Abstract
Retroviral nucleocapsid (NC) is central to viral replication. Nucleic acid chaperoning is a key function for NC through the action of its conserved basic amino acids and zinc-finger structures. NC manipulates genomic RNA from its packaging in the producer cell to reverse transcription into the infected host cell. This chaperone function, in conjunction with NC’s aggregating properties, is up-modulated by successive NC processing events, from the Gag precursor to the fully mature protein, resulting in the condensation of the nucleocapsid within the capsid shell. Reverse transcription also depends on NC processing, whereas this process provokes NC dissociation from double-stranded DNA, leading to a preintegration complex (PIC), competent for host chromosomal integration. In addition NC interacts with cellular proteins, some of which are involved in viral budding, and also with several viral proteins. All of these properties are reviewed here, focusing on HIV-1 as a paradigmatic reference and highlighting the plasticity of the nucleocapsid architecture.
Introduction: NC and Viral Replication—Scope of this Special Review
In this review, attention is given to retroviral nucleocapsid (NC) proteins. These remarkable nonspecific RNA/DNA binding polypeptides modulate the thermodynamic folding pathways of nucleic acids, thereby orchestrating the sophisticated RNA-DNA choreography involved in retroviral biology. Our goal is to focus on two aspects of NC in the context of both its nucleic acid chaperone, and intravirion architectural modulating functions that are critical throughout viral assembly and replication. The first aspect discusses the strong requirement for conserved biochemical and structural features, the basic character of NC and its zinc finger or zinc knuckle (ZF) domains. The second presents contrasting considerations on the chaperone status of the NC domain as member of the Gag precursor compared with processed forms, highlighting the consequences of viral protease (PR) processing in RNA-NC interactions.
Similar strategies are employed by NC proteins from different retroviruses, with the notable exception of the spumaretroviruses that maintain their NC in a precursor form throughout replication. Insights into these strategies have been remarkable from the published works over the past several years, with subtle variations in (i) NC sequences, (ii) processing and (iii) RNA/DNA chaperone properties. These insights become more obvious after a thorough comparison of the paradigmatic NC proteins studied in the human immunodeficiency virus type 1 (HIV-1), simian immunodeficiency virus (SIV), murine leukemia virus (MuLV), Rous sarcoma virus (RSV) and human T-cell leukemia virus type 1 (HTLV-1) retroviral systems. This review will largely be based on HIV-1, comparing and contrasting it with the other retroviruses where possible. Additional aspects regarding NC interactions with viral and cellular proteins, and alternative nucleic acid chaperones will be discussed, providing some perplexing aspects to be considered. illustrates the HIV-1 replicative cycle showing where NC is directly involved, from Gag-RNA assemblies until the completion of reverse transcription.
NC Proteins
Retroviral NC proteins were first observed as the major protein component of reverse transcription-competent ribonucleoprotein (RNP) complexes purified from virions of avian sarcoma-leukosis virus and MuLV.Citation1 Once isolated, NC was characterized as a nucleic acid-binding protein with preference for single-stranded sequences within structured RNA domains.Citation2 Subsequently, NC was shown to be a major driving force in genomic RNA selection, dimerization and packaging during MuLV, RSV and HIV-1 assembly.Citation3–Citation6 NC was also found to direct viral RNA dimerization in vitro and annealing of the primer tRNA to the genome's primer-binding site, both in vitro and in several retroviral systems.Citation7,Citation8 These observations led to the discovery that NC was both the major architectural component of the RNP core complex and a RNA-DNA chaperone protein necessary for bona fide proviral DNA synthesis. These capabilities are now explained by the ability of NC to accelerate the most thermodynamically stable base-paired nucleic acid structures, which provokes both nucleic acid condensation into a RNP complex (i.e., the nucleocapsid) and configuration of RNA tertiary and quaternary structures required for successful reverse transcription (reviewed by J. G. Levin and K. Musier-Forsyth in this special issue). Over the years, specific features of NC have been well characterized: the NC ZFs function to destabilize nucleic acids base pairs, and the basic amino acids aid in aggregating RNA or DNA strands. Several in-depth reviews have described work on the basic amino acid residues and ZF structures in nucleic acid chaperone function.Citation9–Citation12 Due to pleiotropic effects of mutations to NC in cell culture, the best methods for gaining insights into NC's nucleic acid chaperone function still rely on model in vitro systems, which employ a wide array of biochemical, molecular biology and biophysical techniques and technologies.
Structural features of NC.
The N-terminal basic domain. The nucleocapsid proteins from the Retroviridae (http://www.ictvonline.org/virusTaxonomy.asp?version=2009) have a feature in common: high densities of basic amino acids that facilitate interactions with nucleic acids. Both the Orthoretrovirinae and Spumaretrovirinae have this feature. In general, the basic amino acids, Lys and Arg are involved in aggregating and annealing of nucleic acids.Citation13,Citation14 This strand aggregation ability is essential for facilitating the bimolecular nucleation step of strand annealing. The nature of this effect is widely discussed in the literature and is essentially electrostatic, due to the charge complementarities between nucleic acid backbones and the positively charged residues. In HIV-1, the basic residues of the N-terminal domain form a 310 helix, which optimizes interaction with the grooves of hairpin structures.Citation15,Citation16 Together with the N-terminal tail, the basic residues in the linker region between the HIV-1 NC ZFs contribute to robust (high affinity) but dynamic (rapid kinetics) binding/dissociation interactions with nucleic acids, which ensures nucleic acid aggregation and RNP energy minimization.Citation17–Citation20 Both effects are particularly apparent during minus-strand-transfer, from the accelerated annealing of minus-strand strong-stop DNA (containing a copy of the 5′-R region) to the 3′-R-RNA region of the genome.Citation21–Citation23 In agreement, deletion of basic residues or elevation of the buffer ionic strength significantly decrease the affinity of the protein toward nucleic acids.Citation24 Interestingly, a recent molecular dynamics simulation study noted that the 310 helix provides a highly basic surface that could represent a nucleic acid dimerization site,Citation25 which may explain the observation that HIV-1 NC has multiple nucleic acid-binding sites that allow intra- and intermolecular interactions.Citation26 Most mature retroviral NC proteins have isoelectric points in the 10–11 range except for HTLV-1 and -2, which are neutral due to a patch of acidic amino acid residues in the C-terminal half of the proteins. NC proteins typically are highly flexibleCitation27–Citation29 except for the conserved globular zinc-binding structure discussed next.
The ZF domain. A major distinction between the Ortho- and Spuma-retrovirinae subfamilies is that only the former contains a highly conserved element, a mini-globular zinc binding domain, commonly referred to as a ZF.Citation30,Citation31 Divided further, the Gammaretroviruses and Epsilonretroviruses (e.g., MuLV [GenBank accession no.: J02255] and walleye dermal sarcoma virus [GenBank accession no.: L41838], respectively) contain a single ZF in their NC proteins, with all other Orthoretrovirinae containing two of these zinc binding motifs. These structures are remarkably conserved with a general amino acid sequence of: -Cys-X2-Cys-X4-His-X4-Cys- (CCHC), where X are variable amino acids. Typically there are one or two aromatic amino acids within the N-terminal loop, a Gly residue just before the conserved His residue and a carbonyl-containing residue, just prior to the C-terminal Cys residueCitation30,Citation31 in retroviral NC ZFs. More details about NC structure are available in Y. Mely's review in this issue.
NC chaperone function.
As shown in for HIV-1, RSV and MuLV NC, the primary function of this mini-globular metal binding domain is to bind specifically to exposed (unpaired) nucleobases of RNA or DNA. Folding into knuckle structures exposes a hydrophobic pocket centered on aromatic and several non polar residues, forming a cavity, which allows the insertion of a nucleobase (preferentially a guanosine). In free solution, there are transient interactions between these structures in HIV-1 NC, mediated through aromatic residues.Citation29,Citation32 The capability of HIV-1 NC to destabilize nucleic acid duplexes relies on its preferential binding to unpaired nucleic acids (single-stranded regions of RNA or DNA) via the capture of a guanosine by a cavity formed by two aromatic residues located on each ZF.Citation33–Citation35 The complex is stabilized by a network of hydrogen bonds, π-cation and π-π interactions with residues forming the cavity. When bound to nucleic acids, nucleobase capture is critical for destabilization, a process that is necessary for the many nucleic acid rearrangements that take place during viral replication. The culmination of the destabilization and annealing activities constitutes NC's nucleic acid chaperone activity.Citation10–Citation12 By this mechanism, melting by NC, of short helical segments bordered by mismatches or bulges provides a single-stranded nucleation site for the formation of a new, double-stranded helix with a neighboring single-stranded region. In addition, NC-induced duplex destabilization accelerates various processes of strand switching and strand transfers.Citation10 Studies on other NC proteins and precursors remarkably support this mechanism. For example, RSV NC is an even better aggregating agent and exhibits rapid binding kinetics, but is a relatively poor duplex destabilizer. On the other hand, MuLV NC aggregates and destabilizes duplexes effectively; however, due to its slow dissociation kinetics, the protein is a less effective chaperone.Citation19 As illustrated in , for each NC, these characteristics are subtly tuned by variations in the nucleobase binding module (i.e., the ZF) and short, semi-structured basic residue-rich regions.
