Abstract
Ribosome synthesis requires a multitude of cofactors, among them DExD/H-box RNA helicases. Bacterial RNA helicases involved in ribosome assembly are not essential, while eukaryotes strictly require multiple DExD/H-box proteins that are involved in the much more complex ribosome biogenesis pathway. Here, RNA helicases are thought to act in structural remodeling of the RNPs including the modulation of protein binding, and they are required for allowing access or the release of specific snoRNPs from pre-ribosomes. Interestingly, helicase action is modulated by specific cofactors that can regulate recruitment and enzymatic activity. This review summarizes the current knowledge and focuses on recent findings and open questions on RNA helicase function and regulation in ribosome synthesis.
Keywords: :
Introduction
Ribosomes mediate the translation of the nucleotide sequence of mRNAs (mRNAs) into the amino acid sequence of proteins and are essential in all forms of life. They sediment at 70S in bacteria and 80S in eukaryotes, and consist of a small and a large subunit of 30S and 50S in bacteria and of 40S and 60S in eukaryotes. Bacterial ribosomes contain 21 ribosomal proteins (RPs) and the 16S rRNA (rRNA) in the small subunit (SSU), and 34 RPs as well as the 5S and 23S rRNAs in the large subunit (LSU; reviewed in ref. Citation1). In eukaryotes, the composition, assembly and function of ribosomes in mitochondria and chloroplasts are thought to be similar to bacteria, while the biogenesis and function of cytoplasmic ribosomes is much more complex.Citation2,Citation3 Ribosomes of the eukaryotic type contain additional RPs and sequence elements in the rRNAs, termed eukaryotic expansion segments, leading to the higher molecular weight of the ribosomal subunits. The SSU consists of the 18S rRNA as well as 32 or 33 RPs in yeast or mammalian cells, respectively, while the LSU contains 46 RPs and the 25S/28S, 5.8S and 5S rRNAs. In all domains of life, most (or in bacteria all) rRNAs are initially part of a large primary transcript, which is processed in multiple steps to derive the mature rRNAs that form a large part of the catalytic core of the ribosome.Citation1,Citation3,Citation4 The processing of the rRNAs and the assembly of the ribosomal subunits requires a number of non-ribosomal cofactors, such as nucleases, GTPases, RNA modifying enzymes and RNA helicases.
RNA helicases bind and remodel RNA and RNP complexes in an ATP- (or NTP-) dependent manner (reviewed in refs. Citation5–Citation7). The proteins possess a structurally conserved helicase core containing characteristic sequence elements and structural motifs. Additional domains are thought to confer specificity by recruitment to target RNPs, while the conserved helicase core interacts with RNA in a sequence-independent manner. Some DExH-box proteins have been suggested to act processively and translocate along RNA, whereas DEAD-box proteins are thought to mediate duplex unwinding by local strand separation or to act as RNA clamps in annealing (see refs. Citation8 and Citation9).
This review focuses on the DExD/H-box proteins involved in the biogenesis of ribosomal subunits in bacteria and eukaryotes, as RNA helicase function in the archaeal pathway has remained unexplored so far. It is believed that most of them act as ATP-dependent RNA helicases that function in unwinding of double-stranded RNA, assist dissociation of RNA-binding proteins or mediate structural remodeling of pre-ribosomal complexes. We discuss the current knowledge on DExD/H-box proteins involved in ribosome synthesis in bacteria and eukaryotes, with focus on major advances in recent years and on key questions regarding RNA helicase function and regulation in this pathway.
RNA Helicases in Bacterial Ribosome Assembly
In bacterial ribosome assembly, a single primary transcript contains the sequences of all mature rRNAs ().Citation1,Citation4 The transcript is cleaved by RNase III into the 17S, 23S and 9S pre-rRNAs and these are further processed. The mature 16S rRNA is obtained after cleavages by RNase E and G at the 5'-end and by an unknown RNase at the 3'-end. 5'-end processing of the 5S and 23S rRNAs is mediated by an unknown RNase (in case of 5S possibly by RNase E), while the 3'-ends are generated by RNase T (). A set of additional cofactors facilitates bacterial ribosome assembly in vivo (reviewed in ref. Citation1), but, interestingly, biologically active ribosomal subunits could be assembled in vitro from purified components.Citation10,Citation11
Figure 1. Processing of rRNA in bacteria. The schematic representation shows the rRNA processing pathway in Escherichia coli. Cleavage sites and the corresponding nucleases are indicated. Adapted from ref. Citation1.
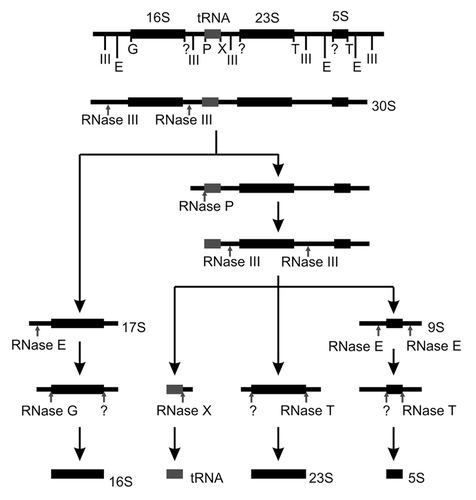
Bacterial ribosome assembly is best understood in Escherichia coli.Citation1,Citation12 Although none of the five DEAD-box RNA helicases found in E. coli is essential, four of them have been implicated in ribosome assembly.
The RNA helicase SrmB was originally identified as a multicopy suppressor of a temperature-sensitive (ts) mutation in the essential RP L24.Citation13 It was further shown to directly and cooperatively interact with the RPs L4, L24 and with 23S rRNA, indicating a mode of early recruitment into 40S particles, which are considered as LSU precursors.Citation14 Deletion of the gene encoding the DEAD-box RNA helicase SrmB results in defects in LSU assembly, causing a reduced growth rate at low temperatures.Citation15 SrmB is recruited through interactions with L4, L24 and 23S rRNA (domain I), and the helicase is required for incorporation of the RP L13 in early stages of LSU assembly. Using rRNA mutants the binding site of SrmB on the rRNA was, however, mapped to sequences in domain II of the 23S rRNA,Citation16 indicating that SrmB might act in a different region of the pre-ribosome than it is originally recruited to.
The cold-shock DEAD-box protein A (CsdA/DeaD) was identified as a suppressor of temperature-sensitive mutations in rpsB, the gene encoding the ribosomal protein S2.Citation17 The expression of CsdA is specifically induced at temperatures below 20°C to facilitate LSU assembly.Citation18 At low temperatures, lack of CsdA leads to slow growth and accumulation of 40S precursor particles.Citation19,Citation20 CsdA binds 50S precursors and was suggested to facilitate late steps of LSU assembly. It was further shown to possess RNA helicase activity in vitro.Citation18 Interestingly, overexpression of CsdA could rescue defects caused by deletion of SrmB, which is thought to act earlier in the pathway.Citation19 Conversely, increased levels of the RNA helicase RhlE can compensate for loss of CsdA at low temperatures, while RhlE has the opposite effect on srmB deletions.Citation21,Citation22
Deletion of the dbpA gene, encoding another RNA helicase, shows no growth defect,Citation20 while mutation of the arginine finger (R331A) in the active site strongly reduces growth and shows dominant negative effects that lead to accumulation of 45S LSU intermediates.Citation23,Citation24 E. coli DbpA and the related Bacillus subtilis YxiN were extensively characterized in vitro and mutations, such as R331A in DbpA, were shown to strongly reduce ATPase and helicase activity (see for example refs. Citation23, Citation25, Citation26) Interestingly, DbpA was shown to specifically recognize helix 92 in the 23S rRNA.Citation27 In turn, the rRNA sequence of this location specifically stimulates ATPase activity of the RNA helicase and mutations in the sequence reduce ATPase stimulation, suggesting a sequence-specific recognition of this RNA. To our knowledge, the related RNA helicases DbpA and YxiN are the only DEAD-box RNA helicases for which sequence-specific stimulation of helicase activity has been described so far.
Eukaryotic organelles, like mitochondria and chloroplasts, are thought to possess a ribosome assembly pathway similar to that in bacteria. To date, only few RNA helicases involved in organellar ribosome biogenesis have been identified and the proteins remain to be further functionally analyzed.Citation28,Citation29
RNA Helicases Involved in Ribosome Biogenesis in Saccharomyces Cerevisiae
In cytoplasmic ribosomes of eukaryotes, three of the four rRNAs, the 18S, 5.8S and 25S/28S rRNAs, are derived from a common RNA Polymerase I (Pol I) transcript, the 35S pre-rRNA in yeast, while the 5S rRNA is transcribed separately by Pol III (reviewed in ref. Citation2, Citation3 and Citation30–Citation32). Up to 2000 ribosomes are made per minute in exponentially growing yeast cells, and 60% of the cellular transcription activity was estimated to supply this pathway.Citation33
Ribosome synthesis in eukaryotes involves a plethora of cofactors. The pathway is best understood in the yeast Saccharomyces cerevisiae, where more than 200 protein cofactors and 75 small nucleolar RNAs (snoRNAs) are recruited to various intermediates.Citation3,Citation30 The biogenesis of ribosomes is initiated by the nucleolar transcription of the 35S pre-rRNA and co-transcriptional assembly of the 90S pre-ribosome, which contains a set of RPs and various cofactors. Besides the sequences of the 18S, 5.8S and 25S rRNAs, the 35S pre-rRNA includes the 5'- and 3'-external transcribed spacers (ETS) as well as the internal transcribed spacers (ITS) 1 and 2 (), which contain processing sites for multiple exo- and endonucleases.Citation3 Early cleavages occur at the sites A0 and A1 followed by cleavage at A2, which leads to separation of the biogenesis pathways of the two ribosomal subunits. The A2 cleavage releases the 20S pre-rRNA in the pre-SSU and can already occur cotranscriptionally.Citation34,Citation35 Further maturation of the SSU involves the endonucleolytic cleavage of the 20S pre-rRNA to generate the 18S rRNA in the cytoplasm, while processing of the 27SA2 intermediate in the pre-LSU continues in the nucleus following either of two alternative pathways that lead to the generation of export-competent pre-60S particles containing the 25S and 5S mature rRNAs and the long or short form of the 6S pre-rRNA, which is matured to the 5.8SL/S rRNA in the cytoplasm (; reviewed in refs. Citation2, Citation3, Citation30). In addition to the complex processing pathway, the rRNA undergoes an extensive series of modifications, most of which are predicted to occur cotranscriptionally. The majority of modifications are mediated by small nucleolar RNPs (snoRNPs), which contain snoRNAs that basepair with the cognate sequence of the pre-rRNA flanking the modification site and thereby guide the enzymes to their target sites (reviewed in ref. Citation36). snoRNPs can catalyze 2’-hydroxyl methylation (box C/D snoRNPs) or pseudouridylation (box H/ACA snoRNPs) of specific residues, or snoRNA basepairing facilitates the processing of the pre-rRNAs.Citation37,Citation38 The duplex of snoRNAs and pre-rRNA can involve more than 20 nucleotides, and due to the expected stability of these helices release of snoRNPs from pre-ribosomes was predicted to require the action of proteins, such as RNA helicases.Citation30,Citation36,Citation39,Citation40 In addition, RNA helicases were suggested to mediate structural remodeling of ribosomes, including the rearrangement of RNA secondary structures and allowing access or release of RNA binding proteins.
Figure 2. Processing of rRNA in Saccharomyces cerevisiae. The schematic representation shows the rRNA processing pathway in yeast. The direction of transcription is indicated for a rDNA repeat by horizontal arrows and processing sites are marked by small vertical arrows. The nuclear envelope is represented by horizontal bars, and nucleus (Nu) and cytoplasm (Cy) are indicated.
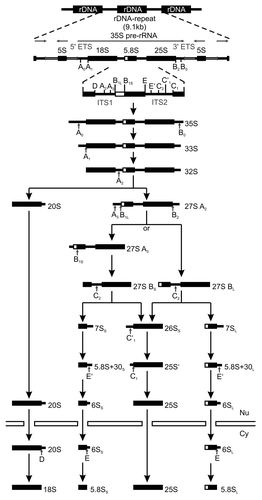
Phenotypic classification of RNA helicases involved in ribosome biogenesis
First evidence for RNA helicase involvement in the process of ribosome biogenesis in yeast was found in 1992 by the analysis of cold-sensitive mutant strains, which revealed Drs1 as an RNA helicase that affects pre-rRNA processing.Citation39,Citation41 To date, 19 putative RNA helicases are thought to directly participate in ribosome synthesis in yeast. With the exception of Mtr4, they all are members of the helicase superfamily 2 (see refs. Citation3 and Citation5). Initial description of pre-rRNA helicases mostly included localization or cell fractionation studies and mapped them predominantly to the nucleolus, the primary site of ribosome biogenesis and the nucleus. Further characterization involved deletion/depletion experiments and analysis of strains bearing mutations in conserved DExD/H motives, some of which displayed temperature dependent growth phenotypes (see ; reviewed in refs. Citation3, Citation5 and Citation32). Results demonstrated that all RNA helicases involved in ribosome biogenesis are essential for cell viability, except for Dbp2, Dbp3 and Dbp7, where genetic deletion causes growth retardation (see and refs. Citation42–Citation44). Density gradient centrifugation followed by polysome profiling as well as pulse-chase and northern blot analysis of RNA helicase depleted cells elucidated RNA helicase influence on precursor and mature rRNA formation and constituted the basis for classification. Seven RNA helicases (Dbp4, Dbp8, Dhr1, Dhr2, Fal1, Rok1, Rrp3) have been implicated in SSU biogenesis, while ten (Dbp2, Dbp3, Dbp6, Dbp7, Dbp9, Dbp10, Drs1, Mak5, Mtr4, Spb4) participate in synthesis of LSU precursors, and two RNA helicases (Prp43, Has1) contribute to the biogenesis of both subunits (see ).
