Abstract
Radiation-induced bystander effects are well-established phenomena, in which DNA damage responses are induced not only in the directly irradiated cells but also in the non-irradiated bystander cells through intercellular signal transmission. Recent studies hint that bystander effects are possibly mediated via small non-coding RNAs, especially microRNAs. Thus, more details about the roles of microRNA in bystander effects are urgently needed to be elucidated. Here we demonstrated that bystander effects were induced in human fetal lung MRC-5 fibroblasts through medium-mediated way by different types of radiation. We identified a set of differentially expressed microRNAs in the cell culture medium after irradiation, among which the up-regulation of miR-21 was further verified with qRT-PCR. In addition, we found significant upregulation of miR-21 in both directly irradiated cells and bystander cells, which was confirmed by the expression of miR-21 precursor and its target genes. Transfection of miR-21 mimics into non-irradiated MRC-5 cells caused bystander-like effects. Taken together, our data reveals that miR-21 is involved in radiation-induced bystander effects. Elucidation of such a miRNA-mediated bystander effect is of utmost importance in understanding the biological processes related to ionizing radiation and cell-to-cell communication.
Abbreviations
IR | = | ionizing radiation |
RIBE | = | radiation-induced bystander effects |
ROS | = | reactive oxygen species |
TNFα | = | tumor necrosis factor-alpha |
TGFβ1 | = | transforming growth factor-beta 1 |
3′-UTR | = | 3′-untranslated region |
miRNA | = | microRNA |
miR-21 | = | microRNA-21 |
pre-miR-21 | = | the precursor of miR-21 |
Introduction
Conventional evaluation of radiation risk is primarily based on the detrimental effects presenting in irradiated cells or tissues. In recent 2 decades, a number of reports about the non-targeted effects after radiation have challenged this concept. It is now well accepted that ionizing radiation (IR) induces DNA damage responses in cells that are not hit directly by IR.Citation1-3 The irradiated cells are capable of transmitting signals into the neighboring cells through gap junction and/or culture medium, resulting in a series of biological responses in non-irradiated cells. These responses are termed as radiation-induced bystander effects (RIBE), which have been identified both in vitro and in vivo.Citation4-7 Bystander effects are manifested by a wide range of biological endpoints, such as micronuclei, sister-chromatid exchange (SCE), chromosomal aberrations, neoplastic transformation, cell death, proliferation and differentiation.Citation2,8-11
In 1997, Mothersill and Seymour first demonstrated a significant reduction of clonogenic survival in both irradiated and non-irradiated cells through a medium-mediated way.Citation12 After that, more research on the RIBE produced by conditioned medium transfer from irradiated cells to non-irradiated cells have been reported.Citation12-15 Later, a novel co-culture system (Transwell system) was used in studying on medium-borne bystander effect.Citation16 It has been proved that some soluble factors in medium harvested from the irradiated cells are clearly involved in the RIBE, such as nitric oxide,Citation17 reactive oxygen species (ROS),Citation18 tumor necrosis factor α (TNFα),Citation19 and microRNAs (miRNAs).Citation20
MiRNAs, a class of 18-24 nt non-coding RNAs, are capable of regulating the expression of their unique target genes by binding to the 3′-untranslated region (3′-UTR) of mRNAs.Citation21 Since the discovery of lin-4, miRNAs have been found to play essential roles in many biological processes such as terminal differentiation,Citation22 cell cycle,Citation23 apoptosis,Citation24 DNA methylationCitation25 and DNA damage responses.Citation26 In addition, recent studies indicate that miRNAs may possibly be involved in bystander effects. For instance, deregulation of sex-specific microRNAome and up-regulation of miR-194 in the non-irradiated bystander spleen have been found in mice after exposure of crania to X-rays.Citation27,28 Moreover, miRNA expression is found to be deregulated in bystander 3-dimensional tissueCitation29 and the miRNA expression profiles are distinct from that of directly irradiated cells or tissues.Citation20,30 These results imply that miRNAs may play important roles in radiation responses and intercellular signaling pathway between irradiated cells and bystander cells. However, more details of miRNAs in response to the RIBE need to be elucidated. Recently, significant amounts of miRNAs were found in extracellular human body fluids including plasma/serum, saliva and urine.Citation31-35 Cultured mammalian cells can also export miRNAs into the extracellular environment.Citation35-37 The secreted miRNAs are packaged into extracellular vesicles such as exosomes and microvesicles, and gain new properties such as resistant to RNase digestion, prolonged room temperature and multiple freeze-thawing.Citation38,39 These new discoveries of miRNAs properties help us to comprehend the long-distance bystander effects.
The specific goal of this study was to determine that whether miRNAs participate in the medium-mediated RIBE, and if so, which specific miRNAs are involved. Elucidation of the miRNA-mediated bystander effects will certainly raise its significant importance in understanding the biological processes related to ionizing radiation and cell-to-cell communication.
Results
RIBE are induced in MRC-5 cells through medium-mediated way by different types of radiation
To investigate whether RIBE could be induced through medium-mediated way in MRC-5 cells, we checked the damage of non-irradiated cells treated with conditioned medium harvested from cells exposed to different doses of X-rays. Three biological endpoints relevant to RIBE were analyzed. As shown in , the frequency of micronuclei in bystander cells increased after medium-transfer, especially in 2 Gy-irradiated conditioned medium (p < 0.05). After co-culture with conditioned medium, the number of 53BP1 foci in bystander cells increased and demonstrated significance difference when the X-ray doses were 0.5 and 2 Gy (p < 0.05) (). As for the clonogenic survival, only 2 Gy of X-ray exposure induced significant bystander effects (p < 0.05) through medium-mediated way (). We also analyzed these 3 endpoints in directly irradiated cells (IR) and bystander cells (Bystander) (). Compared with cells directly irradiated by 2 Gy of X-rays, the bystander cells showed lower-levels of biological effects (p < 0.05).
