.x; PMID: 22376254
- Baggish AL, Hale A, Weiner RB, Lewis GD, Systrom D, Wang F, et al. Dynamic regulation of circulating microRNA during acute exhaustive exercise and sustained aerobic exercise training. J Physiol 2011; 589:3983 - 94; http://dx.doi.org/10.1113/jphysiol.2011.213363; PMID: 21690193
- Somel M, Guo S, Fu N, Yan Z, Hu HY, Xu Y, et al. MicroRNA, mRNA, and protein expression link development and aging in human and macaque brain. Genome Res 2010; 20:1207 - 18; http://dx.doi.org/10.1101/gr.106849.110; PMID: 20647238
- Huang RS, Gamazon ER, Ziliak D, Wen Y, Im HK, Zhang W, et al. Population differences in microRNA expression and biological implications. RNA Biol 2011; 8:692 - 701; http://dx.doi.org/10.4161/rna.8.4.16029; PMID: 21691150
- Schmidt WM, Spiel AO, Jilma B, Wolzt M, Müller M. In vivo profile of the human leukocyte microRNA response to endotoxemia. Biochem Biophys Res Commun 2009; 380:437 - 41; http://dx.doi.org/10.1016/j.bbrc.2008.12.190; PMID: 19284987
- Witwer KW, Sarbanes SL, Liu J, Clements JE. A plasma microRNA signature of acute lentiviral infection: biomarkers of CNS disease. AIDS 2011; 204:1104 - 14
- Rong H, Liu TB, Yang KJ, Yang HC, Wu DH, Liao CP, et al. MicroRNA-134 plasma levels before and after treatment for bipolar mania. J Psychiatr Res 2011; 45:92 - 5; http://dx.doi.org/10.1016/j.jpsychires.2010.04.028; PMID: 20546789
- Rodrigues AC, Li X, Radecki L, Pan YZ, Winter JC, Huang M, et al. MicroRNA expression is differentially altered by xenobiotic drugs in different human cell lines. Biopharm Drug Dispos 2011; 32:355 - 67; http://dx.doi.org/10.1002/bdd.764; PMID: 21796641
- Wang X, Ye L, Zhou Y, Liu MQ, Zhou DJ, Ho WZ. Inhibition of anti-HIV microRNA expression: a mechanism for opioid-mediated enhancement of HIV infection of monocytes. Am J Pathol 2011; 178:41 - 7; http://dx.doi.org/10.1016/j.ajpath.2010.11.042; PMID: 21224041
- Wang LL, Zhang Z, Li Q, Yang R, Pei X, Xu Y, et al. Ethanol exposure induces differential microRNA and target gene expression and teratogenic effects which can be suppressed by folic acid supplementation. Hum Reprod 2009; 24:562 - 79; http://dx.doi.org/10.1093/humrep/den439; PMID: 19091803
- Guo Y, Chen Y, Carreon S, Qiang M. Chronic Intermittent Ethanol Exposure and Its Removal Induce a Different miRNA Expression Pattern in Primary Cortical Neuronal Cultures. Alcohol Clin Exp Res 2011; 36:1058 - 66; PMID: 22141737
- Soares AR, Pereira PM, Ferreira V, Reverendo M, Simões J, Bezerra AR, et al. Ethanol exposure induces upregulation of specific microRNAs in zebrafish embryos. Toxicol Sci 2012; 127:18 - 28; http://dx.doi.org/10.1093/toxsci/kfs068; PMID: 22298809
- Duttagupta R, Jiang R, Gollub J, Getts RC, Jones KW. Impact of cellular miRNAs on circulating miRNA biomarker signatures. PLoS One 2011; 6:e20769; http://dx.doi.org/10.1371/journal.pone.0020769; PMID: 21698099
- Ross SA, Davis CD. MicroRNA, nutrition, and cancer prevention. Adv Nutr 2011; 2:472 - 85; http://dx.doi.org/10.3945/an.111.001206; PMID: 22332090
