Abstract
The localization of mRNAs to specific subcellular sites is widespread, allowing cells to spatially restrict and regulate protein production, and playing important roles in development and cellular physiology. This process has been studied in mechanistic detail for several RNAs. However, the generality or specificity of RNA localization systems and mechanisms that impact the many thousands of localized mRNAs has been difficult to assess. In this review, we discuss the current state of the field in determining which RNAs localize, which RNA sequences mediate localization, the protein factors involved, and the biological implications of localization. For each question, we examine prominent systems and techniques that are used to study individual messages, highlight recent genome-wide studies of RNA localization, and discuss the potential for adapting other high-throughput approaches to the study of localization.
Introduction
Eukaryotic gene expression is regulated in both time and space. Studies focused on temporal regulation have benefited from the use of a variety of high-throughput genomic technologies for analysis of changes in transcripts, translation status and chromatin during cell state transitions and development.Citation1-3 Subcellular localization of a transcript can also have profound effects on a transcript's activity, but with several recent exceptions has usually been studied one transcript at a time. In this review we discuss central questions in the field of RNA localization and highlight recent studies that are starting to use genome-wide strategies to address them.
Importance of RNA localization
RNA localization has been linked to many important phenotypes. Two interesting and well-characterized cases involve the localization of oskar RNA in the Drosophila embryo and β-actin RNA in vertebrate fibroblasts.
In its early stages, the developing Drosophila embryo is a syncytium. Proper patterning of the embryo relies on the presence of distinct gradients of proteins within the embryo. Germ plasm develops at the posterior end of the embryo and its formation is dependent on a high concentration of Oskar protein produced from localized oskar RNA.Citation4 Mis-localization of oskar RNA to the anterior pole results in the formation of germ plasm at the anterior end.Citation5 Interestingly, proper localization of oskar RNA is dependent on the splicing of its first intron, suggesting a link between splicing and localization,Citation6 discussed further below.
In vertebrate fibroblasts, the leading edges of the cell are dense with actin. This is due, in part, to the localization of mRNA coding for β-actin to the leading edges.Citation7 This localization is necessary for proper cell motility, and inhibition of recognition of the sequences that regulate localization result in the loss of cell polarity and motility.Citation8-10
In a general sense, RNA localization provides the foundation for a rapid, robust response to a stimulus (). While protein transport to the subcellular site of interest could, and often does, provide a similar output, localization of the mRNA molecule obviates the need for a drawn-out signal transduction process. Additionally, since several protein molecules can be made from a single mRNA molecule, the response can also be more vigorous. Although the majority of work in the field has focused on the localization of mRNAs, newer work has also demonstrated that long noncoding RNAs (lncRNAs) are often subject to specific subcellular localization as well.Citation11,12
Figure 1. General scheme of mRNA localization. The formation of a splicing-dependent stem-loop and involvement of the EJC is seen in the localization of the transcript oskar in Drosophila embryos. After splicing, mRNAs are exported from the nucleus. Those containing mRNA localization signals, in this case, the stem-loop, are recognized by trans-factors that interact with the localization signal. The trans-factor is integrated into a transport complex that uses motor proteins to move the RNA to its destination. Localized RNAs are often kept in a translationally-repressed state until they reach their destination, whereupon they are released and may be translated to give a localized pool of protein product.
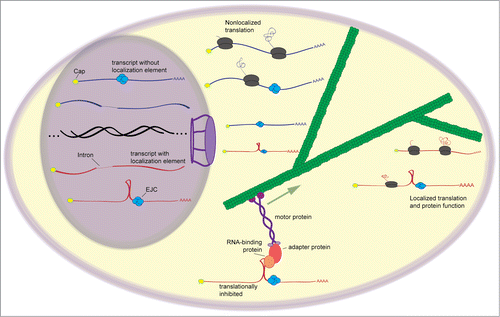
RNA localization systems
RNA localization is often studied in systems where there is clear functional specialization and the level of localization is likely to be robust. Projections of mammalian neurons, both dendrites and axons, are commonly used.Citation13,14 The bud tip is a well-studied example in S. cerevisiae as ASH1 mRNA localizes there during mating type switching.Citation15,16 The high concentration of ASH1 mRNA in the bud tip results in high expression of Ash1p, a protein that represses transcription of the mating type regulator HO. Syncytial fly embryos are often used, as RNA localization to specific places in the embryo is important for proper body patterning and development, and the distance from one pole of the embyro to the other is quite large.Citation4,5 RNAs may also localize to organelles within the cell. Mitochondria are frequently studied as targets for RNA localization,Citation17-19 as are the cytoskeletonCitation20,21 and leading edges of fibroblasts.Citation7
This review is organized around four central questions in RNA localization: (1) which RNAs are localized? (2) What specific mRNA sequences mediate localization? (3) What protein factors contribute to RNA localization? (4) How does RNA localization impact translation or other biological outcomes? In each section, we review state of the art molecular genetic and cell biological methods and discuss emerging genomic approaches for answering each question.
Identifying Localized RNAs
A primary question in the field is to determine which RNAs specifically localize within cells. The current approaches to this question can provide robust and accurate answers but generally require that each RNA be interrogated separately.
Localized RNAs: Single-gene approaches
The most common technique for interrogating RNA localization is RNA fluorescent in situ hybridization (RNA FISH) ().Citation7 By hybridizing many labeled probes to a single RNA, this method has been improved to allow single molecule sensitivity.Citation22,23 Although this technique has been successfully used in a variety of settings, including whole organisms,Citation22 it requires that samples be fixed before probing and is thus not compatible with living cells or organisms.
Figure 2. Classical and genomic approaches for studying RNA localization. (A) RNA FISH images from three systems. RNA encoding β-actin is localized to the leading edges of chicken fibroblasts,Citation124 while ASH1 mRNA localizes to the bud tip of S. cerevisiae during mating type switching.Citation125 RNA encoding odd and the resulting protein product accumulate in distinct segments in the developing Drosophila embryo and are important for proper development and morphology.Citation35 (B) Fractionation of cells followed by high-throughput sequencing can give a more generalized view of RNA localization.Citation21 Here, mouse C2C12 myoblasts were treated first with digitonin, which gently lyses cells but keeps membranes generally intact. Cytoplasmic RNA is collected and the cells are then treated with Triton X-100 to solubilize membranes. Membrane-associated RNA is collected and the resulting sample is treated with high salt to elute RNA associated with insoluble cell components. RNA from each fraction is then analyzed by RNA-seq. The location of activity of the protein encoded by each RNA generally match with the location of the RNA. (C) Method overview of FISSEQ.Citation46 Cellular structures are crosslinked followed by in situ reverse transcription of the crosslinked RNA using random primers and modified nucleotides that allow further crosslinking. The resulting cDNA is crosslinked to cellular structures and the circularized and amplified using rolling circle amplification. cDNA amplicons are then crosslinked to cellular structures and each other and sequenced in situ using SOLiD sequencing.
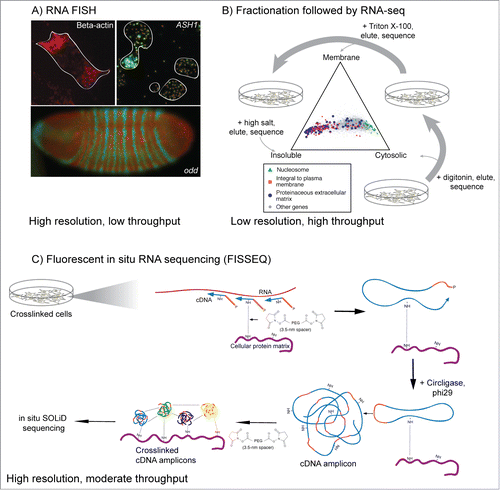
Imaging RNAs in live cells is typically done through the use of an RNA binding protein fused to a fluorescent protein. A commonly used system is fusion of the viral coat protein MS2, which binds to a specific RNA hairpin structure. When this hairpin is appended to an RNA of interest, usually in the 3′ UTR, and coexpressed with an MS2-GFP fusion, GFP fluorescence can be used to track RNA localization in vivo.Citation24 This technique has since been improved and expanded to allow visualization in live mice.Citation25 While this method allows the monitoring of dynamics of RNA localization in vivo, it is still limited to the study of one or a few RNAs at a time.
Other methods include the use of molecular beacons. These probes consist of a fluorescent molecule that is held next to a quencher by basepairing.Citation26 When the loop of sequence outside of the stem hybridizes to an RNA of interest, it forces the fluorescent probe and quencher apart, allowing visualization of the RNA.Citation27 By combining biotin-conjugated fluorescent probes with tetravalent streptavidin, Alonas and colleagues were able to visualize single RNA molecules in developing viruses.Citation28
As an alternative to tracking the localization of a single RNA, others have taken the approach of fractionating cells and cloning the resulting RNA into cDNA libraries. An early study cloned RNAs present in processes of rat hippocampal cells in culture, identifying several RNAs involved in neuronal signalingCitation29-31 and even budded synpatoneurosomes.Citation32 Giant neurites from the sea slug Aplysia have also been analyzed using this approach.Citation33 Sequencing of several hundred clones from this library revealed an enrichment for RNAs encoding elements of the translational machinery as well as cytoskeletal components. A similar fractionation of rat dorsal root ganglia (DRG) using a compartmental culture system yielded RNA from axonsCitation34 and was analyzed by quantitative RT-PCR to test for the presence of several target transcripts in the axon. Although such approaches have less precision in terms of location than imaging-based methods, their increased gene sampling allows a richer picture of localized RNAs.