Figure 1. Bystander effects mediated by conditioned medium. (A) Frequency of micronuclei (MNF) in bystander cells treated for 48 h with conditioned medium harvested from directly irradiated cells. Cells were exposed to 0, 0.2, 0.5 and 2 Gy of X-rays. Conditioned medium was harvested at 3 h after exposure. (B) Yields of 53BP1 foci in bystander cells cultured for 18 h in conditioned medium harvested from irradiated cells 3 h after exposure to 0, 0.2, 0.5 and 2 Gy of X-rays. (C) Survival fraction in bystander cells cultured for 18 h in medium harvested from irradiated cells 3 h after exposure to 0, 0.2, 0.5 and 2 Gy of X-rays. (D) Frequency of micronuclei (MNF) in irradiated or bystander cells treated with X-ray irradiation or conditioned medium. (E) Yields of 53BP1 foci in in irradiated or bystander cells treated with X-ray irradiation or conditioned medium. (F) Survival fraction of irradiated or bystander cells treated with X-ray irradiation or conditioned medium.
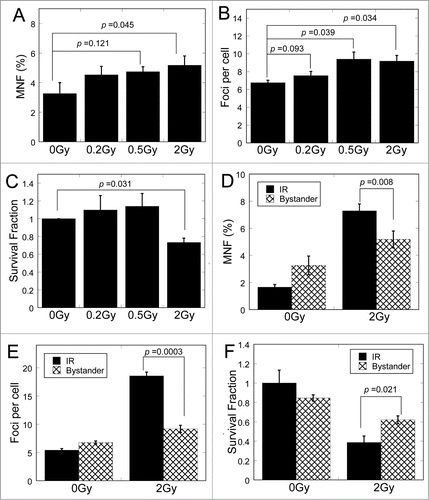
To identify whether the medium-mediated RIBE could be induced by different types of particle irradiation, we checked the same biological endpoints in bystander cells after exposure of MRC-5 cells to 150 MeV/u helium (He150), 135 MeV/u carbon (C135) and 500 MeV/u iron ions (Fe500), respectively. Compared with the bystander cells treated with conditioned medium from non-irradiated cells, the bystander cells treated with conditioned medium from irradiated cells showed apparent increase in the frequency of micronuclei () and 53BP1 foci (), and dramatic decrease in survival fraction (), suggesting that RIBE could be induced by different types of particles.
Figure 2. Medium-mediated bystander effects could be induced by different types of ionizing radiation. (A) Frequency of micronuclei (MNF) in bystander cells cultured for 48 h in medium harvested from irradiated cells 3 h after exposure to 0 and 2 Gy of various type of particles. (B) Yields of 53BP1 foci in bystander cells cultured for 18 h in medium harvested from irradiated cells 3 h after exposure to 0 and 1.5 Gy of various type of particles. (C) Survival fraction of bystander cells cultured for 18 h in medium harvested from irradiated cells 3 h after exposure to 0 and 2 Gy of various type of particles.
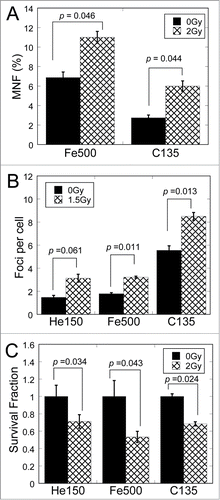
Taken together, these data indicate that RIBE are induced in MRC-5 cells through medium-mediated way, which are consistent with earlier studies.Citation12,16,40,41
Radiation changes miRNA expression profiles of cell culture medium
In our quest to identify the roles of miRNAs in RIBE, we analyzed the miRNA expression profiles of the cell culture medium after MRC-5 cells were exposed to 2 Gy of X-rays. Using well-developed differential-centrifugation methods,Citation36 we isolated the RNAs in culture medium harvested from irradiated cells and non-irradiated cells separately, and sequenced all small molecular RNAs using Solexa sequencing technology.Citation42 Finally, 169 known miRNAs were detected in the non-irradiated sample and 182 in the irradiated sample. There were 136 common known miRNAs in both non-irradiated and irradiated samples, among which 69 miRNAs were up-regulated while 67 were down-regulated by irradiation. The reads and fold change of miRNAs up- or downregulated more than 2-fold in the culture medium after irradiation were listed in .
Figure 3. The microRNAs up- or downregulated more than 2-fold in culture medium detected by Solexa sequencing technology. Left vertical axis: Fold change (log2 Rad./Ctrl) of miRNAs in both samples after reads normalization, represented in dots; Right vertical axis: Standard reads of miRNAs in irradiated medium, represented in bars. Standard reads = Reads / Total reads ×103.
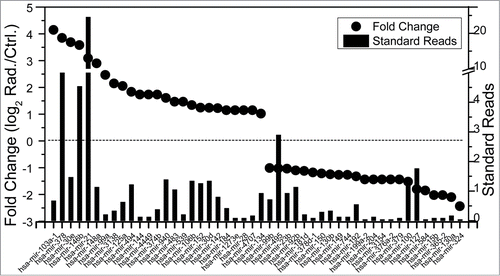
These results indicate that the miRNA expression profiles of culture medium were changed after exposure of MRC-5 cells to X-ray irradiation.
MiR-21 is upregulated in conditioned medium, directly irradiated cells and bystander cells
We selected miR-21, miR-146b and miR-378, which had higher reads and fold change (standard reads in the irradiated sample > 4 and fold change > 2) in the Solexa sequencing analysis, for the qRT-PCR verification. As can be seen in , the miR-21 expression in medium increased significantly more than 2 folds (p < 0.05) following radiation, while miR-146b and miR-378 showed no significant increase.
