Abstract
In plants, endogenes are less prone to RNA silencing than transgenes. While both can be efficiently targeted by small RNAs for post-transcriptional gene silencing (PTGS), generally only transgene PTGS is accompanied by transitivity, RNA-directed DNA methylation (RdDM) and systemic silencing. In order to investigate whether a transgene could mimick an endogene and thus be less susceptible to RNA silencing, we generated an intron-containing, endogene-resembling GREEN FLUORESCENT PROTEIN (GFP) transgene (GFPendo). Upon agroinfiltration of a hairpin GFP (hpF) construct, transgenic Nicotiana benthamiana plants harboring GFPendo (Nb-GFPendo) were susceptible to local PTGS. Yet, in the local area, PTGS was not accompanied by RdDM of the GFPendo coding region. Importantly, hpF-agroinfiltrated Nb-GFPendo plants were resistant to systemic silencing. For reasons of comparison, transgenic N. benthamiana plants (Nb-GFPcDNA) carrying a GFP cDNA transgene (GFPcDNA) were included in the analysis. HpF-agroinfiltrated Nb-GFPcDNA plants exhibited local PTGS and RdDM. In addition, systemic silencing was established in Nb-GFPcDNA plants. In agreement with previous reports using grafted scions, in systemically silenced tissue, siRNAs mapping to the 3′ of GFP were predominantly detectable by Northern blot analysis. Yet, in contrast to other reports, in systemically silenced leaves, PTGS was also accompanied by dense RdDM comprising the entire GFPcDNA coding region. Overall, our analysis indicated that cDNA transgenes are prone to systemic PTGS and RdDM, while endogene-resembling ones are resistant to RNA silencing.
Introduction
RNA silencing refers to a network of small RNA (sRNA)-mediated pathways that are important for normal plant development, genome stability, defense against invading nucleic acids and response to abiotic/biotic stress.Citation1,Citation2 In general, there are two major pathways of RNA silencing in plants; the small interfering RNA (siRNA) and the microRNA (miRNA)-mediated silencing pathways.Citation3,Citation4 The siRNA pathway is induced by double stranded RNA (dsRNA) that is processed by the DICER-LIKE (DCL) endonucleases, DCL2, DCL3 and DCL4, into 22-, 24-, and 21-nucleotides (nt) long siRNAs, respectively.Citation5 Mature siRNAs exhibit 3′ 2-nt overhangs and become stabilized though 3′ end methylation by HUE ENHANCER1 (HEN1).Citation6 Depending on their 5′ terminal nucleotide, siRNAs are loaded onto specific ARGONAUTE (AGO) proteins.Citation7 Only one siRNA strand, the guide strand, is finally selected.Citation8 21-nt siRNAs are loaded primarily onto AGO1, and to lower extent onto AGO2, leading to the formation of RNA-induced silencing complex (RISC).Citation9 RISC mediates post-transcriptional gene silencing (PTGS) through targeting RNA molecules that are complementary to the guide strand. PTGS results in target RNA degradation and/or, in the case of mRNA targets, in translational inhibition.Citation10,Citation11 24-nt siRNAs are loaded onto AGO4/AGO6 and are supposed to target homologous genomic DNA for de novo methylation, in a process termed RNA-directed DNA methylation (RdDM).Citation12,Citation13
PTGS and RdDM are both initiated by dsRNA. Among others, sources of dsRNA are replication intermediates of viruses and viroids, transcription of inverted repeats, stress-induced overlapping antisense transcripts and RNA-DIRECTED RNA POLYMERASE (RDR) transcription of aberrant RNAs (abRNAs).Citation14-Citation16 AbRNAs are most probably transcripts devoid of 5′ cap and/or 3′ polyadenylation tail.Citation17,Citation18 Such transcripts may directly be generated through abnormal DNA-DEPENDENT RNA POLYMERASE II (POL II) transcription, e.g., producing truncated and/or read-through transcripts. In addition, abRNA may derive from RISC-mediated generation of 5′ and 3′ target RNA cleavage products. Thus, while initial cleavage is conducted by primary siRNAs, RDR6 transcription of the cleavage products generates secondary dsRNA that is processed into secondary siRNAs.Citation19,Citation20 In contrast to primary siRNAs, that match only to a sub-region of the target RNA, secondary siRNAs may map upstream and downstream from the binding sites of the primary siRNAs. This spreading of silencing beyond initial target sites is termed transitivity.Citation15,Citation21 The exact nature of secondary siRNAs is not known, but it seems that at least DCL2 is involved in their biogenesis.Citation22 Interestingly, while secondary siRNAs are cleavage-competent, they cannot further support the biogenesis of tertiary siRNAs. Thus, transitivity may spread to 5′ and 3′ direction, but not indefinitely.Citation21,Citation23 Nevertheless, transitivity results in the amplification of siRNAs constituting a self-reinforcing feedback loop of silencing. Silencing may spread through plasmodesmata cell-to-cell and through the vasculare system to systemic parts of the plant.Citation24,Citation25 While cell-to-cell signals are most probably 21-nt duplex siRNAs, the nature of systemic silencing signals is still elusive.Citation26-Citation29 It has been proposed that 24-nt siRNAsCitation30-Citation32 or longer RNAsCitation33 may be the molecules traveling through the phloem to metabolic sink tissues, but solid experimental evidence for either scenario is lacking.
In general, the response of transgenes and endogenes against RNA silencing is diverse. Both, endogenes and transgenes are equally susceptible to sRNA-mediated trans-PTGS. However, with a few exceptions, including TAS genes in Arabidopsis and BETA-1,3-GLUCANASE genes in tobacco, (1) endogenes are generally not prone to transitivity.Citation34,Citation35 Likewise, with the exception of two endogenous INVERTED-REPEAT (IR) loci in Arabidopsis, (2) endogenes are not prone to systemic silencing.Citation26,Citation36,Citation37 In contrast to transgenes, (3) endogenes appear to be resistant to RdDM in N. benthamiana.Citation38 Finally, in contrast to transgenes, (4) endogenes are not prone to spontaneous silencing (cis-silencing).Citation39,Citation40 In order to analyze if a transgene can mimick an endogene with introns and thus, become less prone to RNA silencing, we constructed an intron-containing endogene-resembling GFP transgene (GFPendo). We have previously shown that upon agroinfiltration, GFPendo was poorly processed into siRNAs.Citation41 In this study, we generated transgenic N. benthamiana GFPendo plants (Nb-GFPendo) and we explored the response of GFPendo against RNA silencing. In summary, our data indicated that, similar to most endogenes, GFPendo was no target for RdDM and systemic silencing.