Localized RNAs: Genomic approaches
Large scale FISH
Some groups have taken existing techniques and found ways to automate them, approaching genome-wide scale. A classic example was the “Fly-FISH” study by Lécuyer and colleagues.Citation35 In this study, the established RNA FISH technique was scaled up using hybridizations in 96-well plates to examine the localization patterns of over 3000 genes in the developing Drosophila embryo. Surprisingly, over 70% of the analyzed genes showed distinct, nonuniform localization patterns, demonstrating unexpected and prevalence and diversity of RNA localization.
Microarray-based approaches
In a similar extension, several groups used microarrays to study differential enrichment of RNA species between two cellular compartments. Marc and colleagues isolated polysomes from both cytoplasm and mitochondria-enriched fractions.Citation17 Interestingly, transcripts encoding mitochondrial proteins of bacterial origin were more often associated with the mitochondria than were those of eukaryotic origin. Many of these mitochondrial-associated transcripts encoded proteins with transmembrane domains, suggesting a connection between RNA localization to the surface of the mitochondria and efficient import and structuring of membrane-embedded proteins.Citation36 This microarray-based approach was also applied to other systems. RNA from protruding pseudopodia of mouse fibroblasts was enriched for approximately 50 transcripts,Citation37 some of which were associated with fragile X mental retardation protein (FMRP).
Several studies have fractionated neurons and used microarrays to analyze RNAs present in the distal processes vs. the cell bodies. Poon and colleagues used pore-containing culture membranes to separate distal projections from cell bodies in cultured rodent hippocampal neurons.Citation38 Using microarrays, they identified over 100 projection-localized RNAs, many of which were associated with translation. In mammalian cortical neurons, over 300 transcripts were identified as enriched in projections using microarrays, with many of these transcripts coding for proteins with translational and mitochondrial functions.Citation39 A similar transcriptomic analysis of rat DRG axons revealed the presence of mRNAs from almost 3000 genes in the axons.Citation40 The RNA content of neuronal projections can also change in response to injury, stimulation or during development.Citation39
The RNA content of even smaller cellular structures can also be interrogated, although the reduced RNA yield from these structures often necessitates that the RNA be amplified prior to measurement. For example, RNA collection and amplification from laser dissected growth cones at the tips of neurites revealed that they, too, contain many different RNA species, again with an enrichment for RNAs involved in translation.Citation41
RNA-seq-based approaches
Following developments in high-throughput sequencing, later experiments used RNA-seq to measure expression. Minis and colleagues used similar membrane-based fractionation techniques to separate DRG axons and cell bodies,Citation42 and found transcripts encoding mitochondrial proteins as well as specific transcription factors enriched in the axon fraction.
Cajigas and colleagues further probed the transcriptome of neural projections using Roche/454 sequencing of sections of rat hippocampal neuropil,Citation43 which are generally depleted of cell bodies and enriched in dendrites and axons. Over 2500 transcripts were identified as present in dendrites and/or axons and were enriched for functions including neurotransmitter secretion and synaptic vesicles. This study also demonstrates a key issue in observing localization with tissue dissections: the cleanliness and specificity of the “localized” fraction. Although the neuropil is enriched for projections, it also contains other components. Using a titration strategy in which RNA from other components was doped in, Cajigas and coworkers were able to identify transcripts specifically enriched in the projections.
RNA-seq has also been applied to study localization in other systems. In X. laevis extracts, taxol-stabilized microtubules were isolated by sedimentation. Microtubule-associated RNAs were shown to be enriched in transcripts involved in cell division and spindle formation.Citation44 Fractionation of mouse C2C12 myoblast cells and Drosophila S2 cells into cytosolic, membrane-associated, and insoluble fractions revealed transcripts enriched in each compartment (). The localization patterns of transcripts were generally associated with the functions of the proteins they encoded. For example, nucleosomal and other nuclear protein mRNAs were enriched in the cytosolic compartment, while mRNAs encoding membrane and secreted proteins tended to be enriched in insoluble and membrane compartments.Citation21 In another study, developing Drosophila embryos were cyrosectioned into 60 μm slices and analyzed by RNA-seq, recapitulating RNA localization patterns previously defined by FISH.Citation45 This study puts forward an interesting approach that could pave the way for improved resolution in future studies.
Newer approaches may obviate the need for fractionation altogether. By fixing RNA and then reverse transcribing, amplifying, and sequencing it in situ in the cell, Lee and colleagues have combined the resolution of imaging techniques with the depth and throughput of sequencing-based approachesCitation46 (). This method, named FISSEQ, performs all enzymatic steps necessary for sequencing at the site of the RNA transcript. Combining imaging and sequencing in this way may provide powerful new tools for the future study of RNA localization.
A more complete understanding of RNA sequences involved in localization may have implications in human disease as well. Some factors known to be involved in RNA localization like FMRP and muscleblind already have known disease associations, but the extent to which RNA localization is mis-regulated in these diseases is unknown. The identification of RNAs that localize to particular cell compartments may contribute to explanations of disease phenotypes and provide insights into potential modes of treatment.
Identifying RNA Elements that Direct Localization
RNA localization is mediated by cis-elements, which link the RNA to protein complexes that may 1) associate with cytoskeletal elements for subsequent intracellular transport, 2) preferentially stabilize or degrade the RNA in particular subcellular compartments, or 3) tether or anchor the RNA to a particular locations. These cis-elements may be composed of linear sequence motifs or structures.
Localization elements: Molecular genetic approaches
To date, most cis-elements that direct RNA localization have been identified using a combination of image-based and biochemical assays. Commonly, reporters encoding the putative localization element are introduced into a model system of interest, and deletions/mutations are generated to alter localizing activity. Sequential experiments are often required to pinpoint the localizing element with high precision. For example, initial studies describing -actin mRNA at leading edges of fibroblasts used the entire 3′ UTR to demonstrate localizing activity.Citation47 Subsequent studies narrowed the region to 54 nucleotides,Citation8 culminating in a definition 5-mer and 3-mer with specific spacing requirements, such that each short motif causes the RNA to loop around the localization factor zipcode binding protein 1 (Zbp1).Citation48
While the majority of known localizing elements reside in 3′ UTRs, some occur in 5′ UTRs,Citation49 or within mature, spliced coding sequence. In some instances, particular elements required for localization extend beyond a simple cis-element, and require elements acquired during mRNA processing. For example, localization of oskar mRNA to the posterior pole of the Drosophila embryo requires both a 3′ UTR cis-element, as well as an exon junction complex deposited adjacent to a 28-nucleotide motif generated by splicing.Citation6,50
Finally, a novel approach that linked cell survival to RNA localization was used to identify a 50-nucleotide motif sufficient to localize reporter mRNAs to mitochondria.Citation51 Localization of the ATP2 mRNA to yeast mitochondria is required for proper respiratory function, and provided an assay for a recombination-based screen in which random fragments derived from the ATP2 3′ UTR were used to rapidly screen for localizing activity.
Localization elements: Genomic approaches
Increasingly, genomic approaches are being taken to identify cis-elements involved in RNA localization. In general, an important challenge is to separate cellular compartments in a manner that maximizes spatial resolution, while yielding material suitable for genomic techniques such as microarrays or deep sequencing. Neuron cell bodies have been separated from their projections in rodent and Aplysia systems, typically using membrane filters with passages large enough to allow only projections to pass through,Citation38 by laser capture,Citation41 or by microfluidic isolation.Citation40,52
Following identification of transcripts with biased localization, motif analysis has been performed to identify sequence features enriched in particular regions of those transcripts, or sequences that may be conserved between species. Because cis-elements controlling localization often involve RNA secondary structure,Citation53-56 some may be missed by approaches that search for enrichment or conservation of linear sequences. However, structure-aware algorithms have been developed to aid in the search,Citation57 some of which rely on co-evolution of residues that may be involved in secondary structure formation.Citation58
Future work that further increases spatial resolution may be productive in the search for localizing elements. For example, subcellular fractionation by differential centrifugation has been useful in identifying protein complexes,Citation59,60 and may also be useful for identifying RNAs localized to subcellular compartments, provided that the fractionation procedure does not significantly disrupt interactions between RNA and its associated complexes.
Approaches could also be borrowed from the splicing field where a library of random sequences is selected for a particular function.Citation61,62 For purposes of RNA localization, a randomized sequence could be introduced into the 3′ UTR of a reporter gene, or endogenous gene, using genome engineering approaches. Given a strategy for isolating localized RNAs, high throughput sequencing could then be used to identify RNA elements associated with a particular subcellular location. Numerous variations on this screening methodology can be performed, for example to study different cell types, or different classes of transcripts, e.g., those with signal sequences, genes of varying expression level, or genes with alternative splicing. Following appropriate biochemical and image-based confirmation of cis-element activity, proteins that can interact with active cis-elements can be identified using pulldown/immunoprecipitation-based approaches.