Most of the early work examining the role of NC has been performed comparing mutant and wild-type HIV-1 NC (NCp7 [55 amino acid form] or NCp9 [71 or 72 amino acid form; NCp7+SP2]) or MuLV NCp10 in in vitro assays. In addition, several virus-based assays have been performed to examine the properties of NC at various points in the viral replication cycle. Kafaie and coworkers examined an extensive collection of NC mutations and NC precursor forms on genome dimer maturation,Citation36,Citation37 a process that relies on NC's RNA chaperone function.Citation3,Citation38,Citation39 It was demonstrated in this virus-based system that basic residues in the N-terminal and the linker regions, as well as the two ZFs were necessary for efficient genome dimerization. However there were a number of the mutations that also affected Gag processing and it was shown that processing per se can affect dimer maturation.Citation36 Thus it is difficult to determine whether the mutations themselves or Gag processing defects were responsible for the effect on RNA chaperone-driven dimer maturation.
Recent in vitro studies have started to compare and contrast the nucleic acid chaperone properties of NC proteins from several retroviral Genera including HIV-1 NCp7, MuLV NCp10, RSV NCp12 and HTLV-1 NCp15. The properties of these NC's were characterized using a number of different analyses. The effectiveness of annealing falls into the rank order of HIV-1 and RSV > MuLV >> HTLV-1, which was assessed by gel-shift assays of a mini-TAR DNA/RNA system that mimics the minus-strand transfer process.Citation19 Additionally, HTLV-1 NCp15 was shown by FRET analysis to facilitate annealing of a complementary DNA very poorly.Citation40 Aggregation of nucleic acids, demonstrated by sedimentation showed that the HIV-1, RSV and MuLV NC proteins were equally effective at saturating protein levels, however HTLV-1 NC was a poor aggregator, the reasons for which will become apparent below.Citation19
Effective destabilization afforded by these proteins typically relates to their higher relative binding affinities to single-stranded versus double-stranded nucleic acids. Typically single-stranded bulge regions in nucleic acid stems are starting points for destabilization as mentioned above.Citation41 All four NC proteins have similar destabilization free energies, all favoring binding to single-stranded nucleic acids; fluorescence anisotropy measurements of NCs from various viruses showed that HTLV-1 > RSV ≈ MuLV > HIV-1 in this regard. Thus even though HTLV-1 NC is ineffective at aggregating nucleic acids, it appears to be the best destabilizer. Rapid binding/dissociation rates also contribute to the balance between annealing and destabilization, and thus overall chaperone effectiveness. Single-molecule DNA stretching studies provide excellent insights into the kinetics of binding/dissociation as well as information regarding destabilization; this is discussed in detail in the review of M.C. Williams and I. Rouzina in this issue. HIV-1 and RSV NCs both have rapid on/off rates from single-stranded nucleic acids, MuLV NC's dissociation is much slower accounting for its reduced annealing capacity; HTLV-1 NC has the slowest dissociation rate from single-stranded DNA (ssDNA), which contributes to its poor chaperone function.Citation19
HTLV-1 NCp15 is a particularly poor nucleic acid chaperone due, in part, to its overall neutral charge at physiological pH and its inability to aggregate nucleic acids. However if the C-terminal acidic tail is removed (ΔC29), chaperone activity can be restored to levels seen with the other retroviral NC proteins. Additionally, binding/dissociation kinetics became much more rapid. Curiously, increasing the ionic strength also improved chaperone function of the native protein, leading to the hypothesis that an ionic interaction between the N-terminal basic and C-terminal acidic domains was occurring. ΔC29 HTLV-1 NC was examined for its annealing activity, which was reduced with increasing amounts of added C-terminal peptide; when the ionic strength was increased, annealing was again restored. Additional evidence for the detrimental effects of the C-terminal tail on chaperone function came from examination of an HIV-1 NCp7/HTLV-1 C-terminal tail chimera that had significantly reduced annealing activity.Citation42
Spumaretroviruses as an exception.
Since their discovery, the spumaretroviruses, such as the prototype foamy retrovirus (PFV) have gained the attention of retrovirologists as they retain unique properties during replication, especially at the level of the budding and entry cycle.Citation43 Characterization of the PFV NC-like domain also revealed unique properties.Citation44 It is logically found at the C-terminus of Gag, but is not processed as a separated protein during Gag maturation, and there are no ZFs to contribute to RNA binding or destabilization. The nucleic acid binding properties of the PFV NC-like domain have been briefly described, with non-specific nucleic acid binding being attributed to arginine-rich regions (Gly-Arg motifs, which are also found in RSV NC). These motifs were shown to be necessary for PFV RNA and Pol packaging.Citation45 The RNA chaperone activity of this particular NC has not been assessed, but should be absolutely necessary for assistance with reverse transcription, which is similar to the HIV-1 reverse transcription process although its spatiotemporal regulation is different.Citation46 PFV reverse transcription is indeed performed predominantly prior to budding, resulting in a DNA-containing virus like HBV.Citation47 Moreover, the critical Gag cleavage, which separates the N-terminal moiety, required for viral assembly and egress, from the C-terminus, has been shown to occur after reverse transcription and cell entry, providing for a unique PFV capsid uncoating mechanism.Citation48
NC in Retroviral Replication
The number of NC domain-containing Gag precursors is largely dependent on virion size, but there is considerable debate as to the actual quantity. However, the quantity of NC domains associated with the genome dimer in an immature HIV-1 virion has been estimated to be around 3,000 and approximately 2,000 processed NC molecules are associated with the RNA in the mature particle.Citation49 The NC:RNA molar ratio is therefore not far from 1 for ∼10 nucleotides, a value that is very similar to the ratio required in vitro for optimal efficiency in all assays where NC affords effective assistance.Citation50 This ratio also typically drives efficient coaggregation of NC with nucleic acidsCitation18,Citation51 and one could speculate that the NC domain aggregates with its genomic RNA partner in a macromolecular crowding context, from Gag assembly, formation of the mature nucleocapsid complex, to the reverse transcription complex (RTC). This aspect is common among both RNA chaperones and nucleic-acid architectural proteins.Citation52
NC from most retroviruses functions in both precursor and fully processed forms throughout the replication cycle. Typically, in precursor forms (i.e., Pr55Gag for HIV-1 or Pr65Gag for MuLV), NC is involved in assembly processes, specifically recognizing and binding genomes as RNA dimers.Citation53,Citation54 The precursors are also involved in placement of the tRNA primer onto genomes.Citation38,Citation55 With processing of Gag by PR, successively smaller precursors containing the NC domain are generated until fully processed NC proteins are liberated, as illustrated in the cases of HIV-1 and MuLV (). The functions and characteristics of these precursors will be discussed further below.
Assembly functions of NC as an internal domain of Gag.
The HIV-1 Gag polyprotein () acts essentially as an assembly machine performing simultaneous membrane binding, RNA packaging and cofactor recruitment (for details, see reviews from A. Rein, D. Muriaux and J. L. Darlix, M. C. Williams and I. Rouzina and J. G. Levin and K. Musier-Forsyth in this issue). The NC domain of Gag plays a direct role by selecting two copies of viral RNA from a large pool of cellular RNA molecules. This is accomplished through the specific binding of several NC domains of Gag, with the four RNA stem loops (termed Ψ) that dimerize through self-complementarity. Structures of NC in complex with two of the isolated stem loops from HIV-1 Ψ show that RNA recognition is supported by specific contacts between the basic residues and ZFs, with exposed loop nucleotides (). For MuLV, an elegant mechanism combines genome dimerization and packaging in which the high-affinity binding site for NC becomes exposed upon dimerization.Citation56–Citation58 In HIV-1, the binding of several NC domains to the Ψ RNA region is thought to initiate particle assembly by congregating Gag on the RNA dimer, which then acts as a nucleation point for aggregation of other Gag units through Gag-Gag interactions and NC/RNA binding.Citation59–Citation61 Loading of Gag and Gag-Pol on the RNA, which functions as a scaffold for self-assembly of the particle, presumably relies on specific and nonspecific interactions between NC domains and RNA stems and the backbone.Citation15 Although NC is believed to be the major determinant for binding to nucleic acids during retroviral assembly, participation of a basic amino acid patch in matrix (MA) has also been implicated in the case of HIV-1,Citation62–Citation64 and deltaretroviruses.Citation65 Accordingly, the DNA binding constant of recombinant GagΔp6 is 10-fold higher than either NCp15, NCp9 or NCp7, as it is stabilized by additional Gag-Gag interactions,Citation17,Citation66 facilitated through the capsid (CA) domain.Citation67 Gag has not been shown to facilitate more complex nucleic acid rearrangements, other than tRNAlys3 annealing to the primer binding site (PBS). In contrast, NCp7 is solely capable of facilitating minus-strand transfer,Citation21,Citation68 although in vitro, at low concentrations, Gag (with p6 deleted) can do this as well.Citation67
From Gag processing to nucleocapsid condensation.