Table 1. RNA helicases involved in ribosome biogenesis in Saccharomyces cerevisiae
Involvement of RNA helicases in early stages (90S) and 40S biogenesis, for example of Dbp4, Dhr1, Dhr2, Fal1 and Rrp3, typically coincides with defects in processing at the sites A0, A1 or in ITS1.Citation45-Citation50 This results in a reduction in 18S rRNA and its direct precursor 20S as well as in enrichment of the 35S pre-rRNA and of aberrant intermediates, such as 23S and 21S pre-rRNAs. These aberrant pre-rRNAs are probably not processed further but rather targeted for degradation. 23S appears after cleavage at site A3 under conditions where processing at sites A0 to A2 is compromised. Besides the 27SA2 pre-rRNA, none of the LSU precursors is usually significantly affected by SSU helicase impairment, as cleavage at site A3 rescues LSU biogenesis.
The deletion or depletion of LSU helicases causes different phenotypes in pre-rRNA processing. Most LSU helicases, such as Dbp2, Dbp3, Dbp6 and Dbp7 seem to act at early or intermediate stages of 60S biogenesis. Besides reduction of mature rRNAs (25S and 5.8S), these helicases generally cause some accumulation of SSU precursors and 23S as well as a minor reduction in 18S rRNA.Citation43,Citation51-Citation54 These processing phenotypes are sometimes similar to defects observed for RNA helicases involved in formation of the SSU, though usually not as drastic. However, their primary implication into maturation of the LSU is in most cases clearly demonstrated by a decrease of mature 60S subunits in polysome profiles. In contrast to the other RNA helicases, deletion of Dbp3 induces accumulation of 27SA2 precursors and it was shown to facilitate cleavage at position A3 by the RNase MRP.Citation54
Mak5 has been described to bind to both early and late intermediates.Citation51,Citation55 Depletion of the RNA helicases causes similar defects as those described for the early LSU helicases, however, no significant effects on SSU intermediates were observed. A different phenotype of processing defects in LSU assembly is caused by depletion of Spb4 or Dbp10.Citation56-Citation58 This causes the characteristic accumulation of the 27SB rRNA precursor, which indicates defects in ITS2 cleavage at site C2 and leads to their classification as late acting LSU RNA helicases. Spb4 was shown to be associated with both early and late pre-60S particles, and proposed to dissociate after 27SB processing.Citation59 These findings might suggest that Spb4 already binds to earlier intermediates, but that activation of the helicase occurs later to allow 27SB processing and its dissociation from pre-ribosomes.
Finally, depletion of the essential RNA helicases Prp43 or Has1 causes changes in the levels of rRNA intermediates and mature RNAs of the SSU and the LSU, indicating a dual role of these proteins in both pathways.Citation60-Citation63
RNA helicase functions in yeast ribosome biogenesis
Besides the classification of the RNA helicases based on pre-rRNA processing defects and polysome profiles, information on the molecular function of these proteins is mostly lacking. Analyses of the composition of pre-ribosomal complexes and protein interaction studies have mapped many helicases to distinct pre-ribosomal intermediates (see for example refs. Citation64–Citation66). Some of these particles contain several RNA helicases and in a few cases, a subcomplex containing RNA helicases or direct interaction partners has been identified.
One of the most prominent complexes in ribosome biogenesis is the SSU processome (see ref. Citation67 and reviewed in ref. Citation68). This 2.2 MDa particle assembles cotranscriptionally from pre-formed subcomplexes, such as the UTP-A/tUTP, the UTP-B and the UTP-C complexes, as well as snoRNPs and individual proteins (see refs. Citation64, Citation69–Citation72). Associated snoRNPs include the essential snR30, U3 and U14 snoRNPs, which are required for early cleavages of the pre-rRNA (reviewed in refs. Citation36, Citation37). Among a multitude of other cofactors, the RNA helicases Dbp4, Dbp8, Dhr1, Has1, Dhr2, Fal1, Prp43, Rok1 and Rrp3 were found in the SSU processome. While Dhr1 was found to associate with Mpp10 as well as the U3 snoRNP and suggested to remodel the U3-pre-rRNA pseudoknot structure,Citation46,Citation70 the closely related Dhr2 was found to interact with Utp25 and Nop19.Citation73 Utp25 is an essential RNA helicase-like protein that shows only limited conservation of the helicase core domain.Citation74,Citation75 This domain seems to be important for Utp3/Sas10 interaction in the SSU processome, however, RNA helicase activity is unlikely since even the highly conserved residues of motif Ia, usually implicated in substrate binding, seem to be dispensable for Utp25 function.
Dbp8 is another DEAD-Box protein involved in maturation of the SSU.Citation76 Its cofactor Esf2, a nucleolar RNA binding protein, was shown to be associated with the U3 snoRNP.Citation77 The C-terminal domain of Esf2 allows Dbp8 binding and stimulates its ATPase activity.Citation78 Since Esf2 is suggested to bind preferentially to pre-rRNA within the 5'-ETS,Citation77 it was proposed that Esf2 not only stimulates the enzymatic activity, but could in addition recruit Dbp8 to its substrate pre-rRNAs.Citation78
Several RNA helicases are required for the release of snoRNPs from pre-ribosomes (see ref. Citation36 and references therein). Density gradient centrifugation of cellular material and subsequent analysis of snoRNA association with pre-ribosomal particles by northern blotting or quantitative RT-PCR identified snoRNAs that accumulate on pre-ribosomes when specific RNA helicases are depleted. Systematic analysis of RNA helicases involved in SSU biogenesis showed that especially the essential snoRNAs require RNA helicases for their release from pre-ribosomal particles.Citation79 Depletion of the helicase Rok1 leads to pre-ribosomal accumulation of the essential snoRNA snR30, suggesting a role of Rok1 in the release of the snR30-containing snoRNP.Citation79 Rok1 also genetically interacts with snR10 and Rrp5 and was reported to associate with the SSU processome in a Rrp5 dependent manner.Citation80-Citation83 Interestingly, Rok1 was also identified as a high copy suppressor of Xrn1/Kem1 and to rescue the phenotype of Xrn1 deletions on pheromone induced nuclear fusion.Citation84 The cell cycle-dependent expression of Rok1 is regulated by an upstream open reading frame in the Rok1 5'-UTR.Citation85
The RNA helicase Dbp4 was shown to be required for release of U14 and snR41 snoRNAs from pre-ribosomes.Citation47 This finding is in line with data that show suppression of U14 mutations in the Y-domain upon Dpb4 overexpression.Citation49 Interestingly, it was further shown that depletion of Has1 causes retention of U3, U14 and some additional modification guiding snoRNAs on pre-ribosomal subunits.Citation86 Quantitative RT-PCR analysis of snoRNA association to pre-ribosomal particles after Dbp4 or Has1 depletion, however, only confirmed an implication of both RNA helicases in association of U14 snoRNA.Citation79 Has1 was further suggested to play a role in U6 snRNP biogenesis, as depletion of Has1 leads to the reduction of cellular U6 snRNA levels.Citation86
One of the best studied RNA helicases acting in ribosome synthesis is the DEAH-box protein Prp43. It was originally identified as a helicase involved in spliceosome disassembly and intron lariat release.Citation87 Later on, its involvement in the biogenesis of both ribosomal subunits was shown,Citation60-Citation62 but the molecular functions remained elusive. Recently, the application of the UV crosslinking and analysis of cDNA (CRAC) approach (see refs. Citation88 and Citation89) identified a number of putative interaction sites of Prp43 on pre-rRNAs and other RNA species.Citation90 Prp43 was found to crosslink to sites both in the SSU and in the LSU. Interestingly, Prp43 is required for the release of a subset of box-C/D snoRNAs (snR39, snR39b, snR50, snR59, snR60 and snR72) from pre-ribosomes, which guide the modification of the pre-rRNA at a cluster of methylation sites in helices 32 to 35 of the 25S rRNA sequence. Moreover, Prp43 crosslinks to snoRNAs guiding methylation in this cluster and to their basepairing sites in the pre-rRNA, suggesting a direct involvement of the RNA helicase in snoRNA unwinding and supporting the proposed role of Prp43 in snoRNA recycling.Citation62,Citation90 In addition, depletion of Prp43 not only leads to accumulation of snoRNAs, but the protein also supports association of the snoRNAs snR64 and snR67 with pre-ribosomal intermediates. These findings are in line with a previously observed defect in methylation of the 25S rRNA guided by snR64 in the Prp43-Q423N mutant background (see ref. Citation62) and could suggest a role for Prp43 in rearranging secondary structures in the pre-rRNA to allow snoRNA basepairing and recruitment of the snoRNPs.
The major interaction site of Prp43 in the SSU locates to helix 44, close to the 3'-end of the 18S rRNA.Citation90 Interestingly, genetic analysis linked Prp43 to the endonuclease Nob1, which mediates 3'-end formation of 18S in the cytoplasm, and is conserved in eukaryotes and archaea (see refs. Citation91–Citation94 and references therein). Here, Prp43 has been suggested to remodel late pre-ribosomal particles in order to allow Nob1 cleavage, but both the Prp43 target site and the mechanism are controversially discussed and still remain to be elucidated.Citation91,Citation92,Citation94 The functions of Prp43 in ribosome synthesis thus not only involves its role in the release of snoRNPs, but probably also structural remodeling of pre-ribosomal particles to allow access of other snoRNPs and to facilitate pre-rRNA processing.
Another interesting aspect of Prp43 function is its interaction with various cofactors, mainly glycine-rich domain (G-patch) containing proteins. These proteins are thought to recruit Prp43 to its target RNPs and to regulate the activity of this versatile RNA helicase. In splicing, G-patch proteins were found to interact with Prp43 and another DExD/H-box protein, Prp2.Citation95,Citation96 Prp43 is recruited to spliceosomes by the G-patch protein Spp382/Ntr1 and the cofactor Ntr2, and its helicase activity can be regulated by interaction with Spp382.Citation97-Citation99 Ribosome synthesis involves the two G-patch proteins Sqs1/Pfa1 and Pxr1/Gno1 and both were shown to interact with Prp43.Citation61,Citation100,Citation101 Sqs1 and Pxr1 are already recruited to 90S pre-ribosomes, and Pfa1 was found in 40S as well as 60S precursors, indicating that this protein assists Prp43 in the maturation of both subunits. Sqs1 and Prp43 were also linked to Nob1 and the putative export adaptor Ltv1 for 20S to 18S processing and the final steps in SSU biogenesis.Citation92,Citation101 In vitro binding and yeast two-hybrid analyses have shown that Sqs1 interacts with Prp43 via several domains and that it can stimulate the ATPase and helicase activity of Prp43.Citation101 Prp43 has recently been structurally analyzed, and its C-terminal oligonucleotide/oligosaccharide-binding domain was found to locate close to the RNA binding cavity and to interact with Sqs1.Citation102,Citation103 Different G-patch proteins also seem to interact and regulate each other. Sqs1 was reported to interact with Spp382 in two-hybrid assays and lack of Pxr1 leads to a reduction in the levels of Sqs1.Citation101,Citation104 Even though the regulatory network of interacting cofactors for Prp43 is not fully understood, it is tempting to speculate that Prp43 requires a number of cofactors to be able to act in such diverse functions as RNP disassembly after splicing, snoRNP release from pre-ribosomes and most likely the remodeling of pre-ribosomal particles. The cofactors seem to recruit Prp43 to its sites of action and probably regulate its catalytic activity and timing.
