Abstract
The nucleolinus is a nuclear subcompartment long ago posited to play a role in cell division. In a recent study using surf clam oocytes, cytoplasmic foci containing a nucleolinar protein were shown to later recruit γ-tubulin, identifying them as centrosomal precursors.Citation1 We now demonstrate the presence of structural RNAs from the nucleolinus in these procentrosomes. They include the well-known but poorly understood rRNA-transcribed spacer regions. In situ hybridization revealed a specific and dynamic association of these structural RNAs with the cell division apparatus that extends through the early stages of meiosis. In addition to their bearing on the debate over the nature of centrosome- and spindle-associated RNAs, the observations also suggest that rRNA spacer regions are not simply waste products to be discarded immediately, but may be functional byproducts that play a role in formation of the cell division apparatus.
Introduction
Genes for rRNA are transcribed into a single pre-rRNA unit, referred to as the 45S cassette. In eukaryotes, the overall structure of the 45S cassette is highly conserved. From the 5′ to the 3′ end, it consists of a 5′ external transcribed spacer region (5′-ETS), the 18S rRNA subunit, internal transcribed spacer region-1 (ITS-1), 5.8S rRNA, ITS-2, 28S rRNA and a 3′-ETS. In addition to this conserved topology, the nucleotide sequence of the rRNA subunits is also highly conserved. The sequences of the spacer regions, however, are not. Indeed, divergence in rRNA spacer regions can be so great even between related taxa that informatics analyses reveal no significant similarities. This variability in transcribed spacer regions has been exploited to great effect in studies of both prokaryotic and eukaryotic molecular systematics.Citation2-Citation4
Processing pre-rRNA to mature rRNA is well-studied and, with some variation in the sequence of events, follows a characterized pattern of rapid trimming, excision and degradation of the spacer regions.Citation5 The studies demonstrating these events were, however, strictly biochemical. As such, they could not effectively address whether a portion of pre-rRNA transcribed spacers might persist in the cell or, if so, whether they are localized in specific structures. Eukaryotic rRNA transcribed spacers are, therefore, generally viewed as cellular chaffe. They contain necessary recognition sites for pre-RNA processing,Citation6,Citation7 but aside from this, there is no known role for rRNA spacer regions. Yet, if only a tiny ration of rRNA spacers persisted after processing of pre-rRNA to mature rRNA, this pool could represent a massive source of structural and regulatory RNAs for the cell. A finding that these structural domains are destined for more than just immediate degradation would open up new areas of research and enlighten our understanding of broader nucleolar function.
Closely associated with the nucleolus and yet a distinct compartment in Spisula oocytes is the nucleolinus. Although we know very little about this structure, it was discovered over 150 y ago,Citation8,Citation9 has been described in a variety of cell types and is thought to play a role in cell division.Citation10-Citation14 In this report, we show that rRNA transcribed spacer regions are stored in the nucleolinus of unfertilized surf clam oocytes. After fertilization and nuclear envelope breakdown, rRNA spacer regions persist in the oocyte cytoplasm in association with the developing centrosomes and spindle. Therefore, in addition to matters of rRNA processing and nucleolar function, the observations help elucidate the resident transcriptome of the nucleolinus and the nature of nucleic acids associated with the cell division apparatus—by itself an old and controversial subject area.Citation15,Citation16
Results
Nucleolinar RNAs include rRNA transcribed spacer regions
Approximately 50 different RNAs have been found associated with the nucleolinus in biochemical preparations, including six confirmed by in situ localization.Citation1,Citation17 The focus of these previous studies was on RNA found exclusively in the nucleolinus, so clones containing rRNA sequences were screened out of the analyses. However, some clones were later found to join the 3′ end of a sequence originally designated NLi-1 and the 5′ end of Spisula 18S rRNA. To clarify the organization and context of these sequences, we determined the sequence of the entire Spisula 45S pre-rRNA cassette (). Fragmentary data from other molluscs (Tritura and Crepidula) were initially used as a scaffold to aid with the assembly of clones present in our existing Spisula library. All gaps in the Spisula sequence were subsequently filled with PCR products generated in a stepwise fashion. It was determined from this effort that NLi-1 RNA corresponds precisely to the 5′-ETS of Spisula 45S rRNA. Finding the 5′-ETS present and persistent (among other RNAs) in the nucleolinus, a structure demonstrated to play a role in cell division,Citation1,Citation17 was unexpected.