Results
Transgenic Nb-GFPendo and Nb-GFPcDNA plants
The GFPendo construct was designed to resemble the structure of one of the highly transcribed small subunit of RIBULOSE-1,5-BIPHOSPHATE CARBOXYLASE OXYGENASE (RuBisCO) genes.Citation41 The GFPendo consists of the RuBisCO promoter/5′UTR/two introns/3′ UTR/terminator and three (arbitrarily chosen) GFP exons (). RuBisCO genes are highly transcribed endogenes that are not prone to RDR6-mediated processing.Citation15 Previously, we could show that upon agroinfiltration, GFPendo transcripts were inefficiently processed by RDR6-mediated pathway.Citation41 In addition to transitivity, RDR6 is involved in the establishment of RdDM and systemic silencing.Citation15,Citation42,Citation43 We assumed that reduced RDR6-processivity of the agroinfiltrated GFPendo construct could also have an impact on the efficiency of RdDM and systemic silencing of a genome-integrated GFPendo construct. Thus, transgenic N. benthamiana GFPendo plants (Nb-GFPendo) were generated. For reasons of comparison, the N. benthamiana plant line 16C carrying a GFP cDNA transgeneCitation44 was used. Line 16C (hereafter referred to as Nb-GFPcDNA) is exceptional in that it shows remarkably high GFP expression without being prone to spontaneous cis-silencing.Citation36,Citation39,Citation40 Importantly, Nb-GFPendo and Nb-GFPcDNA plants exhibited almost similar GFP expression (, and S1). Thus, any putative divergence of responses against RNA silencing of the two transgenes should reflect qualitative rather than quantitative effects.
Figure 1. Schematic representation of GFPendo, hpF and GFPcDNA constructs. P35S: CaMV 35S promoter; TNOS: nopaline synthase (NOS) terminator; GFP: green fluorescent protein; PRbc: tobacco RuBisCO promoter including 5′ UTR; TRbc: tobacco RuBisCO terminator including 3′ UTR; Intron 1 and 2: tobacco RuBisCO introns 1 and 2. The hybridization probes used for northern blot analysis () and the 182 bp and 134 bp regions analyzed by bisulfite sequencing (, S2 and S3) are indicated by black bars.
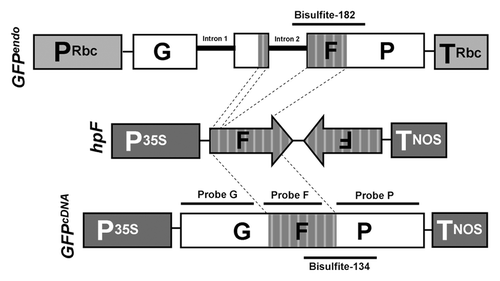
Figure 2. GFP monitoring of hpF-agroinfiltrated GFPendo and GFPcDNAN. benthamiana plants. The development of RNA silencing was monitored at local levels (4 and 8 dpi) and systemic levels (21 and 46 dpi).
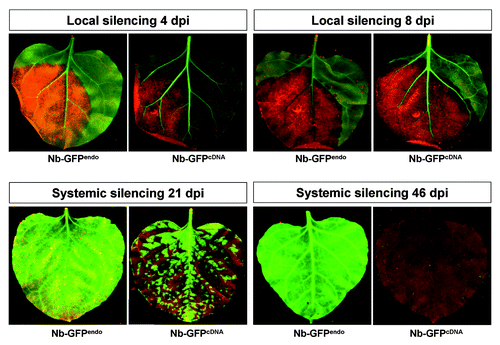
Figure 3. Northern blot analysis of RNA samples of hpF-agroinfiltrated GFPendo and GFPcDNAN. benthamiana plants. Large and small RNA analysis was performed at local levels (4 and 8 dpi) and systemic levels (21 dpi). The regions corresponding to hybridization probes are indicated in . Ethidium bromide staining and miR159 accumulation served as loading controls. 'Mock' corresponds to 10 mM MgCl2 infiltration.
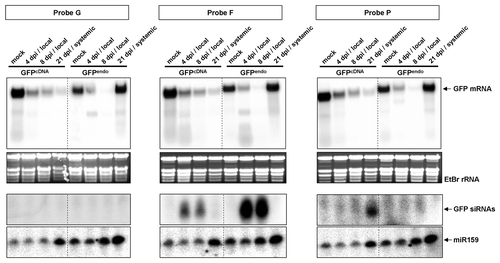
Analysis of PTGS in locally silenced leaves
Endogenes and transgenes are prone to RNA silencing when an exogenous trigger e.g., hairpin RNA (hpRNA) is present and processed into siRNAs (trans-silencing).Citation45,Citation46 In order to compare trans-silencing of GFPendo and GFPcDNA, a hairpin GFP (hpF) construct covering 139 bp of the GFP middle region (F) (), was agroinfiltrated into Nb-GFPendo and Nb-GFPcDNA plants. For each treatment, three plants were agroinfiltrated (three leaves for each plant) and the pooled tissue was used for subsequent analysis. Both, Nb-GFPendo and Nb-GFPcDNA plants revealed local PTGS already 4 days post infiltration (dpi) and displayed full local GFP silencing at 8 dpi (). SRNA-mediated silencing in plants results in mRNA degradation and/or translational inhibition.Citation10,Citation39 In order to discriminate between the two processes, RNA was extracted from a pool of agroinfiltrated leaves and subjected to Northern blot analysis. Our data revealed that primary GFP siRNAs, originating from the agroinfiltrated hpF construct, targeted GFPendo and GFPcDNA transcripts for degradation (, probe F). In addition to the production of primary siRNAs, transitivity-derived secondary siRNAs may also be generated by RDR6/DCLs processing of abRNA. However, in samples of locally silenced tissue, no secondary 5′ and 3′ siRNAs were detectable under the applied conditions (, probes G and P). Secondary siRNAs production may be slowCitation23 and may take longer than 8 dpi. However, progressive physiological degeneration of agroinfiltrated tissue 8 dpi did not allow analysis at later stages.