The successful application of deep sequencing to these questions should accelerate the discovery of RNA localizing cis-elements. We now have improved sensitivity to detect transcripts localized to subcellular compartments and the depth of coverage to survey synthetic libraries of cis-elements in an unbiased manner.
Protein Factors Involved in RNA Localization
Mechanisms of RNA localization
In a general sense, selective enrichment of RNA molecules in specific cellular locations occurs through one of three different mechanisms. In the first, RNA is stabilized in one location and degraded in others. In the Drosophila embryo, hsp83 and nanos mRNA are degraded throughout the embryo except for the posterior pole where they are protected from degradation by elements in their 3′ UTR.Citation63,64 Second, mRNAs passively diffusing through the cytoplasm can be bound and retained by anchoring proteins that are themselves localized to specific cellular sites. This system has been shown to function in at least two different systems: Xenopus oocytesCitation65 with the RNAs Xcat2 and Xdazl and Drosophila embryos with nanos mRNA.Citation66 Finally, RNAs can also be actively directed to a particular location. Sequence elements that influence active localization often function through recognition of the element by a specific RNA binding protein (RBP) and subsequent targeting of the RNA to localization RNPs. These RNPs, often containing motor proteins like kinesin and dynein, are then transported along the cytoskeletal infrastructure to their destinations.
Efforts to identify a more complete list of all RNA binding proteins through RNA purification and mass spectrometry identified almost 800 proteins with the capacity to bind RNA.Citation67 Further work is needed to determine which are involved in RNA localization, but the pool of potential localization factors is clearly quite diverse.
Localization factors: Biochemical and molecular genetic approaches
A number of proteins have been found to interact with a localized RNA and be important for its efficient transport. We will focus on three of the best known: Zbp1, staufen, and fragile X mental retardation protein (FMRP), and present proteomic strategies for identifying new components of localization RNPs.
Zbp1
The mRNA encoding β-actin contains in its 3′ UTR a 54-nucleotide element, termed a zipcode, that is necessary for its localization to the cellular periphery.Citation7-9 To identify the factor responsible for binding this element, a biochemical approach was taken in which the factor was affinity-purified from a complex extract using probes of the zipcode sequence as bait.Citation68 This study identified Zbp1 as the primary factor bound to the zipcode. Zbp1 was later shown to be functionally relevant as RNAi-mediated knockdown of its mammalian homologs resulted in defects in cell morphology.Citation69 Later studies demonstrated the importance of Zbp1-mediated β-actin mRNA localization in other systems, in particular neurons and neuronal growth cones.Citation14,Citation70-72
Staufen
In contrast to the biochemical identification of Zbp1, staufen was first identified through a genetic screen for Drosophila in which pole cell formation and pattern formation across the embryo was inhibited.Citation56 Staufen was later shown to be required for proper transport and localization of bicoid and oskar RNA in the embryo and to require specific sequences in the 3′ UTR of bicoid in order to direct its localization.Citation53,73 In adult flies, staufen is important in many neuronal processes, including long-term memory formation.Citation74,75
In mammals, there are two staufen paralogs. They are involved in RNA transport in neurons, particularly to dendrites.Citation55,76 Expression of a dominant negative form of staufen results in inhibition of RNA transport to dendrites,Citation77 and neurons from knockout mice are deficient in dendritic delivery of RNPs while the mice themselves show defects in locomotor activity.Citation78 Mammalian staufen has four double-stranded RNA binding domains, although not all of them have strong double-stranded RNA binding activity.Citation54 Besides a preference for double-stranded RNA, staufen has long been thought to be relatively nonspecific in its RNA binding, but recent work has demonstrated that staufen may in fact prefer distinct double-stranded structures.Citation79
Fragile X mental retardation protein
The fragile X mental retardation protein (FMRP) was identified through its location in a fragile site on the X chromosome associated with mental retardation.Citation80 The FMRP transcript contains a CGG trinucleotide repeat in its 5′ UTR. When this repeat is expanded, the gene becomes methylated and transcriptionally silenced.Citation81,82
In mammalian neurons, FMRP is localized to spines and growth cones, while also localizing with a distinct population of RNAs.Citation83,84 This localization to dendrites is particularly robust in response to depolarization.Citation85 In addition, FMRP colocalizes with ribosomes and is believed to be involved in translational repressionCitation83 as neurons from FMRP knockout mice show high basal levels of translation but are deficient in translation in response to a stimulus.Citation86 In response to stimulation, FMRP regulates localization of its targets as well as their translation as FMRP targets are increased in dendritic cultures after stimulation, but not in FMRP knockout neurons.Citation87
Localization factors: Genome-wide approaches
Each of the proteins discussed above was identified individually. As new approaches to nucleic acid sequencing allow richer and more complete pictures of localized transcriptomes, newer proteomic approaches allow more complete characterization of localization RNPs, their components, and their RNA cargos.
Identification of trans factors
Efforts to identify and characterize localization RNPs have mainly centered around strategies of RNP purification whereby a known component of an RNP is purified from lysate and copurifying proteins are identified by mass spectrometry. One of the first studies to do this with an eye toward RNA localization used this strategy with a GST-tagged fragment of the kinesin Kif5 and lysate from mouse brain.Citation88 Over 40 proteins, including FMRP and Staufen, copurified, as well as the known dendritically-localizing RNAs CaMKII-α and Arc. RNAi knockdown of some of these proteins inhibited dendritic transport of reporter RNAs.
A similar study using IMP1, a mammalian ortholog of ZBP1, as the purification bait identified hnRNP proteins and ribosomal proteins as RNP components.Citation89 An identification of the interactors of staufen2 also identified ribosomal proteins and cytoskeletal components.Citation90 Fritzsche and colleagues analyzed the interacting partners of two proteins, barentsz and staufen2, finding several proteins in common between the two but also a large number of differences, implying that the composition of different localization RNP particles may be more diverse than expected.Citation91 Several translational repressors were found in both complexes, consistent with the idea that transported RNA molecules are translationally repressed while they are in transit.Citation92 Interestingly, RNA-induced silencing complex (RISC) components were also found in these particles, raising the possibility of location-specific small RNA-mediated regulation in these cells.
Identification of trans-factor binding sites
Currently, a determination of the binding sites of RNA binding proteins generally involves in vivo crosslinking of proteins to RNA using UV irradiation, followed by purification of the protein of interest and sequencing of the associated RNAs.Citation93-95 Such approaches, with variations, have been undertaken for many proteins involved in other aspects of RNA metabolism, including alternative splicing, and have recently been applied to proteins involved in RNA localization.
Staufen and FMRP have been particularly studied using such methods. FMRP has been proposed to bind a G-quartet RNA structure.Citation96,97 Immunoprecipitation of FMRP from mouse brain lysates followed by microarray analysis of the coimmunoprecipitated RNA revealed over 400 potential RNA binding targets of FMRP.Citation84 Many of these messages were translationally misregulated in the absence of FMRP and also contained putative G-quartet structures. A later PAR-CLIPCitation98 based study identified two independent preferred motifs for FMRP from sequences immunoprecipitated with FMRP.Citation99 Biochemical analyses demonstrated that these motifs corresponded to the sites bound by two independent RNA-binding KH2 domains.
Identifying targets of staufen and the rationale behind its binding has been less straightforward. Staufen binds to double-stranded RNA, but whether it has specificity for particular sequences or structural motifs is unclear. RNA immunoprecpitation followed by microarray (RIP-CHIP) analysis of staufen targets in Drosophila embryos revealed a pattern of staufen binding. 3′ UTRs of genes bound by staufen tended to be long and contain structured regions of particular combinations of basepaired regions and loops,Citation79 providing evidence for specificity in binding. A similar analysis in C. elegans demonstrated that the worm ortholog, STAU-1, also possesses an ability to bind double-stranded RNA while retaining a specificity for distinct transcripts in vivo.Citation100 Analyses of human Stau1 and Stau2 by RIP-CHIP showed that there may be few mRNA targets in common between the two human staufen homologs,Citation101 and a separate study showed a possible role for staufen in neural lineage progression by promoting asymmetric segregation of some target RNAs.Citation102 RIP-CHIP of staufen from rat brain again demonstrated an RNA-binding specificity for staufen, with an enrichment for long 3′ UTRs and stem structures at least 12 base pairs long and containing no more than 2 unpaired bases.Citation103 However, since the studies above identify whole transcripts bound by staufen, they give comparatively little immediate data on the direct binding sites of the protein.
In a variant of RIP called RIPiT in which proteins are immunoprecipitated after formaldehyde crosslinking,Citation104 binding sites are narrowed from a search space of an entire transcript to a short RNA fragment that is protected from nuclease digestion by the bound proteins.Citation105 In these experiments, Stau1 is observed associating with translating ribosomes and long, stable secondary structures such as inverted Alu pairs. The authors argue that it is the propensity to form double-stranded regions, both in the coding sequence and 3′ UTR, that is the main driver of Stau1 binding.
