Abstract
The transactivating response element (TAR) of human immunodeficiency virus 1 (HIV-1) is essential for promoter transactivation by the viral transactivator of transcription (Tat). The Tat-TAR interaction thereby recruits active positive transcription elongation factor b (P-TEFb) from its inactive, 7SK/HEXIM1-bound form, leading to efficient viral transcription. Here, we show that the 7SK RNA-associating chromatin regulator HMGA1 can specifically bind to the HIV-1 TAR element and that 7SK RNA can thereby compete with TAR. The HMGA1-binding interface of TAR is located within the binding site for Tat and other cellular activators, and we further provide evidence for competition between HMGA1 and Tat for TAR-binding. HMGA1 negatively influences the expression of a HIV-1 promoter-driven reporter in a TAR-dependent manner, both in the presence and in the absence of Tat. The overexpression of the HMGA1-binding substructure of 7SK RNA results in a TAR-dependent gain of HIV-1 promoter activity similar to the effect of the shRNA-mediated knockdown of HMGA1. Our results support a model in which the HMGA1/TAR interaction prevents the binding of transcription-activating cellular co-factors and Tat, subsequently leading to reduced HIV-1 transcription.
Introduction
Gene expression of the human immunodeficiency virus 1 (HIV-1) genome is predominantly controlled at the level of transcriptional elongation.Citation1 Viral transcription relies essentially on the host machinery, and the cellular positive transcription elongation factor b (P-TEFb) has been shown to play a key role in regulating HIV genome expression.Citation2-Citation6 Active P-TEFb is composed of the cyclin-dependent kinase 9 (Cdk9) and one of the cyclins T1 (CycT1) or T2.Citation7-Citation9 It phosphorylates the carboxy-terminal domain (CTD) of promoter proximal paused RNA Polymerase II (Pol II) to release the transcriptional block resulting in efficient transcription elongation.Citation7,Citation9 In this way, P-TEFb regulates the expression of the vast majority of cellular Pol II-transcribed genesCitation10-Citation12 and also of the integrated HIV-1 genome in infected cells. P-TEFb activity is negatively regulated by the 7SK non-coding (nc) RNA and the protein HEXIM1, which capture P-TEFb in a small nuclear ribonucleoprotein complex (snRNP) resulting in a strong reduction of Cdk9 kinase activity.Citation13,Citation14 The 7SK snRNP is believed to serve as a scaffold of inactive P-TEFb, from which the active form can be released under stress conditions. One of the mechanisms of such a release involves the bromodomain protein Brd4, which can bind to the active form of P-TEFb to recruit it by interactions with the Mediator complex to cellular promoters.Citation15,Citation16 In the case of the HIV-1 promoter, the viral transactivator of transcription (Tat) interacts with P-TEFb and a RNA structure of the nascent viral transcript , the transactivating response element (TAR), which is thought to eject 7SK ncRNA and HEXIM1 to efficiently enhance viral transcriptional elongation.Citation7,Citation17 Tat/P-TEFb complexes of distinct compositions have been identified, one containing the active form of P-TEFb as well as MLL fusion partners and another one containing the inactive 7SK snRNP and being resistant to cellular stress.Citation18,Citation19 More recent studies have shown that the inactive 7SK RNA-containing P-TEFb complex is present at pre-initiation complexes (PICs) at the HIV-1 core promoter.Citation17 Its recruitment to the viral promoter has been shown to be TAR-independent, but to essentially require the basal transcription factor Sp1.Citation17
Efficient viral replication starts upon Tat-mediated promoter transactivation, but how Tat is produced in the first place is still subject of discussion. However, various host cellular transcription factors have been identified to bind specifically to different regulatory regions within the HIV-1 promoter (reviewed in ref. Citation20). Among those, predominantly Sp1 and NFκb have been shown to play key roles for basal promoter activity.Citation21 Notably, also TAR impacts basal HIV-1 transcription in the absence of Tat. Its specific interaction with the host cellular proteins TAR-binding protein (TARBP) 1 and 2,Citation22,Citation23 YB-1,Citation24 Pur-αCitation25 and RNA helicase ACitation26 has been previously shown to result in an increased viral promoter activity.
We have recently identified the chromatin master regulator HMGA1 as a 7SK RNA-binding partner capable of being also an integral component of the inactive 7SK RNA/P-TEFb complex.Citation12,Citation27 HMGA1 is an architectural transcription factor containing three DNA-binding domains, so-called A/T hooks, which preferentially bind the minor groove of A/T-rich DNA.Citation20 The first, N-terminally located A/T hook of HMGA1, binds specifically to a substructure of 7SK RNA not involved in the formation of 7SK/P-TEFb/Tat complexes.Citation28 HMGA1 has been previously shown to be a host cellular co-factor in the HIV-1 pre-integration complex.Citation29-Citation31 Furthermore, it is involved in HIV-1 splice site regulationCitation32 and the recruitment of SWI/SNF to the HIV-1 LTR.Citation33 Several high- and low-affinity HMGA1-binding sites have been identified within the HIV-1 LTR, some of them directly upstream of the transcription start site, suggesting a role of HMGA1 during HIV-1 transcription.Citation34 Proteomic studies have shown that HMGA1 associates with the cellular TAR RNA-binding protein (TARBP).Citation35 HMGA1 also physically interacts with various transcription factors, among those NFκB and the basal transcription factor Sp1, which can bind a region spanning from the second A/T hook to the linker between the second and third A/T hook of HMGA1.Citation36
Here we set out to investigate whether the highly specific RNA-binding capacity of HMGA1 also allows for HIV-1 TAR recognition. We are able to show a direct interaction between HMGA1 and HIV-1 TAR, which results in diminished HIV-1 transcription, both in the absence and in the presence of Tat.
Results
HMGA1 can specifically bind HIV-1 TAR
HIV-1 TAR is likely able to form similar RNA secondary structures as the 7SK L2 substructure does. More precisely, in both RNAs, the terminal stem-loop structure is followed by a bulge starting by an uracil followed by a guanine (see ). We have previously shown this particular part of 7SK L2 RNA to be critical for HMGA1 binding.Citation12,Citation27 Furthermore, HMGA1 has been previously shown to associate with the TAR RNA-binding protein (TRBP),Citation35 but whether this interaction is direct or RNA-mediated remained elusive in these studies. Thus, we here set out to elucidate whether or not HMGA1 is able to bind HIV-1 TAR directly.
Figure 1. The first A/T-hook of HMGA1 recognizes the apical stem structure of TAR. (A) Predicted secondary structures of 7SK L2 RNA and HIV-1 TAR. The putative HMGA1a-binding motif is labeled in green and the sites of interaction with Tat and Cyc T1 are highlighted in gray. (B) Predicted secondary structures of HIV-1 TAR mutants. The putative HMGA1a-binding site is labeled in green and mutated nucleotides are labeled in red. (C) Fam6-labeled HIV-1 TAR RNA was incubated with immunopurified HMGA1a-FLAG in presence of the 10-fold excess of the non-labeled TAR mutants shown in (B). The resulting complexes were analyzed in EMSAs compared with no competitor (-) and HIV-1 TAR wild-type. (D) Comparison of the predicted tertiary structures of the assumed HMGA1-binding motif of 7SK L2 and HIV-1 TAR. The tertiary structure was calculated using Assemble software.40 Adenine residues are labeled in blue, uracil residues in green, guanine residues in red and cytosine residues in yellow. (E) EMSAs with Fam6-labeled HIV-1 TAR and immunopurified HMGA1a-FLAG mutants. HIV-1 TAR was incubated with equal amounts (upper panel) of immunopurified mutants of each HMGA1a A/T-hook alone (HMGA1a ΔA/T1-3) and the resulting complexes were compared in EMSAs to HMGA1a-FLAG wild-type and the triple mutant HMGA1a 3xΔA/T-FLAG (lower panel).