Analysis of RdDM in locally silenced leaves
PTGS is tightly related to RdDM. In the case of transgenes, silencing of mRNA is generally associated with RdDM of its coding region, which in turn, may contribute to maintain PTGS.Citation46 Endogenes and transgenes are efficiently targeted for trans-PTGS. However, why only transgenes are prone to RdDMCitation46 is still elusive. In order to investigate if the endogene-resembling GFPendo differs from GFPcDNA in view of being a target for RdDM, the methylation status of the transgene constructs was analyzed by bisulfite sequencing using pooled samples from locally silenced leaf tissue (8 dpi) (). We found that GFPcDNA was heavily methylated, not only in the region targeted by the hpF silencing trigger, but also in the 3′ downstream sequence ( and S2). This finding was in agreement with another study reporting on RdDM spreading.Citation47 Notably, the observed 3′ spreading of RdDM was not correlated with the accumulation of detectable amounts of 3′ siRNAs (, probe P). In contrast to the GFPcDNA, GFPendo was poorly methylated in locally silenced tissue ( and S3). This finding was striking, since the silencing triggers of Nb-GFPendo and Nb-GFPcDNA were identical (hpF). In addition, abundant primary hpF-derived siRNAs were detectable in Nb-GFPendo and Nb-GFPcDNA samples (, probe F). Thus, one would have expected RdDM of GFPendo, at least, in the region targeted by hpF. These findings suggest that the mere presence of siRNAs was not sufficient to efficiently trigger RdDM.
Figure 4. Histogram representing bisulfite sequencing data from GFPcDNA and GFPendo. Non-agroinfiltrated, 8 dpi local and 21 dpi systemic tissue was used for analysis. 10–15 clones were sequenced and data were analyzed with CyMate software.Citation68 Histogram represents the data presented in Fig. S2 and S3. In non-agroinfiltrated GFPcDNA, transgenerationally maintained CG methylation was detected, in agreement with previous observations.Citation41,Citation70
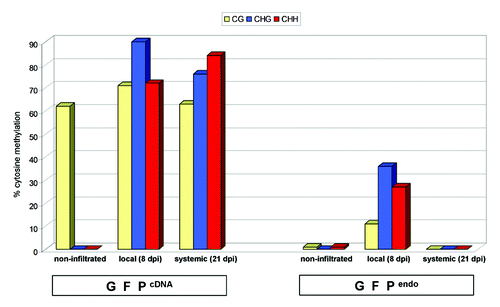
Systemic PTGS analysis
Endogenes and transgenes are efficiently targeted for local PTGS, but only transgenes appeared to be prone to systemic PTGS.Citation25,Citation37,Citation48 Thus, we were interested to investigate if locally silenced Nb-GFPendo plants also become systemically silenced. For this reason, 12 Nb-GFPcDNA and Nb-GFPendo plants displaying local silencing post hpF-agroinfiltration were monitored up to 46 dpi for systemic silencing. All Nb-GFPcDNA plants exhibited systemic PTGS already 21 dpi (). Importantly, and in agreement with previous observations, only 3′ GFP siRNAs were detectable in systemically silenced leaves by Northern blot analysis (, probe P).Citation33,Citation49 In contrast, 21 dpi and even 46 dpi no systemic GFP silencing was observed in Nb-GFPendo plants. At 46 dpi Nb-GFPcDNA plants were fully silenced (). We thus conclude that, similar to most endogenes, GFPendo failed to induce the production of and/or failed to respond to systemic silencing signals.
Analysis of RdDM in systemically silenced leaves
In grafting experiments employing transgenic plants, silenced scions induced systemic PTGS and RdDM in the rootstock.Citation30,Citation32,Citation50 In a reverse experiment using a silenced rootstock, the scion became systemically silenced, but de novo methylation of the transgene was not detectable suggesting that the signals for induction of PTGS and RdDM are not identical.Citation33,Citation51,Citation52 In contrast to grafting experiments, we induced local silencing in leaves of individual Nb-GFPcDNA and Nb-GFPendo plants. DNA was extracted from a pool of newly emerged Nb-GFPcDNA (almost fully silenced) and Nb-GFPendo (non-silenced) leaves 21 dpi, respectively (). The DNA samples were subjected to bisulfite sequencing. The sequencing data showed that, as expected, the GFPendo was not methylated ( and S3). In contrast, the coding region of the GFPcDNA construct was heavily methylated ( and S2). Importantly, RdDM patterns were not restricted to the siRNA-mapping 3′ region but were established along the entire coding region (Fig. S2). Bisulfite sequencing data were validated by Southern blot analysis using the methylation sensitive restriction endonucleases, NcoI, DdeI and PvuII (Fig. S4). In summary, our data suggested that in a non-grafting system, systemic PTGS of GFPcDNA is associated with efficient RdDM of the silenced transgene.
Discussion
The RdDM machinery is considered to be guided by 24-nt siRNAs.Citation53-Citation55 In our experiments, primary GFP siRNAs were detected in samples of agroinfiltrated Nb-GFPendo and Nb-GFPcDNA leaves. However, GFPendo was inefficiently targeted for RdDM. Thus, our data suggests that either the mere presence of siRNAs is not sufficient to trigger RdDM, or molecules, other than siRNAs may target genomic DNA for de novo methylation.Citation56,Citation57 Establishment of RdDM is not only based on the presence of RdDM trigger molecules, but also on the transcription of RdDM targets (DNA and/or nascent transcripts). Typically, DNA-DEPENDENT RNA POLYMERASES IV and V (POLIV and POLV, respectively) are thought to transcribe RdDM targets, thus producing scaffold transcripts for the binding of trigger molecules.Citation13,Citation58,Citation59 In addition, RDR2 and RDR6 transcription of target transcripts appeared to be essential for the establishment and/or amplification of RdDM.Citation43,Citation47 Differential POLIV/POLV/RDR2/RDR6 processing of GFPendo and GFPcDNA may also account for their differential methylation patterns we observed.
Production of systemic silencing signals does not require DCL1, DCL2, DCL3, DCL4, RDR2, RDR6 and POLIV,Citation29 indicating that systemic silencing is not triggered by a typical siRNA. However, upon reception, the response to systemic signals depends on RDR6, RDR2, DCL3 and POLIV.Citation29 Our previous data showed that, when agroinfiltrated into Nb-GFPcDNA leaves, GFPendo induced diminished local and delayed systemic silencing of GFPcDNA.Citation41 Here, we found that upon agroinfiltration of hpF, GFPendo did not respond to systemic silencing signals. Since systemic silencing requires RDR6, one may speculate that GFPendo does not produce RNA molecules that serve as a substrates for RDR6. Thus, similar to typical endogenes,Citation36 transgenes resembling endogenes seem not to respond to systemic silencing signals.