Figure 1. Structure of the Spisula 45S rRNA cassette. Red segments represent transcribed spacer regions, blue segments correspond with mature rRNA subunits. Length of the 45S cassette is indicated in the scale bar, below. Gray bars at the top of the diagram indicate riboprobe target domains for the in situ hybridizations shown in and . Note that the 5′-ETS was targeted with two probes, one of approximately 1,000 nt, and one of 101 nt. The full nucleotide sequence of the Spisula 45S cassette is available under GenBank accession JN196041.

We aimed to determine by in situ hybridization if the 5′-ETS localization was unique, or if other components of the 45S cassette were present specifically in the nucleolinus of unactivated oocytes. We found that ITS-1 and ITS-2 were also highly concentrated in that structure (). The 3′-ETS could not be detected. With limited sequence data on the 3′-ETS, our riboprobe for this domain was necessarily small (93nt), and we would therefore expect sensitivity to be correspondingly low. To be certain the lack of signal for the 3′-ETS was related to low target abundance and not an artifact of low probe sensitivity, a similarly-sized (101nt) riboprobe was made to target the 5′-ETS. This sequence was readily detected in the nucleolinus with high signal intensity, even at lower probe concentration and higher stringency hybridization conditions than used for the 3′-ETS. The inability to detect the 3′-ETS in the nucleolinus or elsewhere in unactivated oocytes therefore truly reflected its abundance.
Figure 2. Localization of pre-rRNA subdomains in the unactivated oocyte. DIC images (top row) of unactivated oocytes and corresponding in situ hybridizations (bottom row). The 5′-ETS, ITS-1 and ITS-2 are strongly concentrated in the nucleolinus. No signal was detected using a riboprobe for the 3′-ETS. 18S and 28S rRNA show a granular pattern and are distributed throughout the cytoplasm as would be expected for ribosomes. Note that the 18S and 28S hybridization micrographs in this figure are taken slightly above the DIC focal plane so that all pertinent structures are visible. Corresponding opposite (sense) strand probes were used in each case as a negative control and yielded no signal above background. One example for each of the two observed labeling patterns (transcribed spacer and rRNA subunits) is shown in the last two pairs of images on the far right. Arrows indicate position of the nucleolinus. Three arrowheads in each of the DIC panels are used to delineate the boundaries of the nucleolus, which are difficult to see in some images.
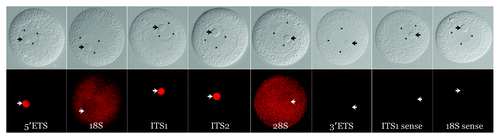
Other controls included the use of riboprobes targeting 18S and 28S rRNA. As expected, these sequences appeared in a granular pattern in the cytoplasm, presumably representing the distribution of ribosomes. There was no apparent concentration of 18S and 28S rRNA in the oocyte nucleolus, indicating that the mass of these transcripts is exported to the cytoplasm prior to cell cycle arrest in prophase of meiosis I. It is important to note that these in situ hybridization probes cannot distinguish between pre- and post-processed transcripts. It is therefore necessary to consider whether transcribed spacer regions concentrated in the nucleolinus were part of intact, full-length pre-rRNA transcripts, looping down into the structure. However, if the 45S transcripts were intact, a concentration of 18S and 28S signal would necessarily be observed surrounding the nucleolinus. Since this was not the case, we conclude that 5′-ETS, ITS-1 and ITS-2 signal in the nucleolinus represents post-processed RNA.