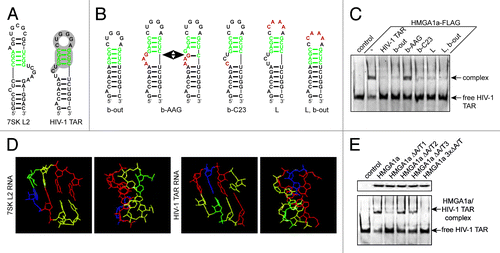
Using electrophoretic mobility shift assays with Fam6-labeled HIV-1 TAR and HMGA1a-FLAG, we were able to show a highly specific TAR-binding activity of HMGA1, which is mediated by the A/T-hook motifs of the protein (, lane 3 vs. lane 1). HMGA1a 3xΔA/T-FLAG, a HMGA1a version carrying point mutations in each A/T-hook leading to diminished DNA-binding,Citation27,Citation37 exhibits a significantly reduced HMGA1-binding activity (, lane 2 vs. lane 1). Both non-labeled 7SK L2 RNA as well as HIV-1 TAR can compete with Fam6-labeled HIV-1 TAR for HMGA1 binding, underlining the specificity of the interaction (, lanes 4–7). Furthermore, the HMGA1/TAR complex can be supershifted using an antibody targeting the HMGA1-FLAG fusion protein (, lane 8). EMSAs using Fam6-labeled 7SK L2 RNA and HMGA1a-FLAG with increasing amounts of non-labeled HIV-1 TAR confirmed that also TAR is able to compete with 7SK L2 RNA for HMGA1 binding (). By microscale thermophoresis (MST) analyses with immuno-purified HMGA1a-FLAG and Fam6-labeled versions of 7SK L2 RNA and HIV-1 TAR, we determined Kd values for HMGA1a with each of these binding partners (). HMGA1a 3xΔA/T-FLAG was used as a control in these experiments. Indeed, the Kds for 7SK L2 RNA and HIV-1 TAR with 213 nM and 198 nM, respectively, turned out to be close.
Figure 2. HMGA1a specifically interacts with HIV-1 TAR. (A) Electrophoretic mobility shift assays with Fam6-labeled HIV-1 TAR and immunopurified HMGA1a-FLAG. The TAR-binding activity of HMGA1a-FLAG (-) was compared with that of a mutant lacking DNA-binding activity (HMGA1a 3xΔA/T-FLAG) and TAR alone (control). Increasing amounts of non-labeled TAR and 7SK L2 RNA compete with labeled TAR for HMGA1a binding. Incubation with anti-FLAG antibody is capable of supershifting the HMGA1a-FLAG/TAR complex. (B) Fam6-labeled 7SK L2 RNA was incubated with immunopurified HMGA1a-FLAG in presence of increasing amounts of non-labeled HIV-1 TAR. The resulting complexes were analyzed in EMSAs. (C) Kd determination of HMGA1a complexes with HIV-1 TAR RNA and 7SK L2 RNA using microscale thermophoresis (MST). Fam6-labeled HIV-1 TAR or 7SK L2 RNA were incubated with increasing concentrations of immunopurified HMGA1a-FLAG compared with HMGA1a 3xΔA/T-FLAG and subsequently subjected to MST.
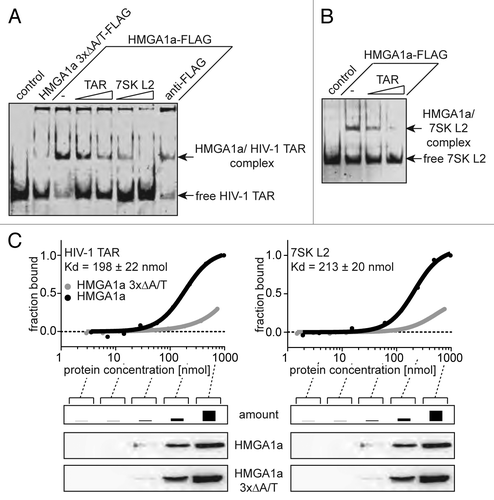
The first A/T-hook of HMGA1 recognizes the apical stem structure of TAR
We next aimed at narrowing down the HMGA1-binding motif on TAR. Previous studies with HMGA1 and 7SK L2 mutants have identified a double-stranded stem structure following a bulge to be the site of interaction with HMGA1Citation27 (, marked in green). A comparison with the secondary structure of HIV-1 TAR gave rise to a stem structure following the HIV-1 Tat-binding bulge as a potential HMGA1 target site. Notably, this site is located within the known binding region for HIV-1 Tat and Cyc T1,Citation38 but also for YB-1 and Pur-αCitation24,Citation25 (, highlighted in gray). Several mutations targeting different structural features within the Tat/Cyc T1-binding site of HIV-1 TAR have been generated in previous studies in order to investigate the HIV-1 Tat-binding regionCitation39 ().
We used EMSAs with Fam6-labeled HIV-1 TAR and HMGA1a-FLAG in presence of the 10-fold excess of non-labeled versions of each of these HIV-1 TAR mutants to analyze the HMGA1a-binding motif (). EMSAs lacking competitor as well as in the presence of a 10-fold excess of non-labeled HIV-1 TAR wild-type served as controls in these experiments, showing that a mutation of the Tat-binding bulge to AAG (, b-AAG) prevents HMGA1 binding. Since a mutant lacking this bulge completely (, b-out) competes with Fam6-labeled HIV-1 TAR wild-type, the bulge itself is unlikely to be directly involved in HMGA1 binding. A shift of a G/C basepairing from one to a neighboring G within the potential HMGA1-binding region of HIV-1 TAR, might serve as an explanation for the loss of HMGA1 binding by the b-AAG mutant (see , b-AAG). Even more likely, a sequence-specific interaction of, for instance, A8 or A9 of the bulge (counted corresponding to the TAR sequence shown in ) with U16 of the loop of this TAR mutant might change the tertiary structure of the HMGA1-binding site and, thus, lead to a loss of HMGA1-binding activity. Such a sequence-specific interaction mediated by a base-triple of A7, U16 and U25 has been previously proven to play a role in TAR tertiary structure formation.Citation40 We calculated the tertiary structures of the assumed HMGA1-binding motifs of both RNAs within the context of the tertiary structure of each RNA substructure, respectively, using Assemble softwareCitation41 (). Both motifs exhibit a helix-like structure, which likely contributes to HMGA1 recognition.