Maturation of the Gag/gRNA nucleoprotein complex results in condensation and establishment of a RNP architecture that is able to support bona fide reverse transcriptase (RT)-catalyzed viral DNA synthesis, with subsequent conversion to a pre-integration complex. Immediately after budding, the C-terminal region of CA, the adjacent spacer peptide (SP1) and NC appear to form a continuous assembly domain, essential for formation of the immature virion.Citation69 In this context, the NC domain of Gag and RNA delineate the innermost layer of the immature virion (). Separation of the RNA-associated NC is the first step in Gag polyprotein cleavage, which neutralizes this assembly domain, separating the CA and NC domains and allowing condensation of the RNP complex that may subsequently function as a substrate for CA condensation.Citation69 The sequential processing of the NC polypeptide by PR then correlates with the improvement of its chaperone character, presumably by increasing the flexibility of the NC and consequently increasing its binding/dissociation dynamics with nucleic acids.Citation17,Citation70 Remarkably, RNA bound to Gag or the various NC intermediates strongly stimulates PR activity, ensuring complete processing of the precursors.Citation71–Citation73 Destabilization of folded complementary sequences, activation of intermolecular base-pairing, sliding of NC, capture of multiple strands,Citation26,Citation74 and neutralization of RNA backbone repulsions by the polycationic character of NC then drives aggregation/condensation of the RNP complex towards the most thermodynamically stable architecture.Citation17,Citation51,Citation75 Simultaneously, RNP condensation drives gRNA dimerizationCitation36,Citation37 and presumably configures the proper RT pre-initiation complex.Citation69,Citation76,Citation77
Several recent studies have shown that an essential step of this aggregative process is the separation of the p6 moiety, as NCp15 does not aggregate with either ssDNACitation51,Citation71 or RNA (Lyonnais S, unpublished result) and dissociates more slowly than NCp7 from ssDNA.Citation17,Citation70 In contrast, NCp9 binds and dissociates much more rapidly, with moderate cooperativity and promotes extensive RNA and ssDNA aggregation.Citation18,Citation20,Citation51 Finally, NCp7 appears to fulfill the optimal functionality, i.e., high mobility, to induce both nonspecific aggregation and facilitate the diffusional search of complementary nucleic acid regions.
The impact of maintaining NC in its precursor forms, NCp9 and NCp15, has also been studied. In virions, where NC is prevented from progressing past the NCp15 precursor, genome dimerization is significantly disrupted.Citation36 Reverse transcription processes in this mutant, from minus-strand strong-stop syntheses to the plus-strand transfer event occur at near wild-type levels. However, conversion of plus-strand transfer products to 2-LTR circles is significantly reduced, due either to inefficient strand-displacement synthesis or NCp15 steric obstruction of the viral DNA ends to nuclear ligases; there are severe defects in integration, thus the mutant is replication defective.Citation78 In mutant virions that maintain the NCp9 form, genome dimer formation and reverse transcription are not inhibited significantly, thus some insights into NC's nucleic acid chaperone function have been obtained from these HIV-1 based studies. As indicated above, due to the pleiotropic effects of mutations to NC, it is often difficult to interpret results in virus-based systems. On the other hand, clinical studies have shown that NC-p1 and p1–p6 regions are preferred sites, outside of PR, that acquire compensatory mutations associated with HIV-1 protease inhibitor resistance,Citation79 the reasons for which require further investigation.
Reverse transcription.
RTCs evolve from within the nucleocapsid after introduction into the newly infected cell.Citation80 Due to its nucleic acid chaperone activity, HIV-1 NCp7 efficiently assists RT in defined critical events such as tRNALys3-directed initiation, the two obligate strand transfers and central DNA flap termination.Citation10,Citation12 Details regarding NCp7's function as a nucleic acid chaperone in reverse transcription and the implications of this activity are reviewed in this issue by J.G. Levin and K. Musier-Forsyth.
NC processing and reverse transcription. The loss of HIV-1 infectivity, observed under suboptimal concentrations of protease inhibitors has shown a predominant effect on reverse transcription, suggesting that CA and NC must be efficiently separated by PR.Citation81 The same effects are observed with mutations that suppress Gag cleavage sites: trans-complementation of wild-type virus with a low level of a Gag mutant that does not complete NC maturation strongly affects wild-type reverse transcription.Citation81 Taken together, these results suggests that at a minimum, NC maturation generating a species with the proper N-terminus (i.e., NCp15) is required in order to optimize the reverse transcription process, with two obvious consequences: (i) to favor the RNA/DNA chaperone activities required for the critical reverse transcription steps and (ii) to promote the RT-driven displacement of bound NC along the template. Non-processed Gag bound to a template would most likely be difficult to displace, similarly to that already shown for bound APOBEC3G.Citation82 In support of this displacement hypothesis, a recent study showed that precursor forms of Gag, containing NC, not separated from CA could prevent reverse transcription at a threshold concentration much lower than peptides without an attached CA domain.Citation67 In contrast, as mentioned above, mutations that impede C-terminal NC processing seem to not substantially affect reverse transcription, however preventing cleavage of the p1–p6 site strongly inhibits single-cycle viral infectivity at or near the completion of reverse transcription.Citation78
Reverse transcription and NC dissociation. Within purified HIV-1 RTCs and PICs, CA and NCp7 appear predominantly to dissociate from the full-length linear viral double-stranded DNA (dsDNA) extracted from infected cells, in contrast to the efficient retention of integrase (IN) and viral protein R (Vpr).Citation83–Citation85 Capsid uncoating has been shown to be critical for the generation of an active PIC.Citation86 The apparent loss of NCp7 also suggests that nucleocapsid disassembly and PIC assembly are directly interconnected during the reverse transcription process.
Changes in the HIV-1 nucleocapsid architecture have not been observed during reverse transcription processes in infected cells due to the difficulty in examining such a highly dynamic process. The mechanism demonstrating the loss of NCp7 arose from in vitro experiments: during DNA synthesis, RT sliding provokes NCp7 displacement from the template, whereas re-association with dsDNA appears severely limited, most likely due to a reduced affinity of NCp7 for dsDNA compared to single-stranded nucleic acids under ionic conditions for optimal RT activity, in vitro.Citation51,Citation71 It is interesting to note that parallel studies indicate that MuLV NCp10 strongly binds to both ss- and dsDNA, whereas it is not displaced by, and is also an inhibitor of HIV-1 RT,Citation71 suggesting that RT and NC are tailored to one another, to manage NC dismantling from the nucleocapsid. Moreover, strategies of NC dismantling seem to be quite variable depending upon the class of retrovirus. In the case of HIV-1, this dismantling may favor IN and Vpr sequestration on dsDNA and the recruitment of suitable cellular dsDNA binding proteins.Citation84 Finally, NCp7 seems easier to remove than NCp9 from nucleoprotein complexes in vitro,Citation71 suggesting that p1 removal prior to initiation of reverse transcription would favor a more efficient RTC to PIC transition.
Thus, two alternative modes have been proposed to disrupt the HIV-1 nucleocapsid architecture in the context of reverse transcription within the cell:Citation71 (i) dismantling of NC from RTCs, restricted to the plus-strand synthesis step resulting in the generation of dsDNA or (ii) progressive displacement of NC associated with RT translocation throughout the process. The first mode is supported by the in vitro aggregation of NCp7 with the ssRNA or ssDNA products observed during minus-strand synthesis,Citation77,Citation87 suggesting that NCp7 dissociation requires dsDNA. In contrast, the second mode could be supported by an additional dispersal force through the action of the RT-associated RNAse H, generating small RNA fragments during minus-strand DNA synthesis, causing competitive NCp7 dissociation from RTCs.Citation88,Citation89 Strands displaced during the course of plus-strand termination would be the last regions to retain NCp7, especially the central DNA flap,Citation71 while the HIV-1 LTR dsDNA ends should promote active IN assemblies.Citation90
Premature reverse transcription. A peculiar observation was made recently by several laboratories, where mutations to HIV-1 NC affected the timing of reverse transcription. Houzet and coworkers observed that when either or both zinc binding motifs were deleted from the NC in HIV-1, reverse transcription initiation and progression shifted from a point after introduction of the RNP complex into newly infected cells, to a step prior to virion budding. This resulted in virions with elevated levels of intravirion DNA, both from unspliced and multiply spliced viral RNAs.Citation91 The study of Thomas and coworkers examined the kinetics of reverse transcription in cells infected with either NC mutant or wild-type HIV-1. Reverse transcription proceeded as expected with wild-type virions, with the increases in viral DNA that were maximal at about 8 h postinfection, whereas maximal levels of reverse transcripts were observed in the NCH23C and NCH44C mutants at the beginning of the time course.Citation92 It was discovered in these particular mutants as well, that reverse transcription occurred before particles were released from cells.Citation12,Citation93 In both cases reverse transcriptase was required for the observed premature reverse transcription phenotype since RT inhibitor-treatment or active site RT mutations effectively reduced the levels of intravirion DNA. The mechanism of premature reverse transcription is not known at this time, although a similar phenotype was observed in HIV-1 with the p6, late domain mutation (PTAP to LIRL) that reduces virion budding.Citation93
Other aspects of NC.