Association of rRNA transcribed spacer regions with the meiotic cell division apparatus
The Spisula oocyte is arrested in prophase of meiosis I. Centrosomes are not present in the unactivated oocyte.Citation18 They have been described as forming “de novo” in the cytoplasm, approximately 4‒5 min following fertilization or parthenogenetic activation with KCl.Citation13 However, a mAb generated against the nucleolinar protein, nucleolinin, reveals forerunners of centrosomes prior to their recruitment of γ-tubulin (). Nucleolinin-containing procentrosomesFootnotea appear to bud from the nucleolinus during the first 2 min of development. They can also be observed in a low percentage (< 10%) of unactivated oocytes.Citation1 MTOCs originating from nuclear components has been observed previously in cells with both closed mitosisCitation19,Citation20 as well as open mitosis.Citation21 Thus, the observation that elements of the MTOC in Spisula oocytes are derived from the nucleus (specifically, the nucleolinus) is not without precedent. We now report that these Spisula oocyte procentrosomes and early centrosomes contain structural RNAs derived from the nucleolinus in the form of 45S pre-rRNA transcribed spacer regions ().
Figure 3. Presence of structural RNA in the procentrosome. Meiotic centrosomes in Spisula oocytes form in the cytoplasm 4‒5 min post-fertilization. They contain the universal centrosomal protein, γ-tubulin. Prior to 4 min, developing procentrosomes express the nucleolinar marker protein, nucleolinin, which is then co-expressed in the centrosome with γ-tubulin (B and C). Structural RNAs from the nucleolinus in the form of rRNA transcribed spacer sequences are present in these nucleolinin-containing procentrosomes (D and E). These particular RNAs are dissipated after γ-tubulin recruitment to the structure, while other transcripts (not necessarily associated with the nucleolinus) persist.Citation31-Citation33,Citation35 (A–C) shows an oocyte 8 min post-insemination co-labeled by indirect immunofluorescence for the centrosomal/procentrosomal marker nucleolinin and by direct immunofluorescence with a fluorophore-conjugated antibody for the centrosomal marker, γ-tubulin. (D–F) shows an oocyte at 2 min post-insemination co-labeled by indirect immunofluorescence with an antibody for the procentrosomal/centrosomal marker nucleolinin and a DIG-UTP riboprobe for the 5′-ETS imaged by direct fluorescence with a sheep-anti-DIG primary antibody.
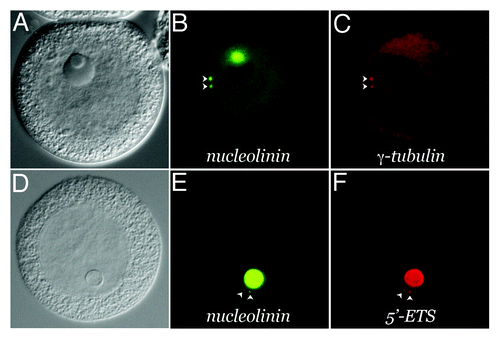
The incidence of rRNA spacer-containing foci is variable (ranging up to 25% of cells) and temporally dynamic. As procentrosomes recruit γ-tubulin (at 4–5 min), they lose signal for rRNA spacers first and nucleolinin shortly thereafter. By 8 min post-insemination, the incidence of foci positive for transcribed spacer RNA is less than 5%. A number of observations strongly suggest that this colocalization with procentrosomes and centrosomes is genuine and specific: (1) control (opposite strand) riboprobes for the 5′-ETS, ITS-1 and ITS-2 yielded no signal; (2) probes for 18S and 28S rRNA subunits exhibited an altogether different labeling pattern; (3) these RNA foci occurred in every batch of oocytes examined and colocalized with nucleolinin; (4) with rare exception, the number of RNA-containing foci did not exceed two per oocyte. We conclude that these puncta truly represent procentrosomes and early centrosomes.