Figure 4. Relative expression of microRNAs and their precursor and target genes. (A) MiRNA expression in conditioned medium from 0 and 2 Gy of X-ray irradiated cells. (B) MiRNAs expression in MRC-5 cells directly irradiated with 0 and 2 Gy of X-rays. (C) MiRNAs expression in bystander MRC-5 cells after co-cultured with 0 and 2 Gy conditioned medium. (D) The expression of pre-miR-21 and pre-miR-146b in directly irradiated cells and bystander cells harvested at 0.5 h after irradiation or medium transfer. (E) The mRNA expression of target genes of miR-21 in bystander cells 24 h after co-cultured with 0 and 2 Gy conditioned medium. (F) Western blot assay shows Bcl-2 expression in bystander cells 48 h after co-cultured with 0 and 2 Gy conditioned medium. β-actin, loading control. (G) Densitometry analysis of Bcl-2 protein levels shown in panel F. (H) The expression of miR-21 relative to unirradiated controls in irradiated cells and conditioned medium. IR Cells were exposed to 0, 0.5, 2 and 4 Gy of X-rays. Conditioned medium was harvested at 3 h after exposure. The relative expression is shown as log2 transformed values. (I) The expression of miR-21 relative to unirradiated controls in 2 Gy irradiated cells and conditioned medium. The miR-21 expression was assessed at 0, 0.5, 4, 12 and 24 h post irradiation. The relative expression is shown as log2 transformed values.
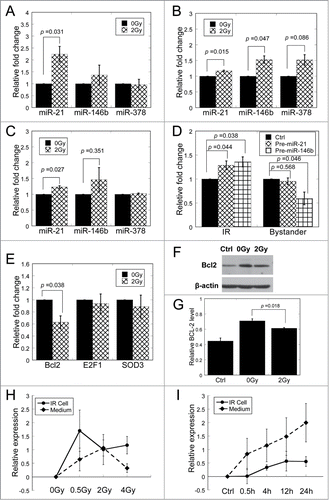
For further investigation, we first measured the expression levels of miR-21, miR-146b and miR-378 in the directly irradiated cells. As shown in , miR-21 and miR-146b were up-regulated significantly in cells exposed to 2 Gy of X-rays. We next measured their expression levels in the bystander cells treated with conditioned medium harvested from irradiated cells. Results similar to cell culture medium were obtained ().
To verify the transcriptional expression of miR-21 and miR-146b after irradiation or medium-transfer, we further measured the expression levels of the precursor of miR-21 (pre-miR-21) and miR-146b (pre-miR-146b) in directly irradiated and bystander cells. The expression of pre-miR-21 was upregulated significantly in directly irradiated cells (P < 0.05) but showed no significant change in bystander cells; the expression level of pre-miR-146b was up-regulated significantly in directly irradiated cells (P < 0.05) but down-regulated in bystander cells (). These data suggested that overexpressed mature miR-21 in bystander cells could be internalized by the cells from the conditioned medium through pino- or endo-cytosis.
MiR-21 is a well described microRNA involved in cellular response to ionizing radiation. Validated target genes of miR-21 have been reported to participate in DNA damage response, apoptosis and cell survival.Citation43-46 Since miRNAs regulate target genes by binding to the 3′-untranslated region (3′-UTR) of mRNAs,Citation21 we detected the mRNA levels of Bcl-2, E2F1 and SOD3, which were approved as the target genes of miR-21, in bystander cells after cultured with 0 and 2 Gy conditioned medium. It was found that the conditioned medium harvested from irradiated cells led to significantly reduced mRNA level in Bcl-2 (p < 0.05), but not in E2F1 and SOD3 (), implying the high sensitivity of Bcl-2 to the slight increase of miR-21 expression (1.23 times) in bystander cells. Consistent with these results, Western blotting results indicated that the protein level of Bcl-2 was also remarkably suppressed 48 h after conditioned-medium transfer ().
Furthermore, we analyzed the dose response of miR-21 expression in irradiated cells and in conditioned medium (). Compared to cells treated with 2 Gy and 4 Gy of X-rays, cells treated with 0.5 Gy X-rays showed relatively higher expression of miR-21. However, the highest expression of miR-21 in conditioned medium was reached at 2 Gy of irradiation, which is significantly different (p < 0.05) in comparison with unirradiated conditioned medium.
The time response of miR-21 expression in irradiated cells and in conditioned medium was also measured (). The expression of miR-21 started to increase after 0.5 h and reached the maximum at 12 h in irradiated cells. However, the upregulation of miR-21 in conditioned medium started before 0.5 h and increased with extended time. Combined with the result that pre-miR-21 was upregulated in irradiated cells at 0.5 h after irradiation (), our data suggested that as response to radiation, the mature miR-21 already existed and newly produced in directly irradiated cells was delivered into extracellular spaces.
The above data indicate that miR-21 is up-regulated in the conditioned medium and directly irradiated cells in response to X-ray irradiation, and in bystander cell after conditioned-medium transfer.
Over-expressed miR-21 in non-irradiated cells induces bystander-like effects
To verify that significant up-regulation of miR-21 played a role in the RIBE, we analyzed the biological effects of overexpressed miR-21 in the non-irradiated fibroblasts. We transfected MRC-5 cells with miR-21 mimics in different concentration, and found that the miR-21 levels increased significantly in 20 pM mimic transfection (2.24 fold, p < 0.05) (). The reduced mRNA expression of Bcl-2, E2F1 and SOD3 was also detected. As shown in , the levels of E2F1 were reduced significantly in 2 pM mimic transfection (p < 0.05) while the level of SOD3 were reduced significantly in 20 pM mimic transfection (p < 0.05), supporting the explanation that different miRNA concentration affects distinct targets which have been observed in the conditioned medium transfer.
Figure 5. Relative fold change of miR-21 and target genes in MRC-5 cells after transfection of miR-21 mimics. (A) MiR-21 expression in MRC-5 cells transfected with different concentrations of miR-21 mimics. (B) The mRNA expression of target genes of miR-21 in bystander cells 12 h after transfection with different concentrations of miR-21 mimics.
