Abstract
Small regulatory RNAs (sRNAs) are influential post-transcriptional modulators of gene expression in bacteria. They regulate gene expression by base pairing to target mRNAs, leading to inhibition of translation and/or alteration of mRNA stability. Recently, several sRNAs have been discovered to regulate genes encoded in operons. In some cases, these sRNAs regulate all the genes encoded by the polycistronic mRNA (coordinate regulation) while in other cases, only a select subset of cistrons is controlled by the sRNA (discoordinate regulation). In this point of view, mechanisms of regulation and characteristics of sRNA-mRNA interactions involving polycistronic mRNAs are described. The diversity in mechanisms represented by these few characterized examples suggests that we still have much to learn about sRNA regulation of long polycistronic messages.
Introduction
Small non-coding RNAs are an important class of post-transcriptional regulators and are found in organisms from all three domains of life. Bacterial small RNAs (sRNAs) range in size from 50–400 nucleotides and regulate many aspects of bacterial physiology, including motility,Citation1 virulenceCitation2-Citation4 and responses to specific stresses such as iron starvation,Citation5 membrane stressCitation6,Citation7 and sugar-phosphate stress.Citation8,Citation9 Many sRNAs alter gene expression via base pairing interactions with sequences in target mRNAs, leading to positive or negative regulation of target protein synthesis. To form productive interactions with target mRNAs, most sRNAs require the RNA chaperone Hfq.Citation10 Hfq is necessary for stability of many sRNAs and, in most cases, facilitates sRNA-mRNA pairingCitation11 (also reviewed in refs. Citation12 and Citation13). The canonical mechanisms and outcomes of sRNA-mediated regulation of gene expression are illustrated in . Activation of target mRNA translation is typically achieved by sRNA base pairing to sequences in the target mRNA secondary structure that otherwise sequester the ribosome binding site (RBS) and obstruct ribosome loading. By preventing the formation of such inhibitory structures, sRNAs increase translation of target proteins ().Citation14,Citation15
Figure 1. Base pairing interactions and regulatory outcomes. Small RNAs (sRNAs) are indicated by blue arrows and mRNAs (mRNAs) by red arrows. The ribosome-binding sites (RBS) of the targets are denoted by the orange box. Overlapping green circles indicate ribosomes. Dotted arrows denote ribosomes unable to load on the target mRNA. sRNA-mRNA base pairing interactions are shown by a series of thin, black lines. RNase E and the degradosome is represented by black, wedged circles. sRNAs can (A) positively regulate targets by base pairing to secondary structures and unmasking the RBS, allowing translation. They can also regulate targets in a negative manner by (B) inhibition of translation and stimulation of degradation (C) only inhibiting translation or (D) only stimulating degradation.
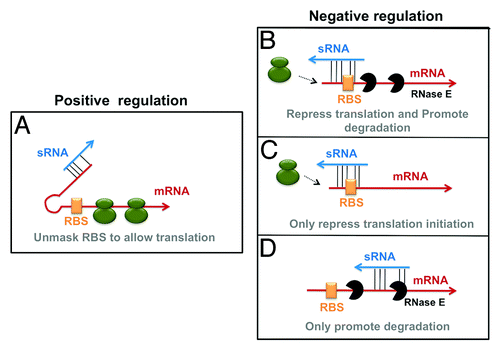
Repression of target mRNAs can be accomplished by inhibiting translation and/or by promoting degradation. sRNAs can repress translation initiation by binding to sites in mRNA 5ʹ untranslated regions (UTRs) and occluding ribosome binding sites (RBSs). Most often, sRNA-mediated translation inhibition also leads to degradation of the sRNA-mRNA complex ().Citation16 In E. coli and Salmonella, the endoribonuclease RNase E and associated components of the degradosomeCitation17 degrade sRNA-mRNA complexes.Citation18,Citation19 While most sRNA repressors cause both translational silencing and mRNA degradation, some sRNAs only affect one of these two processes. For example, the sRNA Spot 42 regulates galK within the galETKM operon mainly by inhibiting translation; the stability of the galK message is not significantly affected ().Citation20 On the other hand, the MicC sRNA does not directly affect translation of ompD; instead, it directly regulates ompD mRNA stability by directly recruiting the degradosome complex to promote ompD mRNA degradation ().Citation21