CLIP experiments have also been performed on SMN, which is involved in snRNP biogenesis, but also colocalizes with RNAs in granules associated with mRNA transport in neurons.Citation106 These experiments identified hundreds of targets of SMN, many of which were previously shown to localize to axons. Furthermore, the axonal localization of many transcripts bound by SMN decreased upon depletion of SMN, implicating SMN in the trafficking and localization of these transcripts. Thus, defective RNA transport may contribute to pathogenesis in spinal muscular atrophy, a disease caused by mutations in the SMN1 gene.
These experiments are some of the first to take a genome-wide approach to identification of the RNA binding sites of localization factors. As more high-resolution binding data become available for more factors, it will be interesting to begin to cross-reference binding sites with sequences of localized transcripts to begin to piece together a more mechanistic understanding of the regulatory principles of RNA localization. Some localized transcripts may be bound by multiple factors, either concurrently or sequentially, raising the possibility of combinatorial effects. The identification of a larger number of binding sites for known localization factors will inform us about the accuracy of the canonical examples of their binding are as well as give us better characterizations of the sequences required for localization.
Localized Translation and Localization of Proteins
Of course, localization of the RNA itself is commonly not the end of the story. Translation of messages is often repressed while it is in transit and may only be activated in response to a specific stimulus. Well-established methods have been developed to study localized translation, but these are limited to the interrogation of only a few genes at a time and usually use reporters, rather than native transcripts. In this section we will summarize these current methods and highlight recent techniques that examine localized translation on a genome-wide scale.
Localized translation and protein localization: Imaging and proteomic approaches
Localized translation
In a sense, almost all methods used to analyze localized translation address one basic problem. Namely, how can a molecule of protein produced in situ in a particular cellular locale be distinguished from molecules made elsewhere that diffuse to the same location? Several methods have been devised that simply and elegantly answer this question using fluorescence-based reporters.
Two successful methods using the translation of fluorescent proteins involve destabilized and anchored proteins. GFP variants that are destabilized to reduce their half-life to a few hoursCitation107,108 have been used to identify newly synthesized proteins with the logic that older ones would be degraded before they could diffuse to the site of interest.Citation109,110 GFP molecules that contain a signal sequence for acylation, usually myristoylation, result in the newly translated GFP being inserted into the cell membrane shortly after its translation.Citation111 This setup has been used to measure the translation of reporter constructs in dendrites and axons in response to stimuliCitation112 and as a proxy for identifying the localization of the mRNA as well as the site of translation.Citation110
Other approaches ablate or modify the spectral properties of the molecule. Fluorescence recovery after photobleaching (FRAP) based approaches remove fluorescence from existing GFP molecules and then wait for the appearance of signal from newly translated GFP molecules at the site of interest before molecules translated elsewhere arrive by diffusion. For example, the appearance of signal in axons on a short timescale (∼20 min) is interpreted as evidence that the new signal is due to locally translated GFP.Citation112,113 Similarly, by converting the fluorescence emitted from photoconvertible GFP molecules from green to red, the appearance of new molecules of protein can be analyzed. This technique has been used to monitor the axonal translation of proteinCitation114 and the retrograde movement of protein from growth cones back toward the cell body.Citation115
Proteomics of organelles
Subcellular organelles often have proteomic environments distinct from the rest of the cell. Several groups have undertaken efforts to purify certain organelles using differential sedimentation and, using mass spectrometry, determine the proteins contained within them. Over 1000 proteins were identified as present in the Golgi and secretory pathway in rat liver homogenates.Citation116 Similarly, several hundred proteins were seen in synaptic vesicles prepared from rat brain,Citation117 and many more in mitochondria.Citation118 Yoon and colleagues used non-canonical amino acids that could be labeled with a fluorescent dye using Click chemistry. These amino acids are incorporated into newly synthesized proteins. By separating axons from cell bodies and culturing them on their own, the appearance of new proteins in the axonal proteome in response to stimuli could be measured on 2D-gels and by mass spectrometry.Citation119 Although these approaches have identified a rich diversity of proteins in several cellular structures, they rely on rigorous and clean biochemical methods to purify these organelles and structures. An alternative strategy instead relies on a localized enzyme that has the ability to tag nearby proteins and allow their efficient purification. By targeting an enzyme that has the ability to convert biotin from an inactive to an active form to the mitochondria, Rhee et al. purified several hundred proteins with a high degree of spatial precision.Citation120
Localized translation: Genome-wide approaches
Although the above organelle-based methods have been highly successful in identifying local proteomes, they are limited to answering questions about which proteins reside in a particular area, not where they are produced. Recent advances in identifying RNA molecules that are in a state of active translation have allowed a more global understanding into the translational output of a cell at any one time.Citation121 These methods include ribosome footprint profiling in which RNA fragments protected from RNase digestion by ribosomes are sequenced and polysome-sequencing in which mRNA molecules that co-sediment with polysomes are sequenced. By applying these techniques to ribosomes localized to specific parts of the cell, new insights have been gained into the spatial nature of translational regulation.
Fractionation of HEK293 cells into cytosolic and ER-associated fractions followed by ribosome footprint profiling revealed that although messages encoding cytosolic transcripts can be translated on ER-associated ribosomes, mRNAs that were associated with the ER were more highly translated than those in the cytosolic compartment.Citation122 Similar experiments in mouse myoblasts showed that the translational activity of mRNAs can be altered by perturbations that change their subcellular localization without affecting their overall abundance.Citation21 The translational status of localized messages is also likely to be influenced by bound protein factors.Citation91
These findings hint at possible broad differences in translation efficiency between different cellular compartments. Only a handful of cellular locations have been probed using approaches that monitor translation globally. However, previous, more targeted work has demonstrated many instances of translational regulation during mRNA localization,Citation84,123 making this a promising area for future global analysis. Further work in this area is needed to determine the extent to which translation is spatially regulated.
Conclusion