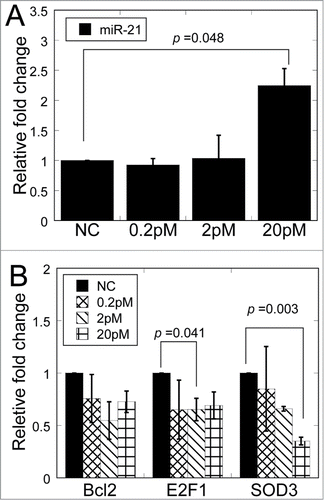
We further inspected the frequency of micronuclei, the 53BP1 foci and the clonogenic survival in MRC-5 cells with transfection of miR-21 mimics. Remarkable increase of frequency of micronuclei was found (P < 0.05) after 0.2 and 2 pM of miR-21 mimic transfection (). Likewise, the number of 53BP1 foci increased after 2 pM of miR-21 mimic transfection (). However, only high level of mimic transfection (20 pM) could lead to the decrease of survival fraction (P < 0.05) (). These data consistent with the results of the RIBE observed by conditioned medium transfer.
Figure 6. Bystander-like effects in MRC-5 cells after transfection of miR-21 mimics. (A) Frequency of micronuclei (MNF) in MRC-5 cells transfected with different concentrations of miR-21 mimics. (B) Yields of 53BP1 foci in MRC-5 cells transfected with different concentrations of miR-21 mimics. (C) Survival fraction of MRC-5 cells transfected with different concentrations of miR-21 mimics.
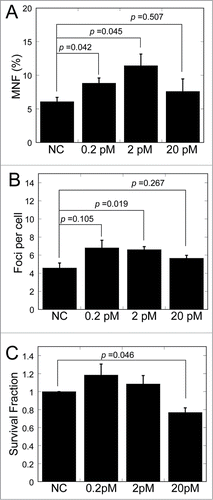
The upregulation of miR-21 after irradiation and the bystander-like effects induced by miR-21 mimic transfection clearly demonstrate the mediator role of miR-21 in the RIBE.
Discussion
Earlier studies have shown that the conditioned medium harvested from irradiated cells is capable of inducing detrimental effects in non-irradiated cells.Citation12-15 Here, we proved that the RIBE can be induced by conditioned medium transfer through analyzing 3 endpoints relevant to the RIBE. They were the micronucleus assay that implies significant chromosome damage and rearrangement,Citation47 the 53BP1 foci formation that is widely used as a marker of DNA double-strand-breaks,Citation48 and the clonogenic survival that shows the long-term progeny survival capacity of bystander cells.Citation40 Interestingly, the 53BP1 foci formation increased significantly in bystander cells treated with the conditioned medium exposed to low-dose of X-rays (0.5 Gy) while the significance was not found in micronucleus induction and clonogenic survival exposed to the same dose. This maybe because DNA double-strand-breaks are relatively sensitive to bystander signals, which are secreted from irradiated cells. For the same reason, the MRC5 cells were exposed to 1.5 Gy of heavy ions to harvest medium for the 53BP1 foci analysis instead of 2 Gy of heavy ions for the analysis of micronucleus assay and clonogenic survival in bystander cells.
Although some studies have reported reactive oxygen species (ROS),Citation18 TNFα,Citation19 TGF-β1 and nitric oxideCitation49 as the signal molecules within medium, few has connected miRNAs with the bystander effects. Inspired by the findings that miRNAs play an important role in the cellular response to ionizing radiation, especially in the intercellular and intracellular signaling pathway,Citation20 we supposed that miRNAs participate in bystander effects by being secreted from irradiated cell and transmitted into bystander recipient cells through extracellular medium. Using RNA isolation, miRNA sequencing and qRT-PCR method, we screened out one particular miRNA, miR-21, which was upregulated in culture medium following irradiation, very similar to TNFα up-regulated in irradiated cells and secreted out to modulate bystander effects.Citation19 Although miR-21 and TNFα exist in different forms in culture medium that one is wrapped in extracellular vesicle and the other is “naked” soluble protein factor, both of them could be secreted from irradiated cells into extracellular medium and accepted by recipient cells to modulate bystander effects.
Then, we examined the expression of miR-21 in irradiated cells and bystander cells treated with conditioned medium. The results indicated that miR-21 was significantly upregulated in the irradiated and bystander cells (). Compared with its relative fold change in medium (2.24 times), the expression levels of miR-21 in irradiated and bystander cells was lower (1.17 and 1.23 times). One explanation for this disunity is that the miRNA transportation is an active process. Some studies have reported that miRNA can be delivered to recipient cells through exosomes, microvesicles and protein-RNA complexes.Citation35,50-52
Although the role of miRNA in the RIBE has been reported,Citation21 miRNA as a mediator participating in the medium-mediated RIBE has not been reported. As verified by miR-21 mimic transfection, miR-21 could induce significant changes in micronucleus formation, 53BP1 foci formation and clonogenic survival in MRC-5 cells, demonstrating that over-expression of miR-21 induces bystander-like effects, which confirmed that miR-21 plays a specific role in the RIBE through a medium-mediated way.
Taken together, our results provide strong evidence that, miR-21 is secreted into medium and received by bystander cells, and finally participates in the induction of detrimental effects in bystander cells. These findings might attract further research on miRNA functions in the RIBE and benefit to understand the mechanisms of radiation-induced bystander effects.
Materials and Methods
Cell culture
Human normal embryonic lung fibroblast cell line MRC-553 were purchased from the American Type Culture Collection (ATCC® CCL-171™). MRC-5 cells was cultured in minimal essential medium (Sigma, USA) supplemented with 10% fetal bovine serum (Hyclone, USA), 100 units/mL penicillin and 100 mg/mL streptomycin and maintained in a 5% CO2 humidified incubator (Thermo Scientific, NC, USA) at 37°C. For the preparation of conditioned medium, the culture medium was replaced with fresh medium supplemented with 10% FBS (depleted of bovine exosomes by overnight centrifugation at 100,000 × g) immediately before treatment with radiation.