The diverse response of GFPendo and GFPcDNA to systemic silencing and RdDM indicated that the availability of RDR6 substrates, probably abRNA, is a critical step in these processes. RDR6 seems to prefer abRNAs that lack either 5′ cap and/or 3′ polyadenylation tail.Citation17,Citation18 Most likely, both GFPendo and GFPcDNA produce abRNAs. In the case of GFPcDNA, abRNAs may refer to truncated and/or read-through transcripts, lacking 5′ cap and/or 3′ polyadenylation tail, thus serving as optimal RDR6 substrates.Citation17,Citation18 In the case of GFPendo, abRNAs may in addition, include intron-containing mispliced and/or unspliced transcripts that were 'overlooked' by the spliceosome. We suggest that, while GFPcDNA abRNAs are predominantly copied by RDR6 and induce RNA silencing, GFPendo abRNAs are not processed by RDR6 but are instead eliminated via exonucleolytic RNA decay mechanism.Citation17,Citation60,Citation61 GFPendo differed in several aspects from GFPcDNA (promoter, UTRs, introns, terminator, integration site). In support of our suggestion, while the presence of UTRs and introns is positively correlated with RNA decay, it is negatively correlated with RNA silencing.Citation23,Citation60,Citation62-Citation64 Spliceosome association likely excludes RDR6 recruitment.Citation16 Thus, endogenous siRNAs matching the transcripts of intron-containing genes are underrepresented in sRNA libraries of Arabidopsis, while siRNAs derived from intronless gene transcripts are highly abundant.Citation63 Nevertheless, a couple of endogenous transcripts are targeted by RDR6 leading to the production of endogenous siRNAs.Citation34,Citation65
In conclusion, we have shown that an endogene-resembling transgene is resistant to systemic silencing and DNA methylation, most probably due to its poor RDR6-mediated processing. The presence of each endogenous element (promoter, 5′ UTR, introns, 3′ UTR, terminator) may have its own contribution to this phenomenon, but we find of particular importance the presence of introns. Yet, investigating the role of each parameter was beyond the scope of our analysis and would have required the production of an enormous amount of independent transgene constructs comprising all free combinations of the individual RuBisCO gene elements. Moreover, such work would not include the introduction of the introns at different GFP coding region sides or the analysis of an alternative reporter gene. Our data suggest that endogene-resembling transgenes may point toward a new generation of transgenes, where high transcription rates would not be compromised by concomitant susceptibility to RNA silencing.
Materials and Methods
Generation of transgenic plants
The generation of pG104-GFPendo construct has been previously described.Citation41 Agrobacterium tumefaciens (strain ATHV) containing pG104-GFPendo was used to transform N. benthamiana via leaf disc transformation, as previously described.Citation66 Since pG104-GFPendo did not contain a selection marker, co-transformation with A. tumefaciens (strain GV3101) containing the binary vector pPCV702SM-Δ67 was conducted for kanamycin selection. Independent T1 lines were characterized by Southern and northern blot analysis for copy number and expression levels of the transgene (Fig. S5). At least two independent GFPendo lines (18 and 25) containing multiple transgene copies, displayed GFP expression levels and GFP fluorescence similar that of GFPcDNA line 16C. Segregants of the GFPendo line 25, containing 2–5 transgene copies (Fig. S5) were used for subsequent analysis.
RNA extraction and northern blot analysis
RNA was isolated with NucleoSpin miRNA kit (Macherey-Nagel, www.mn-net.com) according to the manufacturer's instructions. Large and small RNA fractions were separately isolated and used for mRNA and siRNA analysis. For mRNA analysis, 5 µg of large RNA fraction was analyzed in 1.2% agarose/formaldehyde gels, and blotted onto positively charged nylon membranes BioBond Plus (Sigma-Aldrich, www.sigmaaldrich.com) and UV312nm-cross-linked (300 mJ/cm2). The PerfectHyb Plus 1x (Sigma-Aldrich) was used for overnight hybridization at 64 °C. Membranes were washed at 64 °C with buffer 1 (2x SSC, 0.1% SDS (w/v)) for 30 min and with buffer 2 (0.5x SSC, 0.1% SDS (w/v)) for 15 min. For siRNA analysis, 5 µg of small RNA fraction was analyzed in 15% TBE-Urea gels and blotted onto positively charged nylon membranes Bright Star (Life Technologies, www.lifetechnologies.com) by electro-blotting at 300mA for 1 h and UV312nm-cross-linked (300 mJ/cm2). The PerfectHyb Plus 1x (Sigma-Aldrich) was used for overnight hybridization at 42 °C and membranes were washed twice with buffer 1 (2x SSC, 0.1% SDS (w/v)) at 42 °C for 30 min. For the generation of probes, templates obtained by PCR on GFP sequence were used in a random labeling reaction with DecaLabel DNA labeling kit (ThermoScientific, www.thermoscientific.com) with a-32P dATP. For probe G (291 bp, 62% A/T context), the primers 5′-ATG AAG ACT AAT CTT TTT CT-3′ and 5′-ATC TGG GTA TCT TGA AAA GC-3′ were used. For probe F (144 bp, 47% A/T context), the primers 5′-CAT ATG AAG CGG CAC GAC TT-3′ and 5′-CTC GAT CCT GTT GAC GAG GG-3′ were used. For probe P (336 bp, 53% A/T context), the primers 5′-CTT AAG GGA ATC GAT TTC AA-3′ and 5′-TAG TTC ATC CAT GCC ATG TG-3′ were used. Probes G, F and P are continuous and cover the full GFP sequence. For the detection of miR159, the oligonucleotide 5′-AAG AGC TCC CTT CAA TCC AAA-3′ was used in an end-labeling reaction using T4 polynucleotide kinase (New England Biolabs, www.neb.com) and γ-32P ATP.