NC binding with cellular proteins. NC has been shown to engage in interactions with different cellular proteins, in addition to the viral RT. Relationships have not been established between these interactions and effects on RNA binding/chaperone activity: it is still not clear whether NC can bind simultaneously to RNA and another protein, or if these interactions are more exclusive in the context of the nucleocapsid architecture. Three examples will be highlighted here, with the main idea being that several thousands of copies of NC are able to accommodate many interactions with only a short sequence of amino acids, emphasizing its architectural adaptability within virions.
One of the first cellular proteins shown to be a HIV-1 NC ligand was actin. It binds to both the NC domain in Gag and fully processed NCp7.Citation94,Citation95 Additionally the N-terminal domain of HIV-1 NC has been implicated in this binding.Citation96 More recently, interactions between actin and the NC domains of EIAV, MuLV or HIV-1 Gag have been also shown to positively impact viral budding.Citation97,Citation98 Nevertheless, more studies are expected to better identify these NC-actin interactions, their effects on NC binding to RNA and how they support viral replication.
Another protein that was shown to interact with NC was a cellular restriction factor, APOBEC3G that is incorporated into HIV-1 viral infectivity factor-negative (Vif(-)) virions and inhibits replication.Citation99 Its recruitment involves RNA and both the N-terminal and spacer domains of NC, again in the context of Gag precursor.Citation100,Citation101 Its antiviral action is due to its direct inhibition of reverse transcription plus its cytidine deaminase activity on the HIV-1 minus-strand DNA during reverse transcription.Citation102 Even though this enzyme is incorporated with a low copy number in the absence of Vif (less than 10 copies), one study showed significant inhibition of proper NCp7-chaperoned annealing of tRNALys3 on the PBS site,Citation103 whereas two other studies showed no effect on annealing;Citation82,Citation104 APOBEC3G strongly affected the elongation steps of reverse transcription due to the inability of RT or NCp7 to effectively displace it.Citation82,Citation104
Recently, HIV-1 NC was shown to interact with ALIX and, as a domain in Gag, is necessary for ALIX recruitment together with the Gag late domain, p6.Citation105 This cellular protein strongly enhances viral budding. With its boomerang-shaped Bro1 protein domain, it is involved in providing the correct membrane curvature required for microvesicular budding that is associated with the endosomal sorting complex required for transport (ESCRT). NC's ZFs have been shown to play a critical role in this binding interaction, whereas RNA appears dispensable. Moreover, NC can bind to a large class of proteins carrying Bro1 domains with an enhancement of virion particle release.Citation106
The implications of NC sequences interacting with cellular proteins highlighted here indicate that these interactions are both required or can be a detriment to viral replication.
NC-Vif trans-interactions. Besides NC, it has been reported that two other HIV-1 proteins, trans-activator of transcription (Tat) and Vif, have some nucleic acid chaperone activity in vitro.Citation107,Citation108 The implication of the activities of these auxiliary proteins in vivo is not readily clear, however both proteins have been shown to perform other critical functions, due in large part to protein-protein interactions with other cellular factors.Citation109,Citation110 Little information regarding Tat interactions with NC is available, however Vif may be involved in some indirect modulation of NC function, while mutations in NC may conversely impact these interactions.
Along with its effectiveness in excluding APOBEC3G, Vif has been show to stabilize the condensed nucleocapsid assembly within virionsCitation111 (this may also be a consequence of APOBEC3G exclusion) and to interact with Gag at the MA-CA, SP1-NC and NC-SP2 boundaries. In conjunction with these interactions, it has been proposed that Vif may downregulate viral PR processing at these Gag cleavage sites.Citation112–Citation115 In vitro studies have shown that Vif acts as a negative regulator of NC-assisted RNA dimerization and tRNAlys3 annealing.Citation107 In spite of this, Vif has also been shown to interact with RTCitation116 and to bind cooperatively with the HIV-1 5′-UTR RNA,Citation117 supporting efficient reverse transcription. However, the influence of Vif within viral particles has resulted in intense debate due to its limited amount in the virion:Citation107,Citation118 Vif binding to Gag, Pol and RNA should promote its incorporation, which may be useful for proper timing of both maturation and reverse transcription. Taking into account that high expression of Vif negatively affects HIV-1 replication,Citation115 cooperative Gag assembly followed by aggregation of mature NC with RNA may be a driving force for the exclusion of Vif from viral particles.
NC-p6 cis-interactions. HIV-1 NC binding to nucleic acids has been shown to be modulated by its C-terminal p6 domain within Gag.Citation17,Citation51,Citation71 Interestingly, HTLV-1 NC contains a region that is similar to the HIV-1 p6 domain that also modulates HTLV-1 NC interactions with nucleic acids as mentioned above.Citation42
This C-terminal domain, which is generally lacking in most other retroviruses, confers an optimal coordination for HIV-1 assembly and release with its late domain, containing the PTAP motif that facilitates interactions with the cellular MVB machinery.Citation119 The NC and p6 domains appear to cooperate in their interactions with Alix, NC being bound to Alix Bro1 domain and the p6 LYPxnL motif to Alix V-domain.Citation105,Citation106
The p6 domain is also necessary for efficient GagPol incorporationCitation120 and is required for Vpr packaging into virions,Citation121 incorporating both elements in close proximity to the NC domain that is bound to RNA. As NC interactions that have been described also include RTCitation76 and Vpr,Citation122 any impact of p6 on these interactions should be considered.
Cleavage of the SP2-p6 site results in p6 separation from NC and proteolytic cleavage is greatly enhanced when bound to RNA as mentioned above. This cleavage event leads to NC's efficient separation from within the capsid of the immature virion, whereupon NC and bound RNA undergo a vigorous condensation step to form the nucleocapsid found within the mature virion. More studies are needed to better understand the transient cis-actions between NC and p6 and its mediation by RNA.
Conclusion: Regulation of NC RNA Chaperone and the Spatiotemporal Control of Retroviral Plasticity
As presented in this review, a more complete view of NC behavior is actually emerging, in the context of HIV-1 replication. Becoming clearer is the critical role of the N-terminal domain and the two zinc fingers of this small protein in manipulating the viral RNA genome, from its capture to reverse transcription. As we mentioned above (section III.C.3.), a simple, but remarkable effect underlies this critical role: a simple substitution of the His by a Cys in either of the two conserved zinc knuckles provokes premature reverse transcription. On the other hand, the HIV-1 NC domain is remarkably conserved in its amino acid sequence, as confirmed by an analysis of all the HIV-1 sequences from the HIV sequence database (http://www.hiv.lanl.gov/content/sequence/HIV/mainpage.html): half of its residues (especially within the ZFs) are more than 99% conserved, demonstrating an exceptionally high genetic pressure to greatly maintain optimal NC function.
Another point that is developed here is modulation of the RNA chaperone activity of HIV-1 NC based on its processing state (Gag<NCp15<NCp9<NCp7). In HIV-1 and related lentiviruses, the C-terminal domain, p6, as well as two spacer peptides, SP1 and SP2 that flank the NC domain () are a hallmark of the Gag polyprotein. These retroviruses have developed a complex strategy for the control of NC function, resulting in the viral NC-bound RNA playing a pivotal role in the activation of the three step, C-terminal processing events that direct nucleocapsid condensation ( and ). Thus considering the state of NC processing provides a better understanding of the spatiotemporal regulation of intravirion architecture: optimal RNA chaperone function does not immediately commence after nucleocapsid separation within the capsid, but becomes so after p6 removal. In close relation to this change, NC's architectural properties, linked to RNA aggregation, are also greatly influenced by processing once NC is separated from CA-SP1 (i.e., separation of nucleocapsid from capsid): the first species, NCp15, is poorly aggregative; the second, NCp9, is highly aggregative; and the final, NCp7, is still aggregative but more mobile in its interaction with RNA (see the review of M. C. Williams and I. Rouzina in this issue). Rapid mobility has been proposed to be a favorable characteristic for NCp7 to assist in reverse transcription and the subsequent formation of PICs. Finally, we propose here that regulating both the RNA chaperone and architectural properties of the NC domain is a remarkable property of HIV-1 that confers an optimal architectural plasticity within the viral core, for its transfer from the producer to the target cell.