Irradiation
Heavy ions were generated by the Heavy Ion Research Facility in Lanzhou (HIRFL) at Institute of Modern Physics, Chinese Academy of Sciences, Lanzhou, China and by the Heavy-Ion Medical Accelerator in Chiba (HIMAC) at National Institute of Radiological Sciences (NIRS), Chiba, Japan. Exposure of all samples was performed at room temperature as previously described.Citation54 X-rays were generated by Faxitron RX-650 (Faxitron Bioptics, Lincolnshire, IL, USA), which was operated with 100 kVp and 5 mA at a dose rate of 1.33 Gy/min.
Conditioned medium transfer
MRC-5 cells (2 × 105) were plated in ϕ35 mm dishes for direct irradiation, while bystander MRC-5 cells (1 ×105) were plated in 12-well plates. After 18 h cultivation, MRC-5 cells were replaced with fresh medium supplemented with 10% FBS immediately before treatment with radiation. Conditioned medium was harvested 3 h after irradiation, centrifuged at 1000 × g for 5 min to exclude cells and cellular fragments, then transferred to non-irradiated cells. Control sample was treated in the same way, except for irradiation.
Medium RNA isolation and Solexa sequencing
Medium RNAs were isolated using a multi-step centrifugation procedure.Citation36 Briefly, the medium were collected and centrifuged at 1000 × g for 10 min to remove cells. This supernatant was transferred to a new tube and centrifuged at 16,000 × g for 60 minutes at 4°C, then centrifuged at 120,000 × g for 60 min to get the final pellet. Medium RNA in the pellet was extracted with TRIzol Reagent (Invitrogen, USA) according to the manufacturer's instructions.
At least 1 μg of medium RNA was subjected to Solexa sequencing (BGI, Shenzhen, China). Briefly, RNA was purified by using polyacrylamide gel electrophoresis (PAGE) to enrich small RNA molecules (under 30 bases), and ligated with Solexa adapters to the 5′ and 3′ termini of the RNA for cDNA synthesis. The cDNA was amplified using the adaptor primers, and then the DNA was used directly for cluster generation and sequencing analysis by the Illumina Genome Analyzer (Illumina, San Diego, CA, USA). Sequence reads with Solexa 3′ adapter (the read length being 36 nt) were picked for miRNA mapping (the same adaptor was used for each sequencing run). Each sequence read was passed through a number of quality control filters. Then, the reads were compared with databases. Novel miRNA candidates were selected after computational analysis. At last, the remained reads of each sample was normalized to 103 with following formula:
Normalized reads of a certain miRNA = detected reads of this miRNA / total reads of remained miRNAs × 103.
qRT-PCR of miRNA and mRNA
After irradiation or other treatment, cells were harvested at the indicated time-points. Then, RNA was isolated using TRIzol reagent (Invitrogen, USA). The quantity of isolated RNA was tested using a spectrophotometer (Eppendorf, Germany). For miRNA expression, total RNA reverse transcription and quantitative RT-PCR were preformed according to the protocol of All-in-One™ miRNA qRT-PCR Detection Kit (Genecopoeia, USA). Cel-miR-39 and RNU6 were used as an invariant control for Conditioned medium and cells, respectively. Primers for miR-21, miR-146b, and miR-378 were designed by Genecopoeia Co. (Guangzhou, China). Primer for pre-miR-21 was: GTACCACCTTGTCGGGTAGC. Primer for pre-miR-146b was: TGGCACTGAGAACTGAATTCCATAG. For mRNA expression, reverse transcription was performed using a First-Strand cDNA Synthesis Kit (GeneCopoeia, USA). The qRT-PCR reaction was performed using SYBR Green PCR master mix (GeneCopoeia, USA). Primers for GAPDH, Bcl-2, E2F1 and SOD3 were designed by Genecopoeia Co. (Guangzhou, China). The PCR program was carried out with a Chromo4 system (Bio-Rad, CA, USA) under the following conditions: initiation for 10 min at 95°C, followed by 50 thermal cycles each at 95°C for 10 s and at 60°C for 20 s and at 70°C for at last 10 s. Data were analyzed with the C(t) value comparison method and normalized to RNU6, Cel-miR-39 or GAPDH expression for different sample.
Western blotting
Cells were lysed in RIPA buffer (Beyotime, China). Proteins were separated by 10% SDS-PAGE and transferred to a methanol-activated PVDF membrane (GE Healthcare, USA). The membrane was blocked for 1 h in PBST containing 5% milk and subsequently probed with anti-Bcl-2 antibody (ImmunoWay, USA), and anti-β-actin antibody (Santa Cruz) for 2 h. After 1-h incubation with goat-anti-mouse HRP-conjugated secondary antibody (Santa Cruz), the protein bands were detected with luminal reagent (GE Healthcare) and their relative intensities were quantified using Adobe Photoshop software (Adobe Systems Inc., San Jose, USA).
MiR-21 mimic transfection assay
MiRNA mimics were synthesized and purified by RiboBio Co. (Guangzhou, China). A miRNA with no sequence similarities to any reported human gene sequence was used as negative control (NC). Twenty-4 hours prior to transfection, cells were plated at 40-60% confluence. Transfection was performed with Lipofectamine 2000 (Invitrogen) according to the manufacture's protocol. The medium was replaced with fresh medium 5 h after transfection.
Micronucleus assay
Cells were treated with 15 μL 0.25 mg/mL cytochalasin B, cultured for 48 h, fixed with Carnoy's fluid for 15 min at room temperature, stained with acridine orange (30 μg/mL), and then observed under fluorescence microscope. At least 500 binucleate cells were scored for each sample.
Immunofluorescence staining
Immunofluorescent microscopy was conducted as described with minor modifications.Citation55 Briefly, cells were fixed in 4% paraformaldehyde for 15 min, washed with PBS, and permeabilized in 0.5% Triton-X 100. After blocked with 5% non-fat milk for 1 h, samples were incubated with a mouse monoclonal anti-53BP1 antibody (Upstate Biotechnology, USA) for 2 h at room temperature and washed. Followed by incubation with Alexa Fluor® 594 anti-mouse antibody (Molecular Probes, USA) for 1 h at room temperature, cells were counter-stained with DAPI and observed with a fluorescent microscope (Nikon, Tokyo, Japan). At least 100 cells were scored for each sample.