DNA extraction and Southern blot analysis
To 1 g of grinded fresh leaf material, 4 ml extraction buffer (2% (w/v) CTAB, 20 mM EDTA, 1.4 M NaCl, 100 mM TRIS-HCl, pH = 8), 40 µl proteinase K solution and 8 µl RNase A were added, the mixture was incubated at 65 °C for 1 h then briefly cooled on ice. Four ml of chloroform:octanol (24:1) was added and the sample was centrifuged (6000 rpm, 15 min, 4 °C) on Rotanda 360R. To the upper phase, 400 µl CTAB/NaCl solution (10% (w/v) CTAB, 0.7 M NaCl) and 4 ml chloroform:octanol (24:1) were added and the sample was centrifuged (6000 rpm, 15 min, 4 °C). To the upper phase 4 ml precipitation buffer (1% (w/v) CTAB, 10 mM EDTA, 50 mM TRIS-HCl, pH = 8) were added and the mixture was kept overnight at 37 °C. The DNA was precipitated (6000 rpm, 15 min, 4 °C) and the pellet was resuspended in 1 ml high salt TE solution (1 M NaCl, 0.1 mM EDTA, 10 mM TRIS-HCl, pH = 8) at 65 °C for 30 min. The DNA was re-precipitated (6000 rpm, 15 min, 4 °C) with 1 ml isoporopanol and washed twice (centrifugation at 6000 rpm, 15 min, 4 °C) with 1 ml ethanol 70% (v/v). Finally, the DNA pellet was air-dried for 5 min and resuspended in water. For Southern blot analysis, 10 µg DNA were digested with the corresponding restriction endonucleases, separated onto 1% agarose gels and treated for 30 min with denaturation buffer (1 M NaCl, 0.4 N NaOH). DNA was capillary transferred overnight onto positively charged nylon membranes (BioBond Plus, Sigma-Aldrich, www.sigmaaldrich.com) with denaturation buffer. After transfer, the membranes were treated with equilibration buffer (1 M NaCl, 1 M TRIS-HCl, pH = 7), air-dried and UV312nm-cross-linked (100 mJ/cm2). For the generation of probe 35S, templates obtained by PCR (primers 5′-CCG GAA ACC TCC TCG GAT TCC-3′ and 5′-GAT GAT CCC CCT CTC CAA ATG-3′) on 35S promoter sequence were used in a random labeling reaction with DecaLabel DNA labeling kit (ThermoScientific, www.thermoscientific.com) and a-32P dATP. The PerfectHyb Plus 1x (Sigma-Aldrich, www.sigmaaldrich.com) was used for overnight hybridization at 64 °C. Membranes were washed at 64 °C with buffer 1 (2x SSC, 0.1% (w/v) SDS) for 30 min and with buffer 2 (1x SSC, 0.1% (w/v) SDS) for 15 min. Membranes were exposed to FujiFilm Imaging Plates (Fuji, www.fujifilm.com) for 2 d and scanned using PharosFX Plus PhosphorImager (BioRad, www.bio-rad.com).
Western blot analysis
Protein was extracted from Nb-GFPendo and Nb-GFPcDNA and subjected to western blot analysis with GFP antibody as previously described.Citation67
Bisulfite sequencing
For bisulfite sequencing, 500 ng DNA were pre-digested with DraI and subjected to bisulfite treatment using the EZ DNA Methylation-Lightning Kit (ZYMO Research, www.zymoresearch.com) according to the manufacturer's instructions. The recovered material was amplified by PCR with Zymo Taq DNA Polymerase (ZYMO Research, www.zymoresearch.com) with the primer pair 5′-TTT GTT YYA AAA AAA AAA GAA GAA GAA G-3′ and 5′-RRA RTT RTA RTT RTA TTC CAA CTT-3′ for GFPendo and the primer pair 5′-CTT TRA TRC CRT TCT TTT RCT TRT C-3′ and 5′-TTY AAG GAY GAY GGG AAY TAY AAG A-3′ for GFPcDNA at the following conditions: 10 min at 95 °C, 44 amplification cycles (95 °C for 30 s, gradient 45–47–51 °C for 30 s and 72 °C for 30 s), and 72 °C for 10 min. Gradient amplicons were gel excised with Qiagen gel extraction kit (Qiagen, www.qiagen.com), pooled and ligated into pGEM T Easy (Promega, www.promega.com). 10–15 clones were sequenced for each experiment. For interpretation of bisulfite sequencing data the CyMate software was used.Citation68
Agroinfiltration assay
Agrobacterium tumefaciens (strain GV3101) containing the binary vector pPCV702SM-GpG (hpF)Citation67 was used for N. benthamiana leaf agroinfiltration at an optical density (OD) of 1.0, as previously described.Citation69
GFP monitoring
Pharos FX molecular scanning (Biorad, www.bio-rad-com) was used for scanning of GFP fluorescence, as recommended by the manufacturer‘s instructions. Leaves were scanned by CY3 and FITC filter channels and the merged picture depicted GFP fluorescence.
Additional material
Download Zip (1.3 MB)Acknowledgments
This project was supported by the German Research Foundation (DFG) (grants: Wa1019/8–1). We thank Dr. Mirko Moser and Nora Schwind for their contribution in the production of transgenic plants.