We have previously shown that 7SK RNA is recognized specifically by the first, N-terminally located A/T-hook motif of HMGA1.Citation27 To investigate whether this is the case for HIV-1 TAR as well, we performed EMSAs with Fam6-labeled HIV-1 TAR and point-mutants of each single A/T-hook (HMGA1a ΔA/T1-3) (). Indeed, the mutation in the first A/T-hook results in a loss of HIV-1 TAR-binding activity comparable to that of the triple mutant, while effects of the mutations in the second and third A/T-hook motifs on TAR binding are not measurable. This confirms that the HMGA1/HIV-1 TAR interaction is also mediated by the first A/T-hook motif of the protein.
HMGA1 competes in vitro with HIV-1 Tat for TAR-binding
To investigate whether HIV-1 Tat and HMGA1 are able to bind HIV-1 TAR simultaneously or whether they compete for HIV-1 TAR-binding, we performed EMSAs with Fam6-labeled HIV-1 TAR RNA and immunopurified HMGA1a-FLAG or HIV-1 Tat-FLAG in presence of increasing amounts of the other binding partner (). These EMSAs solely revealed an HMGA1a/HIV-1 TAR complex when consulting the shift of the RNA (, upper panel). HIV-1 Tat has been previously shown to dimerize and Desfosses et al. have identified both Tat monomers as well as Tat dimers to bind HIV-1 TAR.Citation42 Such HIV-1 Tat/HIV-1 TAR complexes, containing Tat monomers as well as Tat dimers, were only visible in western blot analyses of our EMSAs (, middle panel), but not as an RNA shift indicating a stronger affinity of TAR for HMGA1a than for HIV-1 Tat. Note that the HIV-1 Tat-FLAG amounts used in this study were about 5-fold higher than those of HMGA1a-FLAG (data not shown). Western blot analyses using anti-FLAG antibody uncovered that increasing amounts of the HMGA1a-FLAG in presence of HIV-1 Tat-FLAG result in a decrease of HIV-1 Tat monomer/TAR complex and vice versa (, lower panel), pointing at competition of HMGA1 and HIV-1 Tat for HIV-1 TAR binding.
Figure 3. HMGA1 competes in vitro with HIV-1 Tat for TAR-binding. (A) HMGA1 and HIV-1 Tat compete for HIV-1 TAR binding. Fam6-labeled HIV-1 TAR was incubated with immunopurified HMGA1a-FLAG (HIV-1 Tat-FLAG) in presence of increasing amounts of immunopurified HIV-1 Tat-FLAG (HMGA1a-FLAG). The resulting complexes were separated by native PAGE in comparison to HIV-1 TAR RNA or HMGA1a-FLAG and Tat-FLAG alone. The Fam6-labeled RNA was visualized at UV light (upper part, left panel). Protein complexes were subjected to western blot analyses using anti-HIV-1 Tat antibody (middle part, left panel) and anti-FLAG antibody (lower part, left panel). The relative intensities of the signals were plotted using Image J software (right part). (B) Fam6-labeled 7SK L2 RNA was incubated with immunopurified HMGA1a-FLAG or Tat-FLAG and the resulting complexes were analyzed in EMSAs compared with 7SK L2 RNA alone (control). Protein complexes were subjected to western blot analyses using anti-HIV-1 Tat antibody.
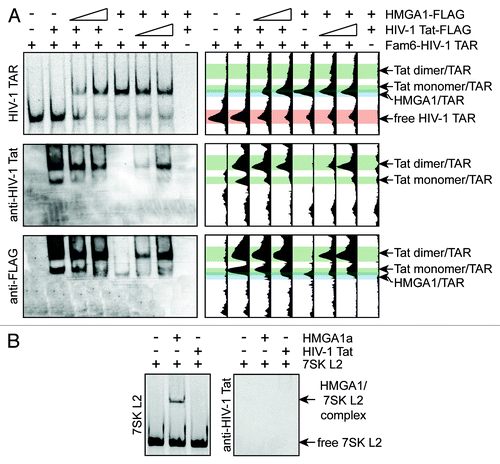
Consistent with previous studies, which have identified a Tat-binding motif in the 5′-stem-loop structure of 7SK RNA to be responsible for the interaction with HIV-1 Tat,Citation43 Tat is not able to bind 7SK L2 RNA as confirmed by EMSAs with Fam6-labeled 7SK L2 RNA and immunopurified Tat-FLAG ().
HMGA1 diminishes HIV-1 transcription in a TAR-dependent manner
In order to assess the influence of the HMGA1/TAR interaction on the HIV-1 promoter activity, we performed promoter activity assays in HeLa cells upon shRNA-mediated knockdown of endogenous HMGA1, overexpression of HMGA1-FLAG or overexpression of the HMGA1-binding L2 substructure of 7SK RNA (). In each condition, we compared the expression of a Renilla luciferase reporter under control of the wild-type HIV-1 LTR in the presence or the absence of HIV-1 Tat with its expression under control of the HIV-1 LTR lacking the sequence from +37 to +78 bp with respect to the transcription start site. Thus, the latter construct is lacking a substancial part of the TAR-coding sequence, which prevents the nascent viral transcript to form the wild-type TAR structure, which allowed us to distinguish between TAR-dependent and -independent effects.
Figure 4. HMGA1 diminishes TAR-dependent HIV-1 transcription. (A) HeLa cells were subjected to a shRNA-mediated knockdown of HMGA1. The relative amount of HMGA1 mRNA was quantified using qantitative RT-PCR. The mRNA amount upon expression of a non-targeting control shRNA was abitrarily set to 1. (B) HMGA1-FLAG fusion protein was overexpressed in HeLa cells compared with mock transfected cells. HMGA1 amounts were quantified by western blot analyses using anti-FLAG and anti-HMGA1 antibodies. Protein amounts were quantified from anti-HMGA1 western blot signals using ImageJ software. (C) The 7SK L2 substructure (EBER2 7SK L2) was overexpressed in HeLa cells and compared with an overexpression of the EBER2 backbone (EBER2-Lb). The expression levels of both constructs were analyzed in quantitiative RT-PCR. β-actin was used as housekeeping gene and the levels of EBER2-Lb RNA were abitrarily set to 1. (D) Cells upon knockdown or overexpression of HMGA1 or overexpression of 7SK L2 (A–C) were either cotransfected with a mock vector or a HIV-1 Tat expression vector. After 24 h, they were transfected with either a wild-type HIV-1 LTR-driven luciferase reporter construct or a TAR-lacking HIV-1 LTR-driven luciferase reporter construct. Another 24 h later, relative promoter activity was assessed by measuring the luciferase activitiy. The promoter activity upon control shRNA, mock transfection and EBER2 backbone expression in the absence of Tat was abitrarily set to 1. (E) The HIV-1 promoter activity in the absence of Tat (D) was correlated with the relative amounts of HMGA1. (F) as in (E), but for the HIV-1 promoter activity in the presence of Tat.