The dynamics that result in a relatively low incidence of rRNA spacer-containing puncta are not elucidated here. We can formulate two possible explanations for the observation: (1) only a portion of procentrosomes/centrosomes contain these transcripts, or (2) the presence of these transcripts in procentrosomes and centrosomes is transient. The former explanation would suggest that developing centrosomes are not qualitatively the same—some incorporating these particular structural RNAs, and others not. The latter possibility is consistent with the observation that MTOC composition is dynamic in general. For example, the essential cell cycle regulator Aurora kinase is present only transiently in the spindle pole body of Plasmodium falciparum.Citation22 In Spisula oocytes, nucleolinin expression in centrosomes is itself temporary.Citation1 The large size, translucent nature and other unique characteristics of Spisula oocytes have provided distinct advantages for studying the structures in question. However, determining the precise sequence of these events will necessitate the use of a different experimental system, one amenable to real-time imaging so that the flow of markers into and out of specific structures can be tracked.
The more general association of these structural RNAs with the developing spindle is also dynamic, but persists over a longer time period ().Footnoteb From 2‒8 min post-insemination, procentrosomes and centrosomes hover at the periphery of the nucleolinar RNA patch before separating from each other and forming asters, which are first seen at the cytoplasmic edge of the collapsing GV. During this time period, the nucleolinus is present as an intact structure. Subsequently, as the DIC-visible nucleolinus dissipates, a concentrated patch of nucleolinar RNA remains behind. By 12 min post-insemination, nucleolinar RNA and centrosomes (with growing spindle and astral microtubule arrays) remain closely associated, with the nucleolinar RNA patch between the two centrosomes. As the spindle begins to elongate the nucleolinar RNA patch, now smaller and more diffused around the edges, becomes positioned near one pole before dissipating. Neither the 5′-ETS, ITS-1 nor ITS-2 are detectable by in situ hybridization at 20 min post-insemination (metaphase). These observations on the fate and associations of nucleolinar RNA are consistent with earlier reports based on phase contrast and DIC images of the nucleolinus itself. Both AllenCitation12,Citation13 and Alliegro et al.Citation17 reported the nucleolinus to take up a position adjacent to one pole of the meiotic spindle.
Figure 4. Association of 5′-ETS RNA with meiotic spindle components. The diagram shown in ) (A‒D) are not drawn to scale. Chromosomes and sperm components (nucleus and asters) are excluded for clarity. Nucleolinar RNA is colored red. Centrosomes, asters and spindle microtubules are colored green. GV, germinal vesicle; N, nucleolus. A, configuration up to approximately 8 min post-insemination. The nucleolinus is still intact. Procentrosomes and centrosomes hover at the periphery of the nucleolinar RNA patch before separating from each other and forming asters (B), which are first seen at the cytoplasmic edge of the collapsing GV (also see ). As the nucleolinus dissipates, a concentrated patch of RNA remains. By 12 min post-insemination, nucleolinar RNA and centrosomes (with growing spindle and astral arrays) remain closely associated (C). The nucleolinar RNA patch can be found between the developing poles, though not always arranged linearly. As the spindle begins to elongate, the nucleolinar RNA patch becomes smaller and more diffused around the edges, taking a position near one pole (D). (E‒H), DIC micrographs of oocytes fixed within the time blocks diagrammed above. (I–L), fluorescent images of the cells in (E–H) showing the distribution of 5′-ETS (red) and α-tubulin (green). Arrowheads in (I) highlight two 5′-ETS foci. Note that spindle integrity and α-tubulin antigenicity in these samples are severely compromised due to harsh hybridization conditions, including proteinase-K treatment and high temperature. Images of α-tubulin (microtubules) distribution in (J–L) are therefore of low quality, but nevertheless mark the vicinity of the asters and two half-spindles (arrowheads in J–L). A third astral array can be observed in some images (arrow in K). They are associated with the sperm nucleus, which is visible with bis-benzimide stain and often by DIC (arrow in G).