References
- Martínez de Alba AE, Elvira-Matelot E, Vaucheret H. Gene silencing in plants: a diversity of pathways. Biochim Biophys Acta 2013; 1829:1300 - 8; http://dx.doi.org/10.1016/j.bbagrm.2013.10.005; PMID: 24185199
- Pumplin N, Voinnet O. RNA silencing suppression by plant pathogens: defence, counter-defence and counter-counter-defence. Nat Rev Microbiol 2013; 11:745 - 60; http://dx.doi.org/10.1038/nrmicro3120; PMID: 24129510
- Voinnet O. Use, tolerance and avoidance of amplified RNA silencing by plants. Trends Plant Sci 2008; 13:317 - 28; http://dx.doi.org/10.1016/j.tplants.2008.05.004; PMID: 18565786
- Voinnet O. Origin, biogenesis, and activity of plant microRNAs. Cell 2009; 136:669 - 87; http://dx.doi.org/10.1016/j.cell.2009.01.046; PMID: 19239888
- Fusaro AF, Matthew L, Smith NA, Curtin SJ, Dedic-Hagan J, Ellacott GA, Watson JM, Wang MB, Brosnan C, Carroll BJ, et al. RNA interference-inducing hairpin RNAs in plants act through the viral defence pathway. EMBO Rep 2006; 7:1168 - 75; http://dx.doi.org/10.1038/sj.embor.7400837; PMID: 17039251
- Yang Z, Ebright YW, Yu B, Chen X. HEN1 recognizes 21-24 nt small RNA duplexes and deposits a methyl group onto the 2′ OH of the 3′ terminal nucleotide. Nucleic Acids Res 2006; 34:667 - 75; http://dx.doi.org/10.1093/nar/gkj474; PMID: 16449203
- Mallory A, Vaucheret H. Form, function, and regulation of ARGONAUTE proteins. Plant Cell 2010; 22:3879 - 89; http://dx.doi.org/10.1105/tpc.110.080671; PMID: 21183704
- Kim VN. Sorting out small RNAs. Cell 2008; 133:25 - 6; http://dx.doi.org/10.1016/j.cell.2008.03.015; PMID: 18394983
- Baulcombe D. RNA silencing in plants. Nature 2004; 431:356 - 63; http://dx.doi.org/10.1038/nature02874; PMID: 15372043
- Brodersen P, Sakvarelidze-Achard L, Bruun-Rasmussen M, Dunoyer P, Yamamoto YY, Sieburth L, Voinnet O. Widespread translational inhibition by plant miRNAs and siRNAs. Science 2008; 320:1185 - 90; http://dx.doi.org/10.1126/science.1159151; PMID: 18483398
- Lanet E, Delannoy E, Sormani R, Floris M, Brodersen P, Crété P, Voinnet O, Robaglia C. Biochemical evidence for translational repression by Arabidopsis microRNAs. Plant Cell 2009; 21:1762 - 8; http://dx.doi.org/10.1105/tpc.108.063412; PMID: 19531599
- Wassenegger M, Heimes S, Riedel L, Sänger HL. RNA-directed de novo methylation of genomic sequences in plants. Cell 1994; 76:567 - 76; http://dx.doi.org/10.1016/0092-8674(94)90119-8; PMID: 8313476
- Wierzbicki AT, Cocklin R, Mayampurath A, Lister R, Rowley MJ, Gregory BD, Ecker JR, Tang H, Pikaard CS. Spatial and functional relationships among Pol V-associated loci, Pol IV-dependent siRNAs, and cytosine methylation in the Arabidopsis epigenome. Genes Dev 2012; 26:1825 - 36; http://dx.doi.org/10.1101/gad.197772.112; PMID: 22855789
- Dalmay T, Hamilton A, Rudd S, Angell S, Baulcombe DC. An RNA-dependent RNA polymerase gene in Arabidopsis is required for posttranscriptional gene silencing mediated by a transgene but not by a virus. Cell 2000; 101:543 - 53; http://dx.doi.org/10.1016/S0092-8674(00)80864-8; PMID: 10850496
- Vaistij FE, Jones L, Baulcombe DC. Spreading of RNA targeting and DNA methylation in RNA silencing requires transcription of the target gene and a putative RNA-dependent RNA polymerase. Plant Cell 2002; 14:857 - 67; http://dx.doi.org/10.1105/tpc.010480; PMID: 11971140
- Wassenegger M, Krczal G. Nomenclature and functions of RNA-directed RNA polymerases. Trends Plant Sci 2006; 11:142 - 51; http://dx.doi.org/10.1016/j.tplants.2006.01.003; PMID: 16473542
- Gazzani S, Lawrenson T, Woodward C, Headon D, Sablowski R. A link between mRNA turnover and RNA interference in Arabidopsis. Science 2004; 306:1046 - 8; http://dx.doi.org/10.1126/science.1101092; PMID: 15528448
- Luo Z, Chen Z. Improperly terminated, unpolyadenylated mRNA of sense transgenes is targeted by RDR6-mediated RNA silencing in Arabidopsis. Plant Cell 2007; 19:943 - 58; http://dx.doi.org/10.1105/tpc.106.045724; PMID: 17384170
- Rajeswaran R, Aregger M, Zvereva AS, Borah BK, Gubaeva EG, Pooggin MM. Sequencing of RDR6-dependent double-stranded RNAs reveals novel features of plant siRNA biogenesis. Nucleic Acids Res 2012; 40:6241 - 54; http://dx.doi.org/10.1093/nar/gks242; PMID: 22434877
- Rajeswaran R, Pooggin MM. RDR6-mediated synthesis of complementary RNA is terminated by miRNA stably bound to template RNA. Nucleic Acids Res 2012; 40:594 - 9; http://dx.doi.org/10.1093/nar/gkr760; PMID: 21930511
- Moissiard G, Parizotto EA, Himber C, Voinnet O. Transitivity in Arabidopsis can be primed, requires the redundant action of the antiviral Dicer-like 4 and Dicer-like 2, and is compromised by viral-encoded suppressor proteins. RNA 2007; 13:1268 - 78; http://dx.doi.org/10.1261/rna.541307; PMID: 17592042
- Mlotshwa S, Pruss GJ, Peragine A, Endres MW, Li J, Chen X, Poethig RS, Bowman LH, Vance V. DICER-LIKE2 plays a primary role in transitive silencing of transgenes in Arabidopsis. PLoS One 2008; 3:e1755; http://dx.doi.org/10.1371/journal.pone.0001755; PMID: 18335032
- Vermeersch L, De Winne N, Depicker A. Introns reduce transitivity proportionally to their length, suggesting that silencing spreads along the pre-mRNA. Plant J 2010; 64:392 - 401; http://dx.doi.org/10.1111/j.1365-313X.2010.04335.x; PMID: 21049564
- Voinnet O, Baulcombe DC. Systemic signalling in gene silencing. Nature 1997; 389:553; http://dx.doi.org/10.1038/39215; PMID: 9335491