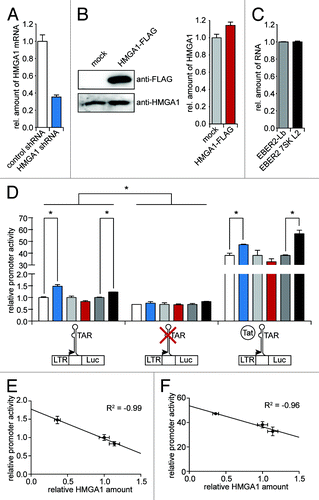
The expression of a validated shRNA targeting HMGA1 mRNACitation27 in HeLa cells leads to a reduction of HMGA1 mRNA levels by approximately 70%, when compared with the expression of a non-targeting control shRNA (). The overexpression of a HMGA1-FLAG fusion protein driven by a CMV promoter results in a approximately 20% increase of the total amount of HMGA1 protein compared with a mock control, as accessed using western blot analyses using an anti-HMGA1 antibody (). To overexpress the 7SK L2 substructure, we utilized the previously described construct EBER2 7SK L2, taking advantage of the strong EBER2 RNA promoter of the Epstein-Barr virus.Citation27 The previously described EBER2-Lb construct, comprising only the EBER2 RNA backbone, was used as a control in these experiments.Citation44 Both RNA chimeras were expressed at similar levels as assessed by quantitative RT-PCR (). In the case of the wild-type HIV-1 LTR-driven reporter in the absence of Tat, the HMGA1 knockdown results in a statistically significant (p < 0.05) increase of reporter expression by about 50% (, left), while the modest overexpression of HMGA1 results in a decrease of promoter activity by approximately 20%. The same tendency as for the HMGA1 knockdown is observable upon overexpression of the 7SK L2 RNA - here the reporter expression is statistically significantly (p < 0.05) increased by about 30% (, left). While the overall promoter activity of the reporter construct driven by the TAR-lacking HIV-1 LTR is statistically significantly (p < 0.05) decreased by about 30% in comparison to the wild-type reporter construct (, middle vs. left), there are only slight and non-significant effects of the HMGA1 knockdown, overexpression and the overexpression of 7SK L2 (, middle). The presence of HIV-1 Tat results in an approximately 35-fold increase of reporter expression from the construct driven by the wild-type HIV-1 LTR, reflecting the strong efficiency of Tat in promoter transactivation (, right vs. left). Also here, the HMGA1 knockdown and the overexpression of 7SK L2 leads to a statistically significantly (p < 0.05) enhanced reporter expression by about 25% and 50%, respectively (, right), while the overexpression of HMGA1-FLAG results in a by approximately 15% decreased promoter activity. We correlated the relative HIV-1 promoter activities of the wild-type reporter construct in the presence and in the absence of Tat with the relative amounts of HMGA1 (), revealing that the HIV-1 promoter activity is strongly negatively correlated with HMGA1 amounts (R2 = -0.96 and -0.99, respectively).
Discussion
A role of HMGA1 during HIV-1 transcription has been suggested previously when several high- and low-affinity HMGA1-binding sites have been identified within the HIV-1 LTR.Citation34 Some of them overlap with known binding sites for basic transcription factors, like the USF-1-binding site, the binding sites for NFκb and the TATA box upstream of the transcription start site as well as an NF-AT and AP-1-binding site downstream of the transcription start site. In the latter case, HMGA1 is able to inhibit binding of the basal AP-1 transcription factor and facilitate the binding of the inducible AP-1 factor ATF-3.Citation34 The DNA-bound ATF-3 can subsequently recruit the chromatin remodeling complex hSWI/SNF, which facilitates transcriptional activation.Citation33 Another HMGA1-binding site within the coding region of HIV-1 TAR has been identified, which does not overlap with recognition motifs of other transcription factors, but rather is located at the dyad of a nucleosome positioned at the 5′ UTR.Citation34 HMGA1 has been shown to also interact with such DNA-binding sites located within an inhibitory nucleosome at the human IL-2Rα promoter.Citation45 Here, the HMGA1-mediated nucleosome remodeling results in IL-2Rα transcription activation.Citation45,Citation46 Also, direct interactions of HMGA1 with NFκB and the basic transcription factor Sp1 have been reported.Citation36,Citation47 High-affinity binding sites for both transcription factors have been identified within the HIV-1 core promoterCitation48,Citation49 and ChIP analyses have revealed that the Sp1 site of the HIV-1 core promoter is occupied with inactive, HEXIM1-containing P-TEFb complexes, even in the absence of HIV-1 Tat and TAR in an RNA-dependent manner.Citation17 Furthermore, the deletion of the Sp1-binding site in the HIV-1 core promoter eliminated these interactions, suggesting that both Sp1 and the 7SK/P-TEFb snRNP contribute to the formation of Tat-responsive complexes.Citation17
Here, we show a highly specific interaction of HMGA1 with the HIV-1 transactivating response element TAR, which is mediated by the same site of the protein as the interaction with 7SK RNA.Citation27 The putative HMGA1-binding motif within HIV-1 TAR is located within the region where the transcriptional activators HIV-1 Tat, Cyclin T1Citation38,Citation50 as well as YB-1 and Pur-αCitation24,Citation25 have been shown to bind. The latter two host cellular factors are able to enhance HIV-1 transcription both in the presence and in the absence of the viral transactivator Tat (24,25). The interaction of HMGA1 with TAR represses basal viral transcription as it does in the presence of Tat, since a LTR-driven reporter lacking the TAR substructure does not respond to a HMGA1 knockdown (). Notably, HeLa cells, which have been used in these reporter assays, have been previously shown to lack ATF-3 expression unless activated by external stimuli.Citation51 Thus, in this system, the transcription-activating HMGA1-mediated recruitment of the hSWI/SNF complex, which occurs via the inducible ATF-3, is not observable, allowing for a detailed analysis of the role of the HMGA1/TAR interaction. Even though an effect of the lacking HMGA1-binding site located within the TAR-coding sequence of the utilized TAR-lacking reporter construct cannot be ruled out completely, a transcription repressive function of this binding site is rather unlikely. As mentioned above, the binding of HMGA1 to DNA sequences located at nucleosomes is thought to remodel the chromatin structure and, thus, rather activate than repress transcription.
Nevertheless, we show here a direct competition of HMGA1a with Tat for TAR-binding (). Since the interaction between Tat and TAR is essential for the P-TEFb-dependent transactivation of the HIV-1 promoter,Citation6,Citation8,Citation52 TAR-bound HMGA1 likely prevents the Tat/TAR interaction, resulting in a diminished HIV-1 transcription. 7SK RNA, as a part of the inactive P-TEFb snRNP, can compete with with TAR for HMGA1 binding, leading to enhanced HIV-1 transcription (). Indeed, recent studies have identified inactive, 7SK RNA-associated P-TEFb to be present at the HIV-1 core promoter.Citation17 Besides being a promoter proximal source for P-TEFb when targeted by Tat, the subsequently released 7SK RNA might then also competitively facilitate the release of HMGA1 from TAR in order to enhance Tat-mediated transactivation (). Note that the overexpressed 7SK L2 substructure does not directly interfere with the Tat/TAR interaction, as it is not capable of Tat-binding ().
Figure 5. Model of the HMGA1-repressive role during HIV-1 transcription. (A) TAR-bound HMGA1 competes with HIV-1 Tat for TAR-binding. Tat targets P-TEFb from the promoter-bound inactive 7SK/P-TEFb snRNP, leading to the ejection of inactivating HEXIM1 and 7SK RNA, which subsequently competes with TAR for HMGA1 binding. That way, 7SK RNA facilitates the Tat/TAR interaction leading to the recruitment of activated P-TEFb to the paused RNA Polymerase II (RNA Pol II) to subsequently start efficient viral transcription. (B) In the absence of Tat, TAR-bound HMGA1 prevents the binding of transcription activating cellular co-factors to TAR (e.g., YB-1 or Pur-α). 7SK RNA competes with TAR vor HMGA1 binding and that way facilitates the TAR-binding of the cellular co-factors in order to activate basal viral transcription.