The molecular mechanisms described above account for sRNA regulation of many mRNA targets. For the most part, sRNA-mRNA interactions have been characterized one-by-one, and the effect of a given base pairing interaction on expression of a single gene elucidated. Operonic gene organization is a mechanism that ensures coordinated transcription of genes encoding related functions. Global studies from a number of laboratoriesCitation22,Citation23 have suggested that sRNAs provide a means to further link expression of genes in operons at a post-transcriptional level. In some cases, sRNA activity results in simultaneous activation or repression of all genes in the operon (coordinate regulation). In other cases, sRNAs uncouple expression of the cistrons, resulting in discoordinate regulation of the operon (). Coordinate post-transcriptional regulation of polycistronic mRNAs can be achieved by a single sRNA-mRNA base pairing interaction that inhibits translation of an upstream cistron, resulting in polar effects on downstream cistrons (either because of coupled translation or destabilization of the entire mRNA). In contrast, discoordinate regulation can occur when sRNA-mRNA base pairing affects translation of one cistron in the operon but has no effect on the others. In other words, the sRNA up- or downregulates expression of some but not all of the genes encoded on a polycistronic mRNA. This type of regulation is useful, for example, when select products of an operon need to be produced only under particular conditions, while other products are needed constitutively. In this point of view, we discuss what is currently known about how bacterial sRNAs post-transcriptionally regulate operons for either coordinate or discoordinate production of the encoded products.
Figure 2. Select mechanisms of sRNAs regulating operons. Symbols representing sRNAs, mRNAs, ribosomes, RNase E and base pairing interactions are described in . The coding regions of various genes in the operons are indicated by gray arrows. (A) Discoordinate regulation of the galETKM polycistron by Spot 42 is performed solely by inhibition of translation. (B) The RyhB sRNA discoordinately regulates the iscSUA cistrons by both translational silencing and promoting degradation. A strong secondary structure in the iscRS intergenic region (represented by hairpin structure) presumably prevents degradation of the iscR mRNA. (C) Coordinate regulation of the YPK_1206–5 operon by SraG is performed by binding of SraG in the coding sequence of YPK_1206. Whether this regulation involves translational inhibition and/or RNase E-mediated degradation is unknown (indicated by question marks). (D) SgrS coordinately regulates manXYZ by binding to two distinct regions on manXYZ, preventing translation (shown by black, dotted arrows from ribosomes) and promoting co-operative degradation (denoted by the blue, curved arrows.)
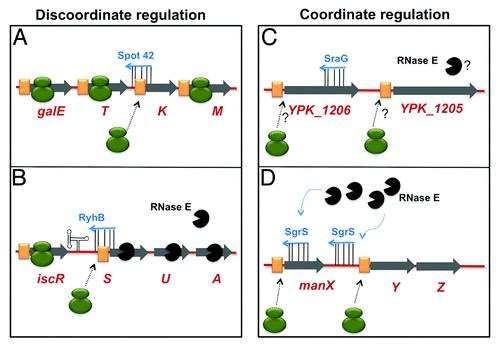
Discoordinate regulation by sRNAs
Spot 42-galETKM mRNA. The 109-nt sRNA Spot 42 was identified in 1979 as a highly abundant RNA species in E. coli.Citation24 Expression of this sRNA was determined to be under the control CRP-cAMP.Citation25 Spot 42 regulates the galETKM operon, which encodes proteins involved in galactose catabolism, the products of which are substrates for cellular glycosylation reactions.Citation20 Relative synthesis of GalE and GalK enzymes varies depending on the activity of CRP-cAMP; GalE:GalK ratios decline 4-fold under catabolite-repressing conditions compared with non-repressing conditions.Citation26 While the GalETKM proteins were observed to be differentially produced in the 1970s,Citation27 it wasn’t until 30 y later that Spot 42 was discovered to mediate this discoordinate regulation. When Spot 42 sRNA is produced, only GalK protein levels are significantly reduced, while synthesis of other proteins from this operon remains unaltered.Citation20 The decrease in GalK levels is due to Spot 42 inhibition of galK translation initiation by binding to a site overlapping the galK RBS. The Spot 42-galK interaction has no significant effect on the stability or steady-state levels of the galETKM mRNA,Citation20 which makes sense, given that synthesis of the galE, T and M gene products is not significantly altered by Spot 42. The data are therefore consistent with a model in which Spot 42 specifically regulates galK by repressing its translation, but allows continued synthesis of other Gal proteins ().Citation20 While GalK activity is only needed for galactose catabolism, the other Gal enzymes are needed constitutively to produce intermediates for cellular glycosylation reactions. The discoordinate regulation of this operon by Spot 42 therefore allows selective repression of a function that is non-essential when preferred carbon sources are available while allowing continued production of required enzymes.