- Ryu MS, Langkamp-Henken B, Chang SM, Shankar MN, Cousins RJ. Genomic analysis, cytokine expression, and microRNA profiling reveal biomarkers of human dietary zinc depletion and homeostasis. Proc Natl Acad Sci U S A 2011; 108:20970 - 5; http://dx.doi.org/10.1073/pnas.1117207108; PMID: 22171008
- Davis CD, Ross SA. Evidence for dietary regulation of microRNA expression in cancer cells. Nutr Rev 2008; 66:477 - 82; http://dx.doi.org/10.1111/j.1753-4887.2008.00080.x; PMID: 18667010
- Buhtz A, Pieritz J, Springer F, Kehr J. Phloem small RNAs, nutrient stress responses, and systemic mobility. BMC Plant Biol 2010; 10:64; http://dx.doi.org/10.1186/1471-2229-10-64; PMID: 20388194
- Dy SM, Lorenz KA, Naeim A, Sanati H, Walling A, Asch SM. Evidence-based recommendations for cancer fatigue, anorexia, depression, and dyspnea. J Clin Oncol 2008; 26:3886 - 95; http://dx.doi.org/10.1200/JCO.2007.15.9525; PMID: 18688057
- Teunissen SC, Wesker W, Kruitwagen C, de Haes HC, Voest EE, de Graeff A. Symptom prevalence in patients with incurable cancer: a systematic review. J Pain Symptom Manage 2007; 34:94 - 104; http://dx.doi.org/10.1016/j.jpainsymman.2006.10.015; PMID: 17509812
- Esper DH, Harb WA. The cancer cachexia syndrome: a review of metabolic and clinical manifestations. Nutr Clin Pract 2005; 20:369 - 76; http://dx.doi.org/10.1177/0115426505020004369; PMID: 16207677
- Martinez-Outschoorn UE, Whitaker-Menezes D, Pavlides S, Chiavarina B, Bonuccelli G, Casey T, et al. The autophagic tumor stroma model of cancer or “battery-operated tumor growth”: A simple solution to the autophagy paradox. Cell Cycle 2010; 9:4297 - 306; http://dx.doi.org/10.4161/cc.9.21.13817; PMID: 21051947
- Blum D, Omlin A, Baracos VE, Solheim TS, Tan BH, Stone P, et al, European Palliative Care Research Collaborative. Cancer cachexia: a systematic literature review of items and domains associated with involuntary weight loss in cancer. Crit Rev Oncol Hematol 2011; 80:114 - 44; http://dx.doi.org/10.1016/j.critrevonc.2010.10.004; PMID: 21216616
- Fearon K, Strasser F, Anker SD, Bosaeus I, Bruera E, Fainsinger RL, et al. Definition and classification of cancer cachexia: an international consensus. Lancet Oncol 2011; 12:489 - 95; http://dx.doi.org/10.1016/S1470-2045(10)70218-7; PMID: 21296615
- Zhang L, Hou D, Chen X, Li D, Zhu L, Zhang Y, et al. Exogenous plant MIR168a specifically targets mammalian LDLRAP1: evidence of cross-kingdom regulation by microRNA. Cell Res 2012; 22:107 - 26; http://dx.doi.org/10.1038/cr.2011.158; PMID: 21931358
- Chen X, Gao C, Li H, Huang L, Sun Q, Dong Y, et al. Identification and characterization of microRNAs in raw milk during different periods of lactation, commercial fluid, and powdered milk products. Cell Res 2010; 20:1128 - 37; http://dx.doi.org/10.1038/cr.2010.80; PMID: 20548333
- Konsman JP, Dantzer R. How the immune and nervous systems interact during disease-associated anorexia. Nutrition 2001; 17:664 - 8; http://dx.doi.org/10.1016/S0899-9007(01)00602-5; PMID: 11448593