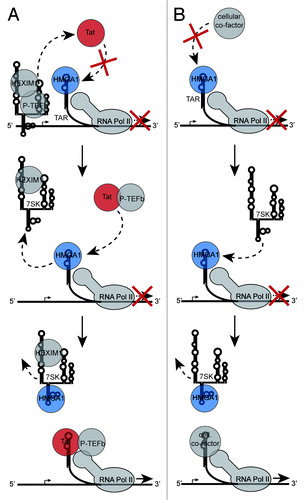
Besides the TAR-dependent effect on HIV-1 transcription in the presence of Tat, the HMGA1/TAR interaction also diminishes basal HIV-1 promoter activity (, left), suggesting that HMGA1 might also compete with activating TAR-binding host cellular factors (). As mentioned above, several such factors have been identified previously binding to the same TAR-region as HIV-1 Tat does. The member of the Y-box protein family YB-1 specifically interacts with HIV-1 TAR and activates basal viral transcription in a concentration-dependent manner.Citation24 Furthermore, the transcription factor Pur-α is able to bind TAR and, besides a synergistic transcriptional activation with Tat, is also able to increase HIV-1 transcription in a Tat-independent fashion.Citation25 The first host cellular TAR interacting protein identified was the TAR-binding protein (TARBP).Citation22,Citation23 TARBP activates viral gene expression by at least two different mechanisms: it represses the activity of the HIV-1 translation-inhibitory protein kinase PKR via a direct interactionCitation53 and it also increases viral translation by a direct interaction with HIV-1 TAR.Citation54 Indeed, HMGA1 has been identified to associate with TARBP in previous studies,Citation35 but the role of this interaction still remains elusive and needs to be addressed in future research.
Besides its important function during the Tat-mediated recruitment of P-TEFb, HIV-1 TAR has recently also been shown to be involved in HIV-1 premature transcription termination in the absence of Tat.Citation55 In this case, Microprocessor, composed of the RNase III Drosha and the dsRNA-binding protein Dgcr8, is recruited to the TAR substructure, leading to the Drosha-mediated cleavage of the transcript. Thereby, a non-adenylated TAR RNA, as well as an uncapped 3′ transcript is generated. The latter product subsequently recruits the termination factor Xrn2, leading to mRNA degradation, while the 3′-end of TAR recruits Rrp6, which processes the RNA to generate transcription-repressive small RNAs. Since this mechanism acts transcription-repressive in the absence of Tat, a potential involvement of HMGA1 in the recruitment of Rrp6 to HIV-1 TAR would be another explaination for the HMGA1-mediated repression of HIV-1 promoter activity in Tat’s absence. However, the proof of such an involvement will need further investigations.
Taken together, our data show a highly specific interaction of the chromatin master regulator HMGA1 with the HIV-1 transactivating response element TAR, which results in a significant reduction of viral promoter activity both in the presence and in the absence of the viral transactivator Tat. HMGA1 is able to directly compete with Tat for TAR-binding and, thus, our findings support a model in which HMGA1 competitively inhibits the binding of transcription-activating factors to HIV-1 TAR, subsequently leading to repressed viral gene expression. That way HMGA1 might be a host cellular factor involved in the establishment of viral latency.
Materials and Methods
Culturing conditions, transfections and extract preparations
HEK293 and HeLa cells were cultured as described previously.Citation27 Transfections were performed using FugeneHD (Roche) according to the manufacturer’s instructions. The preparation cellular extracts was performed as described previously.Citation27
Western blot analyses and antibodies
SDS-PAGE was performed as described previously.Citation56 Antibodies used in western blot analyses were M2 anti-FLAG antibody (Sigma), anti-HMGA1 antibody, anti-HIV-1 Tat antibody (Thermo Fisher Scientific) and anti-β-actin antibody (Santa Cruz Biotechnology) with the corresponding secondary HRP-coupled antibodies (Sigma) as recommended by the manufacturer.
Immunoprecipitations
To obtain a vector for the expression of FLAG fusion proteins, the hybridized oligonucleotides 5′-CTA GAG GGC GAC TAC AAA GAC GAT GAC GAC AAA GGA TGA A-3′ (sense) and 3′-TC CCG CTG ATG TTT CTG CTA CTG CTG TTT CCT ACT TGG CC-5′ (antisense) were inserted into the XbaI and AgeI restriction sites of the vector pcDNA4/TO/myc-his B (Invitrogen). For Tat-FLAG, the coding sequence of HIV-1 Tat from +1 to +264 nt was inserted into the BamHI and XbaI restriction sites of the vector mentioned above. The HMGA1a-FLAG fusion protein was cloned as described previously.Citation27
Immunoprecipitations of FLAG fusion proteins were performed with M2 anti-FLAG agarose (Sigma) as described previously.Citation27 To remove cellular binding partners of HIV-1 Tat and HMGA1 before usage in competitive EMSAs the FLAG fusion proteins bound to anti-FLAG agarose were washed with IP-washing buffer (20 mM HEPES/KOH, pH 7.9, 100 mM KCl, 0.2 mM EDTA, 0,025% Tween 20) containing 500 mM KCl. To restore buffer conditions, two subsequent washing steps with IP-washing buffer were performed before eluting the FLAG fusion proteins by incubation with IP-buffer (20 mM HEPES/KOH, pH 7.9, 100 mM KCl, 0.2 mM EDTA, 20% glycerol) containing an excess of FLAG peptide for 30 min at 4°C.
Electrophoretic mobility shift assays (EMSAs)
For electrophoretic mobility shift assays (EMSAs), 100–200 ng of Fam6-labeled RNA was denatured for 5 min at 65°C and renatured by cooling slowly down to room temperature. The RNA was incubated with immunopurified FLAG fusion protein in EMSA-buffer (10 mM HEPES/KOH, pH 7.9, 100 mM KCl, 5 mM MgCl2, 0.25 mM DTT, 0.1 mM EDTA, 10% glycerol, 10 U RNase Inhibitor and 2 µg yeast tRNA per lane) for 10 min on ice and subsequently for 10 min at 37°C. The resulting complexes were separated by 7.5% or 10% native PAGE and visualized at UV-light. The sequence of the Fam6-labeled HIV-1 TAR element used for EMSAs was 5′-GGG UCU CUC UGG UUA GAC CAG AUC UGA GCC UGG GAG CUC UCU GGC UAA CUA GGG AAC CC-3′, the one of the 7SK L2 RNA was 5′-GGG CGU CCC UCC CGA AGC UGC GCG CUC GGU CGA AGA GGA CGA CCA UCC CCG AUA GAG GAG GAC-3′.
Micro scale thermophoresis (MST)
Immunopurified HMGA1a-FLAG or HMGA1a 3xΔA/T-FLAG were diluted with IP-buffer. For MST experiments, a series of 10 successive dilutions was used ranging from 1:0 to 1:256. The concentration of the non-diluted proteins was measured using Nanodrop technology as recommended by the manufacturer. Five µl of each dilution were incubated with 60 ng of Fam6-labeled RNA for 10 min on ice in EMSA buffer. MST experiments were conducted using a Monolith NT.115 (Nanotemper Technologies) as described previouslyCitation57,Citation58 (Laser-power 12%, Laser-on time 60s, LED-power 30%).