RyhB-iscRSUA mRNA. The RyhB sRNA is an important regulator of bacterial iron homeostasis, and is one of the most extensively studied bacterial sRNAs. Production of the 90-nt RyhB is under the control of Fur, the global transcriptional regulator of iron homeostasis.Citation5 RyhB is therefore only synthesized under conditions of iron starvation. RyhB post-transcriptionally regulates a rather large set of genes that encode iron-dependent enzymes, iron storage proteins and other functions involved in iron uptake and utilization.Citation22,Citation27,Citation28 Recently, Desnoyers, et al. described RyhB-mediated discoordinate regulation of the iscRSUA operon.Citation29 The isc (iron-sulfur cluster) genes are involved in the synthesis and assembly of Fe-S clusters in many proteins in E. coli.Citation30 While the iscS, iscU and iscA genes encode the biogenesis and assembly machinery, IscR is a transcription factor and autorepresses iscRSUA.Citation31 IscR exists in the Holo-form bound by Fe-S clusters or in the unbound Apo-form. Under iron-limiting conditions, Apo-IscR relieves transcriptional repression of the downstream iscRSUA genes.Citation31 Paradoxically, under the same conditions, the sRNA RyhB binds to a site in the ~100-nt iscR-iscS intergenic region, and selectively silences the iscSUA genes.Citation29 The RyhB binding site covers the RBS and the start codon of iscS, implying that this base pairing interaction inhibits iscS translation initiation. The result is endonucleolytic cleavage by RNase E, presumably followed by degradosome-dependent exonucleolytic degradation of the iscSUA portion of the mRNA. The iscR mRNA present upstream of RyhB-iscS pairing is protected by a strong secondary structure at the 3′ end of iscR.Citation29 Taken together, RyhB regulation of iscRSUA is consistent with the model shown in . In this model, RyhB prevents gene expression by both inhibition of translation and selective mRNA degradation.
IscR regulates many genes in addition to iscRSUA, including over 40 genes involved in metabolic pathways, such as anaerobic respiration and oxidative stress response.Citation32 Importantly, Apo-IscR (the form that predominates during iron starvation) activates the suf operon, which encodes alternative Fe-S cluster biogenesis proteins that are used during iron starvation. Thus, RyhB-mediated discoordinate regulation of iscRSUA serves to reduce synthesis of Isc Fe-S cluster biogenesis machinery, but protects synthesis of an important transcription factor which, in turn, induces another pathway for Fe-S cluster assembly.
GlmZ-glmUS mRNA. The sRNA GlmZ mediates discoordinate expression of the glmUS operon,Citation33,Citation34 which encodes proteins involved in amino sugar metabolism, a cellular pathway crucial for cell envelope biogenesis. GlmZ selectively upregulates production of GlmS.Citation33 GlmZ utilizes a canonical anti-antisense mechanism to activate glmS translation ().Citation34 Interestingly, the GlmZ-mediated stimulation of glmS translation is preceded by processing of the dicistronic glmUS message by cleavage at the glmU stop codon. GlmZ pairs only with the processed glmS mRNA to enhance its translation and stability.Citation33 GlmZ is unique in that it discoordinately regulates a polycistronic target by selective activation of one cistron.