The HIV-1 NC domain and mature NCp7 have also been shown to engage in a diversified set of protein-protein interactions, either directly or mediated through the viral RNA. A better understanding of these interactions is now needed in order to determine their influence on the nucleic acid chaperone activity of HIV-1 NC and more generally in the plasticity of the viral architecture from states that allow escape from a producing cell to states that allow efficient introduction of the nucleoprotein complex into a newly infected cell. Such a scheme implies that NC must now be considered a nucleic acid architectural protein that directly coordinates the spatiotemporal progression of the HIV-1 genome from a messenger RNA to a double-stranded nuclear viral DNA poised for integration, with the support of two HIV-1 auxiliary proteins: Vif (escape steps) and Vpr (incoming steps). Such progression, finely tuned by PR, initiates with Gag assembly and the coordinated interactions of the NC and p6 domains in the binding of critical cellular proteins engaged in viral budding or ESCRT machinery. After budding and maturation, NC then packages a nucleocapsid complex associated with RT, Vpr and IN that is competent within a newly infected cell to mediate RTC, then PIC formation, with concomitant capsid uncoating and cytoskeleton-linked transport to the nucleus.
If we now take into account that the number of NC copies within one virion is close to 2000, it is tempting to speculate that division of labor among intravirion NC sub-populations should be a convenient model that can accommodate all of the NC properties described in this review. Thus a simple question can be formulated here: do all NC domains and mature NC proteins need to be bound to RNA within a virion? If so, is this binding exclusive? Development of future HIV-1 NC studies will hopefully clarify this point.
Finally, after examining NC properties, this first glimpse highlights that the general concept of the nucleocapsid is maintained in HIV-1 and other Orthoretroviruses. Nevertheless, significant differences are indeed found in structural features, processing from the Gag precursors and efficiencies of their RNA chaperone activities of NC's from different viruses. As presented in the , NC structural organization can be quite different, with a single ZF and two long flanking domains in the case of MuLV or two ZFs with an extended spacer in the case of RSV. For HTLV-1 NC, a unique acidic C-terminal domain is present that appears, at first glance to be similar to unprocessed HIV-1 NCp15. At the level of processing, most retroviruses contain neither the spacer peptides SP1, SP2 nor p6-like peptides. In any event, NC proteins from these different retroviruses have clearly adopted unique nucleocapsid-based strategies to manage their RNA genomes and to promote their progression from mRNA to dsDNA, when traversing from the producer cell to the newly infected cell. Taking into account that various sub-families of retrovirus are implicated in human cancers (e.g., HTLV-1 and MuLV-related XMRV), we might expect more extensive studies on these retroviruses to improve our knowledge of retroviral nucleocapsids, to better identify their unique biological properties that may contribute to the discovery of new anti-cancer pharmacological strategies.
Abbreviations
CA | = | capsid |
ds | = | double-stranded |
ESCRT | = | endosomal sorting complex required for transport |
HIV-1 | = | human immunodeficiency virus type 1 |
HTLV-1 | = | human T-cell leukemia virus type 1 |
IN | = | integrase |
MuLV | = | murine leukemia virus |
MA | = | matrix |
NC | = | nucleocapsid |
PFV | = | prototype foamy retrovirus |
PIC | = | preintegration complex |
PR | = | viral protease |
RNP | = | ribonucleoprotein |
RSV | = | Rous sarcoma virus |
RT | = | reverse transcriptase |
RTC | = | reverse transcription complex |
SIV | = | simian immunodeficiency virus |
ss | = | single-stranded |
Tat | = | trans-activator of transcription |
Vif | = | viral infectivity factor |
Vpr | = | viral protein R |
ZF | = | zinc finger or zinc knuckle |
Figures and Tables
Figure 1 NC's involvements in HIV-1 replicative cycle. (1) Gag proteins are first produced and migrate through the cytoplasm towards the vicinity of the cellular membrane. N-terminal MA domains of Gag bind to the lipid raft domains of the cellular membrane while the C-termini project into the cytoplasm and bind viral RNA through their NC domains. Gag binding initiates at the Ψ RNA region ensuring viral RNA dimerization, which then acts as a nucleation point to aggregate other Gag and Gag-Pol units though Gag-Gag interactions and NC/RNA binding (MA/RNA binding has also been proposedCitation62–Citation64). Viral RNA capture competes with the translational and/or mRNA docking apparati whereas other viral components are loaded onto Gag such as Vpr. (2) RNA scaffolding and Gag-Gag interactions mediate assembly of the particle which subsequently buds from the cell as an immature and imperfect particle. (3) Contacts between Gag-Pol precursors drive PR dimerization/autoactivation, which initiates virus maturation. (4) Sequential proteolytic events of Gag and Gag-Pol by PR and the self-assembly capabilities of the cleavage products (MA, CA, NC) reorganize the viral core, generating a mature particle. Maturation results in NC/RNA complex (i.e., the nucleocapsid) condensation at the center of the core where NC chaperone activity modulates RNA tertiary and quaternary structures. (5) After entry of the HIV-1 particle into the cytoplasm of a newly infected cell, RT catalyses viral DNA synthesis in the context of a ribonucleoproteic complex where NC proteins accelerate the strand transfers required for (−) and (+) strand synthesis. Viral dsDNA synthesis proceeds with a complete remodeling of the capsid and its contents, which are ultimately converted into a pre-integration complex, depleted of most of the NC initially bound to the viral RNA.
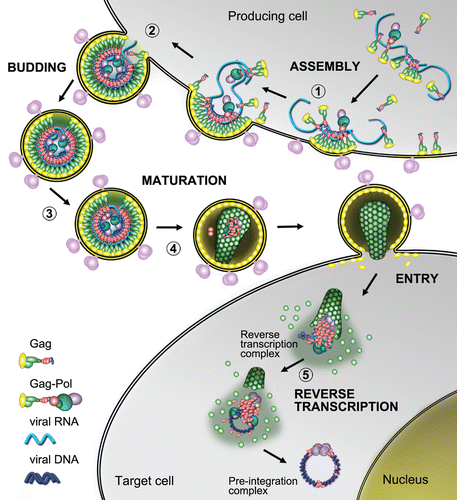
Figure 2 Structural features of HIV-1, RSV and MuLV nucleocapsid proteins—Ψ RNA complexes. The figure focuses on the binding of key guanosine residues (red), by a motif formed by a pocket derived from the side chains of hydrophobic residues (green). Zinc atoms bound by the ZFs are grey spheres. Location of basic amino-acids (Lys and Arg), available for electrostatic binding with RNA, are highlighted in yellow. (A) HIV-1 NC complexed with SL3 Ψ RNA (PDB ID:1A1T). A guanosine residue fits between the two adjacent ZFs which form a hydrophobic pocket around Phe16 and Trp37. The N-terminal basic tail adopts a 310 helix, which binds to the major groove of the SL3 stem.Citation16 (B) RSV NC and µΨ RNA structure (PDB ID:2IHX), where the guanosine base fits into a hydrophobic pocket defined by residues Tyr22, Tyr30, Leu20 and Gln31. Note that the RSV protein contains two ZF domains that adopt canonical folds observed for all other structurally characterized retroviral ZF, Tyr residues are used by RSV ZF1 instead of Trp and Phe residues used by HIV-1 NC. The C-terminal ZF is unusual as it does not contain aromatic residues nor a well-defined hydrophobic pocket. This finger packs against an adenine nucleobase though hydrogen bonds and salt bridges.Citation123 (C) Complex of MuLV NC and the mΨ RNA core encapsidation signal (PDB ID:1U6P) in which the single CCHC ZF of NC interacts with the UCUG sequence (indicated in pink, with G in red). The guanosine base fits deeply into a pocket defined by the side chain of Leu21, Ala27, Trp35 and Ala36. The first U and C pack against the side chain of Tyr28 and the second U packs against the side chains of Ala27 and Leu 21. The binding also appears to be promoted by several interactions between the basic residues and the phosphodiester backbone.Citation56
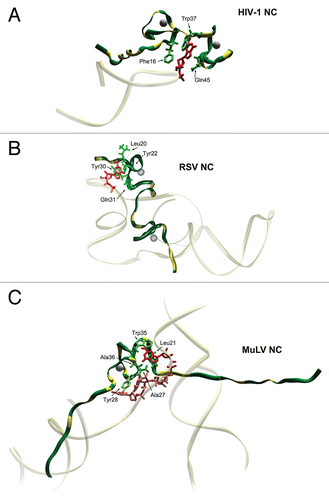
Figure 3 Proteolytic processing of HIV-1 and MuLV Gag by PR. The different protein species that exist during the steps of processing are indicated. (A) In HIV-1 Gag the initial cleavage occurs between SP1 and the NC domain, secondary cleavages occur at approximately 10-fold lower rates than the initial cut. The tertiary cleavages occur approximately 400-fold slower than the primary cleavage.Citation72 During proteolysis, HIV-1 NC exists in two intermediate forms, NCp15 (partial cleavage product containing NC/SP2/p6) and NCp9 (partial cleavage product containing NC/SP2) and the fully processed form, NCp7. All three of these proteins exhibit nucleic acid chaperone activities.Citation17 (B) Primary cleavage of MuLV Gag occurs between p12 and CA with slower secondary cleavages that yield the mature proteins MAp15, p12, CAp30 and NCp10.Citation124 (C) Extended structural model of an HIV-1 Gag polypeptide assembled from high resolution structures of isolated domains (PDB IDs:1UPH, 3GV2, 1A1T, 2C55 respectively for MA, CA, NCp7 and p6). Unavailable and/or unstructured domains are represented by dashed lines. PR cleavage site are indicated by the arrowheads. (D) Schematic models of viral core maturation. SP1-NC cleavage by PR rapidly separates the MA-CA shell and the nucleocapsid complex formed between RNA, NCp15, RT and IN. NCp15 processing by PR into NCp9 and finally NCp7 leads to NC/RNA coaggregation/condensation within the confines of the capsid cone.