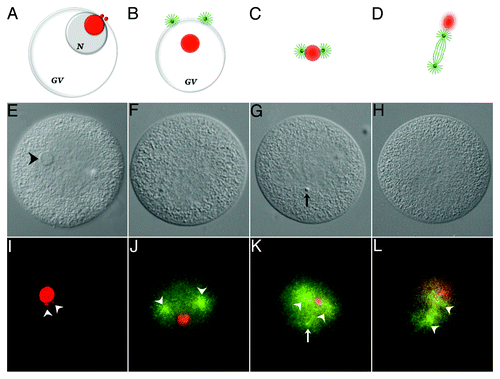
Discussion
The observations on the distribution of rRNA transcribed spacer domains in Spisula oocytes presented here are relevant to several fundamental topics in cell biology. First, they address the composition of the nucleolinus, an enigmatic nuclear compartment described over 150 y agoCitation8,Citation9 and long posited to play a role in cell division.Citation10-Citation14 Until recently, almost nothing was known about the specific molecular composition of the nucleolinus. Along with other non-rRNAs and a collection of proteins involved in RNA metabolism and cell cycle regulation,Citation1 we now know that major components of the nucleolinus include structural RNAs derived from the 45S ribosomal cassette.
Second, these observations impact our understanding of pre-rRNA metabolism. Other than a passive role in the processing event itself,Citation6,Citation7,Citation23 there is no known function for transcribed spacer sequences. Earlier biochemical studies show clearly that they are excised from pre-rRNA and rapidly degraded. This has led to the (perhaps erroneous) assumption that not even an aliquot of this massive pool has a functional existence beyond processing itself. In prokaryotes, transcribed spacer regions contain the precursors of functional tRNAs.Citation3,Citation24 Was similar parsimony discarded at the beginning of the eukaryotic lineage? The sheer quantity of these sequences produced by the cell itself begs the question of functionality. Even if 99% of transcribed spacer sequences were immediately and utterly destroyed, the remaining 1% would still exceed the vast majority of mRNAs in the cell. This mass could serve a number of purposes, including structural roles or as precursors for small regulatory RNAs.
Third, a link between the nucleolus and cell division apparatus is newly revealed. Previously thought of in narrow terms as the cellular site for the genesis of ribosomal precursors, we now know that the nucleolus is a repository for microRNAs and serves as an assembly site for the sequence recognition particle (SRP) used to guide the insertion of integral membrane proteins.Citation25 Evidence that the nucleolus also plays a direct role in cell cycle regulation was first provided in laser microbean studies by Gaulden and Perry.Citation26 The question remained largely dormant for the next 30+ y as the substructure of the nucleolus and its role in ribosome biogenesis was unraveled. However, the intimate relationship of the nucleolus with cell cycle regulation, above and beyond providing the protein synthetic capacity for growth and division, has since been confirmed. Cell cycle regulatory proteins such as p53 and Mdm2 must be sequestered and modified in the nucleolus for normal cell cycle progression to occur.Citation27,Citation28 Inactivation of the nucleolar protein, nucleolin, results in centrosome duplication defects.Citation29 Thus, the nucleolus and cell cycle machinery are functionally tethered in a direct manner. The presence of rRNA spacer regions in the nucleolinus, procentrosome and meiotic spindle provide a material link between the nucleolus and the cell cycle.
Finally, this study impacts the debate on the presence and role of nucleic acids associated with the cell division apparatus, and the centrosomes in particular. The first phase of this debate centered on whether centrosomes contained RNA at all (reviewed in Marshall and RosenbaumCitation30). This question has been settled in the affirmative by several independent laboratories.Citation31-Citation33 The debate now centers on whether centrosomal RNA is structural or informational, transient or resident. These categories are, of course, not mutually exclusive. Combined with these other recent reports, the present observations indicate that centrosomes contain different species of RNA at different stages of their development, including non-coding RNA. It is also important to note that only three RNAs have been interrogated in the present study, and that all three of these belong to just one small group. Estimates for the number of RNAs associated with mature centrosomes run into the dozens.Citation31-Citation33 This may be an underestimate, since two of the three studies published on the subjectCitation32,Citation33 were based on expression libraries and therefore do not account for non-coding RNA. We hypothesize that when we have sampled the full complement of transcripts associated with centrosomes, we will find examples from all four categories—structural, informational, transient and resident RNAs.