Coordinate regulation by sRNAs
SraG-YPK_1206 mRNA. The sRNA SraG was originally identified by bioinformatics studies that identified orphan promoters, consensus transcription initiation and termination sites in E. coli.Citation35 The 5′ and 3′ ends of this sRNA were verified by RACE and northern blotting experiments. As shown in , SraG is an example of an sRNA that apparently binds to one site on a polycistronic mRNA to coordinately regulate both genes in the operon. In E. coli, expression of SraG is growth-phase-dependent and also responsive to heat- and cold-shock treatments.Citation35 While very little is known about the physiological function of SraG, a recent study showed that Yersinia pseudotuberculosis SraG regulates a polycistronic mRNA, YPK_1206–1205.Citation36 While the functions of the YPK_1205 and YPK_1206 proteins are also unknown, translational reporter fusions to both genes were regulated by SraG. Sequences within the coding region of YPK_1206 are necessary and sufficient for negative regulation of both YPK_1206 and the downstream YPK_1205.Citation36 Although a direct binding site between SraG and YPK_1206-1205 has not been identified, truncation analyses revealed that a region between +9–+63 of the YPK_1206 coding region is required for coordinate regulation of the operon.Citation36 Whether SraG affects only translation or both translation and mRNA stability by binding at one site is currently unknown.
SgrS-manXYZ mRNA. SgrS is a 227-nt long sRNA involved in the response to metabolic stress associated with intracellular accumulation of sugar-phosphates (known as glucose-phosphate, or GP, stress).Citation8,Citation9 SgrS production is induced by GP stress, and SgrS represses translation of genes encoding sugar transporters in order to inhibit further sugar uptake and accumulation.Citation8,Citation9,Citation37,Citation38 One sugar transporter mRNA target of SgrS is manXYZ mRNA, the products of which are involved in uptake of a number of sugars.Citation39 The manX and manY genes are separated by 62 nucleotides whereas the manY and the manZ genes are only three nucleotides apart and are translationally coupled.Citation39 The first study characterizing SgrS regulation of manXYZ mRNA showed that SgrS binds to a site in the manX coding region, leading to translational repression of manX and degradation of manXYZ mRNA.Citation37 It was initially hypothesized that coordinate regulation of the manXYZ operon was achieved by SgrS binding to the manX site, repressing manX translation and recruiting the RNase E degradosome for degradation of the manXYZ mRNA. Contrary to that hypothesis, translational regulation of manY and manZ by SgrS was independent of SgrS-manX pairing. Instead, a second SgrS binding site in the manX-manY intergenic region was shown to be responsible for SgrS-dependent repression of manY and manZ.Citation38 These data are also consistent with the fact that translation of manY and manZ is coupled; therefore, pairing at the intergenic site is sufficient to regulate both the downstream cistrons. SgrS pairing at the manX or intergenic sites individually was sufficient for translational repression of manX or manY-manZ, respectively, arguing against cooperativity between the sites with respect to translational regulation. On the contrary, disruption of binding at either of the two sites abolished SgrS-dependent manXYZ mRNA degradation.Citation38 The data are consistent with the model for SgrS regulation of manXYZ shown in , where two separate binding interactions cooperate to promote degradation of full-length mRNA. Why two independent pairing sites are required for SgrS-mediated degradation of the manXYZ message is unclear. It is possible that SgrS binding at both sites somehow cooperates to recruit the RNase E degradosome.
What characteristics influence coordinate and discoordinate regulation of polycistronic mRNAs?
In this point of view, we have described mechanisms of sRNA regulation of polycistronic transcripts. It is apparent that sRNAs can regulate one or more cistrons within operonic transcripts by regulating translation and/or stability of all or portions of the transcript ().