- Voinnet O, Vain P, Angell S, Baulcombe DC. Systemic spread of sequence-specific transgene RNA degradation in plants is initiated by localized introduction of ectopic promoterless DNA. Cell 1998; 95:177 - 87; http://dx.doi.org/10.1016/S0092-8674(00)81749-3; PMID: 9790525
- Dunoyer P, Brosnan CA, Schott G, Wang Y, Jay F, Alioua A, Himber C, Voinnet O. An endogenous, systemic RNAi pathway in plants. EMBO J 2010; 29:1699 - 712; http://dx.doi.org/10.1038/emboj.2010.65; PMID: 20414198
- Dunoyer P, Himber C, Ruiz-Ferrer V, Alioua A, Voinnet O. Intra- and intercellular RNA interference in Arabidopsis thaliana requires components of the microRNA and heterochromatic silencing pathways. Nat Genet 2007; 39:848 - 56; http://dx.doi.org/10.1038/ng2081; PMID: 17558406
- Dunoyer P, Schott G, Himber C, Meyer D, Takeda A, Carrington JC, Voinnet O. Small RNA duplexes function as mobile silencing signals between plant cells. Science 2010; 328:912 - 6; http://dx.doi.org/10.1126/science.1185880; PMID: 20413458
- Dunoyer P, Voinnet O. Mixing and matching: the essence of plant systemic silencing?. Trends Genet 2008; 24:151 - 4; http://dx.doi.org/10.1016/j.tig.2008.01.005; PMID: 18325623
- Melnyk CW, Molnar A, Bassett A, Baulcombe DC. Mobile 24 nt small RNAs direct transcriptional gene silencing in the root meristems of Arabidopsis thaliana. Curr Biol 2011; 21:1678 - 83; http://dx.doi.org/10.1016/j.cub.2011.08.065; PMID: 21962713
- Melnyk CW, Molnar A, Baulcombe DC. Intercellular and systemic movement of RNA silencing signals. EMBO J 2011; 30:3553 - 63; http://dx.doi.org/10.1038/emboj.2011.274; PMID: 21878996
- Molnar A, Melnyk CW, Bassett A, Hardcastle TJ, Dunn R, Baulcombe DC. Small silencing RNAs in plants are mobile and direct epigenetic modification in recipient cells. Science 2010; 328:872 - 5; http://dx.doi.org/10.1126/science.1187959; PMID: 20413459
- Brosnan CA, Mitter N, Christie M, Smith NA, Waterhouse PM, Carroll BJ. Nuclear gene silencing directs reception of long-distance mRNA silencing in Arabidopsis. Proc Natl Acad Sci U S A 2007; 104:14741 - 6; http://dx.doi.org/10.1073/pnas.0706701104; PMID: 17785412
- Axtell MJ, Jan C, Rajagopalan R, Bartel DP. A two-hit trigger for siRNA biogenesis in plants. Cell 2006; 127:565 - 77; http://dx.doi.org/10.1016/j.cell.2006.09.032; PMID: 17081978
- Sanders M, Maddelein W, Depicker A, Van Montagu M, Cornelissen M, Jacobs J. An active role for endogenous beta-1,3-glucanase genes in transgene-mediated co-suppression in tobacco. EMBO J 2002; 21:5824 - 32; http://dx.doi.org/10.1093/emboj/cdf586; PMID: 12411500
- Palauqui JC, Elmayan T, De Borne FD, Crete P, Charles C, Vaucheret H. Frequencies, Timing, and Spatial Patterns of Co-Suppression of Nitrate Reductase and Nitrite Reductase in Transgenic Tobacco Plants. Plant Physiol 1996; 112:1447 - 56; PMID: 12226457
- Wassenegger M, Pélissier T. Signalling in gene silencing. Trends Plant Sci 1999; 4:207 - 9; http://dx.doi.org/10.1016/S1360-1385(99)01416-8; PMID: 10366874
- Jones L, Ratcliff F, Baulcombe DC. RNA-directed transcriptional gene silencing in plants can be inherited independently of the RNA trigger and requires Met1 for maintenance. Curr Biol 2001; 11:747 - 57; http://dx.doi.org/10.1016/S0960-9822(01)00226-3; PMID: 11378384
- Dalakouras A, Moser M, Boonrod K, Krczal G, Wassenegger M. Diverse spontaneous silencing of a transgene among two Nicotiana species. Planta 2011; 234:699 - 707; http://dx.doi.org/10.1007/s00425-011-1433-9; PMID: 21617990
- Kalantidis K, Tsagris M, Tabler M. Spontaneous short-range silencing of a GFP transgene in Nicotiana benthamiana is possibly mediated by small quantities of siRNA that do not trigger systemic silencing. Plant J 2006; 45:1006 - 16; http://dx.doi.org/10.1111/j.1365-313X.2006.02664.x; PMID: 16507090
- Dadami E, Moser M, Zwiebel M, Krczal G, Wassenegger M, Dalakouras A. An endogene-resembling transgene delays the onset of silencing and limits siRNA accumulation. FEBS Lett 2013; 587:706 - 10; http://dx.doi.org/10.1016/j.febslet.2013.01.045; PMID: 23380068
- Schwach F, Vaistij FE, Jones L, Baulcombe DC. An RNA-dependent RNA polymerase prevents meristem invasion by potato virus X and is required for the activity but not the production of a systemic silencing signal. Plant Physiol 2005; 138:1842 - 52; http://dx.doi.org/10.1104/pp.105.063537; PMID: 16040651
- Vaistij FE, Jones L. Compromised virus-induced gene silencing in RDR6-deficient plants. Plant Physiol 2009; 149:1399 - 407; http://dx.doi.org/10.1104/pp.108.132688; PMID: 19129420
- Ruiz MT, Voinnet O, Baulcombe DC. Initiation and maintenance of virus-induced gene silencing. Plant Cell 1998; 10:937 - 46; http://dx.doi.org/10.1105/tpc.10.6.937; PMID: 9634582
- Gosselé V, Faché I, Meulewaeter F, Cornelissen M, Metzlaff M. SVISS - a novel transient gene silencing system for gene function discovery and validation in tobacco plants. Plant J 2002; 32:859 - 66; http://dx.doi.org/10.1046/j.1365-313X.2002.01471.x; PMID: 12472699
- Jones L, Hamilton AJ, Voinnet O, Thomas CL, Maule AJ, Baulcombe DC. RNA-DNA interactions and DNA methylation in post-transcriptional gene silencing. Plant Cell 1999; 11:2291 - 301; http://dx.doi.org/10.1105/tpc.11.12.2291; PMID: 10590159