Clonogenic survival assay
The clonogenic survival was measured by standard protocols.Citation56 Briefly, cells were harvested and re-suspended in medium supplemented with 10% fetal bovine serum. An appropriate number of cells were plated into each 60 mm dish to produce 20–100 colonies. After incubated for 14 d, colonies were fixed with 70% ethanol and stained with 0.5% crystal violet. Colonies containing more than 50 cells were counted as survivors. At least 3 parallel dishes were scored in 3 or 4 independent replicates for each treatment.
Statistical analysis
All experiments were independently repeated at least 3 times and all data were presented as the means ± standard error. Student's t-tests were used for the statistical analysis. Probability (p) values less than 0.05 were considered to be statistically significant.
Disclosure of Potential Conflicts of Interest
No potential conflicts of interest were disclosed.
Funding
This work was supported by grants from the Major State Basic Research Development Program of China (973 Program, No. 2010CB834201) and the National Natural Science Foundation of China (No. 31270895 and U1232125, 11335011 and 31370846).
References
- Morgan WF. Non-targeted and delayed effects of exposure to ionizing radiation: I. Radiation-induced genomic instability and bystander effects in vitro. Radiat Res 2003; 159:567-80; PMID:12710868; http://dx.doi.org/10.1667/0033-7587(2003)159%5b0567:NADEOE%5d2.0.CO;2
- Mothersill C, Seymour CB. Radiation-induced bystander effects–implications for cancer. Nat Rev Cancer 2004; 4:158-64; PMID:14964312; http://dx.doi.org/10.1038/nrc1277
- Chaudhry MA. Bystander effect: biological endpoints and microarray analysis. Mutat Res 2006; 597:98-112; PMID:16414093; http://dx.doi.org/10.1016/j.mrfmmm.2005.04.023
- Banaz-Yasar F, Lennartz K, Winterhager E, Gellhaus A. Radiation-induced bystander effects in malignant trophoblast cells are independent from gap junctional communication. J Cell Biochem 2008; 103:149-61; PMID:17516549; http://dx.doi.org/10.1002/jcb.21395
- Ryan LA, Smith RW, Seymour CB, Mothersill CE. Dilution of irradiated cell conditioned medium and the bystander effect. Radiat Res 2008; 169:188-96; PMID:18220470; http://dx.doi.org/10.1667/RR1141.1
- Belyakov OV, Mitchell SA, Parikh D, Randers-Pehrson G, Marino SA, Amundson SA, Geard CR, Brenner DJ. Biological effects in unirradiated human tissue induced by radiation damage up to 1 mm away. Proc Nat Acad Sci U S A 2005; 102:14203-8; PMID:16162670; http://dx.doi.org/10.1073/pnas.0505020102
- Mancuso M, Pasquali E, Leonardi S, Tanori M, Rebessi S, Di Majo V, Pazzaglia S, Toni MP, Pimpinella M, Covelli V, et al. Oncogenic bystander radiation effects in Patched heterozygous mouse cerebellum. Proc Nat Acad Sci U S A 2008; 105:12445-50; PMID:18711141; http://dx.doi.org/10.1073/pnas.0804186105
- Azzam EI, de Toledo SM, Little JB. Stress signaling from irradiated to non-irradiated cells. Curr Cancer Drug Targets 2004; 4:53-64; PMID:14965267; http://dx.doi.org/10.2174/1568009043481641
- Hei TK. Cyclooxygenase-2 as a signaling molecule in radiation-induced bystander effect. Mol Carcin 2006; 45:455-60; PMID:16637062; http://dx.doi.org/10.1002/mc.20219
- Huang L, Snyder AR, Morgan WF. Radiation-induced genomic instability and its implications for radiation carcinogenesis. Oncogene 2003; 22:5848-54; PMID:12947391; http://dx.doi.org/10.1038/sj.onc.1206697
- Kassis AI. In vivo validation of the bystander effect. Hum Exp Toxicol 2004; 23:71-3; PMID:15070063; http://dx.doi.org/10.1191/0960327104ht420oa
- Seymour CB, Mothersill C. Delayed expression of lethal mutations and genomic instability in the progeny of human epithelial cells that survived in a bystander-killing environment. Radiat Oncol Invest 1997; 5:106-10; PMID:9303065; http://dx.doi.org/10.1002/(SICI)1520-6823(1997)5:3%3c106::AID-ROI4%3e3.0.CO;2-1
- Lyng FM, Seymour CB, Mothersill C. Production of a signal by irradiated cells which leads to a response in unirradiated cells characteristic of initiation of apoptosis. Brit J Cancer 2000; 83:1223-30; PMID:11027437; http://dx.doi.org/10.1054/bjoc.2000.1433
- Nagar S, Smith LE, Morgan WF. Characterization of a novel epigenetic effect of ionizing radiation: the death-inducing effect. Cancer Res 2003; 63:324-8; PMID:12543783
- Lewis DA, Mayhugh BM, Qin Y, Trott K, Mendonca MS. Production of delayed death and neoplastic transformation in CGL1 cells by radiation-induced bystander effects. Radiat Res 2001; 156:251-8; PMID:11500134; http://dx.doi.org/10.1667/0033-7587(2001)156%5b0251:PODDAN%5d2.0.CO;2
- Yang H, Asaad N, Held KD. Medium-mediated intercellular communication is involved in bystander responses of X-ray-irradiated normal human fibroblasts. Oncogene 2005; 24:2096-103; PMID:15688009; http://dx.doi.org/10.1038/sj.onc.1208439