It is not yet clear why different mechanisms of discoordinate regulation would be utilized for specific sRNA-mRNA pairs, but several characteristics of the target mRNA may be important. The organization of the genes in an operon may dictate the use of different mechanisms to achieve discoordinate regulation. For example, although both Spot 42 and RyhB perform discoordinate regulation of target mRNAs, they regulate their respective targets at different levels. Spot 42 regulates the galETKM message at the level of translational repression of galK, whereas RyhB uses both translational repression and message destabilization to decrease production of the IscSUA proteins. The position of the genes in their respective operons is likely a crucial determinant here. The galK gene is in the middle of its operon flanked by genes that are not regulated by Spot 42. In contrast, the iscSUA genes regulated by RyhB are located downstream of the unregulated iscR. Therefore, RyhB inhibition of iscS translation can be coupled to degradation of the iscSUA portion of the mRNA without interfering with iscR expression.
Another characteristic influencing mechanisms of coordinate or discoordinate regulation could be the extent of target regulation required for a particular physiological response. Coupling translational repression to mRNA degradation makes target regulation more robust by making RNA silencing irreversible, and this more stringent repression mechanism may be important physiologically for certain mRNA targets or under certain stress conditions. Consistent with this idea, disrupting SgrS-dependent degradation of manXYZ mRNA leads to impaired recovery from glucose-phosphate stress, even if SgrS translational repression of manX is left intact.Citation38
The SgrS-manXYZ mRNA pair present a novel case of coordinate sRNA regulation of polycistronic mRNA. The involvement of two distinct binding interactions that are independently responsible for translational repression of different cistrons and seem to cooperate to promote degradation of the full-length mRNACitation38 has not been described for other sRNA-mRNA pairs. Nevertheless, there are certainly numerous transcriptome data sets that provide evidence of sRNA-mediated coordinate up- or downregulation of polycistronic mRNAs.Citation22,Citation23 Moreover, multiple sRNA-binding sites have been predicted or shown for some polycistronic mRNAs, such as the Staphylococcus aureus sRNA RsaE pairing with opp3BCDFA mRNACitation40,Citation41 and Spot 42-nanCM mRNA interactions.42 We speculate that regulation of long mRNAs via multiple sRNA-mRNA-binding interactions may be a common but currently unappreciated mechanism of coordinate regulation. It is noteworthy that the most often-used techniques for defining sRNA-mRNA interactions test predicted interactions by taking sites out of their natural context, either via construction of translational reporter fusions in vivo or in vitro use of select portions of RNAs generated by in vitro transcription. Such experiments have been invaluable for defining molecular mechanisms of sRNA activity, but may also have precluded identification of other mechanisms by eliminating unsuspected binding sites.
Conclusions
sRNAs utilize a variety of mechanisms to regulate gene expression from polycistronic messages. They can alter expression of select genes in an operon by inhibiting their translation or by altering the stability of the message, leading to discoordinate regulation. Alternatively, they can affect expression of all genes in an operon by binding to one or more sites on the target mRNA, causing coordinate regulation of the target polycistronic mRNA. Several factors might influence the different binding interactions and mechanisms used by sRNAs to regulate polycistronic messages. Location of genes within operons and tightness of the required regulation are potential factors that affect target regulation. SgrS-manXYZ mRNA interactions can be used as a model to think about sRNAs regulating via multiple binding sites on a target. Continuing to explore the different mechanisms of regulation of operons will expand our understanding of various modes of sRNA action and also further our knowledge of the physiological impacts of such regulatory mechanisms.
Acknowledgments
We are grateful to Dr. Greg Richards and Dr. Jennifer Rice for valuable comments on the manuscript. This work was funded by the National Institutes of Health Grant [1R01 GM092830].