- Oguntibeju OO, van den Heever WM, Van Schalkwyk FE. The interrelationship between nutrition and the immune system in HIV infection: a review. Pak J Biol Sci 2007; 10:4327 - 38; http://dx.doi.org/10.3923/pjbs.2007.4327.4338; PMID: 19093495
- Faintuch J, Soeters PB, Osmo HG. Nutritional and metabolic abnormalities in pre-AIDS HIV infection. Nutrition 2006; 22:683 - 90; http://dx.doi.org/10.1016/j.nut.2006.03.011; PMID: 16704957
- Argilés JM, López-Soriano FJ, Busquets S. Novel approaches to the treatment of cachexia. Drug Discov Today 2008; 13:73 - 8; http://dx.doi.org/10.1016/j.drudis.2007.10.008; PMID: 18190867
- Ismail FW, Khan RA, Kamani L, Wadalawala AA, Shah HA, Hamid SS, et al. Nutritional status in patients with hepatitis C. J Coll Physicians Surg Pak 2012; 22:139 - 42; PMID: 22414351
- Lee HC, Li L, Gu W, Xue Z, Crosthwaite SK, Pertsemlidis A, et al. Diverse pathways generate microRNA-like RNAs and Dicer-independent small interfering RNAs in fungi. Mol Cell 2010; 38:803 - 14; http://dx.doi.org/10.1016/j.molcel.2010.04.005; PMID: 20417140
- Akhtar S. Oral delivery of siRNA and antisense oligonucleotides. J Drug Target 2009; 17:491 - 5; http://dx.doi.org/10.1080/10611860903057674; PMID: 19530907
- Aouadi M, Tesz GJ, Nicoloro SM, Wang M, Chouinard M, Soto E, et al. Orally delivered siRNA targeting macrophage Map4k4 suppresses systemic inflammation. Nature 2009; 458:1180 - 4; http://dx.doi.org/10.1038/nature07774; PMID: 19407801
- Xu J, Ganesh S, Amiji M. Non-condensing polymeric nanoparticles for targeted gene and siRNA delivery. Int J Pharm 2012; 427:21 - 34; http://dx.doi.org/10.1016/j.ijpharm.2011.05.036; PMID: 21621597
- Hata T, Murakami K, Nakatani H, Yamamoto Y, Matsuda T, Aoki N. Isolation of bovine milk-derived microvesicles carrying mRNAs and microRNAs. Biochem Biophys Res Commun 2010; 396:528 - 33; http://dx.doi.org/10.1016/j.bbrc.2010.04.135; PMID: 20434431
- Schorey JS, Bhatnagar S. Exosome function: from tumor immunology to pathogen biology. Traffic 2008; 9:871 - 81; http://dx.doi.org/10.1111/j.1600-0854.2008.00734.x; PMID: 18331451
- Soo CY, Song Y, Zheng Y, Campbell EC, Riches AC, Gunn-Moore F, et al. Nanoparticle tracking analysis monitors microvesicle and exosome secretion from immune cells. Immunology 2012; 136:192 - 7; http://dx.doi.org/10.1111/j.1365-2567.2012.03569.x; PMID: 22348503
- Fabbri M, Paone A, Calore F, Galli R, Gaudio E, Santhanam R, et al. MicroRNAs bind to Toll-like receptors to induce prometastatic inflammatory response. Proc Natl Acad Sci U S A 2012; 109:E2110 - 6; http://dx.doi.org/10.1073/pnas.1209414109; PMID: 22753494
- Jiang M, Sang X, Hong Z. Beyond nutrients: food-derived microRNAs provide cross-kingdom regulation. Bioessays 2012; 34:280 - 4; http://dx.doi.org/10.1002/bies.201100181; PMID: 22354805
- Hirschi KD. New foods for thought. Trends Plant Sci 2012; 17:123 - 5; http://dx.doi.org/10.1016/j.tplants.2012.01.004; PMID: 22265093