Materials and Methods
Gravid Spisula solidissima were obtained from the Marine Resources Center at the Marine Biological Laboratory. Gametes were collected by dissection and filtered through cheesecloth. Oocytes were rinsed several times in 0.45 µm filtered sea water, fertilized with sperm (diluted 1/20,000) in Tris-buffered (pH 8.0), filtered sea water and fixed at specific time points in 4% paraformaldehyde + 0.6% Brij 58 as described previously.Citation17 All brightfield and immunofluorescence imaging was performed with a Nikon 90i microscope equipped with DIC, epifluorescence and a Photometrics CoolSNAP EZ digital camera. Immunofluorescence was performed, with minor modifications, as described in an earlier report.Citation34 To visualize centrosomes and avoid cross-talk between secondary antibodies, Sigma GTU-88 anti-γ-tubulin IgG was directly conjugated to Alexa fluor-594 (Invitrogen). Nucleolinin was labeled with mAb23 followed by an Alexa Fluor 488-conjugated goat anti-mouse 2° antibody. The Roche anti-digoxygenin antibody used to visualize hybridized riboprobe was derived from sheep. Every localization experiment was accompanied by a battery of controls for antibody specificity, cross-reactivity and fluorophore channel overlap.
RNA was extracted from isolated nucleoliniCitation1 using Qiagen RNeasy reagents, reverse transcribed using universal primers described previouslyCitation31 and blunt-cloned into Invitrogen PCR-script plasmid. More than 3,000 clones were sequenced by the Sanger method. Sequence analysis and assembly were performed using MacVector and CodonCode Aligner software. Methods for in situ hybridization were described in an earlier report.Citation17 The protocol was optimized to conserve nucleolar and nucleolinar morphology (both of which are labile) throughout the hybridization regimen, as well as γ-tubulin antigenicity. In situ hybridization conditions could not be found that did not compromise the antigenicity of α- or β-tubulin. Samples were hybridized with digoxygenin-labeled nucleolinar RNA probes for 2 d at 58–60°C. Roche Texas Red- or fluorescein-conjugated sheep anti-DIG antibody was used to visualize in situ signal. Specificity controls for in situ hybridization included opposite strand (“sense”) hybridizations and comparison of labeling pattern with other RNA probes.
Abbreviations: | ||
MTOC | = | microtubule organizing center |
ETS | = | external transcribed spacer |
ITS | = | internal transcribed spacer |
SRP | = | recognition particle |
mAb | = | monoclonal antibody |
Acknowledgments
This work was supported by a grant from the NIH (GM088503) to M.C.A.
Notes
a In this report, centrosomes are defined as the two γ-tubulin-containing foci that form in the cytoplasm between 4 and 5 min post-fertilization and take up positions at the spindle poles during the course of meiosis. Procentrosomes are defined as cytoplasmic nucleolinin-containing foci that appear within the first 2 min post-fertilization and become γ-tubulin-positive shortly thereafter.
b Note that spindle integrity and α-tubulin antigenicity in these hybridized samples is severely compromised due to the required proteinase-K treatment and stringent hybridization conditions, including 2 d at 60°C. The spindle images shown in are therefore of low quality, but still reveal the position and extent of the spindle, and are consistent with observations using γ-tubulin antibody to reveal centrosomes.Citation1
References
- Alliegro MC, Hartson S, Alliegro MA. Composition and dynamics of the nucleolinus, a physiological link between the nucleolus and cell division apparatus. J Biol Chem 2012; 287:6702 - 13; http://dx.doi.org/10.1074/jbc.M111.288506; PMID: 22219192
- Baldwin BG. Phylogenetic utility of the internal transcribed spacers of nuclear ribosomal DNA in plants: an example from the compositae. Mol Phylogenet Evol 1992; 1:3 - 16; http://dx.doi.org/10.1016/1055-7903(92)90030-K; PMID: 1342921
- Boyer SL, Flechtner VR, Johansen JR. Is the 16S-23S rRNA internal transcribed spacer region a good tool for use in molecular systematics and population genetics? A case study in cyanobacteria. Mol Biol Evol 2001; 18:1057 - 69; http://dx.doi.org/10.1093/oxfordjournals.molbev.a003877; PMID: 11371594
- Alvarez I, Wendel JF. Ribosomal ITS sequences and plant phylogenetic inference. Mol Phylogenet Evol 2003; 29:417 - 34; http://dx.doi.org/10.1016/S1055-7903(03)00208-2; PMID: 14615184
- Lodish H, Berk A, Zipursky SL. Molecular Cell Biology. Processing of rRNA and tRNA 2000; 4th edition. W. H. Freeman, New York.