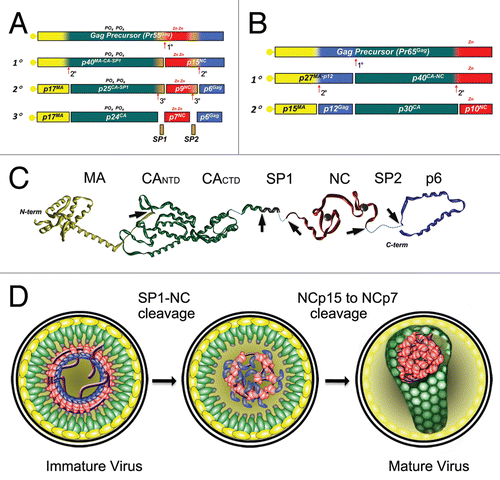
Acknowledgements
G.M. and S.L. are greatly indebted to Jose Maria Gatell and Laura Zamora for their kind hosting and support at the Infectious Disease and AIDS Unit. We wish to thank Sophie Brouillet, Joel Pothier (ABI, UPMC, Paris) and Anne Voinet (MTI-Université Paris Diderot, Paris) for the alignment of HIV-1 NC sequences. We also wish to thank James A. Thomas (NCI-Frederick) and Judith G. Levin (NICHD, NIH) for critical reading of this manuscript. S.L. is funded by a Marie Curie Intra-European Fellowship IEF N° 237738. (R.J.G.) this project has been funded in whole or in part with federal funds from the National Cancer Institute, National Institutes of Health, under contracts N01-CO-12400 and HHSN261200800001E. The content of this publication does not necessarily reflect the views or policies of the Department of Health and Human Services, nor does mention of trade names, commercial products or organizations imply endorsement by the US Government.
References
- Davis NL, Rueckert RR. Properties of a ribonucleoprotein particle isolated from Nonidet P-40-treated Rous sarcoma virus. J Virol 1972; 10:1010 - 1020
- Davis J, Scherer M, Tsai WP, Long C. Low-molecular-weight Rauscher leukemia virus protein with preferential binding for single-stranded RNA and DNA. J Virol 1976; 18:709 - 718
- Feng YX, Copeland TD, Henderson LE, Gorelick RJ, Bosche WJ, Levin JG, et al. HIV-1 nucleocapsid protein induces “maturation” of dimeric retroviral RNA in vitro. Proc Natl Acad Sci USA 1996; 93:7577 - 7581
- Gorelick RJ, Henderson LE, Hanser JP, Rein A. Point mutants of Moloney murine leukemia virus that fail to package viral RNA: evidence for specific RNA recognition by a “zinc finger-like” protein sequence. Proc Natl Acad Sci USA 1988; 85:8420 - 8424
- Meric C, Goff SP. Characterization of Moloney murine leukemia virus mutants with single-amino-acid substitutions in the Cys-His box of the nucleocapsid protein. J Virol 1989; 63:1558 - 1568
- Meric C, Gouilloud E, Spahr PF. Mutations in Rous sarcoma virus nucleocapsid protein p12 (NC): deletions of Cys-His boxes. J Virol 1988; 62:3328 - 3333
- Darlix JL, Gabus C, Nugeyre MT, Clavel F, Barre-Sinoussi F. Cis elements and trans-acting factors involved in the RNA dimerization of the human immunodeficiency virus HIV-1. J Mol Biol 1990; 216:689 - 699
- Prats AC, Sarih L, Gabus C, Litvak S, Keith G, Darlix JL. Small finger protein of avian and murine retroviruses has nucleic acid annealing activity and positions the replication primer tRNA onto genomic RNA. EMBO J 1988; 7:1777 - 1783
- Bampi C, Jacquenet S, Lener D, Decimo D, Darlix JL. The chaperoning and assistance roles of the HIV-1 nucleocapsid protein in proviral DNA synthesis and maintenance. Int J Biochem Cell Biol 2004; 36:1668 - 1686
- Levin JG, Guo J, Rouzina I, Musier-Forsyth K. Nucleic acid chaperone activity of HIV-1 nucleocapsid protein: Critical role in reverse transcription and molecular mechanism. Progress in Nucleic Acids Res and Mol Biol 2005; 80:217 - 286
- Rein A, Henderson LE, Levin JG. Nucleic-acid-chaperone activity of retroviral nucleocapsid proteins: significance for viral replication. Trends Biochem Sci 1998; 23:297 - 301
- Thomas JA, Gorelick RJ. Nucleocapsid protein function in early infection processes. Virus Res 2008; 134:39 - 63
- De Rocquigny H, Gabus C, Vincent A, Fournie-Zaluski MC, Roques B, Darlix JL. Viral RNA annealing activities of human immunodeficiency virus type 1 nucleocapsid protein require only peptide domains outside the zinc fingers. Proc Natl Acad Sci USA 1992; 89:6472 - 6476
- Prats AC, Housset V, de Billy G, Cornille F, Prats H, Roques B, et al. Viral RNA annealing activities of the nucleocapsid protein of Moloney murine leukemia virus are zinc independent. Nucleic Acids Res 1991; 19:3533 - 3541
- Amarasinghe GK, De Guzman RN, Turner RB, Chancellor KJ, Wu ZR, Summers MF. NMR structure of the HIV-1 nucleocapsid protein bound to stem-loop SL2 of the psi-RNA packaging signal. Implications for genome recognition. J Mol Biol 2000; 301:491 - 511
- De Guzman RN, Wu ZR, Stalling CC, Pappalardo L, Borer PN, Summers MF. Structure of the HIV-1 nucleocapsid protein bound to the SL3 psi-RNA recognition element. Science 1998; 279:384 - 388
- Cruceanu M, Urbaneja MA, Hixson CV, Johnson DG, Datta SA, Fivash MJ, et al. Nucleic acid binding and chaperone properties of HIV-1 Gag and nucleocapsid proteins. Nucleic Acids Res 2006; 34:593 - 605
- Le Cam E, Coulaud D, Delain E, Petitjean P, Roques BP, Gerard D, et al. Properties and growth mechanism of the ordered aggregation of a model RNA by the HIV-1 nucleocapsid protein: an electron microscopy investigation. Biopolymers 1998; 45:217 - 229
- Stewart-Maynard KM, Cruceanu M, Wang F, Vo MN, Gorelick RJ, Williams MC, et al. Retroviral nucleocapsid proteins display nonequivalent levels of nucleic acid chaperone activity. J Virol 2008; 82:10129 - 10142
- Stoylov SP, Vuilleumier C, Stoylova E, De Rocquigny H, Roques BP, Gerard D, et al. Ordered aggregation of ribonucleic acids by the human immunodeficiency virus type 1 nucleocapsid protein. Biopolymers 1997; 41:301 - 312
- Guo J, Wu T, Anderson J, Kane BF, Johnson DG, Gorelick RJ, et al. Zinc finger structures in the human immunodeficiency virus type 1 nucleocapsid protein facilitate efficient minus- and plus-strand transfer. J Virol 2000; 74:8980 - 8988
- Peliska JA, Balasubramanian S, Giedroc DP, Benkovic SJ. Recombinant HIV-1 nucleocapsid protein accelerates HIV-1 reverse transcriptase catalyzed DNA strand transfer reactions and modulates RNase H activity. Biochemistry 1994; 33:13817 - 13823
- You JC, McHenry CS. Human immunodeficiency virus nucleocapsid protein accelerates strand transfer of the terminally redundant sequences involved in reverse transcription. J Biol Chem 1994; 269:31491 - 1495
- Dannull J, Surovoy A, Jung G, Moelling K. Specific binding of HIV-1 nucleocapsid protein to PSI RNA in vitro requires N-terminal zinc finger and flanking basic amino acid residues. EMBO J 1994; 13:1525 - 1533
- Mori M, Dietrich U, Manetti F, Botta M. Molecular dynamics and DFT study on HIV-1 nucleocapsid protein-7 in complex with viral genome. J Chem Inf Model 2010; 50:638 - 650
- Fisher RJ, Fivash MJ, Stephen AG, Hagan NA, Shenoy SR, Medaglia MV, et al. Complex interactions of HIV-1 nucleocapsid protein with oligonucleotides. Nucleic Acids Res 2006; 34:472 - 484
- Demene H, Jullian N, Morellet N, de Rocquigny H, Cornille F, Maigret B, et al. Three-dimensional 1H NMR structure of the nucleocapsid protein NCp10 of Moloney murine leukemia virus. J Biomol NMR 1994; 4:153 - 170