Besides Prp43, the RNA helicase Dbp2 has also been implicated in ribosome synthesis and mRNA metabolism.Citation43,Citation105 Protein interaction analyses assigned Dbp2 as member of a protein complex involved in nonsense-mediated mRNA decay (NMD). Dbp2 was shown to directly interact with the RNA helicase Upf1 and suggested to act in the dissociation of the ribosome termination complex and thereby to facilitate decapping of mRNAs, besides a possible role in transcription regulation.Citation43,Citation106
As indicated before, the deletion of the DEAD-box protein Dbp3 leads to unusual rRNA processing defects, including the accumulation of the 27SA2 pre-rRNA.Citation54 The defects observed resemble those of RNase MRP inactivation, which could indicate that Dbp3 facilitates RNase MRP mediated pre-rRNA cleavage at site A3 in ITS1.Citation54 Dbp3 was therefore suggested to modulate the secondary structure of a stable stem-loop near the A3 processing site and to thereby allow the effective recruitment and function of the RNase MRP complex.Citation107
Current knowledge on the three DEAD-box helicases Dbp6, Dbp7 and Dbp9 supports a model of interaction and partial cooperation of these RNA helicases in early steps of LSU biogenesis. Dbp6 is part of a 550 to 600 kDa subcomplex containing the ribosome synthesis cofactors Urb1/Npa1, Urb2/Npa2, Nop8 and Rsa3, which can associate independently of RNA.Citation108-Citation111 Apart from these proteins, Dbp6 genetically interacts with Rsa1 and Urb2 and it is linked to Rpl3 and the RNA helicases Dbp7 and Dbp9.Citation52,Citation108,Citation112 Dbp6 and Dbp9 seem to be partially redundant, since Dbp9 can rescue several mutations in Dbp6.Citation113 Based on genetic interactions of Rpl3 with all components of the Dbp6-containing subcomplex as well as Dbp7 and Dbp9, the subcomplex was suggested to contribute to incorporation of Rpl3 into 60S intermediates.Citation108 As both Dbp6 and Dbp9 are essential, the two proteins can be expected to have distinct functions, which still remain to be elucidated.
Two of the helicases that were linked to ribosome synthesis, Sen1 and Mtr4, do not belong to the helicase superfamily 2. Sen1, an Upf1-like superfamily 1 helicase, is most likely not directly involved in the pathway, but affects ribosome synthesis by its roles in transcription termination and snoRNA biogenesis.Citation114-Citation117 The RNA helicase Mtr4/Dob1 occurs free or as part of the TRAMP complex, which can add short 3'-oligoA tails to RNA and thereby target it for processing or degradation by the exosome.Citation118,Citation119 Besides its function in unwinding of secondary structures in substrate RNAs, Mtr4 was also shown to regulate polyadenylation of substrates and even 3'-oligoA tail length.Citation120-Citation122 Mtr4 and the TRAMP complex are required for multiple pathways in RNA/RNP biogenesis and turnover together with the exosome and in some cases other cofactors (see refs. Citation123–Citation125). In ribosome biogenesis, the Mtr4 and the TRAMP complex are involved in nuclear steps of the 3'-end formation pathway of 5.8S rRNA as well as the degradation of aberrant intermediates and the 5'-A0 spacer sequence.Citation126,Citation127
RNA Helicases in Human Ribosome Biogenesis
As in yeast, ribosome biogenesis in vertebrates is largely characterized by the sequential processing of the rRNA.Citation128 The genes coding for rRNA are present in multiple copies and in tandem arrays at the rDNA loci in the human genome.Citation129 Ribosome biogenesis is initiated by RNA Pol I mediated transcription of the 47S rRNA precursor from rDNA loci on the five acrocentric chromosomes in nucleoli, while the precursor of 5S rRNA is transcribed by Pol III in the nucleoplasm from a different genomic locus on chromosome 1. As described for yeast, the large primary transcript (47S pre-rRNA) contains 5'- and 3'-ETS sequences as well as ITS1 and ITS2 in addition to the sequences of the mature 28S, 18S and 5.8S rRNAs. The ITS and ETS sequences are removed during ribosome maturation by sequential nucleolytic cleavage steps ().Citation128 The nascent 47S pre-rRNA associates with a subset of ribosomal proteins, small nucleolar ribonucleoprotein particles (snoRNPs) and trans-acting factors to form a 90S pre-ribosomal particle. It is rapidly converted into the 45S pre-rRNA by cleavages at sites 01 and 02 that remove the 3'-ETS as well as part of the 5'-ETS. Further processing of the 45S pre-rRNA proceeds via alternative pathways generating different rRNA intermediates. Common to both pathways is the separation of the pre-40S particle from the pre-60S particle by cleavage at site 2 located in ITS-1.Citation128 The pre-rRNA component of the small ribosomal subunit is converted into the 18SE intermediate that gives rise to mature 18S rRNA after export from the nucleus into the cytoplasm. The 32S pre-rRNA species of the large ribosomal subunit is processed into the mature 28S and 5.8S rRNA (), where 5.8S is formed via 12S and 7S rRNA intermediates. Incorporation of the 5S rRNA into the 60S particle occurs in the nucleus.
Figure 3. Processing of rRNA in human cells. The schematic representation shows the rRNA processing pathway in human cells. Processing sites are marked by vertical arrows. The nuclear envelope is represented by horizontal bars, and nucleus (Nu) and cytoplasm (Cy) are indicated. Modified from ref. Citation128.
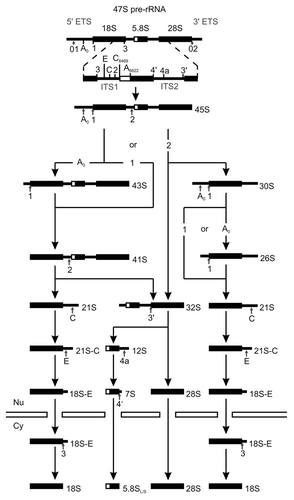
Several RNA helicases have been linked to ribosome biogenesis in vertebrates, but only few have been characterized in more detail so far. Since the ribosome biogenesis pathway per se is expected to be highly conserved among eukaryotes, various mammalian RNA helicases have been determined as homologs of yeast helicases and are generally expected to fulfill similar functions.Citation130 This has been experimentally supported in some cases, such as DDX10 (homolog of Dbp4 in yeast), DDX24 (Mak5), DDX47 (Rrp3), DHX37 (Dhr1) and SK2L2 (Mtr4) (see ).Citation131-Citation134 The concept has to be taken with a grain of salt, however, as in other cases localization or knockdown experiments with human proteins could not confirm the yeast function for the potential human homologs (see for example refs. Citation131 and Citation135). Conversely, lack of evidence using a single assay certainly does not prove that a protein is not involved in the pathway. In addition, several yeast RNA helicases have more than one homolog in mammals, and there are also mammal-specific RNA helicases implicated in ribosome biogenesis that have no counterpart in yeast.Citation130,Citation136
Table 2. RNA helicases implicated in ribosome biogenesis in human cells
Proteomic analysis identified more than 30 putative RNA helicases in the human nucleolus.Citation137-Citation139 Among a multitude of other proteins, 23 putative RNA helicases were recently screened for involvement in ribosome biogenesis by RNA interference (RNAi) in stable cell lines expressing GFP-tagged ribosomal proteins or a ribosome biogenesis cofactor and using localization of the reporters as readout.Citation131 Seven helicases were found to have an impact on the biogenesis of the SSU (DDX10, DDX18, DHX15, DHX37, eIF4A3) or the LSU (DDX24, DDX56). A lentiviral RNAi screen with 28 putative DEAD/H-box RNA helicases known to localize to the nucleolus or nucleus and whose yeast homologs have been implicated in ribosome biogenesis, Zhang and colleagues (2011) addressed protein involvement in Pol I-mediated transcription of the 47S rRNA precursor.Citation135 They observed an almost 10-fold decrease of the pre-rRNA transcript after knockdown of DHX9 and DHX33, while the 47S rRNA level was reduced by more than 50% after knockdown of 12 other candidate proteins, namely DDX3X, DDX10, DHX16, DDX17, DDX18, DDX23, DDX46, DDX47, DDX48, DDX50, DDX51 and DDX56. Further functional and mechanistic analysis of DHX33 revealed that it localizes to rDNA loci and regulates RNA Pol I recruitment by interacting with the Upstream Binding Factor (UBF), a well-known rDNA chromatin remodeling protein.Citation135 Since both the NTPase and DNA binding activities of DHX33 were necessary to regulate 47S rRNA transcription, DHX33 might assist in conformational changes of rDNA loci through ATP hydrolysis. Based on the observation that knockdown of DHX33 did not result in accumulation of any downstream rRNA precursor or a change in the ratios of rRNA species, it was proposed that DHX33 is not involved in pre-rRNA processing per se but might rather act as a DNA helicase in transcription.Citation135 However, to learn more about the substrate specificity and enzymatic activity of DHX33, further in vitro characterization of the protein will be necessary.
The involvement of DHX9, also known as nuclear DNA helicase II (NDH II) and RNA helicase A (RHA), in rRNA transcription is also suggested by studies of Zhang and colleagues which found that DHX9 localizes to the nucleolus and that nucleolar localization depends on active rRNA transcription.Citation140,Citation141 Since DHX9 can unwind RNA-RNA, DNA-DNA and DNA-RNA duplexes in vitro as well as more complex DNA-RNA hybrids containing forks, displacement loops (D- and R-loops) and also G-quadruplexes that can form during transcription (see ref. Citation142), further studies are necessary to clarify by which mechanism DHX9 regulates rRNA transcription and whether this requires RNA or DNA helicase activity. However, DHX9 contains an intrinsic transcriptional activation domain,Citation143 which could also permit functions independent of helicase activity.Citation144 In addition, DHX9 has been proposed to act in the translation of specific mRNAs.Citation145
While no yeast homologs were identified for DHX9 and DHX33, the human DEAD-box helicase eIF4AIII (DDX48) is homologous to the yeast protein Fal1, which is essential and required for 18S rRNA biogenesis in S. cerevisiae. Although eIF4AIII is well described as a component of the exon junction complex (see for example refs. Citation146 and Citation147), expression of human eIF4AIII rescued the lethal phenotype and the 18S rRNA biogenesis defect of fal1 deletion in yeast.Citation148 Mutations in the Walker A and Walker B motifs of the human protein prevented complementation of the fal1 deletion, indicating that the ATPase activity is essential. NOM1 was identified as an eIF4G-like interaction partner of human eIF4AIII, while its yeast homolog Sgd1 interacts with Fal1.Citation148 In human cells, siRNA-induced knockdown of eIF4AIII decreased the levels of the 41S and 21S pre-rRNAs as well as the mature 18S rRNA, and lead to an increase in the ratio of 45S pre-rRNA to 30S/32S pre-rRNA, indicating that eIF4AIII participates in ribosome biogenesis.Citation148 A function of eIF4AIII in SSU biogenesis is further supported by reporter assays, in which knockdown of eIF4AIII affected RPS2-YFP and ENP1 localization.Citation131
DDX21, also named RNA helicase II or Gu(α) protein, was originally cloned from HeLa cells and has been linked to watermelon stomach disease.Citation149,Citation150 DDX21 and its Xenopus homologs xGu-1 and xGu-2 were shown to be required for ribosome synthesis in Xenopus oocytes and human cells, suggesting its conservation in vertebrates.Citation151,Citation152 The protein localizes to nucleoli in both Xenopus and mammalian cells. Antisense oligonucleotide mediated knockdown of xGu-1/2 in Xenopus oocytes resulted in decreased 18S and increased 20S levels as well as reduced amounts of 28S rRNA. In addition, faster migrating RNA species, other than 18S rRNA appeared in the absence of xGu-1/2 and were confirmed to be degradation products of 28S rRNA, suggesting that the reduction in 28S rRNA levels was due to reduced stability.Citation151 Although microinjection of wildtype xGu-1 mRNA into oocytes rescued the antisense effect, a mutant deficient in RNA unwinding due to a mutation in the DEVD motif did not, indicating that helicase activity is required for efficient production of 18S and 28S rRNA. In human cells, knockdown of DDX21 reduced 18S and 28S rRNA levels accompanied by a minor decrease in the 32S rRNA precursor.Citation152 In contrast to the Xenopus study, neither an accumulation of 20S intermediates nor the degradation of 28S rRNA was observed in mammalian cells. Surprisingly, a helicase-deficient mutant of human DDX21 (SAT mutant) rescued 28S rRNA production, but did not restore 18S rRNA levels, pointing to an involvement of DDX21 RNA unwinding activity in 18S but not in 28S rRNA biosynthesis in mammalian cells.Citation152 However, a DDX21 DEVD mutant could neither restore 18S nor 28S rRNA levels.Citation153 Interestingly, the overexpression of the DDX21 paralog DDX50 (RHII/Guβ) inhibited rRNA maturation, even in the wildtype background with normal levels of DDX21,Citation152 suggesting antagonistic roles of the two paralogues. DDX50 was also shown to colocalize with the splicing factor SC35 in nuclear speckles.Citation154
Several interaction partners of DDX21 have been identified and suggested to recruit DDX21 and regulate its function in ribosome biogenesis. DDX21 was shown to directly interact with RPL4.Citation153 Another report identified DDX21 as a component of a > 700 kD protein complex containing several ribosomal proteins and the cofactors Pes1, EBP2 and nucleostemin.Citation155 The association with components of the PeBoW complex (see ref. Citation156) is supported by the identification of DDX21 in pulldowns together with parvulin and Bop1.Citation157 The C-terminal tail of DDX21 has been shown to interact with the transcription factor c-Jun that stimulates the intrinsic rRNA binding activity of DDX21 and is required for targeting DDX21 to the nucleolus.Citation158
DDX51 was identified as an interaction partner of the mammalian ribosome synthesis cofactor Nog1 in a yeast two-hybrid screen and shown to colocalize with Nog1 to the nucleolus of a murine fibroblast cell line.Citation159 RNAi against DDX51 resulted in decreased levels of the 32S and 12S rRNA intermediates, while the early 47S/45S precursors accumulated. Further, accumulation of 3'-ETS-extended rRNA precursors indicated an impaired processing at site 6 located downstream of mouse 28S rRNA, which was also observed upon overexpression of a dominant negative helicase mutant. These findings indicate that efficient rRNA processing requires helicase activity. Indeed, inactivation of DDX51 results in pre-ribosomal accumulation of the U8 snoRNA, which is required for 3'-processing of the 28S rRNA (see ref. Citation159 and references therein).