- Allmang C, Tollervey D. The role of the 3′ external transcribed spacer in yeast pre-rRNA processing. J Mol Biol 1998; 278:67 - 78; http://dx.doi.org/10.1006/jmbi.1998.1693; PMID: 9571034
- Liiv A, Tenson T. Margus, Remme J. Multiple functions of the transcribed spacers in ribosomal RNA operons. J Biol Chem 1998; 379:783 - 93
- Agassiz L. Contributions to the Natural History of the United States of America. 1857; First Monograph. Part IIL Embryology of the Turtle. Boston.
- Haeckel E. The Evolution of Man: A Popular Exposition of the Principal Points of Human Ontogeny and Phylogeny (in German). Appleton Press, New York, 1874.
- Lavdowsky M.. Formation of the chromatic and achromatic substances in animal and plant cells. Merkel Bonnet’s Anat Hefte 1894; 4:13
- Carleton HM. Observations on an intra-nucleolar body in columnar epithelium cells of the intestine. Quart J Microsc Sci 1920; 64:329 - 341
- Allen RD. The role of the nucleolus in spindle formation. Biol. Bull. 1951; 101:214
- Allen RD. Fertilization and artificial activation in the egg of the surf calm, Spisula solidissima.. Biol Bull 1953; 105:213 - 39; http://dx.doi.org/10.2307/1538639
- Love R, Wildy P. Cytochemical studies of the nucleoproteins of HeLa cells infected with herpes virus. J Cell Biol 1963; 17:237 - 54; http://dx.doi.org/10.1083/jcb.17.2.237; PMID: 19866625
- Alliegro MC. The implications of centrosomal RNA. RNA Biol 2008; 5:198 - 200; PMID: 18948757
- Alliegro MC. The centrosome and spindle as a ribonucleoprotein complex. Chromosome Res 2011; 19:367 - 76; http://dx.doi.org/10.1007/s10577-011-9186-7; PMID: 21287260
- Alliegro MA, Henry JJ, Alliegro MC. Rediscovery of the nucleolinus, a dynamic RNA-rich organelle associated with the nucleolus, spindle, and centrosomes. Proc Natl Acad Sci USA 2010; 107:13718 - 23; http://dx.doi.org/10.1073/pnas.1008469107; PMID: 20643950
- Palazzo RE, Vaisberg E, Cole RW, Rieder CL. Centriole duplication in lysates of Spisula solidissima oocytes. Science 1992; 256:219 - 21; http://dx.doi.org/10.1126/science.1566068; PMID: 1566068
- Laane MM, Haugli FB. Division centers in mitotic nuclei of Physarum polycephalum plasmodia. Nor. J Bot 1974; 21:309 - 18
- Tanaka K. Intranuclear microtubule organizing center in early prophase nuclei of the plasmodium of the slime mold, Physarum polycephalum.. J Cell Biol 1973; 57:220 - 4; http://dx.doi.org/10.1083/jcb.57.1.220; PMID: 4734866
- Ferree PM, McDonald K, Fasulo B, Sullivan W. The origin of centrosomes in parthenogenetic hymenopteran insects. Curr Biol 2006; 16:801 - 7; http://dx.doi.org/10.1016/j.cub.2006.03.066; PMID: 16631588