- Morellet N, de Rocquigny H, Mely Y, Jullian N, Demene H, Ottmann M, et al. Conformational behaviour of the active and inactive forms of the nucleocapsid NCp7 of HIV-1 studied by 1H NMR. J Mol Biol 1994; 235:287 - 301
- Lee BM, De Guzman RN, Turner BG, Tjandra N, Summers MF. Dynamical behavior of the HIV-1 nucleocapsid protein. J Mol Biol 1998; 279:633 - 649
- Berg JM. Potential metal-binding domains in nucleic acid binding proteins. Science 1986; 232:485 - 487
- Covey SN. Amino acid sequence homology in gag region of reverse transcribing elements and the coat protein gene of cauliflower mosaic virus. Nucleic Acids Res 1986; 14:623 - 633
- Morellet N, Jullian N, De Rocquigny H, Maigret B, Darlix JL, Roques BP. Determination of the structure of the nucleocapsid protein NCp7 from the human immunodeficiency virus type 1 by 1H NMR. EMBO J 1992; 11:3059 - 3065
- Lam WC, Maki AH, Casas-Finet JR, Erickson JW, Kane BP, Sowder RC 2nd, et al. Phosphorescence and optically detected magnetic resonance investigation of the binding of the nucleocapsid protein of the human immunodeficiency virus type 1 and related peptides to RNA. Biochemistry 1994; 33:10693 - 10700
- Morellet N, Demene H, Teilleux V, Huynh-Dinh T, de Rocquigny H, Fournie-Zaluski MC, et al. Structure of the complex between the HIV-1 nucleocapsid protein NCp7 and the single-stranded pentanucleotide d(ACG CC). J Mol Biol 1998; 283:419 - 434
- Urbaneja MA, Kane BP, Johnson DG, Gorelick RJ, Henderson LE, Casas-Finet JR. Binding properties of the human immunodeficiency virus type 1 nucleocapsid protein p7 to a model RNA: elucidation of the structural determinants for function. J Mol Biol 1999; 287:59 - 75
- Kafaie J, Dolatshahi M, Ajamian L, Song R, Mouland AJ, Rouiller I, et al. Role of capsid sequence and immature nucleocapsid proteins p9 and p15 in Human Immunodeficiency Virus type 1 genomic RNA dimerization. Virology 2009; 385:233 - 244
- Kafaie J, Song R, Abrahamyan L, Mouland AJ, Laughrea M. Mapping of nucleocapsid residues important for HIV-1 genomic RNA dimerization and packaging. Virology 2008; 375:592 - 610
- Feng YX, Campbell S, Harvin D, Ehresmann B, Ehresmann C, Rein A. The human immunodeficiency virus type 1 Gag polyprotein has nucleic acid chaperone activity: possible role in dimerization of genomic RNA and placement of tRNA on the primer binding site. J Virol 1999; 73:4251 - 4256
- Feng YX, Fu W, Winter AJ, Levin JG, Rein A. Multiple regions of Harvey sarcoma virus RNA can dimerize in vitro. J Virol 1995; 69:2486 - 2490
- Darugar Q, Kim H, Gorelick RJ, Landes C. Human T-cell lymphotropic virus type 1 nucleocapsid protein-induced structural changes in transactivation response DNA hairpin measured by single-molecule fluorescence resonance energy transfer. J Virol 2008; 82:12164 - 12171
- Beltz H, Azoulay J, Bernacchi S, Clamme JP, Ficheux D, Roques B, et al. Impact of the terminal bulges of HIV-1 cTAR DNA on its stability and the destabilizing activity of the nucleocapsid protein NCp7. J Mol Biol 2003; 328:95 - 108
- Qualley DF, Stewart-Maynard KM, Wang F, Mitra M, Gorelick RJ, Rouzina I, et al. C-terminal domain modulates the nucleic acid chaperone activity of human T-cell leukemia virus type 1 nucleocapsid protein via an electrostatic mechanism. J Biol Chem 2010; 285:295 - 307
- Linial ML. Foamy viruses are unconventional retroviruses. J Virol 1999; 73:1747 - 1755
- Yu SF, Edelmann K, Strong RK, Moebes A, Rethwilm A, Linial ML. The carboxyl terminus of the human foamy virus Gag protein contains separable nucleic acid binding and nuclear transport domains. J Virol 1996; 70:8255 - 8262
- Stenbak CR, Linial ML. Role of the C terminus of foamy virus Gag in RNA packaging and Pol expression. J Virol 2004; 78:9423 - 9430
- Zamborlini A, Renault N, Saib A, Delelis O. Early reverse transcription is essential for productive foamy virus infection. PLoS One 2010; 5:11023
- Yu SF, Sullivan MD, Linial ML. Evidence that the human foamy virus genome is DNA. J Virol 1999; 73:1565 - 1572
- Lehmann-Che J, Giron ML, Delelis O, Lochelt M, Bittoun P, Tobaly-Tapiero J, et al. Protease-dependent uncoating of a complex retrovirus. J Virol 2005; 79:9244 - 9253
- Ganser-Pornillos BK, Yeager M, Sundquist WI. The structural biology of HIV assembly. Curr Opin Struct Biol 2008; 18:203 - 217
- Khan R, Giedroc DP. Nucleic acid binding properties of recombinant Zn2 HIV-1 nucleocapsid protein are modulated by COOH-terminal processing. J Biol Chem 1994; 269:22538 - 22546
- Mirambeau G, Lyonnais S, Coulaud D, Hameau L, Lafosse S, Jeusset J, et al. Transmission electron microscopy reveals an optimal HIV-1 nucleocapsid aggregation with single-stranded nucleic acids and the mature HIV-1 nucleocapsid protein. J Mol Biol 2006; 364:496 - 511
- Rajkowitsch L, Chen D, Stampfl S, Semrad K, Waldsich C, Mayer O, et al. RNA chaperones, RNA annealers and RNA helicases. RNA Biol 2007; 4:118 - 130
- Chen J, Nikolaitchik O, Singh J, Wright A, Bencsics CE, Coffin JM, et al. High efficiency of HIV-1 genomic RNA packaging and heterozygote formation revealed by single virion analysis. Proc Natl Acad Sci USA 2009; 106:13535 - 13540
- Fu W, Dang Q, Nagashima K, Freed EO, Pathak VK, Hu WS. Effects of Gag mutation and processing on retroviral dimeric RNA maturation. J Virol 2006; 80:1242 - 1249
- Hargittai MR, Gorelick RJ, Rouzina I, Musier-Forsyth K. Mechanistic insights into the kinetics of HIV-1 nucleocapsid protein-facilitated tRNA annealing to the primer binding site. J Mol Biol 2004; 337:951 - 968
- D'Souza V, Summers MF. Structural basis for packaging the dimeric genome of Moloney murine leukaemia virus. Nature 2004; 431:586 - 590
- Dey A, York D, Smalls-Mantey A, Summers MF. Composition and sequence-dependent binding of RNA to the nucleocapsid protein of Moloney murine leukemia virus. Biochemistry 2005; 44:3735 - 3744
- Gherghe C, Lombo T, Leonard CW, Datta SAK, Bess JW, Gorelick RJ, et al. Definition of a high-affinity Gag recognition structure mediating packaging of a retroviral RNA genome. Proc Natl Acad Sci USA 2010; 107:19248 - 19253
- Tanchou V, Gabus C, Rogemond V, Darlix JL. Formation of stable and functional HIV-1 nucleoprotein complexes in vitro. J Mol Biol 1995; 252:563 - 571
- Muriaux D, Mirro J, Harvin D, Rein A. RNA is a structural element in retrovirus particles. Proc Natl Acad Sci USA 2001; 98:5246 - 5251
- Anderson EC, Lever AM. Human immunodeficiency virus type 1 Gag polyprotein modulates its own translation. J Virol 2006; 80:10478 - 10486
- Ott DE, Coren LV, Gagliardi TD. Redundant roles for nucleocapsid and matrix RNA-binding sequences in human immunodeficiency virus type 1 assembly. J Virol 2005; 79:13839 - 13847