In vitro transcription and RNA structure prediction
In vitro transcription was performed using the corresponding templates under control of the promoter of bacteriophage T7 with the High Yield T7 Transcription Kit (Fermentas) as recommended by the manufacturer. The secondary structures of RNAs were calculated by RNAstructure (Version 4.4).Citation59 RNA tertiary structures were calculated using Assemble software (Version 1.04).Citation40
shRNA knockdown of HMGA1
The expression of endogenous HMGA1a was knocked down using specific, HMGA1a mRNA-targeting shRNA as described previously.Citation27
7SK L2 overexpression
For the overexpression of the 7SK L2 substructure under control of the strong viral EBER2 promoter of Epstein-Barr virus we utilized the vector EBER2 7SK L2.Citation27,Citation44
Total RNA preparation and quantitative RT-PCR analyses
Total RNA from transfected or non-transfected cells was prepared using the RNeasy Midi Kit (Qiagen) as recommended by the manufacturer. qRT-PCR analyses were performed using SuperScript™ 2 Reverse Transcriptase (Invitrogen), Lightcycler® (Roche) and Fast Start DNA MasterPLUS SYBR Green I reaction mix (Roche) as recommended by the manufacturers. Primers used to quantify the mRNA levels of the housekeeping gene β-actin were 5′-TCT TCC AGC CTT CCT TCC TG-3′ (sense) and 5′-CAC GGA GTA CTT GCG CTC AG-3′ (antisense). Primers used to quantify mRNA levels of HMGA1 were 5′-TCC CAG CCA TCA CTC TTC-3′ (sense) and 5′-CTC CTT CTG ACT CCC TAC C-3′ (antisense). Primers for the quantification of the EBER2 chimeras were 5′-CCG TTG CCC TAG TGG TTT C-3′ (sense) and 5′-ACA GCG GAC AAG CCG AAT A-3′ (antisense.).
HIV-1 promoter-driven reporter assays
The wild-type LTR-driven Renilla luciferase reporter construct has been described previously.Citation60 To obtain the reporter plasmid lacking intact TAR, the latter wild-type reporter construct was digested with SacI and HindIII, the restriction sites were filled up by Klenow fragment and the vector was religated, resulting in the lack of 42 bp from +37 to +78 with respect to the transcription start site. Twenty-four hours post-co-transfection of HMGA1 shRNA, control shRNA, EBER2 7SK L2 or EBER2-Lb with either mock vector or a vector expressing HIV-1 Tat under control of the CMV promoter, HeLa cells were transfected with the HIV-1 wt LTR or the HIV-1 -TAR LTR reporter plasmid. After 24 h, cells were washed with PBS and lysed using the Renilla Luciferase Assay System (Promega) as recommended by the manufacturer. Luciferase activity was subsequently measured using GloMax®-96 Microplate Luminometer (Promega) according to the manufacturer’s instructions and normalized by protein amounts.
Acknowledgments
S.E. is recipient of a post-doctoral fellowship of the Agence Nationale de recherches sur le SIDA et les hépatites virales (ANRS). This work was furthermore made possible through funding to A.B. by the Agence Nationale pour la Recherche (ANR ISPA2007) and the Genopole Evry.
Disclosure of Potential Conflicts of Interest
No potential conflicts of interest were disclosed.
References
- Kao SY, Calman AF, Luciw PA, Peterlin BM. Anti-termination of transcription within the long terminal repeat of HIV-1 by tat gene product. Nature 1987; 330:489 - 93; http://dx.doi.org/10.1038/330489a0; PMID: 2825027
- Herrmann CH, Gold MO, Rice AP. Viral transactivators specifically target distinct cellular protein kinases that phosphorylate the RNA polymerase II C-terminal domain. Nucleic Acids Res 1996; 24:501 - 8; http://dx.doi.org/10.1093/nar/24.3.501; PMID: 8602364
- Herrmann CH, Rice AP. Lentivirus Tat proteins specifically associate with a cellular protein kinase, TAK, that hyperphosphorylates the carboxyl-terminal domain of the large subunit of RNA polymerase II: candidate for a Tat cofactor. J Virol 1995; 69:1612 - 20; PMID: 7853496
- Marshall NF, Peng J, Xie Z, Price DH. Control of RNA polymerase II elongation potential by a novel carboxyl-terminal domain kinase. J Biol Chem 1996; 271:27176 - 83; http://dx.doi.org/10.1074/jbc.271.43.27176; PMID: 8900211
- Marshall NF, Price DH. Purification of P-TEFb, a transcription factor required for the transition into productive elongation. J Biol Chem 1995; 270:12335 - 8; http://dx.doi.org/10.1074/jbc.270.21.12335; PMID: 7759473
- Zhu Y, Pe’ery T, Peng J, Ramanathan Y, Marshall N, Marshall T, et al. Transcription elongation factor P-TEFb is required for HIV-1 tat transactivation in vitro. Genes Dev 1997; 11:2622 - 32; http://dx.doi.org/10.1101/gad.11.20.2622; PMID: 9334325
- Peterlin BM, Price DH. Controlling the elongation phase of transcription with P-TEFb. Mol Cell 2006; 23:297 - 305; http://dx.doi.org/10.1016/j.molcel.2006.06.014; PMID: 16885020
- Wei P, Garber ME, Fang SM, Fischer WH, Jones KA. A novel CDK9-associated C-type cyclin interacts directly with HIV-1 Tat and mediates its high-affinity, loop-specific binding to TAR RNA. Cell 1998; 92:451 - 62; http://dx.doi.org/10.1016/S0092-8674(00)80939-3; PMID: 9491887
- Zhou Q, Yik JH. The Yin and Yang of P-TEFb regulation: implications for human immunodeficiency virus gene expression and global control of cell growth and differentiation. Microbiol Mol Biol Rev 2006; 70:646 - 59; http://dx.doi.org/10.1128/MMBR.00011-06; PMID: 16959964
- Chao SH, Price DH. Flavopiridol inactivates P-TEFb and blocks most RNA polymerase II transcription in vivo. J Biol Chem 2001; 276:31793 - 9; http://dx.doi.org/10.1074/jbc.M102306200; PMID: 11431468
- Rahl PB, Lin CY, Seila AC, Flynn RA, McCuine S, Burge CB, et al. c-Myc regulates transcriptional pause release. Cell 2010; 141:432 - 45; http://dx.doi.org/10.1016/j.cell.2010.03.030; PMID: 20434984
- Eilebrecht S, Benecke BJ, Benecke A. 7SK snRNA-mediated, gene-specific cooperativity of HMGA1 and P-TEFb. RNA Biol 2011; 8:1084 - 93; http://dx.doi.org/10.4161/rna.8.6.17015; PMID: 21957495