- Kosaka N, Ochiya T. Unraveling the Mystery of Cancer by Secretory microRNA: Horizontal microRNA Transfer between Living Cells. Front Genet 2011; 2:97; PMID: 22303391
- Wei LQ, Yan LF, Wang T. Deep sequencing on genome-wide scale reveals the unique composition and expression patterns of microRNAs in developing pollen of Oryza sativa. Genome Biol 2011; 12:R53; http://dx.doi.org/10.1186/gb-2011-12-6-r53; PMID: 21679406
- Chen C, Ridzon DA, Broomer AJ, Zhou Z, Lee DH, Nguyen JT, et al. Real-time quantification of microRNAs by stem-loop RT-PCR. Nucleic Acids Res 2005; 33:e179; http://dx.doi.org/10.1093/nar/gni178; PMID: 16314309
- Bissels U, Wild S, Tomiuk S, Holste A, Hafner M, Tuschl T, et al. Absolute quantification of microRNAs by using a universal reference. RNA 2009; 15:2375 - 84; http://dx.doi.org/10.1261/rna.1754109; PMID: 19861428
- Kurashige J, Kamohara H, Watanabe M, Tanaka Y, Kinoshita K, Saito S, et al. Serum microRNA-21 is a novel biomarker in patients with esophageal squamous cell carcinoma. J Surg Oncol 2012; 106:188 - 92; http://dx.doi.org/10.1002/jso.23064; PMID: 22354855
- Komatsu S, Ichikawa D, Takeshita H, Tsujiura M, Morimura R, Nagata H, et al. Circulating microRNAs in plasma of patients with oesophageal squamous cell carcinoma. Br J Cancer 2011; 105:104 - 11; http://dx.doi.org/10.1038/bjc.2011.198; PMID: 21673684
- Zhang C, Wang C, Chen X, Yang C, Li K, Wang J, et al. Expression profile of microRNAs in serum: a fingerprint for esophageal squamous cell carcinoma. Clin Chem 2010; 56:1871 - 9; http://dx.doi.org/10.1373/clinchem.2010.147553; PMID: 20943850
- Zhang HS, Wu TC, Sang WW, Ruan Z. MiR-217 is involved in Tat-induced HIV-1 long terminal repeat (LTR) transactivation by down-regulation of SIRT1. Biochim Biophys Acta 2012; 1823:1017 - 23; http://dx.doi.org/10.1016/j.bbamcr.2012.02.014; PMID: 22406815
- Wang K, Zhang S, Weber J, Baxter D, Galas DJ. Export of microRNAs and microRNA-protective protein by mammalian cells. Nucleic Acids Res 2010; 38:7248 - 59; http://dx.doi.org/10.1093/nar/gkq601; PMID: 20615901
- Najafi-Shoushtari SH, Kristo F, Li Y, Shioda T, Cohen DE, Gerszten RE, et al. MicroRNA-33 and the SREBP host genes cooperate to control cholesterol homeostasis. Science 2010; 328:1566 - 9; http://dx.doi.org/10.1126/science.1189123; PMID: 20466882
- Rayner KJ, Suárez Y, Dávalos A, Parathath S, Fitzgerald ML, Tamehiro N, et al. MiR-33 contributes to the regulation of cholesterol homeostasis. Science 2010; 328:1570 - 3; http://dx.doi.org/10.1126/science.1189862; PMID: 20466885
- Sun D, Zhang J, Xie J, Wei W, Chen M, Zhao X. MiR-26 controls LXR-dependent cholesterol efflux by targeting ABCA1 and ARL7. FEBS Lett 2012; 586:1472 - 9; http://dx.doi.org/10.1016/j.febslet.2012.03.068; PMID: 22673513
- Kim J, Yoon H, Ramírez CM, Lee SM, Hoe HS, Fernández-Hernando C, et al. MiR-106b impairs cholesterol efflux and increases Aβ levels by repressing ABCA1 expression. Exp Neurol 2012; 235:476 - 83; http://dx.doi.org/10.1016/j.expneurol.2011.11.010; PMID: 22119192