DDX5 (p68) was shown to localize to nuclei and nucleoli and associate with the rDNA promoter in a cell cycle-dependent and p19Arf-regulated manner.Citation160,Citation161 Arf can prevent interaction of DDX5 with nucleophosmin, which is required for nucleolar DDX5 recruitment. DDX5 is closely related to another member of the DEAD box family, DDX17, which is expressed in the two isoforms p72 and p82. The two helicases can form a heterodimer and have been implicated in several pathways of RNA metabolism.Citation162,Citation163 DDX5 negatively regulates expression of DDX17 and both helicases have a redundant function in cell proliferation and viability.Citation164 Detailed functional analysis revealed that co-silencing of both genes disturbed nucleolar structure and impaired processing of the 32S rRNA precursor. Mutant mice lacking either of the helicases showed reduced levels in mature 5.8S rRNA.Citation162 Further studies indicated that the redundant roles of DDX5 and DDX17 were based on the catalysis of RNA rearrangements. Interestingly, U8 snoRNA slightly accumulated upon co-silencing of DDX5 and DDX17 in the 60S/90S pre-ribosomal fractions.Citation164 It remains to be shown, whether this is due to involvement of the helicases in snoRNA release, or an indirect effect due to a lack of structural remodeling in early pre-ribosomal complexes.
DDX56, previously named nucleolar helicase of 61 kDa (NOH61), is considered as the homolog of yeast Dbp9.Citation136 DDX56 was shown to possess ATPase activity in vitro, to localize to the nucleolus and to associate with pre-60S particles.Citation165 Its involvement in LSU biogenesis is further supported by nuclear accumulation of RPL29-GFP upon knockdown of DDX56.Citation131
Similarly, the yeast homolog of DHX15, Prp43, acts both in pre-mRNA splicing and ribosome biogenesis (see above), while human DHX15 was only found to localize to splicing factor containing nuclear speckles and not to nucleoli.Citation166 Dhx15 knockdown was observed in the fluorescent reporter screen to affect RPS2-YFP localization, indicating a role in SSU biogenesis,Citation131 but no direct evidence for a function in ribosome synthesis has been published so far.
DDX10, DDX18 and DHX37 were found in the human SSU processome.Citation133 Both DDX10 and DDX18 were also picked up in the reporter screen using RPS2-YFP to study SSU maturation.Citation131 DDX10 was found together with nucleolin, RRP5 and the U3 snoRNP in a 50S particle, which accumulated in response to inhibition of Pol I transcription by actinomycin D treatment or upon depletion of tUTP proteins.Citation133 Based on these results, the authors proposed that the 50S U3 snoRNP represents a SSU processome assembly intermediate and that its incorporation into the processing complex requires the presence of pre-rRNA and the tUTP proteins. However, the detailed functional characterization of human DDX10 and the functional comparison to its yeast homolog Dbp4 will be interesting topics of future research.
Outlook
Since DExD/H-box RNA helicases play key roles in all major pathways of RNA metabolism, it is not surprising that a number of these proteins are involved in ribosome synthesis. While basic concepts of helicase function apply to ribosome synthesis in all organisms, the current state of knowledge and the major questions differ significantly between model organisms. In bacteria, the players are known and the timing of their action as well as ribosomal proteins that depend on helicases for their incorporation into nascent ribosomal subunits have been analyzed.Citation1 Some of the proteins, for example DbpA/YxiN, have been studied extensively in vitro, including kinetics, RNA binding and structural rearrangements during the ATPase cycle.
In yeast, the best understood eukaryotic model system, the molecular function of the majority of proteins involved in ribosome biogenesis has remained elusive so far and the question remains, why so many putative RNA helicases are required for this pathway. As one possible answer to this question, it was suggested that snoRNAs in general might require RNA helicases for their unwinding and release from pre-rRNA.Citation30 Stable basepairing of snoRNAs with pre-rRNA has been further supported by the recent identification of additional basepairing sequences,Citation40 and by the fact that snoRNA-guided modifications cluster in certain functional regions of the rRNA leading to overlapping basepairing sites. Indeed, several RNA helicases are required for the release of specific snoRNPs from pre-ribosomes (reviewed in ref. Citation36), even though they have not been formally shown to unwind snoRNA-pre-rRNA basepairing. The model of helicase-mediated unwinding is supported by recent evidence for Prp43, where the DEAH-box protein is required for release of a number of snoRNPs that modify a cluster of sites in the 25S rRNA. Here, Prp43 was found to crosslink to snoRNAs and to their basepairing sites on the pre-rRNA, suggesting direct contact and involvement of the RNA helicase in snoRNA unwinding.Citation90
Despite the extended basepairing, most snoRNPs do not seem to rely on (individual) helicases for their release, as was systematically investigated for SSU biogenesis.Citation79 It is well possible that several helicases are recruited to the same part of the pre-ribosome and will turn out to be partially redundant, if they act in release of overlapping sets of snoRNAs. It needs to be kept in mind, however, that most of the helicases implicated in ribosome synthesis in yeast are essential, suggesting at least one unique cellular function for each of these proteins. Also, only few RNA helicases have been implicated in other cellular pathways in addition to ribosome biogenesis so far, but more such cases can be expected.
Many pre-rRNA helicases are functionally not as well understood as some other RNA helicases, such as proteins involved in splicing. This is probably due to the high complexity and dynamics of the pathway and the relative inaccessibility of the nucleolus. A major aim in the next few years will be the identification of recruitment and target sites of RNA helicases within pre-ribosomal complexes, and in RNPs in general. UV crosslinking in combination with deep sequencing has turned out to be a powerful tool to identify interaction sites of RNA binding proteins (see for example refs. Citation88 and Citation167). This will, on the one hand, allow the use of substrate RNAs in in vitro studies, which have so far been done using model substrates (for example see refs. Citation50, Citation168–Citation170). The kinetics might turn out to be different when the authentic substrate RNAs are used in these assays. On the other hand, the identification of interaction sites will allow a more detailed functional analysis of RNA helicases, including their regulation, the roles of cofactors and their molecular function. These studies are supported by the recent structures of the eukaryotic ribosome (see for example refs. Citation171 and Citation172), which allows the mapping of putative interaction sites in 3D. Structural analysis of pre-ribosomal complexes (for example see ref. Citation173), however, will be required to better understand the context in which RNA helicases act in pre-ribosomes that might be structurally different than mature ribosomal subunits.
Ribosome biogenesis is much less understood in multicellular eukaryotes. Only two RNA helicases have been shown to be involved in ribosome synthesis in plants,Citation174,Citation175 with many more to be identified. In general, the genes of most yeast helicases are conserved in many eukaryotes and the corresponding proteins thus expected to fulfill similar if not equivalent functions. As detailed above, further helicases add to the complexity of the pathway in mammalian cells, and it can be expected that more proteins involved in ribosome synthesis will be found. For most of the proteins identified so far, their binding sites on pre-ribosomes, molecular functions and regulation also remain to be analyzed. The regulation of RNA helicases is a very interesting topic that has started to be addressed in recent years. Remarkably, it was observed that some helicases seem to bind early in a pathway but only to be specifically activated later on, probably by protein cofactors (e.g., Prp43). A further level of regulation is helicase expression, which is often deregulated in cancers. In addition, it will be interesting to see how helicase activity is modulated by signaling networks and how these are cross-regulated in case of helicase function in several pathways of RNA metabolism.
Abbreviations: | ||
ATP | = | adenosine triphosphate |
cDNA | = | complementary DNA |
CRAC | = | UV crosslinking and analysis of cDNA |
E. coli | = | Escherichia coli |
ETS | = | external transcribed spacer |
G-patch | = | glycine-rich domain |
ITS | = | internal transcribed spacer |
kDa | = | kilodalton |
LSU | = | large subunit |
MDa | = | megadalton |
mRNA | = | messenger RNA |
NMD | = | nonsense-mediated mRNA decay |
NTP | = | nucleoside triphosphate |
Pol | = | polymerase |
rDNA | = | ribosomal DNA |
RNAi | = | RNA interference |
RNase | = | ribonuclease |
RNP | = | ribonucleoprotein particle |
RP | = | ribosomal protein |
rRNA | = | ribosomal RNA |
RT-PCR | = | real-time polymerase chain reaction |
siRNA | = | small interfering RNA |
snoRNA | = | small nucleolar RNA |
snoRNP | = | small nucleolar ribonucleoprotein particle |
snRNA | = | small nuclear RNA |
snRNP | = | small nuclear ribonucleoprotein particle |
SSU | = | small subunit |
Acknowledgments
We thank Nicholas Watkins, Sara Müller and Matthias Leisegang for critical comments on the manuscript and the Deutsche Forschungsgemeinschaft (BO 3442/1–1 and SFB860) as well as the Göttingen University Medical Department for funding. We would like to apologize to all colleagues whose work could not be cited due to limitations in space and the number of references.