References
- De Lay N, Gottesman S. A complex network of small non-coding RNAs regulate motility in Escherichia coli. Mol Microbiol 2012; 86:524 - 38; http://dx.doi.org/10.1111/j.1365-2958.2012.08209.x; PMID: 22925049
- Sharma C, Heidrich N. Small RNAs and virulence in bacterial pathogens. RNA Biol 2012; 9:361 - 3; http://dx.doi.org/10.4161/rna.20517; PMID: 22546934
- Mann B, van Opijnen T, Wang J, Obert C, Wang YD, Carter R, et al. Control of virulence by small RNAs in Streptococcus pneumoniae. PLoS Pathog 2012; 8:e1002788; http://dx.doi.org/10.1371/journal.ppat.1002788; PMID: 22807675
- Schiano CA, Lathem WW. Post-transcriptional regulation of gene expression in yersinia species. Front Cell Infect Microbiol 2012; 2:129; http://dx.doi.org/10.3389/fcimb.2012.00129; PMID: 23162797
- Massé E, Gottesman S. A small RNA regulates the expression of genes involved in iron metabolism in Escherichia coli. Proc Natl Acad Sci USA 2002; 99:4620 - 5; http://dx.doi.org/10.1073/pnas.032066599; PMID: 11917098
- Guillier M, Gottesman S, Storz G. Modulating the outer membrane with small RNAs. Genes Dev 2006; 20:2338 - 48; http://dx.doi.org/10.1101/gad.1457506; PMID: 16951250
- Papenfort K, Pfeiffer V, Mika F, Lucchini S, Hinton JC, Vogel J. SigmaE-dependent small RNAs of Salmonella respond to membrane stress by accelerating global omp mRNA decay. Mol Microbiol 2006; 62:1674 - 88; http://dx.doi.org/10.1111/j.1365-2958.2006.05524.x; PMID: 17427289
- Richards GR, Vanderpool CK. Molecular call and response: the physiology of bacterial small RNAs. Biochim Biophys Acta 2011; 1809:525 - 31; http://dx.doi.org/10.1016/j.bbagrm.2011.07.013; PMID: 21843668
- Vanderpool CK, Gottesman S. Involvement of a novel transcriptional activator and small RNA in post-transcriptional regulation of the glucose phosphoenolpyruvate phosphotransferase system. Mol Microbiol 2004; 54:1076 - 89; http://dx.doi.org/10.1111/j.1365-2958.2004.04348.x; PMID: 15522088
- Møller T, Franch T, Højrup P, Keene DR, Bächinger HP, Brennan RG, et al. Hfq: a bacterial Sm-like protein that mediates RNA-RNA interaction. Mol Cell 2002; 9:23 - 30; PMID: 11804583
- Kawamoto H, Koide Y, Morita T, Aiba H. Base-pairing requirement for RNA silencing by a bacterial small RNA and acceleration of duplex formation by Hfq. Mol Microbiol 2006; 61:1013 - 22; http://dx.doi.org/10.1111/j.1365-2958.2006.05288.x; PMID: 16859494
- Vogel J, Luisi BF. Hfq and its constellation of RNA. Nat Rev Microbiol 2011; 9:578 - 89; http://dx.doi.org/10.1038/nrmicro2615; PMID: 21760622
- Valentin-Hansen P, Eriksen M, Udesen C. The bacterial Sm-like protein Hfq: a key player in RNA transactions. Mol Microbiol 2004; 51:1525 - 33; http://dx.doi.org/10.1111/j.1365-2958.2003.03935.x; PMID: 15009882
- Soper T, Mandin P, Majdalani N, Gottesman S, Woodson SA. Positive regulation by small RNAs and the role of Hfq. Proc Natl Acad Sci USA 2010; 107:9602 - 7; http://dx.doi.org/10.1073/pnas.1004435107; PMID: 20457943
- Fröhlich KS, Vogel J. Activation of gene expression by small RNA. Curr Opin Microbiol 2009; 12:674 - 82; http://dx.doi.org/10.1016/j.mib.2009.09.009; PMID: 19880344