- Boutz DR, Collins PJ, Suresh U, Lu M, Ramírez CM, Fernández-Hernando C, et al. Two-tiered approach identifies a network of cancer and liver disease-related genes regulated by miR-122. J Biol Chem 2011; 286:18066 - 78; http://dx.doi.org/10.1074/jbc.M110.196451; PMID: 21402708
- Esau C, Davis S, Murray SF, Yu XX, Pandey SK, Pear M, et al. miR-122 regulation of lipid metabolism revealed by in vivo antisense targeting. Cell Metab 2006; 3:87 - 98; http://dx.doi.org/10.1016/j.cmet.2006.01.005; PMID: 16459310
- Nakanishi N, Nakagawa Y, Tokushige N, Aoki N, Matsuzaka T, Ishii K, et al. The up-regulation of microRNA-335 is associated with lipid metabolism in liver and white adipose tissue of genetically obese mice. Biochem Biophys Res Commun 2009; 385:492 - 6; http://dx.doi.org/10.1016/j.bbrc.2009.05.058; PMID: 19460359
- Ou Z, Wada T, Gramignoli R, Li S, Strom SC, Huang M, et al. MicroRNA hsa-miR-613 targets the human LXRα gene and mediates a feedback loop of LXRα autoregulation. Mol Endocrinol 2011; 25:584 - 96; http://dx.doi.org/10.1210/me.2010-0360; PMID: 21310851
- Ramirez CM, Dávalos A, Goedeke L, Salerno AG, Warrier N, Cirera-Salinas D, et al. MicroRNA-758 regulates cholesterol efflux through posttranscriptional repression of ATP-binding cassette transporter A1. Arterioscler Thromb Vasc Biol 2011; 31:2707 - 14; http://dx.doi.org/10.1161/ATVBAHA.111.232066; PMID: 21885853
- Fernández-Hernando C, Moore KJ. MicroRNA modulation of cholesterol homeostasis. Arterioscler Thromb Vasc Biol 2011; 31:2378 - 82; http://dx.doi.org/10.1161/ATVBAHA.111.226688; PMID: 22011750
- Dávalos A, Goedeke L, Smibert P, Ramírez CM, Warrier NP, Andreo U, et al. miR-33a/b contribute to the regulation of fatty acid metabolism and insulin signaling. Proc Natl Acad Sci U S A 2011; 108:9232 - 7; http://dx.doi.org/10.1073/pnas.1102281108; PMID: 21576456
- Shah AA, Leidinger P, Keller A, Wendschlag A, Backes C, Baus-Loncar M, et al. The intestinal factor Tff3 and a miRNA network regulate murine caloric metabolism. RNA Biol 2011; 8:77 - 81; http://dx.doi.org/10.4161/rna.8.1.13687; PMID: 21289491
- Tarling EJ, Edwards PA. Dancing with the sterols: Critical roles for ABCG1, ABCA1, miRNAs, and nuclear and cell surface receptors in controlling cellular sterol homeostasis. Biochim Biophys Acta 2012; 1821:386 - 95; http://dx.doi.org/10.1016/j.bbalip.2011.07.011; PMID: 21824529
- Rayner KJ, Sheedy FJ, Esau CC, Hussain FN, Temel RE, Parathath S, et al. Antagonism of miR-33 in mice promotes reverse cholesterol transport and regression of atherosclerosis. J Clin Invest 2011; 121:2921 - 31; http://dx.doi.org/10.1172/JCI57275; PMID: 21646721
- Rayner KJ, Esau CC, Hussain FN, McDaniel AL, Marshall SM, van Gils JM, et al. Inhibition of miR-33a/b in non-human primates raises plasma HDL and lowers VLDL triglycerides. Nature 2011; 478:404 - 7; http://dx.doi.org/10.1038/nature10486; PMID: 22012398