- Nguyen VT, Kiss T, Michels AA, Bensaude O. 7SK small nuclear RNA binds to and inhibits the activity of CDK9/cyclin T complexes. Nature 2001; 414:322 - 5; http://dx.doi.org/10.1038/35104581; PMID: 11713533
- Yang Z, Zhu Q, Luo K, Zhou Q. The 7SK small nuclear RNA inhibits the CDK9/cyclin T1 kinase to control transcription. Nature 2001; 414:317 - 22; http://dx.doi.org/10.1038/35104575; PMID: 11713532
- Jang MK, Mochizuki K, Zhou M, Jeong HS, Brady JN, Ozato K. The bromodomain protein Brd4 is a positive regulatory component of P-TEFb and stimulates RNA polymerase II-dependent transcription. Mol Cell 2005; 19:523 - 34; http://dx.doi.org/10.1016/j.molcel.2005.06.027; PMID: 16109376
- Yang Z, Yik JH, Chen R, He N, Jang MK, Ozato K, et al. Recruitment of P-TEFb for stimulation of transcriptional elongation by the bromodomain protein Brd4. Mol Cell 2005; 19:535 - 45; http://dx.doi.org/10.1016/j.molcel.2005.06.029; PMID: 16109377
- D’Orso I, Frankel AD. RNA-mediated displacement of an inhibitory snRNP complex activates transcription elongation. Nat Struct Mol Biol 2010; 17:815 - 21; http://dx.doi.org/10.1038/nsmb.1827; PMID: 20562857
- He N, Liu M, Hsu J, Xue Y, Chou S, Burlingame A, et al. HIV-1 Tat and host AFF4 recruit two transcription elongation factors into a bifunctional complex for coordinated activation of HIV-1 transcription. Mol Cell 2010; 38:428 - 38; http://dx.doi.org/10.1016/j.molcel.2010.04.013; PMID: 20471948
- Sobhian B, Laguette N, Yatim A, Nakamura M, Levy Y, Kiernan R, et al. HIV-1 Tat assembles a multifunctional transcription elongation complex and stably associates with the 7SK snRNP. Mol Cell 2010; 38:439 - 51; http://dx.doi.org/10.1016/j.molcel.2010.04.012; PMID: 20471949
- Pereira LA, Bentley K, Peeters A, Churchill MJ, Deacon NJ. A compilation of cellular transcription factor interactions with the HIV-1 LTR promoter. Nucleic Acids Res 2000; 28:663 - 8; http://dx.doi.org/10.1093/nar/28.3.663; PMID: 10637316
- Gaynor R. Cellular transcription factors involved in the regulation of HIV-1 gene expression. AIDS 1992; 6:347 - 63; http://dx.doi.org/10.1097/00002030-199204000-00001; PMID: 1616633
- Gatignol A, Buckler-White A, Berkhout B, Jeang KT. Characterization of a human TAR RNA-binding protein that activates the HIV-1 LTR. Science 1991; 251:1597 - 600; http://dx.doi.org/10.1126/science.2011739; PMID: 2011739
- Gatignol A, Duarte M, Daviet L, Chang YN, Jeang KT. Sequential steps in Tat trans-activation of HIV-1 mediated through cellular DNA, RNA, and protein binding factors. Gene Expr 1996; 5:217 - 28; PMID: 8723388
- Ansari SA, Safak M, Gallia GL, Sawaya BE, Amini S, Khalili K. Interaction of YB-1 with human immunodeficiency virus type 1 Tat and TAR RNA modulates viral promoter activity. J Gen Virol 1999; 80:2629 - 38; PMID: 10573156
- Chepenik LG, Tretiakova AP, Krachmarov CP, Johnson EM, Khalili K. The single-stranded DNA binding protein, Pur-alpha, binds HIV-1 TAR RNA and activates HIV-1 transcription. Gene 1998; 210:37 - 44; http://dx.doi.org/10.1016/S0378-1119(98)00033-X; PMID: 9524214
- Fujii R, Okamoto M, Aratani S, Oishi T, Ohshima T, Taira K, et al. A Role of RNA Helicase A in cis-Acting Transactivation Response Element-mediated Transcriptional Regulation of Human Immunodeficiency Virus Type 1. J Biol Chem 2001; 276:5445 - 51; http://dx.doi.org/10.1074/jbc.M006892200; PMID: 11096080
- Eilebrecht S, Brysbaert G, Wegert T, Urlaub H, Benecke BJ, Benecke A. 7SK small nuclear RNA directly affects HMGA1 function in transcription regulation. Nucleic Acids Res 2011; 39:2057 - 72; http://dx.doi.org/10.1093/nar/gkq1153; PMID: 21087998
- Reeves R, Nissen MS. The A.T-DNA-binding domain of mammalian high mobility group I chromosomal proteins. A novel peptide motif for recognizing DNA structure. J Biol Chem 1990; 265:8573 - 82; PMID: 1692833
- Farnet CM, Bushman FD. HIV-1 cDNA integration: requirement of HMG I(Y) protein for function of preintegration complexes in vitro. Cell 1997; 88:483 - 92; http://dx.doi.org/10.1016/S0092-8674(00)81888-7; PMID: 9038339
- Gao K, Gorelick RJ, Johnson DG, Bushman F. Cofactors for human immunodeficiency virus type 1 cDNA integration in vitro. J Virol 2003; 77:1598 - 603; http://dx.doi.org/10.1128/JVI.77.2.1598-1603.2003; PMID: 12502875
- Van Maele B, Busschots K, Vandekerckhove L, Christ F, Debyser Z. Cellular co-factors of HIV-1 integration. Trends Biochem Sci 2006; 31:98 - 105; http://dx.doi.org/10.1016/j.tibs.2005.12.002; PMID: 16403635
- Tsuruno C, Ohe K, Kuramitsu M, Kohma T, Takahama Y, Hamaguchi Y, et al. HMGA1a is involved in specific splice site regulation of human immunodeficiency virus type 1. Biochem Biophys Res Commun 2011; 406:512 - 7; http://dx.doi.org/10.1016/j.bbrc.2011.02.059; PMID: 21329653
- Henderson A, Holloway A, Reeves R, Tremethick DJ. Recruitment of SWI/SNF to the human immunodeficiency virus type 1 promoter. Mol Cell Biol 2004; 24:389 - 97; http://dx.doi.org/10.1128/MCB.24.1.389-397.2004; PMID: 14673171
- Henderson A, Bunce M, Siddon N, Reeves R, Tremethick DJ. High-mobility-group protein I can modulate binding of transcription factors to the U5 region of the human immunodeficiency virus type 1 proviral promoter. J Virol 2000; 74:10523 - 34; http://dx.doi.org/10.1128/JVI.74.22.10523-10534.2000; PMID: 11044097
- Chi YH, Semmes OJ, Jeang KT. A proteomic study of TAR-RNA binding protein (TRBP)-associated factors. Cell Biosci 2011; 1:9; http://dx.doi.org/10.1186/2045-3701-1-9; PMID: 21711701
- Foti D, Iuliano R, Chiefari E, Brunetti A. A nucleoprotein complex containing Sp1, C/EBP beta, and HMGI-Y controls human insulin receptor gene transcription. Mol Cell Biol 2003; 23:2720 - 32; http://dx.doi.org/10.1128/MCB.23.8.2720-2732.2003; PMID: 12665574