- Reininger L, Wilkes JM, Bourgade H, Miranda-Saavedra D, Doerig C. An essential Aurora-related kinase transiently associates with spindle pole bodies during Plasmodium falciparum erythrocytic schizogony. Mol Microbiol 2011; 79:205 - 21; http://dx.doi.org/10.1111/j.1365-2958.2010.07442.x; PMID: 21166904
- Musters W, Boon K, van der Sande CA, van Heerikhuizen H, Planta RJ. Functional analysis of transcribed spacers of yeast ribosomal DNA. EMBO J 1990; 9:3989 - 96; PMID: 2249660
- Srivastava AK, Schlessinger D. Mechanism and regulation of bacterial ribosomal RNA processing. Annu Rev Microbiol 1990; 44:105 - 29; http://dx.doi.org/10.1146/annurev.mi.44.100190.000541; PMID: 1701293
- Pederson T. The nucleolus. Cold Spring Harb Perspect Biol 2011; 3:1 - 15; http://dx.doi.org/10.1101/cshperspect.a000638
- Gaulden ME, Perry RP. Influence of the nucleolus on mitosis as revealed by ultraviolet microbeam irradiation. Proc Natl Acad Sci USA 1958; 44:553 - 9; http://dx.doi.org/10.1073/pnas.44.6.553; PMID: 16590238
- Weber JD, Taylor LJ, Roussel MF, Sherr CJ, Bar-Sagi D. Nucleolar Arf sequesters Mdm2 and activates p53. Nat Cell Biol 1999; 1:20 - 6; http://dx.doi.org/10.1038/8991; PMID: 10559859
- Kulikov R, Letienne J, Kaur M, Grossman SR, Arts J, Blattner C. Mdm2 facilitates the association of p53 with the proteasome. Proc Natl Acad Sci USA 2010; 107:10038 - 43; http://dx.doi.org/10.1073/pnas.0911716107; PMID: 20479273
- Ugrinova I, Monier K, Ivaldi C, Thiry M, Storck S, Mongelard F, et al. Inactivation of nucleolin leads to nucleolar disruption, cell cycle arrest and defects in centrosome duplication. BMC Mol Biol 2007; 8:66; http://dx.doi.org/10.1186/1471-2199-8-66; PMID: 17692122
- Marshall WF, Rosenbaum JL. Are there nucleic acids in the centrosome?. Curr Top Dev Biol 2000; 49:187 - 205; http://dx.doi.org/10.1016/S0070-2153(99)49009-X; PMID: 11005019
- Alliegro MC, Alliegro MA, Palazzo RE. Centrosome-associated RNA in surf clam oocytes. Proc Natl Acad Sci USA 2006; 103:9034 - 8; http://dx.doi.org/10.1073/pnas.0602859103; PMID: 16754862
- Kingsley EP, Chan XY, Duan Y, Lambert JD. Widespread RNA segregation in a spiralian embryo. Evol Dev 2007; 9:527 - 39; http://dx.doi.org/10.1111/j.1525-142X.2007.00194.x; PMID: 17976050
- Lécuyer E, Yoshida H, Parthasarathy N, Alm C, Babak T, Cerovina T, et al. Global analysis of mRNA localization reveals a prominent role in organizing cellular architecture and function. Cell 2007; 131:174 - 87; http://dx.doi.org/10.1016/j.cell.2007.08.003; PMID: 17923096
- Alliegro MC, Alliegro MA. Differential expression of tyrosinated tubulin in Spisula solidissima polar bodies. Dev Dyn 2005; 232:216 - 20; http://dx.doi.org/10.1002/dvdy.20208; PMID: 15580622
- Alliegro MC, Alliegro MA. Centrosomal RNA correlates with intron-poor nuclear genes in Spisula oocytes. Proc Natl Acad Sci USA 2008; 105:6993 - 7; http://dx.doi.org/10.1073/pnas.0802293105; PMID: 18458332