- Shao C, Stewart V, Folkard M, Michael BD, Prise KM. Nitric oxide-mediated signaling in the bystander response of individually targeted glioma cells. Cancer Res 2003; 63:8437-42; PMID:14679007
- Narayanan PK, Goodwin EH, Lehnert BE. Alpha particles initiate biological production of superoxide anions and hydrogen peroxide in human cells. Cancer Res 1997; 57:3963-71; PMID:9307280
- Zhou H, Ivanov VN, Lien YC, Davidson M, Hei TK. Mitochondrial function and nuclear factor-kappaB-mediated signaling in radiation-induced bystander effects. Cancer Res 2008; 68:2233-40; PMID:18381429; http://dx.doi.org/10.1158/0008-5472.CAN-07-5278
- Chaudhry MA, Omaruddin RA. Differential regulation of microRNA expression in irradiated and bystander cells. Molekuliarnaia Biologiia 2012; 46:634-43; PMID:23113353
- Bartel DP. MicroRNAs: target recognition and regulatory functions. Cell 2009; 136:215-33; PMID:19167326; http://dx.doi.org/10.1016/j.cell.2009.01.002
- Foshay KM, Gallicano GI. Small RNAs, big potential: the role of MicroRNAs in stem cell function. Curr Stem Cell Res Ther 2007; 2:264-71; PMID:18220910; http://dx.doi.org/10.2174/157488807782793781
- Carleton M, Cleary MA, Linsley PS. MicroRNAs and cell cycle regulation. Cell Cycle 2007; 6:2127-32; PMID:17786041; http://dx.doi.org/10.4161/cc.6.17.4641
- Jovanovic M, Hengartner MO. miRNAs and apoptosis: RNAs to die for. Oncogene 2006; 25:6176-87; PMID:17028597; http://dx.doi.org/10.1038/sj.onc.1209912
- Fabbri M, Garzon R, Cimmino A, Liu Z, Zanesi N, Callegari E, Liu S, Alder H, Costinean S, Fernandez-Cymering C, et al. MicroRNA-29 family reverts aberrant methylation in lung cancer by targeting DNA methyltransferases 3A and 3B. Proc Nat Acad Sci U S A 2007; 104:15805-10; PMID:17890317; http://dx.doi.org/10.1073/pnas.0707628104
- Hu H, Gatti RA. MicroRNAs: new players in the DNA damage response. J Mol Cell Biol 2011; 3:151-8; PMID:21183529; http://dx.doi.org/10.1093/jmcb/mjq042
- Koturbash I, Zemp FJ, Kutanzi K, Luzhna L, Loree J, Kolb B, Kovalchuk O. Sex-specific microRNAome deregulation in the shielded bystander spleen of cranially exposed mice. Cell Cycle 2008; 7:1658-67; PMID:18560276; http://dx.doi.org/10.4161/cc.7.11.5981
- Koturbash I, Boyko A, Rodriguez-Juarez R, McDonald RJ, Tryndyak VP, Kovalchuk I, Pogribny IP, Kovalchuk O. Role of epigenetic effectors in maintenance of the long-term persistent bystander effect in spleen in vivo. Carcinogenesis 2007; 28:1831-8; PMID:17347136; http://dx.doi.org/10.1093/carcin/bgm053
- Kovalchuk O, Zemp FJ, Filkowski JN, Altamirano AM, Dickey JS, Jenkins-Baker G, Marino SA, Brenner DJ, Bonner WM, Sedelnikova OA. microRNAome changes in bystander three-dimensional human tissue models suggest priming of apoptotic pathways. Carcinogenesis 2010; 31:1882-8; PMID:20643754; http://dx.doi.org/10.1093/carcin/bgq119
- Dickey JS, Zemp FJ, Martin OA, Kovalchuk O. The role of miRNA in the direct and indirect effects of ionizing radiation. Radiat EnvironBiophys 2011; 50:491-9; PMID:21928045; http://dx.doi.org/10.1007/s00411-011-0386-5
- Hanson EK, Lubenow H, Ballantyne J. Identification of forensically relevant body fluids using a panel of differentially expressed microRNAs. Analytical Biochem 2009; 387:303-14; PMID:19454234; http://dx.doi.org/10.1016/j.ab.2009.01.037
- Ai J, Zhang R, Li Y, Pu JL, Lu YJ, Jiao JD, Li K, Yu B, Li Z, Wang R, et al. Circulating microRNA-1 as a potential novel biomarker for acute myocardial infarction. Biochem Biophys Res Commun 2010; 391:73-7; PMID:19896465; http://dx.doi.org/10.1016/j.bbrc.2009.11.005
- Michael A, Bajracharya SD, Yuen PST, Zhou H, Star RA, Illei GG, Alevizos I. Exosomes from human saliva as a source of microRNA biomarkers. Oral Dis 2010; 16:34-8; PMID:19627513; http://dx.doi.org/10.1111/j.1601-0825.2009.01604.x
- Hanke M, Hoefig K, Merz H, Feller AC, Kausch I, Jocham D, Warnecke JM, Sczakiel G. A robust methodology to study urine microRNA as tumor marker: microRNA-126 and microRNA-182 are related to urinary bladder cancer. Urol Oncol-Semin Ori 2010; 28:655-61; PMID:19375957; http://dx.doi.org/10.1016/j.urolonc.2009.01.027
- Valadi H, Ekstrom K, Bossios A, Sjostrand M, Lee JJ, Lotvall JO. Exosome-mediated transfer of mRNAs and microRNAs is a novel mechanism of genetic exchange between cells. Nat Cell Biol 2007; 9:654-U72; PMID:17486113; http://dx.doi.org/10.1038/ncb1596
- Wang K, Zhang S, Weber J, Baxter D, Galas DJ. Export of microRNAs and microRNA-protective protein by mammalian cells. Nucleic acids Res 2010; 38:7248-59; PMID:20615901; http://dx.doi.org/10.1093/nar/gkq601