- Harrer M, Lührs H, Bustin M, Scheer U, Hock R. Dynamic interaction of HMGA1a proteins with chromatin. J Cell Sci 2004; 117:3459 - 71; http://dx.doi.org/10.1242/jcs.01160; PMID: 15213251
- Wei P, Garber ME, Fang SM, Fischer WH, Jones KA. A novel CDK9-associated C-type cyclin interacts directly with HIV-1 Tat and mediates its high-affinity, loop-specific binding to TAR RNA. Cell 1998; 92:451 - 62; http://dx.doi.org/10.1016/S0092-8674(00)80939-3; PMID: 9491887
- Berkhout B, Jeang KT. trans activation of human immunodeficiency virus type 1 is sequence specific for both the single-stranded bulge and loop of the trans-acting-responsive hairpin: a quantitative analysis. J Virol 1989; 63:5501 - 4; PMID: 2479775
- Huthoff H, Girard F, Wijmenga SS, Berkhout B. Evidence for a base triple in the free HIV-1 TAR RNA. RNA 2004; 10:412 - 23; http://dx.doi.org/10.1261/rna.5161304; PMID: 14970387
- Jossinet F, Ludwig TE, Westhof E. Assemble: an interactive graphical tool to analyze and build RNA architectures at the 2D and 3D levels. Bioinformatics 2010; 26:2057 - 9; http://dx.doi.org/10.1093/bioinformatics/btq321; PMID: 20562414
- Desfosses Y, Solis M, Sun Q, Grandvaux N, Van Lint C, Burny A, et al. Regulation of human immunodeficiency virus type 1 gene expression by clade-specific Tat proteins. J Virol 2005; 79:9180 - 91; http://dx.doi.org/10.1128/JVI.79.14.9180-9191.2005; PMID: 15994812
- Muniz L, Egloff S, Ughy B, Jády BE, Kiss T. Controlling cellular P-TEFb activity by the HIV-1 transcriptional transactivator Tat. PLoS Pathog 2010; 6:e1001152; http://dx.doi.org/10.1371/journal.ppat.1001152; PMID: 20976203
- Eilebrecht S, Pellay FX, Odenwälder P, Brysbaert G, Benecke BJ, Benecke A. EBER2 RNA-induced transcriptome changes identify cellular processes likely targeted during Epstein Barr Virus infection. BMC Res Notes 2008; 1:100; http://dx.doi.org/10.1186/1756-0500-1-100; PMID: 18957101
- Reeves R, Leonard WJ, Nissen MS. Binding of HMG-I(Y) imparts architectural specificity to a positioned nucleosome on the promoter of the human interleukin-2 receptor alpha gene. Mol Cell Biol 2000; 20:4666 - 79; http://dx.doi.org/10.1128/MCB.20.13.4666-4679.2000; PMID: 10848593
- John S, Reeves RB, Lin JX, Child R, Leiden JM, Thompson CB, et al. Regulation of cell-type-specific interleukin-2 receptor alpha-chain gene expression: potential role of physical interactions between Elf-1, HMG-I(Y), and NF-kappa B family proteins. Mol Cell Biol 1995; 15:1786 - 96; PMID: 7862168
- Zhang XM, Verdine GL. A small region in HMG I(Y) is critical for cooperation with NF-kappaB on DNA. J Biol Chem 1999; 274:20235 - 43; http://dx.doi.org/10.1074/jbc.274.29.20235; PMID: 10400641
- Kretzschmar M, Meisterernst M, Scheidereit C, Li G, Roeder RG. Transcriptional regulation of the HIV-1 promoter by NF-kappa B in vitro. Genes Dev 1992; 6:761 - 74; http://dx.doi.org/10.1101/gad.6.5.761; PMID: 1577271
- Harrich D, Garcia J, Wu F, Mitsuyasu R, Gonazalez J, Gaynor R. Role of SP1-binding domains in in vivo transcriptional regulation of the human immunodeficiency virus type 1 long terminal repeat. J Virol 1989; 63:2585 - 91; PMID: 2657100
- Sedore SC, Byers SA, Biglione S, Price JP, Maury WJ, Price DH. Manipulation of P-TEFb control machinery by HIV: recruitment of P-TEFb from the large form by Tat and binding of HEXIM1 to TAR. Nucleic Acids Res 2007; 35:4347 - 58; http://dx.doi.org/10.1093/nar/gkm443; PMID: 17576689
- Mashima T, Udagawa S, Tsuruo T. Involvement of transcriptional repressor ATF3 in acceleration of caspase protease activation during DNA damaging agent-induced apoptosis. J Cell Physiol 2001; 188:352 - 8; http://dx.doi.org/10.1002/jcp.1130; PMID: 11473362
- Mancebo HS, Lee G, Flygare J, Tomassini J, Luu P, Zhu Y, et al. P-TEFb kinase is required for HIV Tat transcriptional activation in vivo and in vitro. Genes Dev 1997; 11:2633 - 44; http://dx.doi.org/10.1101/gad.11.20.2633; PMID: 9334326
- Sanghvi VR, Steel LF. The cellular TAR RNA binding protein, TRBP, promotes HIV-1 replication primarily by inhibiting the activation of double-stranded RNA-dependent kinase PKR. J Virol 2011; 85:12614 - 21; http://dx.doi.org/10.1128/JVI.05240-11; PMID: 21937648
- Dorin D, Bonnet MC, Bannwarth S, Gatignol A, Meurs EF, Vaquero C. The TAR RNA-binding protein, TRBP, stimulates the expression of TAR-containing RNAs in vitro and in vivo independently of its ability to inhibit the dsRNA-dependent kinase PKR. J Biol Chem 2003; 278:4440 - 8; http://dx.doi.org/10.1074/jbc.M208954200; PMID: 12475984
- Wagschal A, Rousset E, Basavarajaiah P, Contreras X, Harwig A, Laurent-Chabalier S, et al. Microprocessor, Setx, Xrn2, and Rrp6 co-operate to induce premature termination of transcription by RNAPII. Cell 2012; 150:1147 - 57; http://dx.doi.org/10.1016/j.cell.2012.08.004; PMID: 22980978
- Laemmli UK. Cleavage of structural proteins during the assembly of the head of bacteriophage T4. Nature 1970; 227:680 - 5; http://dx.doi.org/10.1038/227680a0; PMID: 5432063
- Wienken CJ, Baaske P, Rothbauer U, Braun D, Duhr S. Protein-binding assays in biological liquids using microscale thermophoresis. Nat Commun 2010; 1:100; http://dx.doi.org/10.1038/ncomms1093; PMID: 20981028
- Wienken CJ, Baaske P, Duhr S, Braun D. Thermophoretic melting curves quantify the conformation and stability of RNA and DNA. Nucleic Acids Res 2011; 39:e52; http://dx.doi.org/10.1093/nar/gkr035; PMID: 21297115
- Zuker M. Computer prediction of RNA structure. Methods Enzymol 1989; 180:262 - 88; http://dx.doi.org/10.1016/0076-6879(89)80106-5; PMID: 2482418
- Wilhelm E, Doyle MC, Nzaramba I, Magdzinski A, Dumais N, Bell B. CTGC motifs within the HIV core promoter specify Tat-responsive pre-initiation complexes. Retrovirology 2012; 9:62; http://dx.doi.org/10.1186/1742-4690-9-62; PMID: 22834489