Although genomic techniques have been around for many years, they have only recently become sensitive enough to be efficiently applied to RNA localization. Studies in transcription and splicing have benefited from genomic insights, and future studies in RNA localization may also benefit from applying these methods. The diversity of systems and biological processes associated with RNA localization may also provide new opportunities for the application of high-throughput methods.
Disclosure of Potential Conflicts of Interest
No potential conflicts of interest were disclosed.
References
- Graveley BR, Brooks AN, Carlson JW, Duff MO, Landolin JM, Yang L, Artieri CG, van Baren MJ, Boley N, Booth BW, et al. The developmental transcriptome of Drosophila melanogaster. Nature 2011; 471:473-9; PMID:21179090; http://dx.doi.org/10.1038/nature09715
- Bazzini AA, Lee MT, Giraldez AJ. Ribosome profiling shows that miR-430 reduces translation before causing mRNA decay in zebrafish. Science 2012; 336:233-7; PMID:22422859; http://dx.doi.org/10.1126/science.1215704
- Smith ZD, Chan MM, Mikkelsen TS, Gu H, Gnirke A, Regev A, Meissner A. A unique regulatory phase of DNA methylation in the early mammalian embryo. Nature 2012; 484:339-44; PMID:22456710; http://dx.doi.org/10.1038/nature10960
- Ephrussi A, Dickinson LK, Lehmann R. Oskar organizes the germ plasm and directs localization of the posterior determinant nanos. Cell 1991; 66:37-50; PMID:2070417; http://dx.doi.org/10.1016/0092-8674(91)90137-N
- Ephrussi A, Lehmann R. Induction of germ cell formation by oskar. Nature 1992; 358:387-92; PMID:1641021; http://dx.doi.org/10.1038/358387a0
- Hachet O, Ephrussi A. Splicing of oskar RNA in the nucleus is coupled to its cytoplasmic localization. Nature 2004; 428:959-63; PMID:15118729; http://dx.doi.org/10.1038/nature02521
- Lawrence JB, Singer RH. Intracellular localization of messenger RNAs for cytoskeletal proteins. Cell 1986; 45:407-15; PMID:3698103; http://dx.doi.org/10.1016/0092-8674(86)90326-0
- Kislauskis EH, Zhu X, Singer RH. Sequences responsible for intracellular localization of beta-actin messenger RNA also affect cell phenotype. J Cell Biol 1994; 127:441-51; PMID:7929587; http://dx.doi.org/10.1083/jcb.127.2.441
- Shestakova EA, Wyckoff J, Jones J, Singer RH, Condeelis J. Correlation of beta-actin messenger RNA localization with metastatic potential in rat adenocarcinoma cell lines. Cancer Res 1999; 59:1202-5; PMID:10096548
- Katz ZB, Wells AL, Park HY, Wu B, Shenoy SM, Singer RH. β-Actin mRNA compartmentalization enhances focal adhesion stability and directs cell migration. Genes Dev 2012; 26:1885-90; PMID:22948660; http://dx.doi.org/10.1101/gad.190413.112
- van Heesch S, van Iterson M, Jacobi J, Boymans S, Essers PB, de Bruijn E, Hao W, MacInnes AW, Cuppen E, Simonis M. Extensive localization of long noncoding RNAs to the cytosol and mono- and polyribosomal complexes. Genome Biol 2014; 15:R6; PMID:24393600; http://dx.doi.org/10.1186/gb-2014-15-1-r6
- Zhang B, Gunawardane L, Niazi F, Jahanbani F, Chen X, Valadkhan S. A novel RNA motif mediates the strict nuclear localization of a long noncoding RNA. Mol Cell Biol 2014; 34:2318-29; PMID:24732794; http://dx.doi.org/10.1128/MCB.01673-13
- Bassell GJ, Zhang H, Byrd AL, Femino AM, Singer RH, Taneja KL, Lifshitz LM, Herman IM, Kosik KS. Sorting of beta-actin mRNA and protein to neurites and growth cones in culture. J Neurosci 1998; 18:251-65; PMID:9412505
- Eom T, Antar LN, Singer RH, Bassell GJ. Localization of a beta-actin messenger ribonucleoprotein complex with zipcode-binding protein modulates the density of dendritic filopodia and filopodial synapses. J Neurosci 2003; 23:10433-44; PMID:14614102
- Long RM, Singer RH, Meng X, Gonzalez I, Nasmyth K, Jansen RP. Mating type switching in yeast controlled by asymmetric localization of ASH1 mRNA. Science 1997; 277:383-7; PMID:9219698; http://dx.doi.org/10.1126/science.277.5324.383
- Takizawa PA, Sil A, Swedlow JR, Herskowitz I, Vale RD. Actin-dependent localization of an RNA encoding a cell-fate determinant in yeast. Nature 1997; 389:90-3; PMID:9288973; http://dx.doi.org/10.1038/38015
- Marc P, Margeot A, Devaux F, Blugeon C, Corral-Debrinski M, Jacq C. Genome-wide analysis of mRNAs targeted to yeast mitochondria. EMBO Rep 2002; 3:159-64; PMID:11818335; http://dx.doi.org/10.1093/embo-reports/kvf025
- Sylvestre J, Margeot A, Jacq C, Dujardin G, Corral-Debrinski M. The role of the 3′ untranslated region in mRNA sorting to the vicinity of mitochondria is conserved from yeast to human cells. Mol Biol Cell 2003; 14:3848-56; PMID:12972568; http://dx.doi.org/10.1091/mbc.E03-02-0074
- Gadir N, Haim-Vilmovsky L, Kraut-Cohen J, Gerst JE. Localization of mRNAs coding for mitochondrial proteins in the yeast Saccharomyces cerevisiae. RNA 2011; 17:1551-65; PMID:21705432; http://dx.doi.org/10.1261/rna.2621111
- Bassell GJ, Powers CM, Taneja KL, Singer RH. Single mRNAs visualized by ultrastructural in situ hybridization are principally localized at actin filament intersections in fibroblasts. J Cell Biol 1994; 126:863-76; PMID:7914201; http://dx.doi.org/10.1083/jcb.126.4.863
- Wang ET, Cody NAL, Jog S, Biancolella M, Wang TT, Treacy DJ, Luo S, Schroth GP, Housman DE, Reddy S, et al. Transcriptome-wide regulation of pre-mRNA splicing and mRNA localization by muscleblind proteins. Cell 2012; 150:710-24; PMID:22901804; http://dx.doi.org/10.1016/j.cell.2012.06.041
- Raj A, van den Bogaard P, Rifkin SA, van Oudenaarden A, Tyagi S. Imaging individual mRNA molecules using multiple singly labeled probes. Nat Methods 2008; 5:877-9; PMID:18806792; http://dx.doi.org/10.1038/nmeth.1253
- Femino AM, Fay FS, Fogarty K, Singer RH. Visualization of single RNA transcripts in situ. Science 1998; 280:585-90; PMID:9554849; http://dx.doi.org/10.1126/science.280.5363.585
- Bertrand E, Chartrand P, Schaefer M, Shenoy SM, Singer RH, Long RM. Localization of ASH1 mRNA particles in living yeast. Mol Cell 1998; 2:437-45; PMID:9809065; http://dx.doi.org/10.1016/S1097-2765(00)80143-4
- Park HY, Lim H, Yoon YJ, Follenzi A, Nwokafor C, Lopez-Jones M, Meng X, Singer RH. Visualization of dynamics of single endogenous mRNA labeled in live mouse. Science 2014; 343:422-4; PMID:24458643; http://dx.doi.org/10.1126/science.1239200
- Tyagi S, Kramer FR. Molecular beacons: probes that fluoresce upon hybridization. Nat Biotechnol 1996; 14:303-8; PMID:9630890; http://dx.doi.org/10.1038/nbt0396-303
- Alami NH, Smith RB, Carrasco MA, Williams LA, Winborn CS, Han SSW, Kiskinis E, Winborn B, Freibaum BD, Kanagaraj A, et al. Axonal transport of TDP-43 mRNA granules is impaired by ALS-causing mutations. Neuron 2014; 81:536-43; PMID:24507191; http://dx.doi.org/10.1016/j.neuron.2013.12.018
- Alonas E, Lifland AW, Gudheti M, Vanover D, Jung J, Zurla C, Kirschman J, Fiore VF, Douglas A, Barker TH, et al. Combining single RNA sensitive probes with subdiffraction-limited and live-cell imaging enables the characterization of virus dynamics in cells. ACS Nano 2014; 8:302-15; PMID:24351207; http://dx.doi.org/10.1021/nn405998v
- Miyashiro K, Dichter M, Eberwine J. On the nature and differential distribution of mRNAs in hippocampal neurites: implications for neuronal functioning. Proc Natl Acad Sci U S A 1994; 91:10800-4; PMID:7971965; http://dx.doi.org/10.1073/pnas.91.23.10800
- Tian QB, Nakayama K, Okano A, Suzuki T. Identification of mRNAs localizing in the postsynaptic region. Brain Res Mol Brain Res 1999; 72:147-57; PMID:10529473; http://dx.doi.org/10.1016/S0169-328X(99)00214-4
- Eberwine J, Belt B, Kacharmina JE, Miyashiro K. Analysis of subcellularly localized mRNAs using in situ hybridization, mRNA amplification, and expression profiling. Neurochem Res 2002; 27:1065-77; PMID:12462405; http://dx.doi.org/10.1023/A:1020956805307
- Sung Y-J, Weiler IJ, Greenough WT, Denman RB. Selectively enriched mRNAs in rat synaptoneurosomes. Brain Res Mol Brain Res 2004; 126:81-7; PMID:15207920; http://dx.doi.org/10.1016/j.molbrainres.2004.03.013