- Kosaka N, Iguchi H, Yoshioka Y, Takeshita F, Matsuki Y, Ochiya T. Secretory mechanisms and intercellular transfer of microRNAs in living cells. J Biol Chem 2010; 285:17442-52; PMID:20353945; http://dx.doi.org/10.1074/jbc.M110.107821
- Chen X, Ba Y, Ma LJ, Cai X, Yin Y, Wang KH, Guo J, Zhang Y, Chen J, Guo X, et al. Characterization of microRNAs in serum: a novel class of biomarkers for diagnosis of cancer and other diseases. Cell Res 2008; 18:997-1006; PMID:18766170; http://dx.doi.org/10.1038/cr.2008.282
- Mitchell PS, Parkin RK, Kroh EM, Fritz BR, Wyman SK, Pogosova-Agadjanyan EL, Peterson A, Noteboom J, O'Briant KC, Allen A, et al. Circulating microRNAs as stable blood-based markers for cancer detection. Proc Nat Acad Sci U S A 2008; 105:10513-8; PMID:18663219; http://dx.doi.org/10.1073/pnas.0804549105
- Mothersill C, Seymour C. Medium from irradiated human epithelial cells but not human fibroblasts reduces the clonogenic survival of unirradiated cells. Int J Radiat Biol 1997; 71:421-7; PMID:9154145; http://dx.doi.org/10.1080/095530097144030
- Sokolov MV, Smilenov LB, Hall EJ, Panyutin IG, Bonner WM, Sedelnikova OA. Ionizing radiation induces DNA double-strand breaks in bystander primary human fibroblasts. Oncogene 2005; 24:7257-65; PMID:16170376; http://dx.doi.org/10.1038/sj.onc.1208886
- Chen X, Li Q, Wang J, Guo X, Jiang X, Ren Z, Weng C, Sun G, Wang X, Liu Y, et al. Identification and characterization of novel amphioxus microRNAs by Solexa sequencing. Genome Biol 2009; 10:R78
- Wang P, Zou F, Zhang X, Li H, Dulak A, Tomko RJ Jr, Lazo JS, Wang Z, Zhang L, Yu J. microRNA-21 negatively regulates Cdc25A and cell cycle progression in colon cancer cells. Cancer Res 2009; 69:8157-65; PMID:19826040; http://dx.doi.org/10.1158/0008-5472.CAN-09-1996
- Si ML, Zhu S, Wu H, Lu Z, Wu F, Mo YY. miR-21-mediated tumor growth. Oncogene 2007; 26:2799-803; PMID:17072344; http://dx.doi.org/10.1038/sj.onc.1210083
- Pan Q, Luo X, Chegini N. microRNA 21: response to hormonal therapies and regulatory function in leiomyoma, transformed leiomyoma and leiomyosarcoma cells. Mol Hum Reproduction 2010; 16:215-27; PMID:19906824; http://dx.doi.org/10.1093/molehr/gap093
- Zhang X, Ng WL, Wang P, Tian L, Werner E, Wang H, Doetsch P, Wang Y. MicroRNA-21 modulates the levels of reactive oxygen species by targeting SOD3 and TNFalpha. Cancer Res 2012; 72:4707-13; PMID:22836756; http://dx.doi.org/10.1158/0008-5472.CAN-12-0639
- Prise KM, Folkard M, Michael BD. Bystander responses induced by low LET radiation. Oncogene 2003; 22:7043-9; PMID:14557809; http://dx.doi.org/10.1038/sj.onc.1206991
- Markova E, Schultz N, Belyaev IY. Kinetics and dose-response of residual 53BP1gamma-H2AX foci: co-localization, relationship with DSB repair and clonogenic survival. Int J Radiat Biol 2007; 83:319-29; PMID:17457757; http://dx.doi.org/10.1080/09553000601170469
- Shao C, Folkard M, Prise KM. Role of TGF-beta1 and nitric oxide in the bystander response of irradiated glioma cells. Oncogene 2008; 27:434-40; PMID:17621264; http://dx.doi.org/10.1038/sj.onc.1210653
- Arroyo JD, Chevillet JR, Kroh EM, Ruf IK, Pritchard CC, Gibson DF, Mitchell PS, Bennett CF, Pogosova-Agadjanyan EL, Stirewalt DL, et al. Argonaute2 complexes carry a population of circulating microRNAs independent of vesicles in human plasma. Proc Nat Acad Sci U S A 2011; 108:5003-8; PMID:21383194; http://dx.doi.org/10.1073/pnas.1019055108
- Vickers KC, Palmisano BT, Shoucri BM, Shamburek RD, Remaley AT. MicroRNAs are transported in plasma and delivered to recipient cells by high-density lipoproteins. Nat Cell Biol 2011; 13:423-33; PMID:21423178; http://dx.doi.org/10.1038/ncb2210
- Yang M, Chen JQ, Su F, Yu B, Su FX, Lin L, Liu Y, Huang JD, Song E. Microvesicles secreted by macrophages shuttle invasion-potentiating microRNAs into breast cancer cells. Mol Cancer 2011; 10:117; PMID:21939504; http://dx.doi.org/10.1186/1476-4598-10-117
- Jacobs J, Jones C, Baille J. Characteristics of a human diploid cell designated MRC-5. Nature 1970; 227:168-70; PMID:4316953
- Pei H, Chen W, Hu W, Zhu M, Liu T, Wang J, Zhou G. GANRA-5 protects both cultured cells and mice from various radiation types by functioning as a free radical scavenger. Free radical research 2014; 48:670-8; PMID:24580122; http://dx.doi.org/10.3109/10715762.2014.898843
- Cowell IG, Sunter NJ, Singh PB, Austin CA, Durkacz BW, Tilby MJ. γH2AX foci form preferentially in euchromatin after ionising-radiation. PloS One 2007; 2:e1057; PMID:17957241; http://dx.doi.org/10.1371/journal.pone.0001057
- Chang L, Hu W, Ye C, Yao B, Song L, Wu X, Ding N, Wang J, Zhou G. miR-3928 activates ATR pathway by targeting Dicer. RNA Biol 2012; 9:1247-54; PMID:22922797; http://dx.doi.org/10.4161/rna.21821