- Kosaka N, Iguchi H, Yoshioka Y, Takeshita F, Matsuki Y, Ochiya T. Secretory mechanisms and intercellular transfer of microRNAs in living cells. J Biol Chem 2010; 285:17442 - 52; http://dx.doi.org/10.1074/jbc.M110.107821; PMID: 20353945
- Chen TS, Lai RC, Lee MM, Choo AB, Lee CN, Lim SK. Mesenchymal stem cell secretes microparticles enriched in pre-microRNAs. Nucleic Acids Res 2010; 38:215 - 24; http://dx.doi.org/10.1093/nar/gkp857; PMID: 19850715
- Vickers KC, Remaley AT. Lipid-based carriers of microRNAs and intercellular communication. Curr Opin Lipidol 2012; 23:91 - 7; http://dx.doi.org/10.1097/MOL.0b013e328350a425; PMID: 22418571
- Yanai I, Benjamin H, Shmoish M, Chalifa-Caspi V, Shklar M, Ophir R, et al. Genome-wide midrange transcription profiles reveal expression level relationships in human tissue specification. Bioinformatics 2005; 21:650 - 9; http://dx.doi.org/10.1093/bioinformatics/bti042; PMID: 15388519
- Shmueli O, Horn-Saban S, Chalifa-Caspi V, Shmoish M, Ophir R, Benjamin-Rodrig H, et al. GeneNote: whole genome expression profiles in normal human tissues. C R Biol 2003; 326:1067 - 72; http://dx.doi.org/10.1016/j.crvi.2003.09.012; PMID: 14744114
- Assanasen C, Mineo C, Seetharam D, Yuhanna IS, Marcel YL, Connelly MA, et al. Cholesterol binding, efflux, and a PDZ-interacting domain of scavenger receptor-BI mediate HDL-initiated signaling. J Clin Invest 2005; 115:969 - 77; PMID: 15841181
- Kocher O, Birrane G, Tsukamoto K, Fenske S, Yesilaltay A, Pal R, et al. In vitro and in vivo analysis of the binding of the C terminus of the HDL receptor scavenger receptor class B, type I (SR-BI), to the PDZ1 domain of its adaptor protein PDZK1. J Biol Chem 2010; 285:34999 - 5010; http://dx.doi.org/10.1074/jbc.M110.164418; PMID: 20739281
- Rigotti A, Trigatti BL, Penman M, Rayburn H, Herz J, Krieger M. A targeted mutation in the murine gene encoding the high density lipoprotein (HDL) receptor scavenger receptor class B type I reveals its key role in HDL metabolism. Proc Natl Acad Sci U S A 1997; 94:12610 - 5; http://dx.doi.org/10.1073/pnas.94.23.12610; PMID: 9356497
- Kocher O, Yesilaltay A, Cirovic C, Pal R, Rigotti A, Krieger M. Targeted disruption of the PDZK1 gene in mice causes tissue-specific depletion of the high density lipoprotein receptor scavenger receptor class B type I and altered lipoprotein metabolism. J Biol Chem 2003; 278:52820 - 5; http://dx.doi.org/10.1074/jbc.M310482200; PMID: 14551195
- He XX, Chang Y, Meng FY, Wang MY, Xie QH, Tang F, et al. MicroRNA-375 targets AEG-1 in hepatocellular carcinoma and suppresses liver cancer cell growth in vitro and in vivo. Oncogene 2011; 31:3357 - 69; PMID: 22056881
- Zanella AM, Nakazone MA, Pinhel MA, Souza DR. Lipid profile, apolipoprotein A-I and oxidative stress in professional footballers, sedentary individuals, and their relatives. Arq Bras Endocrinol Metabol 2011; 55:121 - 6; http://dx.doi.org/10.1590/S0004-27302011000200004; PMID: 21584429
- Stahl SM, Mignon L, Meyer JM. Which comes first: atypical antipsychotic treatment or cardiometabolic risk?. Acta Psychiatr Scand 2009; 119:171 - 9; http://dx.doi.org/10.1111/j.1600-0447.2008.01334.x; PMID: 19178394