References
- Shajani Z, Sykes MT, Williamson JR. Assembly of bacterial ribosomes. Annu Rev Biochem 2011; 80:501 - 26; http://dx.doi.org/10.1146/annurev-biochem-062608-160432; PMID: 21529161
- Lafontaine DL, Tollervey D. The function and synthesis of ribosomes. Nat Rev Mol Cell Biol 2001; 2:514 - 20; http://dx.doi.org/10.1038/35080045; PMID: 11433365
- Henras AK, Soudet J, Gérus M, Lebaron S, Caizergues-Ferrer M, Mougin A, et al. The post-transcriptional steps of eukaryotic ribosome biogenesis. Cell Mol Life Sci 2008; 65:2334 - 59; http://dx.doi.org/10.1007/s00018-008-8027-0; PMID: 18408888
- Nissen P, Hansen J, Ban N, Moore PB, Steitz TA. The structural basis of ribosome activity in peptide bond synthesis. Science 2000; 289:920 - 30; http://dx.doi.org/10.1126/science.289.5481.920; PMID: 10937990
- Bleichert F, Baserga SJ. The long unwinding road of RNA helicases. Mol Cell 2007; 27:339 - 52; http://dx.doi.org/10.1016/j.molcel.2007.07.014; PMID: 17679086
- Linder P, Jankowsky E. From unwinding to clamping - the DEAD box RNA helicase family. Nat Rev Mol Cell Biol 2011; 12:505 - 16; http://dx.doi.org/10.1038/nrm3154; PMID: 21779027
- Cordin O, Banroques J, Tanner NK, Linder P. The DEAD-box protein family of RNA helicases. Gene 2006; 367:17 - 37; http://dx.doi.org/10.1016/j.gene.2005.10.019; PMID: 16337753
- Yang Q, Jankowsky E. The DEAD-box protein Ded1 unwinds RNA duplexes by a mode distinct from translocating helicases. Nat Struct Mol Biol 2006; 13:981 - 6; http://dx.doi.org/10.1038/nsmb1165; PMID: 17072313
- Tijerina P, Bhaskaran H, Russell R. Nonspecific binding to structured RNA and preferential unwinding of an exposed helix by the CYT-19 protein, a DEAD-box RNA chaperone. Proc Natl Acad Sci USA 2006; 103:16698 - 703; http://dx.doi.org/10.1073/pnas.0603127103; PMID: 17075070
- Nierhaus KH, Dohme F. Total reconstitution of functionally active 50S ribosomal subunits from Escherichia coli. Proc Natl Acad Sci USA 1974; 71:4713 - 7; http://dx.doi.org/10.1073/pnas.71.12.4713; PMID: 4612527
- Nomura M, Traub P. Structure and function of Escherichia coli ribosomes. 3. Stoichiometry and rate of the reconstitution of ribosomes from subribosomal particles and split proteins. J Mol Biol 1968; 34:609 - 19; http://dx.doi.org/10.1016/0022-2836(68)90184-8; PMID: 4938560
- Iost I, Dreyfus M. DEAD-box RNA helicases in Escherichia coli. Nucleic Acids Res 2006; 34:4189 - 97; http://dx.doi.org/10.1093/nar/gkl500; PMID: 16935881
- Nishi K, Morel-Deville F, Hershey JW, Leighton T, Schnier J. An eIF-4A-like protein is a suppressor of an Escherichia coli mutant defective in 50S ribosomal subunit assembly. Nature 1988; 336:496 - 8; http://dx.doi.org/10.1038/336496a0; PMID: 2461520
- Trubetskoy D, Proux F, Allemand F, Dreyfus M, Iost I. SrmB, a DEAD-box helicase involved in Escherichia coli ribosome assembly, is specifically targeted to 23S rRNA in vivo. Nucleic Acids Res 2009; 37:6540 - 9; http://dx.doi.org/10.1093/nar/gkp685; PMID: 19734346
- Charollais J, Pflieger D, Vinh J, Dreyfus M, Iost I. The DEAD-box RNA helicase SrmB is involved in the assembly of 50S ribosomal subunits in Escherichia coli. Mol Microbiol 2003; 48:1253 - 65; http://dx.doi.org/10.1046/j.1365-2958.2003.03513.x; PMID: 12787353
- Proux F, Dreyfus M, Iost I. Identification of the sites of action of SrmB, a DEAD-box RNA helicase involved in Escherichia coli ribosome assembly. Mol Microbiol 2011; 82:300 - 11; http://dx.doi.org/10.1111/j.1365-2958.2011.07779.x; PMID: 21859437
- Toone WM, Rudd KE, Friesen JD. deaD, a new Escherichia coli gene encoding a presumed ATP-dependent RNA helicase, can suppress a mutation in rpsB, the gene encoding ribosomal protein S2. J Bacteriol 1991; 173:3291 - 302; PMID: 2045359
- Jones PG, Mitta M, Kim Y, Jiang W, Inouye M. Cold shock induces a major ribosomal-associated protein that unwinds double-stranded RNA in Escherichia coli. Proc Natl Acad Sci USA 1996; 93:76 - 80; http://dx.doi.org/10.1073/pnas.93.1.76; PMID: 8552679
- Charollais J, Dreyfus M, Iost I. CsdA, a cold-shock RNA helicase from Escherichia coli, is involved in the biogenesis of 50S ribosomal subunit. Nucleic Acids Res 2004; 32:2751 - 9; http://dx.doi.org/10.1093/nar/gkh603; PMID: 15148362
- Peil L, Virumäe K, Remme J. Ribosome assembly in Escherichia coli strains lacking the RNA helicase DeaD/CsdA or DbpA. FEBS J 2008; 275:3772 - 82; http://dx.doi.org/10.1111/j.1742-4658.2008.06523.x; PMID: 18565105
- Awano N, Xu C, Ke H, Inoue K, Inouye M, Phadtare S. Complementation analysis of the cold-sensitive phenotype of the Escherichia coli csdA deletion strain. J Bacteriol 2007; 189:5808 - 15; http://dx.doi.org/10.1128/JB.00655-07; PMID: 17557820
- Jain C. The E. coli RhlE RNA helicase regulates the function of related RNA helicases during ribosome assembly. RNA 2008; 14:381 - 9; http://dx.doi.org/10.1261/rna.800308; PMID: 18083833
- Elles LM, Uhlenbeck OC. Mutation of the arginine finger in the active site of Escherichia coli DbpA abolishes ATPase and helicase activity and confers a dominant slow growth phenotype. Nucleic Acids Res 2008; 36:41 - 50; http://dx.doi.org/10.1093/nar/gkm926; PMID: 17986459
- Sharpe Elles LM, Sykes MT, Williamson JR, Uhlenbeck OC. A dominant negative mutant of the E. coli RNA helicase DbpA blocks assembly of the 50S ribosomal subunit. Nucleic Acids Res 2009; 37:6503 - 14; http://dx.doi.org/10.1093/nar/gkp711; PMID: 19734347
- Kossen K, Uhlenbeck OC. Cloning and biochemical characterization of Bacillus subtilis YxiN, a DEAD protein specifically activated by 23S rRNA: delineation of a novel sub-family of bacterial DEAD proteins. Nucleic Acids Res 1999; 27:3811 - 20; http://dx.doi.org/10.1093/nar/27.19.3811; PMID: 10481020
- Tsu CA, Uhlenbeck OC. Kinetic analysis of the RNA-dependent adenosinetriphosphatase activity of DbpA, an Escherichia coli DEAD protein specific for 23S ribosomal RNA. Biochemistry 1998; 37:16989 - 96; http://dx.doi.org/10.1021/bi981837y; PMID: 9836593
- Tsu CA, Kossen K, Uhlenbeck OC. The Escherichia coli DEAD protein DbpA recognizes a small RNA hairpin in 23S rRNA. RNA 2001; 7:702 - 9; http://dx.doi.org/10.1017/S1355838201010135; PMID: 11350034
- Asakura Y, Galarneau ER, Watkins KP, Barkan A, van Wijk KJ. Chloroplast RH3 DEAD Box RNA Helicases in Maize and Arabidopsis Function in Splicing of Specific Group II Introns and Affect Chloroplast Ribosome Biogenesis. Plant Physiol 2012; 159:961 - 74; http://dx.doi.org/10.1104/pp.112.197525; PMID: 22576849
- Chi W, He B, Mao J, Li Q, Ma J, Ji D, et al. The function of RH22, a DEAD RNA helicase, in the biogenesis of the 50S ribosomal subunits of Arabidopsis chloroplasts. Plant Physiol 2012; 158:693 - 707; http://dx.doi.org/10.1104/pp.111.186775; PMID: 22170977
- Venema J, Tollervey D. Ribosome synthesis in Saccharomyces cerevisiae. Annu Rev Genet 1999; 33:261 - 311; http://dx.doi.org/10.1146/annurev.genet.33.1.261; PMID: 10690410
- Fromont-Racine M, Senger B, Saveanu C, Fasiolo F. Ribosome assembly in eukaryotes. Gene 2003; 313:17 - 42; http://dx.doi.org/10.1016/S0378-1119(03)00629-2; PMID: 12957375
- Kressler D, Hurt E, Bassler J. Driving ribosome assembly. Biochim Biophys Acta 2010; 1803:673 - 83; http://dx.doi.org/10.1016/j.bbamcr.2009.10.009; PMID: 19879902
- Warner JR. The economics of ribosome biosynthesis in yeast. Trends Biochem Sci 1999; 24:437 - 40; http://dx.doi.org/10.1016/S0968-0004(99)01460-7; PMID: 10542411
- Kos M, Tollervey D. Yeast pre-rRNA processing and modification occur cotranscriptionally. Mol Cell 2010; 37:809 - 20; http://dx.doi.org/10.1016/j.molcel.2010.02.024; PMID: 20347423
- Osheim YN, French SL, Keck KM, Champion EA, Spasov K, Dragon F, et al. Pre-18S ribosomal RNA is structurally compacted into the SSU processome prior to being cleaved from nascent transcripts in Saccharomyces cerevisiae. Mol Cell 2004; 16:943 - 54; http://dx.doi.org/10.1016/j.molcel.2004.11.031; PMID: 15610737
- Watkins NJ, Bohnsack MT. The box C/D and H/ACA snoRNPs: key players in the modification, processing and the dynamic folding of ribosomal RNA. Wiley Interdiscip Rev RNA 2012; 3:397 - 414; http://dx.doi.org/10.1002/wrna.117; PMID: 22065625
- Kiss T. Small nucleolar RNAs: an abundant group of noncoding RNAs with diverse cellular functions. Cell 2002; 109:145 - 8; http://dx.doi.org/10.1016/S0092-8674(02)00718-3; PMID: 12007400
- Decatur WA, Fournier MJ. RNA-guided nucleotide modification of ribosomal and other RNAs. J Biol Chem 2003; 278:695 - 8; http://dx.doi.org/10.1074/jbc.R200023200; PMID: 12431975
- Ripmaster TL, Vaughn GP, Woolford JL Jr.. A putative ATP-dependent RNA helicase involved in Saccharomyces cerevisiae ribosome assembly. Proc Natl Acad Sci USA 1992; 89:11131 - 5; http://dx.doi.org/10.1073/pnas.89.23.11131; PMID: 1454790
- van Nues RW, Granneman S, Kudla G, Sloan KE, Chicken M, Tollervey D, et al. Box C/D snoRNP catalysed methylation is aided by additional pre-rRNA base-pairing. EMBO J 2011; 30:2420 - 30; http://dx.doi.org/10.1038/emboj.2011.148; PMID: 21556049
- Ripmaster TL, Vaughn GP, Woolford JL Jr.. DRS1 to DRS7, novel genes required for ribosome assembly and function in Saccharomyces cerevisiae. Mol Cell Biol 1993; 13:7901 - 12; PMID: 8247005
- Barta I, Iggo R. Autoregulation of expression of the yeast Dbp2p ‘DEAD-box’ protein is mediated by sequences in the conserved DBP2 intron. EMBO J 1995; 14:3800 - 8; PMID: 7641698
- Bond AT, Mangus DA, He F, Jacobson A. Absence of Dbp2p alters both nonsense-mediated mRNA decay and rRNA processing. Mol Cell Biol 2001; 21:7366 - 79; http://dx.doi.org/10.1128/MCB.21.21.7366-7379.2001; PMID: 11585918
- Cherry JM, Hong EL, Amundsen C, Balakrishnan R, Binkley G, Chan ET, et al. Saccharomyces Genome Database: the genomics resource of budding yeast. Nucleic Acids Res 2012; 40:Database issue D700 - 5; http://dx.doi.org/10.1093/nar/gkr1029; PMID: 22110037
- Granneman S, Bernstein KA, Bleichert F, Baserga SJ. Comprehensive mutational analysis of yeast DEXD/H box RNA helicases required for small ribosomal subunit synthesis. Mol Cell Biol 2006; 26:1183 - 94; http://dx.doi.org/10.1128/MCB.26.4.1183-1194.2006; PMID: 16449634
- Colley A, Beggs JD, Tollervey D, Lafontaine DL. Dhr1p, a putative DEAH-box RNA helicase, is associated with the box C+D snoRNP U3. Mol Cell Biol 2000; 20:7238 - 46; http://dx.doi.org/10.1128/MCB.20.19.7238-7246.2000; PMID: 10982841
- Kos M, Tollervey D. The Putative RNA Helicase Dbp4p Is Required for Release of the U14 snoRNA from Preribosomes in Saccharomyces cerevisiae. Mol Cell 2005; 20:53 - 64; http://dx.doi.org/10.1016/j.molcel.2005.08.022; PMID: 16209945