- Moccia R, Chen D, Lyles V, Kapuya E, e Y, Kalachikov S, Spahn CMT, Frank J, Kandel ER, Barad M, et al. An unbiased cDNA library prepared from isolated Aplysia sensory neuron processes is enriched for cytoskeletal and translational mRNAs. J Neurosci 2003; 23:9409-17; PMID:14561869
- Vogelaar CF, Gervasi NM, Gumy LF, Story DJ, Raha-Chowdhury R, Leung K-M, Holt CE, Fawcett JW. Axonal mRNAs: characterisation and role in the growth and regeneration of dorsal root ganglion axons and growth cones. Mol Cell Neurosci 2009; 42:102-15; PMID:19520167; http://dx.doi.org/10.1016/j.mcn.2009.06.002
- Lécuyer E, Yoshida H, Parthasarathy N, Alm C, Babak T, Cerovina T, Hughes TR, Tomancak P, Krause HM. Global analysis of mRNA localization reveals a prominent role in organizing cellular architecture and function. Cell 2007; 131:174-87; PMID:17923096; http://dx.doi.org/10.1016/j.cell.2007.08.003
- Matsumoto S, Uchiumi T, Saito T, Yagi M, Takazaki S, Kanki T, Kang D. Localization of mRNAs encoding human mitochondrial oxidative phosphorylation proteins. Mitochondrion 2012; 12:391-8; PMID:22406259; http://dx.doi.org/10.1016/j.mito.2012.02.004
- Mili S, Moissoglu K, Macara IG. Genome-wide screen reveals APC-associated RNAs enriched in cell protrusions. Nature 2008; 453:115-9; PMID:18451862; http://dx.doi.org/10.1038/nature06888
- Poon MM, Choi S-H, Jamieson CAM, Geschwind DH, Martin KC. Identification of process-localized mRNAs from cultured rodent hippocampal neurons. J Neurosci 2006; 26:13390-9; PMID:17182790; http://dx.doi.org/10.1523/JNEUROSCI.3432-06.2006
- Taylor AM, Berchtold NC, Perreau VM, Tu CH, Li Jeon N, Cotman CW. Axonal mRNA in uninjured and regenerating cortical mammalian axons. J Neurosci 2009; 29:4697-707; PMID:19369540; http://dx.doi.org/10.1523/JNEUROSCI.6130-08.2009
- Gumy LF, Yeo GSH, Tung Y-CL, Zivraj KH, Willis D, Coppola G, Lam BYH, Twiss JL, Holt CE, Fawcett JW. Transcriptome analysis of embryonic and adult sensory axons reveals changes in mRNA repertoire localization. RNA 2011; 17:85-98; PMID:21098654; http://dx.doi.org/10.1261/rna.2386111
- Zivraj KH, Tung YCL, Piper M, Gumy L, Fawcett JW, Yeo GSH, Holt CE. Subcellular profiling reveals distinct and developmentally regulated repertoire of growth cone mRNAs. J Neurosci 2010; 30:15464-78; PMID:21084603; http://dx.doi.org/10.1523/JNEUROSCI.1800-10.2010
- Minis A, Dahary D, Manor O, Leshkowitz D, Pilpel Y, Yaron A. Subcellular transcriptomics-Dissection of the mRNA composition in the axonal compartment of sensory neurons. Devel Neurobio 2014; 74:365-81; PMID:24127433; http://dx.doi.org/10.1002/dneu.22140
- Cajigas IJ, Tushev G, Will TJ, tom Dieck S, Fuerst N, Schuman EM. The local transcriptome in the synaptic neuropil revealed by deep sequencing and high-resolution imaging. Neuron 2012; 74:453-66; PMID:22578497; http://dx.doi.org/10.1016/j.neuron.2012.02.036
- Sharp JA, Plant JJ, Ohsumi TK, Borowsky M, Blower MD. Functional analysis of the microtubule-interacting transcriptome. Mol Biol Cell 2011; 22:4312-23; PMID:21937723; http://dx.doi.org/10.1091/mbc.E11-07-0629
- Combs PA, Eisen MB. Sequencing mRNA from cryo-sliced Drosophila embryos to determine genome-wide spatial patterns of gene expression. PLoS One 2013; 8:e71820; PMID:23951250; http://dx.doi.org/10.1371/journal.pone.0071820
- Lee JH, Daugharthy ER, Scheiman J, Kalhor R, Yang JL, Ferrante TC, Terry R, Jeanty SSF, Li C, Amamoto R, et al. Highly multiplexed subcellular RNA sequencing in situ. Science 2014; 343:1360-3; PMID:24578530; http://dx.doi.org/10.1126/science.1250212
- Kislauskis EH, Li Z, Singer RH, Taneja KL. Isoform-specific 3′-untranslated sequences sort alpha-cardiac and beta-cytoplasmic actin messenger RNAs to different cytoplasmic compartments. [published erratum appears in J Cell Biol 1993 Dec;123(6 Pt 2):following 1907]. J Cell Biol 1993; 123:165-72; PMID:8408195; http://dx.doi.org/10.1083/jcb.123.1.165
- Chao JA, Patskovsky Y, Patel V, Levy M, Almo SC, Singer RH. ZBP1 recognition of beta-actin zipcode induces RNA looping. Genes Dev 2010; 24:148-58; PMID:20080952; http://dx.doi.org/10.1101/gad.1862910
- Meer EJ, Wang DO, Kim S, Barr I, Guo F, Martin KC. Identification of a cis-acting element that localizes mRNA to synapses. Proc Natl Acad Sci U S A 2012; 109:4639-44; PMID:22383561; http://dx.doi.org/10.1073/pnas.1116269109
- Ghosh S, Marchand V, Gáspár I, Ephrussi A. Control of RNP motility and localization by a splicing-dependent structure in oskar mRNA. Nat Struct Mol Biol 2012; 19:441-9; PMID:22426546; http://dx.doi.org/10.1038/nsmb.2257
- Liu JM, Liu DR. Discovery of a mRNA mitochondrial localization element in Saccharomyces cerevisiae by nonhomologous random recombination and in vivo selection. Nucleic Acids Res 2007; 35:6750-61; PMID:17916575; http://dx.doi.org/10.1093/nar/gkm777
- Taylor AM, Blurton-Jones M, Rhee SW, Cribbs DH, Cotman CW, Jeon NL. A microfluidic culture platform for CNS axonal injury, regeneration and transport. Nat Methods 2005; 2:599-605; PMID:16094385; http://dx.doi.org/10.1038/nmeth777
- Ferrandon D, Koch I, Westhof E, Nüsslein-Volhard C. RNA-RNA interaction is required for the formation of specific bicoid mRNA 3′ UTR-STAUFEN ribonucleoprotein particles. EMBO J 1997; 16:1751-8; PMID:9130719; http://dx.doi.org/10.1093/emboj/16.7.1751
- Wickham L, Duchaîne T, Luo M, Nabi IR, DesGroseillers L. Mammalian staufen is a double-stranded-RNA- and tubulin-binding protein which localizes to the rough endoplasmic reticulum. Mol Cell Biol 1999; 19:2220-30; PMID:10022909
- Kiebler MA, Hemraj I, Verkade P, Köhrmann M, Fortes P, Marión RM, Ortín J, Dotti CG. The mammalian staufen protein localizes to the somatodendritic domain of cultured hippocampal neurons: implications for its involvement in mRNA transport. J Neurosci 1999; 19:288-97; PMID:9870958
- St Johnston D, Beuchle D, Nüsslein-Volhard C. Staufen, a gene required to localize maternal RNAs in the Drosophila egg. Cell 1991; 66:51-63; PMID:1712672; http://dx.doi.org/10.1016/0092-8674(91)90138-O
- Hofacker IL. RNA consensus structure prediction with RNAalifold. Methods Mol Biol 2007; 395:527-44; PMID:17993696; http://dx.doi.org/10.1007/978-1-59745-514-5_33
- Pedersen JS, Bejerano G, Siepel A, Rosenbloom K, Lindblad-Toh K, Lander ES, Kent J, Miller W, Haussler D. Identification and classification of conserved RNA secondary structures in the human genome. PLoS Comput Biol 2006; 2:e33; PMID:16628248; http://dx.doi.org/10.1371/journal.pcbi.0020033
- Foster LJ, de Hoog CL, Zhang Y, Zhang Y, Xie X, Mootha VK, Mann M. A mammalian organelle map by protein correlation profiling. Cell 2006; 125:187-99; PMID:16615899; http://dx.doi.org/10.1016/j.cell.2006.03.022
- Cox B, Emili A. Tissue subcellular fractionation and protein extraction for use in mass-spectrometry-based proteomics. Nat Protoc 2006; 1:1872-8; PMID:17487171; http://dx.doi.org/10.1038/nprot.2006.273
- Wang Z, Rolish ME, Yeo G, Tung V, Mawson M, Burge CB. Systematic identification and analysis of exonic splicing silencers. Cell 2004; 119:831-45; PMID:15607979; http://dx.doi.org/10.1016/j.cell.2004.11.010
- Ke S, Shang S, Kalachikov SM, Morozova I, Yu L, Russo JJ, Ju J, Chasin LA. Quantitative evaluation of all hexamers as exonic splicing elements. Genome Res 2011; 21:1360-74; PMID:21659425; http://dx.doi.org/10.1101/gr.119628.110
- Bashirullah A, Halsell SR, Cooperstock RL, Kloc M, Karaiskakis A, Fisher WW, Fu W, Hamilton JK, Etkin LD, Lipshitz HD. Joint action of two RNA degradation pathways controls the timing of maternal transcript elimination at the midblastula transition in Drosophila melanogaster. EMBO J 1999; 18:2610-20; PMID:10228172; http://dx.doi.org/10.1093/emboj/18.9.2610
- Bergsten SE, Gavis ER. Role for mRNA localization in translational activation but not spatial restriction of nanos RNA. Development 1999; 126:659-69; PMID:9895314