- Prévost K, Desnoyers G, Jacques JF, Lavoie F, Massé E. Small RNA-induced mRNA degradation achieved through both translation block and activated cleavage. Genes Dev 2011; 25:385 - 96; http://dx.doi.org/10.1101/gad.2001711; PMID: 21289064
- Carpousis AJ. The Escherichia coli RNA degradosome: structure, function and relationship in other ribonucleolytic multienzyme complexes. Biochem Soc Trans 2002; 30:150 - 5; http://dx.doi.org/10.1042/BST0300150; PMID: 12035760
- Caron MP, Lafontaine DA, Massé E. Small RNA-mediated regulation at the level of transcript stability. RNA Biol 2010; 7:140 - 4; http://dx.doi.org/10.4161/rna.7.2.11056; PMID: 20220305
- Bandyra KJ, Said N, Pfeiffer V, Górna MW, Vogel J, Luisi BF. The seed region of a small RNA drives the controlled destruction of the target mRNA by the endoribonuclease RNase E. Mol Cell 2012; 47:943 - 53; http://dx.doi.org/10.1016/j.molcel.2012.07.015; PMID: 22902561
- Møller T, Franch T, Udesen C, Gerdes K, Valentin-Hansen P. Spot 42 RNA mediates discoordinate expression of the E. coli galactose operon. Genes Dev 2002; 16:1696 - 706; http://dx.doi.org/10.1101/gad.231702; PMID: 12101127
- Pfeiffer V, Papenfort K, Lucchini S, Hinton JC, Vogel J. Coding sequence targeting by MicC RNA reveals bacterial mRNA silencing downstream of translational initiation. Nat Struct Mol Biol 2009; 16:840 - 6; http://dx.doi.org/10.1038/nsmb.1631; PMID: 19620966
- Massé E, Vanderpool CK, Gottesman S. Effect of RyhB small RNA on global iron use in Escherichia coli. J Bacteriol 2005; 187:6962 - 71; http://dx.doi.org/10.1128/JB.187.20.6962-6971.2005; PMID: 16199566
- Sharma CM, Papenfort K, Pernitzsch SR, Mollenkopf HJ, Hinton JC, Vogel J. Pervasive post-transcriptional control of genes involved in amino acid metabolism by the Hfq-dependent GcvB small RNA. Mol Microbiol 2011; 81:1144 - 65; http://dx.doi.org/10.1111/j.1365-2958.2011.07751.x; PMID: 21696468
- Sahagan BG, Dahlberg JE. A small, unstable RNA molecule of Escherichia coli: spot 42 RNA. I. Nucleotide sequence analysis. J Mol Biol 1979; 131:573 - 92; http://dx.doi.org/10.1016/0022-2836(79)90008-1; PMID: 390161
- Polayes DA, Rice PW, Garner MM, Dahlberg JE. Cyclic AMP-cyclic AMP receptor protein as a repressor of transcription of the spf gene of Escherichia coli. J Bacteriol 1988; 170:3110 - 4; PMID: 2454912
- Joseph E, Danchin A, Ullmann A. Regulation of galactose operon expression: glucose effects and role of cyclic adenosine 3′,5′-monophosphate. J Bacteriol 1981; 146:149 - 54; PMID: 6260740
- Prévost K, Salvail H, Desnoyers G, Jacques JF, Phaneuf E, Massé E. The small RNA RyhB activates the translation of shiA mRNA encoding a permease of shikimate, a compound involved in siderophore synthesis. Mol Microbiol 2007; 64:1260 - 73; http://dx.doi.org/10.1111/j.1365-2958.2007.05733.x; PMID: 17542919
- Kim JN, Kwon YM. Genetic and phenotypic characterization of the RyhB regulon in Salmonella Typhimurium. Microbiol Res 2013; 168:41 - 9; http://dx.doi.org/10.1016/j.micres.2012.06.007; PMID: 22824499
- Desnoyers G, Morissette A, Prévost K, Massé E. Small RNA-induced differential degradation of the polycistronic mRNA iscRSUA. EMBO J 2009; 28:1551 - 61; http://dx.doi.org/10.1038/emboj.2009.116; PMID: 19407815