- Kressler D, de la Cruz J, Rojo M, Linder P. Fal1p is an essential DEAD-box protein involved in 40S-ribosomal-subunit biogenesis in Saccharomyces cerevisiae. Mol Cell Biol 1997; 17:7283 - 94; PMID: 9372960
- Liang WQ, Clark JA, Fournier MJ. The rRNA-processing function of the yeast U14 small nucleolar RNA can be rescued by a conserved RNA helicase-like protein. Mol Cell Biol 1997; 17:4124 - 32; PMID: 9199348
- O’Day CL, Chavanikamannil F, Abelson J. 18S rRNA processing requires the RNA helicase-like protein Rrp3. Nucleic Acids Res 1996; 24:3201 - 7; http://dx.doi.org/10.1093/nar/24.16.3201; PMID: 8774901
- Bernstein KA, Granneman S, Lee AV, Manickam S, Baserga SJ. Comprehensive mutational analysis of yeast DEXD/H box RNA helicases involved in large ribosomal subunit biogenesis. Mol Cell Biol 2006; 26:1195 - 208; http://dx.doi.org/10.1128/MCB.26.4.1195-1208.2006; PMID: 16449635
- Daugeron MC, Linder P. Dbp7p, a putative ATP-dependent RNA helicase from Saccharomyces cerevisiae, is required for 60S ribosomal subunit assembly. RNA 1998; 4:566 - 81; http://dx.doi.org/10.1017/S1355838298980190; PMID: 9582098
- Kressler D, de la Cruz J, Rojo M, Linder P. Dbp6p is an essential putative ATP-dependent RNA helicase required for 60S-ribosomal-subunit assembly in Saccharomyces cerevisiae. Mol Cell Biol 1998; 18:1855 - 65; PMID: 9528757
- Weaver PL, Sun C, Chang TH. Dbp3p, a putative RNA helicase in Saccharomyces cerevisiae, is required for efficient pre-rRNA processing predominantly at site A3. Mol Cell Biol 1997; 17:1354 - 65; PMID: 9032262
- Zagulski M, Kressler D, Bécam AM, Rytka J, Herbert CJ. Mak5p, which is required for the maintenance of the M1 dsRNA virus, is encoded by the yeast ORF YBR142w and is involved in the biogenesis of the 60S subunit of the ribosome. Mol Genet Genomics 2003; 270:216 - 24; http://dx.doi.org/10.1007/s00438-003-0913-4; PMID: 13680366
- Sachs AB, Davis RW. Translation initiation and ribosomal biogenesis: involvement of a putative rRNA helicase and RPL46. Science 1990; 247:1077 - 9; http://dx.doi.org/10.1126/science.2408148; PMID: 2408148
- de la Cruz J, Kressler D, Rojo M, Tollervey D, Linder P. Spb4p, an essential putative RNA helicase, is required for a late step in the assembly of 60S ribosomal subunits in Saccharomyces cerevisiae. RNA 1998; 4:1268 - 81; http://dx.doi.org/10.1017/S1355838298981158; PMID: 9769101
- Burger F, Daugeron MC, Linder P. Dbp10p, a putative RNA helicase from Saccharomyces cerevisiae, is required for ribosome biogenesis. Nucleic Acids Res 2000; 28:2315 - 23; http://dx.doi.org/10.1093/nar/28.12.2315; PMID: 10871363
- García-Gómez JJ, Lebaron S, Froment C, Monsarrat B, Henry Y, de la Cruz J. Dynamics of the putative RNA helicase Spb4 during ribosome assembly in Saccharomyces cerevisiae. Mol Cell Biol 2011; 31:4156 - 64; http://dx.doi.org/10.1128/MCB.05436-11; PMID: 21825077
- Combs DJ, Nagel RJ, Ares M Jr., Stevens SW. Prp43p is a DEAH-box spliceosome disassembly factor essential for ribosome biogenesis. Mol Cell Biol 2006; 26:523 - 34; http://dx.doi.org/10.1128/MCB.26.2.523-534.2006; PMID: 16382144
- Lebaron S, Froment C, Fromont-Racine M, Rain JC, Monsarrat B, Caizergues-Ferrer M, et al. The splicing ATPase prp43p is a component of multiple preribosomal particles. Mol Cell Biol 2005; 25:9269 - 82; http://dx.doi.org/10.1128/MCB.25.21.9269-9282.2005; PMID: 16227579
- Leeds NB, Small EC, Hiley SL, Hughes TR, Staley JP. The splicing factor Prp43p, a DEAH box ATPase, functions in ribosome biogenesis. Mol Cell Biol 2006; 26:513 - 22; http://dx.doi.org/10.1128/MCB.26.2.513-522.2006; PMID: 16382143
- Emery B, de la Cruz J, Rocak S, Deloche O, Linder P. Has1p, a member of the DEAD-box family, is required for 40S ribosomal subunit biogenesis in Saccharomyces cerevisiae. Mol Microbiol 2004; 52:141 - 58; http://dx.doi.org/10.1111/j.1365-2958.2003.03973.x; PMID: 15049817
- Krogan NJ, Peng WT, Cagney G, Robinson MD, Haw R, Zhong G, et al. High-definition macromolecular composition of yeast RNA-processing complexes. Mol Cell 2004; 13:225 - 39; http://dx.doi.org/10.1016/S1097-2765(04)00003-6; PMID: 14759368
- Lebreton A, Rousselle JC, Lenormand P, Namane A, Jacquier A, Fromont-Racine M, et al. 60S ribosomal subunit assembly dynamics defined by semi-quantitative mass spectrometry of purified complexes. Nucleic Acids Res 2008; 36:4988 - 99; http://dx.doi.org/10.1093/nar/gkn469; PMID: 18658244
- Nissan TA, Bassler J, Petfalski E, Tollervey D, Hurt E. 60S pre-ribosome formation viewed from assembly in the nucleolus until export to the cytoplasm. EMBO J 2002; 21:5539 - 47; http://dx.doi.org/10.1093/emboj/cdf547; PMID: 12374754
- Dragon F, Gallagher JE, Compagnone-Post PA, Mitchell BM, Porwancher KA, Wehner KA, et al. A large nucleolar U3 ribonucleoprotein required for 18S ribosomal RNA biogenesis. Nature 2002; 417:967 - 70; http://dx.doi.org/10.1038/nature00769; PMID: 12068309
- Phipps KR, Charette JM, Baserga SJ. The small subunit processome in ribosome biogenesis—progress and prospects. Wiley Interdiscip Rev RNA 2011; 2:1 - 21; http://dx.doi.org/10.1002/wrna.57; PMID: 21318072
- Gallagher JE, Dunbar DA, Granneman S, Mitchell BM, Osheim Y, Beyer AL, et al. RNA polymerase I transcription and pre-rRNA processing are linked by specific SSU processome components. Genes Dev 2004; 18:2506 - 17; http://dx.doi.org/10.1101/gad.1226604; PMID: 15489292
- Grandi P, Rybin V, Bassler J, Petfalski E, Strauss D, Marzioch M, et al. 90S pre-ribosomes include the 35S pre-rRNA, the U3 snoRNP, and 40S subunit processing factors but predominantly lack 60S synthesis factors. Mol Cell 2002; 10:105 - 15; http://dx.doi.org/10.1016/S1097-2765(02)00579-8; PMID: 12150911
- Pérez-Fernández J, Román A, De Las Rivas J, Bustelo XR, Dosil M. The 90S preribosome is a multimodular structure that is assembled through a hierarchical mechanism. Mol Cell Biol 2007; 27:5414 - 29; http://dx.doi.org/10.1128/MCB.00380-07; PMID: 17515605
- Rudra D, Mallick J, Zhao Y, Warner JR. Potential interface between ribosomal protein production and pre-rRNA processing. Mol Cell Biol 2007; 27:4815 - 24; http://dx.doi.org/10.1128/MCB.02062-06; PMID: 17452446
- Choque E, Marcellin M, Burlet-Schiltz O, Gadal O, Dez C. The nucleolar protein Nop19p interacts preferentially with Utp25p and Dhr2p and is essential for the production of the 40S ribosomal subunit in Saccharomyces cerevisiae. RNA Biol 2011; 8:1158 - 72; http://dx.doi.org/10.4161/rna.8.6.17699; PMID: 21941128
- Charette JM, Baserga SJ. The DEAD-box RNA helicase-like Utp25 is an SSU processome component. RNA 2010; 16:2156 - 69; http://dx.doi.org/10.1261/rna.2359810; PMID: 20884785
- Goldfeder MB, Oliveira CC. Utp25p, a nucleolar Saccharomyces cerevisiae protein, interacts with U3 snoRNP subunits and affects processing of the 35S pre-rRNA. FEBS J 2010; 277:2838 - 52; http://dx.doi.org/10.1111/j.1742-4658.2010.07701.x; PMID: 20528918
- Daugeron MC, Linder P. Characterization and mutational analysis of yeast Dbp8p, a putative RNA helicase involved in ribosome biogenesis. Nucleic Acids Res 2001; 29:1144 - 55; http://dx.doi.org/10.1093/nar/29.5.1144; PMID: 11222764
- Hoang T, Peng WT, Vanrobays E, Krogan N, Hiley S, Beyer AL, et al. Esf2p, a U3-associated factor required for small-subunit processome assembly and compaction. Mol Cell Biol 2005; 25:5523 - 34; http://dx.doi.org/10.1128/MCB.25.13.5523-5534.2005; PMID: 15964808
- Granneman S, Lin C, Champion EA, Nandineni MR, Zorca C, Baserga SJ. The nucleolar protein Esf2 interacts directly with the DExD/H box RNA helicase, Dbp8, to stimulate ATP hydrolysis. Nucleic Acids Res 2006; 34:3189 - 99; http://dx.doi.org/10.1093/nar/gkl419; PMID: 16772403
- Bohnsack MT, Kos M, Tollervey D. Quantitative analysis of snoRNA association with pre-ribosomes and release of snR30 by Rok1 helicase. EMBO Rep 2008; 9:1230 - 6; http://dx.doi.org/10.1038/embor.2008.184; PMID: 18833290
- Venema J, Bousquet-Antonelli C, Gelugne JP, Caizergues-Ferrer M, Tollervey D. Rok1p is a putative RNA helicase required for rRNA processing. Mol Cell Biol 1997; 17:3398 - 407; PMID: 9154839
- Vos HR, Bax R, Faber AW, Vos JC, Raué HA. U3 snoRNP and Rrp5p associate independently with Saccharomyces cerevisiae 35S pre-rRNA, but Rrp5p is essential for association of Rok1p. Nucleic Acids Res 2004; 32:5827 - 33; http://dx.doi.org/10.1093/nar/gkh904; PMID: 15523097
- Torchet C, Jacq C, Hermann-Le Denmat S. Two mutant forms of the S1/TPR-containing protein Rrp5p affect the 18S rRNA synthesis in Saccharomyces cerevisiae. RNA 1998; 4:1636 - 52; http://dx.doi.org/10.1017/S1355838298981511; PMID: 9848659
- Venema J, Tollervey D. RRP5 is required for formation of both 18S and 5.8S rRNA in yeast. EMBO J 1996; 15:5701 - 14; PMID: 8896463
- Song Y, Kim S, Kim J. ROK1, a high-copy-number plasmid suppressor of kem1, encodes a putative ATP-dependent RNA helicase in Saccharomyces cerevisiae. Gene 1995; 166:151 - 4; http://dx.doi.org/10.1016/0378-1119(96)80010-2; PMID: 8529880
- Jeon S, Kim J. Upstream open reading frames regulate the cell cycle-dependent expression of the RNA helicase Rok1 in Saccharomyces cerevisiae. FEBS Lett 2010; 584:4593 - 8; http://dx.doi.org/10.1016/j.febslet.2010.10.019; PMID: 20969870
- Liang XH, Fournier MJ. The helicase Has1p is required for snoRNA release from pre-rRNA. Mol Cell Biol 2006; 26:7437 - 50; http://dx.doi.org/10.1128/MCB.00664-06; PMID: 16908538
- Arenas JE, Abelson JN. Prp43: An RNA helicase-like factor involved in spliceosome disassembly. Proc Natl Acad Sci USA 1997; 94:11798 - 802; http://dx.doi.org/10.1073/pnas.94.22.11798; PMID: 9342317
- Bohnsack MT, Tollervey D, Granneman S. Identification of RNA helicase target sites by UV cross-linking and analysis of cDNA. Methods Enzymol 2012; 511:275 - 88; PMID: 22713325
- Granneman S, Kudla G, Petfalski E, Tollervey D. Identification of protein binding sites on U3 snoRNA and pre-rRNA by UV cross-linking and high-throughput analysis of cDNAs. Proc Natl Acad Sci USA 2009; 106:9613 - 8; http://dx.doi.org/10.1073/pnas.0901997106; PMID: 19482942
- Bohnsack MT, Martin R, Granneman S, Ruprecht M, Schleiff E, Tollervey D. Prp43 bound at different sites on the pre-rRNA performs distinct functions in ribosome synthesis. Mol Cell 2009; 36:583 - 92; http://dx.doi.org/10.1016/j.molcel.2009.09.039; PMID: 19941819
- Lebaron S, Schneider C, van Nues RW, Swiatkowska A, Walsh D, Böttcher B, et al. Proofreading of pre-40S ribosome maturation by a translation initiation factor and 60S subunits. Nat Struct Mol Biol 2012; 19:744 - 53; http://dx.doi.org/10.1038/nsmb.2308; PMID: 22751017