- Chang P, Torres J, Lewis RA, Mowry KL, Houliston E, King ML. Localization of RNAs to the mitochondrial cloud in Xenopus oocytes through entrapment and association with endoplasmic reticulum. Mol Biol Cell 2004; 15:4669-81; PMID:15292452; http://dx.doi.org/10.1091/mbc.E04-03-0265
- Forrest KM, Gavis ER. Live imaging of endogenous RNA reveals a diffusion and entrapment mechanism for nanos mRNA localization in Drosophila. Curr Biol 2003; 13:1159-68; PMID:12867026; http://dx.doi.org/10.1016/S0960-9822(03)00451-2
- Baltz AG, Munschauer M, Schwanhäusser B, Vasile A, Murakawa Y, Schueler M, Youngs N, Penfold-Brown D, Drew K, Milek M, et al. The mRNA-bound proteome and its global occupancy profile on protein-coding transcripts. Mol Cell 2012; 46:674-90; PMID:22681889; http://dx.doi.org/10.1016/j.molcel.2012.05.021
- Ross AF, Oleynikov Y, Kislauskis EH, Taneja KL, Singer RH. Characterization of a beta-actin mRNA zipcode-binding protein. Mol Cell Biol 1997; 17:2158-65; PMID:9121465
- Vikesaa J, Hansen TVO, Jønson L, Borup R, Wewer UM, Christiansen J, Nielsen FC. RNA-binding IMPs promote cell adhesion and invadopodia formation. EMBO J 2006; 25:1456-68; PMID:16541107; http://dx.doi.org/10.1038/sj.emboj.7601039
- Zhang HL, Eom T, Oleynikov Y, Shenoy SM, Liebelt DA, Dictenberg JB, Singer RH, Bassell GJ. Neurotrophin-induced transport of a beta-actin mRNP complex increases beta-actin levels and stimulates growth cone motility. Neuron 2001; 31:261-75; PMID:11502257; http://dx.doi.org/10.1016/S0896-6273(01)00357-9
- Yao J, Sasaki Y, Wen Z, Bassell GJ, Zheng JQ. An essential role for beta-actin mRNA localization and translation in Ca2+-dependent growth cone guidance. Nat Neurosci 2006; 9:1265-73; PMID:16980965; http://dx.doi.org/10.1038/nn1773
- Donnelly CJ, Willis DE, Xu M, Tep C, Jiang C, Yoo S, Schanen NC, Kirn-Safran CB, van Minnen J, English A, et al. Limited availability of ZBP1 restricts axonal mRNA localization and nerve regeneration capacity. EMBO J 2011; 30:4665-77; PMID:21964071; http://dx.doi.org/10.1038/emboj.2011.347
- Ferrandon D, Elphick L, Nüsslein-Volhard C, St Johnston D. Staufen protein associates with the 3’UTR of bicoid mRNA to form particles that move in a microtubule-dependent manner. Cell 1994; 79:1221-32; PMID:8001156; http://dx.doi.org/10.1016/0092-8674(94)90013-2
- Lebeau G, Miller LC, Tartas M, McAdam R, Laplante I, Badeaux F, DesGroseillers L, Sossin WS, Lacaille J-C. Staufen 2 regulates mGluR long-term depression and Map1b mRNA distribution in hippocampal neurons. Learn Mem 2011; 18:314-26; PMID:21508097; http://dx.doi.org/10.1101/lm.2100611
- Dubnau J, Chiang AS, Grady L, Barditch J, Gossweiler S, McNeil J, Smith P, Buldoc F, Scott R, Certa U, et al. The staufen/pumilio pathway is involved in Drosophila long-term memory. Curr Biol 2003; 13:286-96; PMID:12593794; http://dx.doi.org/10.1016/S0960-9822(03)00064-2
- Goetze B, Tuebing F, Xie Y, Dorostkar MM, Thomas S, Pehl U, Boehm S, Macchi P, Kiebler MA. The brain-specific double-stranded RNA-binding protein Staufen2 is required for dendritic spine morphogenesis. J Cell Biol 2006; 172:221-31; PMID:16418534; http://dx.doi.org/10.1083/jcb.200509035
- Tang SJ, Meulemans D, Vazquez L, Colaco N, Schuman E. A role for a rat homolog of staufen in the transport of RNA to neuronal dendrites. Neuron 2001; 32:463-75; PMID:11709157; http://dx.doi.org/10.1016/S0896-6273(01)00493-7
- Vessey JP, Macchi P, Stein JM, Mikl M, Hawker KN, Vogelsang P, Wieczorek K, Vendra G, Riefler J, Tübing F, et al. A loss of function allele for murine Staufen1 leads to impairment of dendritic Staufen1-RNP delivery and dendritic spine morphogenesis. Proc Natl Acad Sci U S A 2008; 105:16374-9; PMID:18922781; http://dx.doi.org/10.1073/pnas.0804583105
- Laver JD, Li X, Ancevicius K, Westwood JT, Smibert CA, Morris QD, Lipshitz HD. Genome-wide analysis of Staufen-associated mRNAs identifies secondary structures that confer target specificity. Nucleic Acids Res 2013; 41:9438-60; PMID:23945942; http://dx.doi.org/10.1093/nar/gkt702
- Verkerk AJ, Pieretti M, Sutcliffe JS, Fu YH, Kuhl DP, Pizzuti A, Reiner O, Richards S, Victoria MF, Zhang FP, et al. Identification of a gene (FMR-1) containing a CGG repeat coincident with a breakpoint cluster region exhibiting length variation in fragile X syndrome. Cell 1991; 65:905-14; PMID:1710175; http://dx.doi.org/10.1016/0092-8674(91)90397-H
- Oberlé I, Rousseau F, Heitz D, Kretz C, Devys D, Hanauer A, Boué J, Bertheas MF, Mandel JL. Instability of a 550-base pair DNA segment and abnormal methylation in fragile X syndrome. Science 1991; 252:1097-102; PMID:2031184; http://dx.doi.org/10.1126/science.252.5009.1097
- Sutcliffe JS, Nelson DL, Zhang F, Pieretti M, Caskey CT, Saxe D, Warren ST. DNA methylation represses FMR-1 transcription in fragile X syndrome. Hum Mol Genet 1992; 1:397-400; PMID:1301913; http://dx.doi.org/10.1093/hmg/1.6.397
- Antar LN, Dictenberg JB, Plociniak M, Afroz R, Bassell GJ. Localization of FMRP-associated mRNA granules and requirement of microtubules for activity-dependent trafficking in hippocampal neurons. Genes Brain Behav 2005; 4:350-9; PMID:16098134; http://dx.doi.org/10.1111/j.1601-183X.2005.00128.x
- Brown V, Jin P, Ceman S, Darnell JC, O’Donnell WT, Tenenbaum SA, Jin X, Feng Y, Wilkinson KD, Keene JD, et al. Microarray identification of FMRP-associated brain mRNAs and altered mRNA translational profiles in fragile X syndrome. Cell 2001; 107:477-87; PMID:11719188; http://dx.doi.org/10.1016/S0092-8674(01)00568-2
- Antar LN, Afroz R, Dictenberg JB, Carroll RC, Bassell GJ. Metabotropic glutamate receptor activation regulates fragile x mental retardation protein and FMR1 mRNA localization differentially in dendrites and at synapses. J Neurosci 2004; 24:2648-55; PMID:15028757; http://dx.doi.org/10.1523/JNEUROSCI.0099-04.2004
- Muddashetty RS, Kelić S, Gross C, Xu M, Bassell GJ. Dysregulated metabotropic glutamate receptor-dependent translation of AMPA receptor and postsynaptic density-95 mRNAs at synapses in a mouse model of fragile X syndrome. J Neurosci 2007; 27:5338-48; PMID:17507556; http://dx.doi.org/10.1523/JNEUROSCI.0937-07.2007
- Dictenberg JB, Swanger SA, Antar LN, Singer RH, Bassell GJ. A direct role for FMRP in activity-dependent dendritic mRNA transport links filopodial-spine morphogenesis to fragile X syndrome. Dev Cell 2008; 14:926-39; PMID:18539120; http://dx.doi.org/10.1016/j.devcel.2008.04.003
- Kanai Y, Dohmae N, Hirokawa N. Kinesin transports RNA: isolation and characterization of an RNA-transporting granule. Neuron 2004; 43:513-25; PMID:15312650; http://dx.doi.org/10.1016/j.neuron.2004.07.022
- Jønson L, Vikesaa J, Krogh A, Nielsen LK, Hansen Tv, Borup R, Johnsen AH, Christiansen J, Nielsen FC. Molecular composition of IMP1 ribonucleoprotein granules. Mol Cell Proteomics 2007; 6:798-811; PMID:17289661; http://dx.doi.org/10.1074/mcp.M600346-MCP200
- Maher-Laporte M, Berthiaume F, Moreau M, Julien L-A, Lapointe G, Mourez M, DesGroseillers L. Molecular composition of staufen2-containing ribonucleoproteins in embryonic rat brain. PLoS One 2010; 5:e11350; PMID:20596529; http://dx.doi.org/10.1371/journal.pone.0011350
- Fritzsche R, Karra D, Bennett KL, Ang FY, Heraud-Farlow JE, Tolino M, Doyle M, Bauer KE, Thomas S, Planyavsky M, et al. Interactome of two diverse RNA granules links mRNA localization to translational repression in neurons. Cell Rep 2013; 5:1749-62; PMID:24360960; http://dx.doi.org/10.1016/j.celrep.2013.11.023
- Sutton MA, Schuman EM. Dendritic protein synthesis, synaptic plasticity, and memory. Cell 2006; 127:49-58; PMID:17018276; http://dx.doi.org/10.1016/j.cell.2006.09.014
- Licatalosi DD, Mele A, Fak JJ, Ule J, Kayikci M, Chi SW, Clark TA, Schweitzer AC, Blume JE, Wang X, et al. HITS-CLIP yields genome-wide insights into brain alternative RNA processing. Nature 2008; 456:464-9; PMID:18978773; http://dx.doi.org/10.1038/nature07488
- Ule J, Stefani G, Mele A, Ruggiu M, Wang X, Taneri B, Gaasterland T, Blencowe BJ, Darnell RB. An RNA map predicting Nova-dependent splicing regulation. Nature 2006; 444:580-6; PMID:17065982; http://dx.doi.org/10.1038/nature05304