- Daxinger L, Kanno T, Bucher E, van der Winden J, Naumann U, Matzke AJ, Matzke M. A stepwise pathway for biogenesis of 24-nt secondary siRNAs and spreading of DNA methylation. EMBO J 2009; 28:48 - 57; http://dx.doi.org/10.1038/emboj.2008.260; PMID: 19078964
- Palauqui JC, Elmayan T, Pollien JM, Vaucheret H. Systemic acquired silencing: transgene-specific post-transcriptional silencing is transmitted by grafting from silenced stocks to non-silenced scions. EMBO J 1997; 16:4738 - 45; http://dx.doi.org/10.1093/emboj/16.15.4738; PMID: 9303318
- McHale M, Eamens AL, Finnegan EJ, Waterhouse PM. A 22-nt artificial microRNA mediates widespread RNA silencing in Arabidopsis. Plant J 2013; 76:519 - 29; http://dx.doi.org/10.1111/tpj.12306; PMID: 23937661
- Bai S, Kasai A, Yamada K, Li T, Harada T. A mobile signal transported over a long distance induces systemic transcriptional gene silencing in a grafted partner. J Exp Bot 2011; 62:4561 - 70; http://dx.doi.org/10.1093/jxb/err163; PMID: 21652532
- Haque AK, Yamaoka N, Nishiguchi M. Cytosine methylation is associated with RNA silencing in silenced plants but not with systemic and transitive RNA silencing through grafting. Gene 2007; 396:321 - 31; http://dx.doi.org/10.1016/j.gene.2007.04.003; PMID: 17521830
- Mallory AC, Mlotshwa S, Bowman LH, Vance VB. The capacity of transgenic tobacco to send a systemic RNA silencing signal depends on the nature of the inducing transgene locus. Plant J 2003; 35:82 - 92; http://dx.doi.org/10.1046/j.1365-313X.2003.01785.x; PMID: 12834404
- Bender J. RNA-directed DNA methylation: getting a grip on mechanism. Curr Biol 2012; 22:R400 - 1; http://dx.doi.org/10.1016/j.cub.2012.04.010; PMID: 22625855
- Haag JR, Pikaard CS. Multisubunit RNA polymerases IV and V: purveyors of non-coding RNA for plant gene silencing. Nat Rev Mol Cell Biol 2011; 12:483 - 92; http://dx.doi.org/10.1038/nrm3152; PMID: 21779025
- Law JA, Jacobsen SE. Establishing, maintaining and modifying DNA methylation patterns in plants and animals. Nat Rev Genet 2010; 11:204 - 20; http://dx.doi.org/10.1038/nrg2719; PMID: 20142834
- Dalakouras A, Dadami E, Wassenegger M. Viroid-induced DNA methylation in plants. BioMol Concepts 2013; 4:557 - 65
- Dalakouras A, Wassenegger M. Revisiting RNA-directed DNA methylation. RNA Biol 2013; 10:453 - 5; http://dx.doi.org/10.4161/rna.23542; PMID: 23324611
- Wierzbicki AT, Haag JR, Pikaard CS. Noncoding transcription by RNA polymerase Pol IVb/Pol V mediates transcriptional silencing of overlapping and adjacent genes. Cell 2008; 135:635 - 48; http://dx.doi.org/10.1016/j.cell.2008.09.035; PMID: 19013275
- Wierzbicki AT, Ream TS, Haag JR, Pikaard CS. RNA polymerase V transcription guides ARGONAUTE4 to chromatin. Nat Genet 2009; 41:630 - 4; http://dx.doi.org/10.1038/ng.365; PMID: 19377477
- Christie M, Brosnan CA, Rothnagel JA, Carroll BJ. RNA decay and RNA silencing in plants: competition or collaboration?. Front Plant Sci 2011; 2:99; http://dx.doi.org/10.3389/fpls.2011.00099; PMID: 22639621
- Gy I, Gasciolli V, Lauressergues D, Morel JB, Gombert J, Proux F, Proux C, Vaucheret H, Mallory AC. Arabidopsis FIERY1, XRN2, and XRN3 are endogenous RNA silencing suppressors. Plant Cell 2007; 19:3451 - 61; http://dx.doi.org/10.1105/tpc.107.055319; PMID: 17993620
- Christie M, Carroll BJ. SERRATE is required for intron suppression of RNA silencing in Arabidopsis. Plant Signal Behav 2011; 6:2035 - 7; http://dx.doi.org/10.4161/psb.6.12.18238; PMID: 22112452
- Christie M, Croft LJ, Carroll BJ. Intron splicing suppresses RNA silencing in Arabidopsis. Plant J 2011; 68:159 - 67; http://dx.doi.org/10.1111/j.1365-313X.2011.04676.x; PMID: 21689169
- Kertész S, Kerényi Z, Mérai Z, Bartos I, Pálfy T, Barta E, Silhavy D. Both introns and long 3′-UTRs operate as cis-acting elements to trigger nonsense-mediated decay in plants. Nucleic Acids Res 2006; 34:6147 - 57; http://dx.doi.org/10.1093/nar/gkl737; PMID: 17088291
- Howell MD, Fahlgren N, Chapman EJ, Cumbie JS, Sullivan CM, Givan SA, Kasschau KD, Carrington JC. Genome-wide analysis of the RNA-DEPENDENT RNA POLYMERASE6/DICER-LIKE4 pathway in Arabidopsis reveals dependency on miRNA- and tasiRNA-directed targeting. Plant Cell 2007; 19:926 - 42; http://dx.doi.org/10.1105/tpc.107.050062; PMID: 17400893
- Horsch R, Fry J, Hoffmann N, Eichholz D, Rogers S, Fraley R. A simple and general method for transferring genes into plants. Science 1985; 227:1229 - 31; http://dx.doi.org/10.1126/science.227.4691.1229; PMID: 17757866
- Dalakouras A, Moser M, Zwiebel M, Krczal G, Hell R, Wassenegger M. A hairpin RNA construct residing in an intron efficiently triggered RNA-directed DNA methylation in tobacco. Plant J 2009; 60:840 - 51; http://dx.doi.org/10.1111/j.1365-313X.2009.04003.x; PMID: 19702668
- Hetzl J, Foerster AM, Raidl G, Mittelsten Scheid O. CyMATE: a new tool for methylation analysis of plant genomic DNA after bisulphite sequencing. Plant J 2007; 51:526 - 36; http://dx.doi.org/10.1111/j.1365-313X.2007.03152.x; PMID: 17559516
- Helm JM, Dadami E, Kalantidis K. Local RNA silencing mediated by agroinfiltration. Methods Mol Biol 2011; 744:97 - 108; http://dx.doi.org/10.1007/978-1-61779-123-9_7; PMID: 21533688
- Dalakouras A, Dadami E, Zwiebel M, Krczal G, Wassenegger M. Transgenerational maintenance of transgene body CG but not CHG and CHH methylation. Epigenetics 2012; 7:1071 - 8; http://dx.doi.org/10.4161/epi.21644; PMID: 22863736