- Takahashi Y, Nakamura M. Functional assignment of the ORF2-iscS-iscU-iscA-hscB-hscA-fdx-ORF3 gene cluster involved in the assembly of Fe-S clusters in Escherichia coli. J Biochem 1999; 126:917 - 26; http://dx.doi.org/10.1093/oxfordjournals.jbchem.a022535; PMID: 10544286
- Schwartz CJ, Giel JL, Patschkowski T, Luther C, Ruzicka FJ, Beinert H, et al. IscR, an Fe-S cluster-containing transcription factor, represses expression of Escherichia coli genes encoding Fe-S cluster assembly proteins. Proc Natl Acad Sci USA 2001; 98:14895 - 900; http://dx.doi.org/10.1073/pnas.251550898; PMID: 11742080
- Giel JL, Rodionov D, Liu M, Blattner FR, Kiley PJ. IscR-dependent gene expression links iron-sulphur cluster assembly to the control of O2-regulated genes in Escherichia coli. Mol Microbiol 2006; 60:1058 - 75; http://dx.doi.org/10.1111/j.1365-2958.2006.05160.x; PMID: 16677314
- Kalamorz F, Reichenbach B, März W, Rak B, Görke B. Feedback control of glucosamine-6-phosphate synthase GlmS expression depends on the small RNA GlmZ and involves the novel protein YhbJ in Escherichia coli. Mol Microbiol 2007; 65:1518 - 33; http://dx.doi.org/10.1111/j.1365-2958.2007.05888.x; PMID: 17824929
- Urban JH, Vogel J. Two seemingly homologous noncoding RNAs act hierarchically to activate glmS mRNA translation. PLoS Biol 2008; 6:e64; http://dx.doi.org/10.1371/journal.pbio.0060064; PMID: 18351803
- Argaman L, Hershberg R, Vogel J, Bejerano G, Wagner EG, Margalit H, et al. Novel small RNA-encoding genes in the intergenic regions of Escherichia coli. Curr Biol 2001; 11:941 - 50; http://dx.doi.org/10.1016/S0960-9822(01)00270-6; PMID: 11448770
- Lu P, Zhang Y, Li L, Hu Y, Huang L, Li Y, et al. Small non-coding RNA SraG regulates the operon YPK_1206-1205 in Yersinia pseudotuberculosis. FEMS Microbiol Lett 2012; 331:37 - 43; http://dx.doi.org/10.1111/j.1574-6968.2012.02548.x; PMID: 22428705
- Rice JB, Vanderpool CK. The small RNA SgrS controls sugar-phosphate accumulation by regulating multiple PTS genes. Nucleic Acids Res 2011; 39:3806 - 19; http://dx.doi.org/10.1093/nar/gkq1219; PMID: 21245045
- Rice JB, Balasubramanian D, Vanderpool CK. Small RNA binding-site multiplicity involved in translational regulation of a polycistronic mRNA. Proc Natl Acad Sci USA 2012; 109:E2691 - 8; http://dx.doi.org/10.1073/pnas.1207927109; PMID: 22988087
- Erni B, Zanolari B, Kocher HP. The mannose permease of Escherichia coli consists of three different proteins. Amino acid sequence and function in sugar transport, sugar phosphorylation, and penetration of phage lambda DNA. J Biol Chem 1987; 262:5238 - 47; PMID: 2951378
- Geissmann T, Chevalier C, Cros MJ, Boisset S, Fechter P, Noirot C, et al. A search for small noncoding RNAs in Staphylococcus aureus reveals a conserved sequence motif for regulation. Nucleic Acids Res 2009; 37:7239 - 57; http://dx.doi.org/10.1093/nar/gkp668; PMID: 19786493
- Beisel C, et al. Multiple factors dictate target selection by Hfq-binding small RNAs. EMBO J 2012; 31:1961 - 7; http://dx.doi.org/10.1093/nar/gkq462; PMID: 20511587