- König J, Zarnack K, Rot G, Curk T, Kayikci M, Zupan B, Turner DJ, Luscombe NM, Ule J. iCLIP reveals the function of hnRNP particles in splicing at individual nucleotide resolution. Nat Struct Mol Biol 2010; 17:909-15; PMID:20601959; http://dx.doi.org/10.1038/nsmb.1838
- Darnell JC, Jensen KB, Jin P, Brown V, Warren ST, Darnell RB. Fragile X mental retardation protein targets G quartet mRNAs important for neuronal function. Cell 2001; 107:489-99; PMID:11719189; http://dx.doi.org/10.1016/S0092-8674(01)00566-9
- Schaeffer C, Bardoni B, Mandel JL, Ehresmann B, Ehresmann C, Moine H. The fragile X mental retardation protein binds specifically to its mRNA via a purine quartet motif. EMBO J 2001; 20:4803-13; PMID:11532944; http://dx.doi.org/10.1093/emboj/20.17.4803
- Hafner M, Landthaler M, Burger L, Khorshid M, Hausser J, Berninger P, Rothballer A, Ascano M Jr., Jungkamp A-C, Munschauer M, et al. Transcriptome-wide identification of RNA-binding protein and microRNA target sites by PAR-CLIP. Cell 2010; 141:129-41; PMID:20371350; http://dx.doi.org/10.1016/j.cell.2010.03.009
- Ascano M Jr., Mukherjee N, Bandaru P, Miller JB, Nusbaum JD, Corcoran DL, Langlois C, Munschauer M, Dewell S, Hafner M, et al. FMRP targets distinct mRNA sequence elements to regulate protein expression. Nature 2012; 492:382-6; PMID:23235829; http://dx.doi.org/10.1038/nature11737
- LeGendre JB, Campbell ZT, Kroll-Conner P, Anderson P, Kimble J, Wickens M. RNA targets and specificity of Staufen, a double-stranded RNA-binding protein in Caenorhabditis elegans. J Biol Chem 2013; 288:2532-45; PMID:23195953; http://dx.doi.org/10.1074/jbc.M112.397349
- Furic L, Maher-Laporte M, DesGroseillers L. A genome-wide approach identifies distinct but overlapping subsets of cellular mRNAs associated with Staufen1- and Staufen2-containing ribonucleoprotein complexes. RNA 2008; 14:324-35; PMID:18094122; http://dx.doi.org/10.1261/rna.720308
- Kusek G, Campbell M, Doyle F, Tenenbaum SA, Kiebler M, Temple S. Asymmetric segregation of the double-stranded RNA binding protein Staufen2 during mammalian neural stem cell divisions promotes lineage progression. Cell Stem Cell 2012; 11:505-16; PMID:22902295; http://dx.doi.org/10.1016/j.stem.2012.06.006
- Heraud-Farlow JE, Sharangdhar T, Li X, Pfeifer P, Tauber S, Orozco D, Hörmann A, Thomas S, Bakosova A, Farlow AR, et al. Staufen2 regulates neuronal target RNAs. Cell Rep 2013; 5:1511-8; PMID:24360961; http://dx.doi.org/10.1016/j.celrep.2013.11.039
- Singh G, Ricci EP, Moore MJ. RIPiT-Seq: a high-throughput approach for footprinting RNA:protein complexes. Methods 2014; 65:320-32; PMID:24096052; http://dx.doi.org/10.1016/j.ymeth.2013.09.013
- Ricci EP, Kucukural A, Cenik C, Mercier BC, Singh G, Heyer EE, Ashar-Patel A, Peng L, Moore MJ. Staufen1 senses overall transcript secondary structure to regulate translation. Nat Struct Mol Biol 2014; 21:26-35; PMID:24336223; http://dx.doi.org/10.1038/nsmb.2739
- Rage F, Boulisfane N, Rihan K, Neel H, Gostan T, Bertrand E, Bordonné R, Soret J. Genome-wide identification of mRNAs associated with the protein SMN whose depletion decreases their axonal localization. RNA 2013; 19:1755-66; PMID:24152552; http://dx.doi.org/10.1261/rna.040204.113
- Li X, Zhao X, Fang Y, Jiang X, Duong T, Fan C, Huang CC, Kain SR. Generation of destabilized green fluorescent protein as a transcription reporter. J Biol Chem 1998; 273:34970-5; PMID:9857028; http://dx.doi.org/10.1074/jbc.273.52.34970
- Corish P, Tyler-Smith C. Attenuation of green fluorescent protein half-life in mammalian cells. Protein Eng 1999; 12:1035-40; PMID:10611396; http://dx.doi.org/10.1093/protein/12.12.1035
- Kitsera N, Khobta A, Epe B. Destabilized green fluorescent protein detects rapid removal of transcription blocks after genotoxic exposure. Biotechniques 2007; 43:222-7; PMID:17824390; http://dx.doi.org/10.2144/000112479
- Willis DE, Xu M, Donnelly CJ, Tep C, Kendall M, Erenstheyn M, English AW, Schanen NC, Kirn-Safran CB, Yoon SO, et al. Axonal Localization of transgene mRNA in mature PNS and CNS neurons. J Neurosci 2011; 31:14481-7; PMID:21994364; http://dx.doi.org/10.1523/JNEUROSCI.2950-11.2011
- Moriyoshi K, Richards LJ, Akazawa C, O’Leary DD, Nakanishi S. Labeling neural cells using adenoviral gene transfer of membrane-targeted GFP. Neuron 1996; 16:255-60; PMID:8789941; http://dx.doi.org/10.1016/S0896-6273(00)80044-6
- Aakalu G, Smith WB, Nguyen N, Jiang C, Schuman EM. Dynamic visualization of local protein synthesis in hippocampal neurons. Neuron 2001; 30:489-502; PMID:11395009; http://dx.doi.org/10.1016/S0896-6273(01)00295-1
- Yudin D, Hanz S, Yoo S, Iavnilovitch E, Willis D, Gradus T, Vuppalanchi D, Segal-Ruder Y, Ben-Yaakov K, Hieda M, et al. Localized regulation of axonal RanGTPase controls retrograde injury signaling in peripheral nerve. Neuron 2008; 59:241-52; PMID:18667152; http://dx.doi.org/10.1016/j.neuron.2008.05.029
- Leung K-M, van Horck FPG, Lin AC, Allison R, Standart N, Holt CE. Asymmetrical beta-actin mRNA translation in growth cones mediates attractive turning to netrin-1. Nat Neurosci 2006; 9:1247-56; PMID:16980963; http://dx.doi.org/10.1038/nn1775
- Cox LJ, Hengst U, Gurskaya NG, Lukyanov KA, Jaffrey SR. Intra-axonal translation and retrograde trafficking of CREB promotes neuronal survival. Nat Cell Biol 2008; 10:149-59; PMID:18193038; http://dx.doi.org/10.1038/ncb1677
- Gilchrist A, Au CE, Hiding J, Bell AW, Fernandez-Rodriguez J, Lesimple S, Nagaya H, Roy L, Gosline SJC, Hallett M, et al. Quantitative proteomics analysis of the secretory pathway. Cell 2006; 127:1265-81; PMID:17174899; http://dx.doi.org/10.1016/j.cell.2006.10.036
- Takamori S, Holt M, Stenius K, Lemke EA, Grønborg M, Riedel D, Urlaub H, Schenck S, Brügger B, Ringler P, et al. Molecular anatomy of a trafficking organelle. Cell 2006; 127:831-46; PMID:17110340; http://dx.doi.org/10.1016/j.cell.2006.10.030
- Pagliarini DJ, Calvo SE, Chang B, Sheth SA, Vafai SB, Ong S-E, Walford GA, Sugiana C, Boneh A, Chen WK, et al. A mitochondrial protein compendium elucidates complex I disease biology. Cell 2008; 134:112-23; PMID:18614015; http://dx.doi.org/10.1016/j.cell.2008.06.016
- Yoon BC, Jung H, Dwivedy A, O’Hare CM, Zivraj KH, Holt CE. Local translation of extranuclear lamin B promotes axon maintenance. Cell 2012; 148:752-64; PMID:22341447; http://dx.doi.org/10.1016/j.cell.2011.11.064
- Rhee H-W, Zou P, Udeshi ND, Martell JD, Mootha VK, Carr SA, Ting AY. Proteomic mapping of mitochondria in living cells via spatially restricted enzymatic tagging. Science 2013; 339:1328-31; PMID:23371551; http://dx.doi.org/10.1126/science.1230593
- Ingolia NT, Ghaemmaghami S, Newman JRS, Weissman JS. Genome-wide analysis in vivo of translation with nucleotide resolution using ribosome profiling. Science 2009; 324:218-23; PMID:19213877; http://dx.doi.org/10.1126/science.1168978
- Reid DW, Nicchitta CV. Primary role for endoplasmic reticulum-bound ribosomes in cellular translation identified by ribosome profiling. J Biol Chem 2012; 287:5518-27; PMID:22199352; http://dx.doi.org/10.1074/jbc.M111.312280
- Buxbaum AR, Wu B, Singer RH. Single β-actin mRNA detection in neurons reveals a mechanism for regulating its translatability. Science 2014; 343:419-22; PMID:24458642; http://dx.doi.org/10.1126/science.1242939
- Mingle LA, Okuhama NN, Shi J, Singer RH, Condeelis J, Liu G. Localization of all seven messenger RNAs for the actin-polymerization nucleator Arp2/3 complex in the protrusions of fibroblasts. J Cell Sci 2005; 118:2425-33; PMID:15923655; http://dx.doi.org/10.1242/jcs.02371
- Trcek T, Chao JA, Larson DR, Park HY, Zenklusen D, Shenoy SM, Singer RH. Single-mRNA counting using fluorescent in situ hybridization in budding yeast. Nat Protoc 2012; 7:408-19; PMID:22301778; http://dx.doi.org/10.1038/nprot.2011.451