- Pertschy B, Schneider C, Gnädig M, Schäfer T, Tollervey D, Hurt E. RNA helicase Prp43 and its co-factor Pfa1 promote 20 to 18 S rRNA processing catalyzed by the endonuclease Nob1. J Biol Chem 2009; 284:35079 - 91; http://dx.doi.org/10.1074/jbc.M109.040774; PMID: 19801658
- Veith T, Martin R, Wurm JP, Weis BL, Duchardt-Ferner E, Safferthal C, et al. Structural and functional analysis of the archaeal endonuclease Nob1. Nucleic Acids Res 2012; 40:3259 - 74; http://dx.doi.org/10.1093/nar/gkr1186; PMID: 22156373
- Lamanna AC, Karbstein K. An RNA conformational switch regulates pre-18S rRNA cleavage. J Mol Biol 2011; 405:3 - 17; http://dx.doi.org/10.1016/j.jmb.2010.09.064; PMID: 20934433
- Silverman EJ, Maeda A, Wei J, Smith P, Beggs JD, Lin RJ. Interaction between a G-patch protein and a spliceosomal DEXD/H-box ATPase that is critical for splicing. Mol Cell Biol 2004; 24:10101 - 10; http://dx.doi.org/10.1128/MCB.24.23.10101-10110.2004; PMID: 15542821
- Tsai RT, Fu RH, Yeh FL, Tseng CK, Lin YC, Huang YH, et al. Spliceosome disassembly catalyzed by Prp43 and its associated components Ntr1 and Ntr2. Genes Dev 2005; 19:2991 - 3003; http://dx.doi.org/10.1101/gad.1377405; PMID: 16357217
- Boon KL, Auchynnikava T, Edwalds-Gilbert G, Barrass JD, Droop AP, Dez C, et al. Yeast ntr1/spp382 mediates prp43 function in postspliceosomes. Mol Cell Biol 2006; 26:6016 - 23; http://dx.doi.org/10.1128/MCB.02347-05; PMID: 16880513
- Tsai RT, Tseng CK, Lee PJ, Chen HC, Fu RH, Chang KJ, et al. Dynamic interactions of Ntr1-Ntr2 with Prp43 and with U5 govern the recruitment of Prp43 to mediate spliceosome disassembly. Mol Cell Biol 2007; 27:8027 - 37; http://dx.doi.org/10.1128/MCB.01213-07; PMID: 17893323
- Tanaka N, Aronova A, Schwer B. Ntr1 activates the Prp43 helicase to trigger release of lariat-intron from the spliceosome. Genes Dev 2007; 21:2312 - 25; http://dx.doi.org/10.1101/gad.1580507; PMID: 17875666
- Guglielmi B, Werner M. The yeast homolog of human PinX1 is involved in rRNA and small nucleolar RNA maturation, not in telomere elongation inhibition. J Biol Chem 2002; 277:35712 - 9; http://dx.doi.org/10.1074/jbc.M205526200; PMID: 12107183
- Lebaron S, Papin C, Capeyrou R, Chen YL, Froment C, Monsarrat B, et al. The ATPase and helicase activities of Prp43p are stimulated by the G-patch protein Pfa1p during yeast ribosome biogenesis. EMBO J 2009; 28:3808 - 19; http://dx.doi.org/10.1038/emboj.2009.335; PMID: 19927118
- He Y, Andersen GR, Nielsen KH. Structural basis for the function of DEAH helicases. EMBO Rep 2010; 11:180 - 6; http://dx.doi.org/10.1038/embor.2010.11; PMID: 20168331
- Walbott H, Mouffok S, Capeyrou R, Lebaron S, Humbert O, van Tilbeurgh H, et al. Prp43p contains a processive helicase structural architecture with a specific regulatory domain. EMBO J 2010; 29:2194 - 204; http://dx.doi.org/10.1038/emboj.2010.102; PMID: 20512115
- Pandit S, Paul S, Zhang L, Chen M, Durbin N, Harrison SM, et al. Spp382p interacts with multiple yeast splicing factors, including possible regulators of Prp43 DExD/H-Box protein function. Genetics 2009; 183:195 - 206; http://dx.doi.org/10.1534/genetics.109.106955; PMID: 19581443
- He F, Jacobson A. Identification of a novel component of the nonsense-mediated mRNA decay pathway by use of an interacting protein screen. Genes Dev 1995; 9:437 - 54; http://dx.doi.org/10.1101/gad.9.4.437; PMID: 7883168
- Cloutier SC, Ma WK, Nguyen LT, Tran EJ. The DEAD-box RNA Helicase Dbp2 Connects RNA Quality Control with Repression of Aberrant Transcription. J Biol Chem 2012; 287:26155 - 66; http://dx.doi.org/10.1074/jbc.M112.383075; PMID: 22679025
- Kressler D, Linder P, de La Cruz J. Protein trans-acting factors involved in ribosome biogenesis in Saccharomyces cerevisiae. Mol Cell Biol 1999; 19:7897 - 912; PMID: 10567516
- Rosado IV, Dez C, Lebaron S, Caizergues-Ferrer M, Henry Y, de la Cruz J. Characterization of Saccharomyces cerevisiae Npa2p (Urb2p) reveals a low-molecular-mass complex containing Dbp6p, Npa1p (Urb1p), Nop8p, and Rsa3p involved in early steps of 60S ribosomal subunit biogenesis. Mol Cell Biol 2007; 27:1207 - 21; http://dx.doi.org/10.1128/MCB.01523-06; PMID: 17145778
- de la Cruz J, Lacombe T, Deloche O, Linder P, Kressler D. The putative RNA helicase Dbp6p functionally interacts with Rpl3p, Nop8p and the novel trans-acting Factor Rsa3p during biogenesis of 60S ribosomal subunits in Saccharomyces cerevisiae. Genetics 2004; 166:1687 - 99; http://dx.doi.org/10.1534/genetics.166.4.1687; PMID: 15126390
- Dez C, Froment C, Noaillac-Depeyre J, Monsarrat B, Caizergues-Ferrer M, Henry Y. Npa1p, a component of very early pre-60S ribosomal particles, associates with a subset of small nucleolar RNPs required for peptidyl transferase center modification. Mol Cell Biol 2004; 24:6324 - 37; http://dx.doi.org/10.1128/MCB.24.14.6324-6337.2004; PMID: 15226434
- Rosado IV, de la Cruz J. Npa1p is an essential trans-acting factor required for an early step in the assembly of 60S ribosomal subunits in Saccharomyces cerevisiae. RNA 2004; 10:1073 - 83; http://dx.doi.org/10.1261/rna.7340404; PMID: 15208443
- Kressler D, Doère M, Rojo M, Linder P. Synthetic lethality with conditional dbp6 alleles identifies rsa1p, a nucleoplasmic protein involved in the assembly of 60S ribosomal subunits. Mol Cell Biol 1999; 19:8633 - 45; PMID: 10567587
- Daugeron MC, Kressler D, Linder P. Dbp9p, a putative ATP-dependent RNA helicase involved in 60S-ribosomal-subunit biogenesis, functionally interacts with Dbp6p. RNA 2001; 7:1317 - 34; http://dx.doi.org/10.1017/S1355838201010640; PMID: 11565753
- Rasmussen TP, Culbertson MR. The putative nucleic acid helicase Sen1p is required for formation and stability of termini and for maximal rates of synthesis and levels of accumulation of small nucleolar RNAs in Saccharomyces cerevisiae. Mol Cell Biol 1998; 18:6885 - 96; PMID: 9819377
- Ursic D, Himmel KL, Gurley KA, Webb F, Culbertson MR. The yeast SEN1 gene is required for the processing of diverse RNA classes. Nucleic Acids Res 1997; 25:4778 - 85; http://dx.doi.org/10.1093/nar/25.23.4778; PMID: 9365256
- Kawauchi J, Mischo H, Braglia P, Rondon A, Proudfoot NJ. Budding yeast RNA polymerases I and II employ parallel mechanisms of transcriptional termination. Genes Dev 2008; 22:1082 - 92; http://dx.doi.org/10.1101/gad.463408; PMID: 18413718
- Ursic D, Chinchilla K, Finkel JS, Culbertson MR. Multiple protein/protein and protein/RNA interactions suggest roles for yeast DNA/RNA helicase Sen1p in transcription, transcription-coupled DNA repair and RNA processing. Nucleic Acids Res 2004; 32:2441 - 52; http://dx.doi.org/10.1093/nar/gkh561; PMID: 15121901
- LaCava J, Houseley J, Saveanu C, Petfalski E, Thompson E, Jacquier A, et al. RNA degradation by the exosome is promoted by a nuclear polyadenylation complex. Cell 2005; 121:713 - 24; http://dx.doi.org/10.1016/j.cell.2005.04.029; PMID: 15935758
- Vanácová S, Wolf J, Martin G, Blank D, Dettwiler S, Friedlein A, et al. A new yeast poly(A) polymerase complex involved in RNA quality control. PLoS Biol 2005; 3:e189; http://dx.doi.org/10.1371/journal.pbio.0030189; PMID: 15828860
- Jia H, Wang X, Anderson JT, Jankowsky E. RNA unwinding by the Trf4/Air2/Mtr4 polyadenylation (TRAMP) complex. Proc Natl Acad Sci USA 2012; 109:7292 - 7; http://dx.doi.org/10.1073/pnas.1201085109; PMID: 22532666
- Jia H, Wang X, Liu F, Guenther UP, Srinivasan S, Anderson JT, et al. The RNA helicase Mtr4p modulates polyadenylation in the TRAMP complex. Cell 2011; 145:890 - 901; http://dx.doi.org/10.1016/j.cell.2011.05.010; PMID: 21663793
- Bernstein J, Patterson DN, Wilson GM, Toth EA. Characterization of the essential activities of Saccharomyces cerevisiae Mtr4p, a 3'->5' helicase partner of the nuclear exosome. J Biol Chem 2008; 283:4930 - 42; http://dx.doi.org/10.1074/jbc.M706677200; PMID: 18096702
- Wlotzka W, Kudla G, Granneman S, Tollervey D. The nuclear RNA polymerase II surveillance system targets polymerase III transcripts. EMBO J 2011; 30:1790 - 803; http://dx.doi.org/10.1038/emboj.2011.97; PMID: 21460797
- Houseley J, LaCava J, Tollervey D. RNA-quality control by the exosome. Nat Rev Mol Cell Biol 2006; 7:529 - 39; http://dx.doi.org/10.1038/nrm1964; PMID: 16829983
- Milligan L, Decourty L, Saveanu C, Rappsilber J, Ceulemans H, Jacquier A, et al. A yeast exosome cofactor, Mpp6, functions in RNA surveillance and in the degradation of noncoding RNA transcripts. Mol Cell Biol 2008; 28:5446 - 57; http://dx.doi.org/10.1128/MCB.00463-08; PMID: 18591258
- de la Cruz J, Kressler D, Tollervey D, Linder P. Dob1p (Mtr4p) is a putative ATP-dependent RNA helicase required for the 3' end formation of 5.8S rRNA in Saccharomyces cerevisiae. EMBO J 1998; 17:1128 - 40; http://dx.doi.org/10.1093/emboj/17.4.1128; PMID: 9463390
- Granato DC, Machado-Santelli GM, Oliveira CC. Nop53p interacts with 5.8S rRNA co-transcriptionally, and regulates processing of pre-rRNA by the exosome. FEBS J 2008; 275:4164 - 78; http://dx.doi.org/10.1111/j.1742-4658.2008.06565.x; PMID: 18631361
- Mullineux ST, Lafontaine DL. Mapping the cleavage sites on mammalian pre-rRNAs: where do we stand?. Biochimie 2012; 94:1521 - 32; http://dx.doi.org/10.1016/j.biochi.2012.02.001; PMID: 22342225
- Stults DM, Killen MW, Pierce HH, Pierce AJ. Genomic architecture and inheritance of human ribosomal RNA gene clusters. Genome Res 2008; 18:13 - 8; http://dx.doi.org/10.1101/gr.6858507; PMID: 18025267
- Abdelhaleem M, Maltais L, Wain H. The human DDX and DHX gene families of putative RNA helicases. Genomics 2003; 81:618 - 22; http://dx.doi.org/10.1016/S0888-7543(03)00049-1; PMID: 12782131
- Wild T, Horvath P, Wyler E, Widmann B, Badertscher L, Zemp I, et al. A protein inventory of human ribosome biogenesis reveals an essential function of exportin 5 in 60S subunit export. PLoS Biol 2010; 8:e1000522; http://dx.doi.org/10.1371/journal.pbio.1000522; PMID: 21048991
- Sekiguchi T, Hayano T, Yanagida M, Takahashi N, Nishimoto T. NOP132 is required for proper nucleolus localization of DEAD-box RNA helicase DDX47. Nucleic Acids Res 2006; 34:4593 - 608; http://dx.doi.org/10.1093/nar/gkl603; PMID: 16963496
- Turner AJ, Knox AA, Prieto JL, McStay B, Watkins NJ. A novel small-subunit processome assembly intermediate that contains the U3 snoRNP, nucleolin, RRP5, and DBP4. Mol Cell Biol 2009; 29:3007 - 17; http://dx.doi.org/10.1128/MCB.00029-09; PMID: 19332556
- Schilders G, van Dijk E, Pruijn GJ. C1D and hMtr4p associate with the human exosome subunit PM/Scl-100 and are involved in pre-rRNA processing. Nucleic Acids Res 2007; 35:2564 - 72; http://dx.doi.org/10.1093/nar/gkm082; PMID: 17412707