- Lee DA, Bedont JL, Pak T, Wang H, Song J, Miranda-Angulo A, et al. Tanycytes of the hypothalamic median eminence form a diet-responsive neurogenic niche. Nat Neurosci 2012; 15:700 - 2; http://dx.doi.org/10.1038/nn.3079; PMID: 22446882
- Collino F, Deregibus MC, Bruno S, Sterpone L, Aghemo G, Viltono L, et al. Microvesicles derived from adult human bone marrow and tissue specific mesenchymal stem cells shuttle selected pattern of miRNAs. PLoS One 2010; 5:e11803; http://dx.doi.org/10.1371/journal.pone.0011803; PMID: 20668554
- Hunter MP, Ismail N, Zhang X, Aguda BD, Lee EJ, Yu L, et al. Detection of microRNA expression in human peripheral blood microvesicles. PLoS One 2008; 3:e3694; http://dx.doi.org/10.1371/journal.pone.0003694; PMID: 19002258
- Müller G, Schneider M, Biemer-Daub G, Wied S. Microvesicles released from rat adipocytes and harboring glycosylphosphatidylinositol-anchored proteins transfer RNA stimulating lipid synthesis. Cell Signal 2011; 23:1207 - 23; http://dx.doi.org/10.1016/j.cellsig.2011.03.013; PMID: 21435393
- Montecalvo A, Larregina AT, Shufesky WJ, Stolz DB, Sullivan ML, Karlsson JM, et al. Mechanism of transfer of functional microRNAs between mouse dendritic cells via exosomes. Blood 2012; 119:756 - 66; http://dx.doi.org/10.1182/blood-2011-02-338004; PMID: 22031862
- Petrocca F, Lieberman J. Micromanipulating cancer: microRNA-based therapeutics?. RNA Biol 2009; 6:335 - 40; http://dx.doi.org/10.4161/rna.6.3.9013; PMID: 19535911
- Witwer KW, Clements JE. Evidence for miRNA expression differences of HIV-1-positive, treatment-naive patients and elite suppressors: a re-analysis. Blood 2012; 119:6395 - 6; http://dx.doi.org/10.1182/blood-2012-02-412742; PMID: 22745299
- Fare TL, Coffey EM, Dai H, He YD, Kessler DA, Kilian KA, et al. Effects of atmospheric ozone on microarray data quality. Anal Chem 2003; 75:4672 - 5; http://dx.doi.org/10.1021/ac034241b; PMID: 14632079
- Lusa L, McShane LM, Reid JF, De Cecco L, Ambrogi F, Biganzoli E, et al. Challenges in projecting clustering results across gene expression-profiling datasets. J Natl Cancer Inst 2007; 99:1715 - 23; http://dx.doi.org/10.1093/jnci/djm216; PMID: 18000217
- Pritchard CC, Kroh E, Wood B, Arroyo JD, Dougherty KJ, Miyaji MM, et al. Blood cell origin of circulating microRNAs: a cautionary note for cancer biomarker studies. Cancer Prev Res (Phila) 2012; 5:492 - 7; http://dx.doi.org/10.1158/1940-6207.CAPR-11-0370; PMID: 22158052
- Li LM, Hu ZB, Zhou ZX, Chen X, Liu FY, Zhang JF, et al. Serum microRNA profiles serve as novel biomarkers for HBV infection and diagnosis of HBV-positive hepatocarcinoma. Cancer Res 2010; 70:9798 - 807; http://dx.doi.org/10.1158/0008-5472.CAN-10-1001; PMID: 21098710
- Chen Y, Li L, Zhou Z, Wang N, Zhang CY, Zen K. A pilot study of serum microRNA signatures as a novel biomarker for occult hepatitis B virus infection. Med Microbiol Immunol 2012; 201:389 - 95; PMID: 22392036