- Zhang Y, Forys JT, Miceli AP, Gwinn AS, Weber JD. Identification of DHX33 as a mediator of rRNA synthesis and cell growth. Mol Cell Biol 2011; 31:4676 - 91; http://dx.doi.org/10.1128/MCB.05832-11; PMID: 21930779
- Jankowsky A, Guenther UP, Jankowsky E. The RNA helicase database. Nucleic Acids Res 2011; 39:Database issue D338 - 41; http://dx.doi.org/10.1093/nar/gkq1002; PMID: 21112871
- Andersen JS, Lam YW, Leung AK, Ong SE, Lyon CE, Lamond AI, et al. Nucleolar proteome dynamics. Nature 2005; 433:77 - 83; http://dx.doi.org/10.1038/nature03207; PMID: 15635413
- Boisvert FM, Ahmad Y, Gierliński M, Charrière F, Lamont D, Scott M, et al. A quantitative spatial proteomics analysis of proteome turnover in human cells. Mol Cell Proteomics 2012; 11:M111.011429; http://dx.doi.org/10.1074/mcp.M111.011429; PMID: 21937730
- Scherl A, Couté Y, Déon C, Callé A, Kindbeiter K, Sanchez JC, et al. Functional proteomic analysis of human nucleolus. Mol Biol Cell 2002; 13:4100 - 9; http://dx.doi.org/10.1091/mbc.E02-05-0271; PMID: 12429849
- Zhang S, Herrmann C, Grosse F. Nucleolar localization of murine nuclear DNA helicase II (RNA helicase A). J Cell Sci 1999; 112:2693 - 703; PMID: 10413677
- Zhang S, Köhler C, Hemmerich P, Grosse F. Nuclear DNA helicase II (RNA helicase A) binds to an F-actin containing shell that surrounds the nucleolus. Exp Cell Res 2004; 293:248 - 58; http://dx.doi.org/10.1016/j.yexcr.2003.10.018; PMID: 14729462
- Chakraborty P, Grosse F. Human DHX9 helicase preferentially unwinds RNA-containing displacement loops (R-loops) and G-quadruplexes. DNA Repair (Amst) 2011; 10:654 - 65; http://dx.doi.org/10.1016/j.dnarep.2011.04.013; PMID: 21561811
- Aratani S, Fujii R, Oishi T, Fujita H, Amano T, Ohshima T, et al. Dual roles of RNA helicase A in CREB-dependent transcription. Mol Cell Biol 2001; 21:4460 - 9; http://dx.doi.org/10.1128/MCB.21.14.4460-4469.2001; PMID: 11416126
- Fuller-Pace FV. DExD/H box RNA helicases: multifunctional proteins with important roles in transcriptional regulation. Nucleic Acids Res 2006; 34:4206 - 15; http://dx.doi.org/10.1093/nar/gkl460; PMID: 16935882
- Hartman TR, Qian S, Bolinger C, Fernandez S, Schoenberg DR, Boris-Lawrie K. RNA helicase A is necessary for translation of selected messenger RNAs. Nat Struct Mol Biol 2006; 13:509 - 16; http://dx.doi.org/10.1038/nsmb1092; PMID: 16680162
- Chan CC, Dostie J, Diem MD, Feng W, Mann M, Rappsilber J, et al. eIF4A3 is a novel component of the exon junction complex. RNA 2004; 10:200 - 9; http://dx.doi.org/10.1261/rna.5230104; PMID: 14730019
- Palacios IM, Gatfield D, St Johnston D, Izaurralde E. An eIF4AIII-containing complex required for mRNA localization and nonsense-mediated mRNA decay. Nature 2004; 427:753 - 7; http://dx.doi.org/10.1038/nature02351; PMID: 14973490
- Alexandrov A, Colognori D, Steitz JA. Human eIF4AIII interacts with an eIF4G-like partner, NOM1, revealing an evolutionarily conserved function outside the exon junction complex. Genes Dev 2011; 25:1078 - 90; http://dx.doi.org/10.1101/gad.2045411; PMID: 21576267
- Flores-Rozas H, Hurwitz J. Characterization of a new RNA helicase from nuclear extracts of HeLa cells which translocates in the 5' to 3' direction. J Biol Chem 1993; 268:21372 - 83; PMID: 8407977
- Valdez BC, Henning D, Busch RK, Woods K, Flores-Rozas H, Hurwitz J, et al. A nucleolar RNA helicase recognized by autoimmune antibodies from a patient with watermelon stomach disease. Nucleic Acids Res 1996; 24:1220 - 4; http://dx.doi.org/10.1093/nar/24.7.1220; PMID: 8614622
- Yang H, Zhou J, Ochs RL, Henning D, Jin R, Valdez BC. Down-regulation of RNA helicase II/Gu results in the depletion of 18 and 28 S rRNAs in Xenopus oocyte. J Biol Chem 2003; 278:38847 - 59; http://dx.doi.org/10.1074/jbc.M302258200; PMID: 12851405
- Henning D, So RB, Jin R, Lau LF, Valdez BC. Silencing of RNA helicase II/Gualpha inhibits mammalian ribosomal RNA production. J Biol Chem 2003; 278:52307 - 14; http://dx.doi.org/10.1074/jbc.M310846200; PMID: 14559904
- Yang H, Henning D, Valdez BC. Functional interaction between RNA helicase II/Gu(alpha) and ribosomal protein L4. FEBS J 2005; 272:3788 - 802; http://dx.doi.org/10.1111/j.1742-4658.2005.04811.x; PMID: 16045751
- Valdez BC, Perlaky L, Henning D. Expression, cellular localization, and enzymatic activities of RNA helicase II/Gu(beta). Exp Cell Res 2002; 276:249 - 63; http://dx.doi.org/10.1006/excr.2002.5538; PMID: 12027455
- Romanova L, Grand A, Zhang L, Rayner S, Katoku-Kikyo N, Kellner S, et al. Critical role of nucleostemin in pre-rRNA processing. J Biol Chem 2009; 284:4968 - 77; http://dx.doi.org/10.1074/jbc.M804594200; PMID: 19106111
- Hölzel M, Rohrmoser M, Schlee M, Grimm T, Harasim T, Malamoussi A, et al. Mammalian WDR12 is a novel member of the Pes1-Bop1 complex and is required for ribosome biogenesis and cell proliferation. J Cell Biol 2005; 170:367 - 78; http://dx.doi.org/10.1083/jcb.200501141; PMID: 16043514
- Fujiyama S, Yanagida M, Hayano T, Miura Y, Isobe T, Fujimori F, et al. Isolation and proteomic characterization of human Parvulin-associating preribosomal ribonucleoprotein complexes. J Biol Chem 2002; 277:23773 - 80; http://dx.doi.org/10.1074/jbc.M201181200; PMID: 11960984
- Holmström TH, Mialon A, Kallio M, Nymalm Y, Mannermaa L, Holm T, et al. c-Jun supports ribosomal RNA processing and nucleolar localization of RNA helicase DDX21. J Biol Chem 2008; 283:7046 - 53; http://dx.doi.org/10.1074/jbc.M709613200; PMID: 18180292
- Srivastava L, Lapik YR, Wang M, Pestov DG. Mammalian DEAD box protein Ddx51 acts in 3' end maturation of 28S rRNA by promoting the release of U8 snoRNA. Mol Cell Biol 2010; 30:2947 - 56; http://dx.doi.org/10.1128/MCB.00226-10; PMID: 20404093
- Saporita AJ, Chang HC, Winkeler CL, Apicelli AJ, Kladney RD, Wang J, et al. RNA helicase DDX5 is a p53-independent target of ARF that participates in ribosome biogenesis. Cancer Res 2011; 71:6708 - 17; http://dx.doi.org/10.1158/0008-5472.CAN-11-1472; PMID: 21937682
- Iggo RD, Jamieson DJ, MacNeill SA, Southgate J, McPheat J, Lane DP. p68 RNA helicase: identification of a nucleolar form and cloning of related genes containing a conserved intron in yeasts. Mol Cell Biol 1991; 11:1326 - 33; PMID: 1996094
- Fukuda T, Yamagata K, Fujiyama S, Matsumoto T, Koshida I, Yoshimura K, et al. DEAD-box RNA helicase subunits of the Drosha complex are required for processing of rRNA and a subset of microRNAs. Nat Cell Biol 2007; 9:604 - 11; http://dx.doi.org/10.1038/ncb1577; PMID: 17435748
- Ogilvie VC, Wilson BJ, Nicol SM, Morrice NA, Saunders LR, Barber GN, et al. The highly related DEAD box RNA helicases p68 and p72 exist as heterodimers in cells. Nucleic Acids Res 2003; 31:1470 - 80; http://dx.doi.org/10.1093/nar/gkg236; PMID: 12595555
- Jalal C, Uhlmann-Schiffler H, Stahl H. Redundant role of DEAD box proteins p68 (Ddx5) and p72/p82 (Ddx17) in ribosome biogenesis and cell proliferation. Nucleic Acids Res 2007; 35:3590 - 601; http://dx.doi.org/10.1093/nar/gkm058; PMID: 17485482
- Zirwes RF, Eilbracht J, Kneissel S, Schmidt-Zachmann MS. A novel helicase-type protein in the nucleolus: protein NOH61. Mol Biol Cell 2000; 11:1153 - 67; PMID: 10749921
- Gee S, Krauss SW, Miller E, Aoyagi K, Arenas J, Conboy JG. Cloning of mDEAH9, a putative RNA helicase and mammalian homologue of Saccharomyces cerevisiae splicing factor Prp43. Proc Natl Acad Sci USA 1997; 94:11803 - 7; http://dx.doi.org/10.1073/pnas.94.22.11803; PMID: 9342318
- Darnell RB. HITS-CLIP: panoramic views of protein-RNA regulation in living cells. Wiley Interdiscip Rev RNA 2010; 1:266 - 86; http://dx.doi.org/10.1002/wrna.31; PMID: 21935890
- Martin A, Schneider S, Schwer B. Prp43 is an essential RNA-dependent ATPase required for release of lariat-intron from the spliceosome. J Biol Chem 2002; 277:17743 - 50; http://dx.doi.org/10.1074/jbc.M200762200; PMID: 11886864
- Garcia I, Uhlenbeck OC. Differential RNA-dependent ATPase activities of four rRNA processing yeast DEAD-box proteins. Biochemistry 2008; 47:12562 - 73; http://dx.doi.org/10.1021/bi8016119; PMID: 18975973
- Rocak S, Emery B, Tanner NK, Linder P. Characterization of the ATPase and unwinding activities of the yeast DEAD-box protein Has1p and the analysis of the roles of the conserved motifs. Nucleic Acids Res 2005; 33:999 - 1009; http://dx.doi.org/10.1093/nar/gki244; PMID: 15718299
- Ben-Shem A, Garreau de Loubresse N, Melnikov S, Jenner L, Yusupova G, Yusupov M. The structure of the eukaryotic ribosome at 3.0 Å resolution. Science 2011; 334:1524 - 9; http://dx.doi.org/10.1126/science.1212642; PMID: 22096102
- Klinge S, Voigts-Hoffmann F, Leibundgut M, Arpagaus S, Ban N. Crystal structure of the eukaryotic 60S ribosomal subunit in complex with initiation factor 6. Science 2011; 334:941 - 8; http://dx.doi.org/10.1126/science.1211204; PMID: 22052974
- Strunk BS, Loucks CR, Su M, Vashisth H, Cheng S, Schilling J, et al. Ribosome assembly factors prevent premature translation initiation by 40S assembly intermediates. Science 2011; 333:1449 - 53; http://dx.doi.org/10.1126/science.1208245; PMID: 21835981
- Lange H, Sement FM, Gagliardi D. MTR4, a putative RNA helicase and exosome co-factor, is required for proper rRNA biogenesis and development in Arabidopsis thaliana. Plant J 2011; 68:51 - 63; http://dx.doi.org/10.1111/j.1365-313X.2011.04675.x; PMID: 21682783
- Huang CK, Huang LF, Huang JJ, Wu SJ, Yeh CH, Lu CA. A DEAD-box protein, AtRH36, is essential for female gametophyte development and is involved in rRNA biogenesis in Arabidopsis. Plant Cell Physiol 2010; 51:694 - 706; http://dx.doi.org/10.1093/pcp/pcq045; PMID: 20378763
- Yedavalli VS, Neuveut C, Chi YH, Kleiman L, Jeang KT. Requirement of DDX3 DEAD box RNA helicase for HIV-1 Rev-RRE export function. Cell 2004; 119:381 - 92; http://dx.doi.org/10.1016/j.cell.2004.09.029; PMID: 15507209
- Dubaele S, Chène P. Cellular studies of MrDb (DDX18). Oncol Res 2007; 16:549 - 56; http://dx.doi.org/10.3727/000000007783630015; PMID: 18351129
- Imamura O, Saiki K, Tani T, Ohshima Y, Sugawara M, Furuichi Y. Cloning and characterization of a human DEAH-box RNA helicase, a functional homolog of fission yeast Cdc28/Prp8. Nucleic Acids Res 1998; 26:2063 - 8; http://dx.doi.org/10.1093/nar/26.9.2063; PMID: 9547260
- Qu X, Yang Z, Zhang S, Shen L, Dangel AW, Hughes JH, et al. The human DEVH-box protein Ski2w from the HLA is localized in nucleoli and ribosomes. Nucleic Acids Res 1998; 26:4068 - 77; http://dx.doi.org/10.1093/nar/26.17.4068; PMID: 9705521