- Burniston MT, Cimarelli A, Colgan J, Curtis SP, Luban J. Human immunodeficiency virus type 1 Gag polyprotein multimerization requires the nucleocapsid domain and RNA and is promoted by the capsid-dimer interface and the basic region of matrix protein. J Virol 1999; 73:8527 - 8540
- Purohit P, Dupont S, Stevenson M, Green MR. Sequence-specific interaction between HIV-1 matrix protein and viral genomic RNA revealed by in vitro genetic selection. RNA 2001; 7:576 - 584
- Wang H, Norris KM, Mansky LM. Involvement of the matrix and nucleocapsid domains of the bovine leukemia virus Gag polyprotein precursor in viral RNA packaging. J Virol 2003; 77:9431 - 9438
- Lee EG, Linial ML. Basic residues of the retroviral nucleocapsid play different roles in Gag-Gag and Gag-Psi RNA interactions. J Virol 2004; 78:8486 - 8495
- Wu T, Datta SA, Mitra M, Gorelick RJ, Rein A, Levin JG. Fundamental differences between the nucleic acid chaperone activities of HIV-1 nucleocapsid protein and Gag or Gag-derived proteins: Biological implications. Virology 2010; 405:556 - 567
- Guo J, Wu T, Kane BF, Johnson DG, Henderson LE, Gorelick RJ, et al. Subtle alterations of the native zinc finger structures have dramatic effects on the nucleic acid chaperone activity of human immunodeficiency virus type 1 nucleocapsid protein. J Virol 2002; 76:4370 - 4378
- Briggs JA, Wilk T, Welker R, Krausslich HG, Fuller SD. Structural organization of authentic, mature HIV-1 virions and cores. EMBO J 2003; 22:1707 - 1715
- Cruceanu M, Gorelick RJ, Musier-Forsyth K, Rouzina I, Williams MC. Rapid kinetics of protein-nucleic acid interaction is a major component of HIV-1 nucleocapsid protein's nucleic acid chaperone function. J Mol Biol 2006; 363:867 - 877
- Mirambeau G, Lyonnais S, Coulaud D, Hameau L, Lafosse S, Jeusset J, et al. HIV-1 protease and reverse transcriptase control the architecture of their nucleocapsid partner. PLoS One 2007; 2:669
- Pettit SC, Sheng N, Tritch R, Erickson-Viitanen S, Swanstrom R. The regulation of sequential processing of HIV-1 Gag by the viral protease. Adv Exp Med Biol 1998; 436:15 - 25
- Sheng N, Pettit SC, Tritch RJ, Ozturk DH, Rayner MM, Swanstrom R, et al. Determinants of the human immunodeficiency virus type 1 p15NC-RNA interaction that affect enhanced cleavage by the viral protease. J Virol 1997; 71:5723 - 5732
- Fisher RJ, Rein A, Fivash M, Urbaneja MA, Casas-Finet JR, Medaglia M, et al. Sequence-specific binding of human immunodeficiency virus type 1 nucleocapsid protein to short oligonucleotides. J Virol 1998; 72:1902 - 1909
- Williams MC, Rouzina I, Wenner JR, Gorelick RJ, Musier-Forsyth K, Bloomfield VA. Mechanism for nucleic acid chaperone activity of HIV-1 nucleocapsid protein revealed by single molecule stretching. Proc Natl Acad Sci USA 2001; 98:6121 - 6126
- Grohmann D, Godet J, Mely Y, Darlix JL, Restle T. HIV-1 nucleocapsid traps reverse transcriptase on nucleic acid substrates. Biochemistry 2008; 47:12230 - 12240
- Lener D, Tanchou V, Roques BP, Le Grice SF, Darlix JL. Involvement of HIV-I nucleocapsid protein in the recruitment of reverse transcriptase into nucleoprotein complexes formed in vitro. J Biol Chem 1998; 273:33781 - 33786
- Coren LV, Thomas JA, Chertova E, Sowder RC 2nd, Gagliardi TD, Gorelick RJ, et al. Mutational analysis of the C-terminal Gag cleavage sites in human immunodeficiency virus type 1. J Virol 2007; 81:10047 - 10054
- Kolli M, Stawiski E, Chappey C, Schiffer CA. Human immunodeficiency virus type 1 protease-correlated cleavage site mutations enhance inhibitor resistance. J Virol 2009; 83:11027 - 11042
- Hsu M, Wainberg MA. Interactions between human immunodeficiency virus type 1 reverse transcriptase, tRNA primer and nucleocapsid protein during reverse transcription. J Hum Virol 2000; 3:16 - 26
- Muller B, Anders M, Akiyama H, Welsch S, Glass B, Nikovics K, et al. HIV-1 Gag processing intermediates trans-dominantly interfere with HIV-1 infectivity. J Biol Chem 2009; 284:29692 - 29703
- Iwatani Y, Chan DS, Wang F, Maynard KS, Sugiura W, Gronenborn AM, et al. Deaminase-independent inhibition of HIV-1 reverse transcription by APOBEC3G. Nucleic Acids Res 2007; 35:7096 - 7108
- Fassati A, Goff SP. Characterization of intracellular reverse transcription complexes of human immunodeficiency virus type 1. J Virol 2001; 75:3626 - 3635
- Nermut MV, Fassati A. Structural analyses of purified human immunodeficiency virus type 1 intracellular reverse transcription complexes. J Virol 2003; 77:8196 - 8206
- Iordanskiy S, Berro R, Altieri M, Kashanchi F, Bukrinsky M. Intracytoplasmic maturation of the human immunodeficiency virus type 1 reverse transcription complexes determines their capacity to integrate into chromatin. Retrovirology 2006; 3:4
- Dismuke DJ, Aiken C. Evidence for a functional link between uncoating of the human immunodeficiency virus type 1 core and nuclear import of the viral preintegration complex. J Virol 2006; 80:3712 - 3720
- Anthony RM, Destefano JJ. In vitro synthesis of long DNA products in reactions with HIV-RT and nucleocapsid protein. J Mol Biol 2007; 365:310 - 324
- Wisniewski M, Balakrishnan M, Palaniappan C, Fay PJ, Bambara RA. Unique progressive cleavage mechanism of HIV reverse transcriptase RNase H. Proc Natl Acad Sci USA 2000; 97:11978 - 11983
- Schultz SJ, Zhang M, Champoux JJ. Sequence, distance and accessibility are determinants of 5′-end-directed cleavages by retroviral RNases H. J Biol Chem 2006; 281:1943 - 1955
- Kotova S, Li M, Dimitriadis EK, Craigie R. Nucleoprotein intermediates in HIV-1 DNA integration visualized by atomic force microscopy. J Mol Biol 2010; 399:491 - 500
- Houzet L, Morichaud Z, Didierlaurent L, Muriaux D, Darlix JL, Mougel M. Nucleocapsid mutations turn HIV-1 into a DNA-containing virus. Nucl Acids Res 2008; 36:2311 - 2319
- Thomas JA, Gagliardi TD, Alvord WG, Lubomirski M, Bosche WJ, Gorelick RJ. Human immunodeficiency virus type 1 nucleocapsid zinc-finger mutations cause defects in reverse transcription and integration. Virology 2006; 353:41 - 51
- Thomas JA, Bosche WJ, Shatzer TL, Johnson DG, Gorelick RJ. Mutations in human immunodeficiency virus type 1 nucleocapsid protein zinc fingers cause premature reverse transcription. J Virol 2008; 82:9318 - 9328
- Wilk T, Gowen B, Fuller SD. Actin associates with the nucleocapsid domain of the human immunodeficiency virus Gag polyprotein. J Virol 1999; 73:1931 - 1940
- Liu B, Dai R, Tian CJ, Dawson L, Gorelick R, Yu XF. Interaction of the human immunodeficiency virus type 1 nucleocapsid with actin. J Virol 1999; 73:2901 - 2908
- Krogstad P, Geng YZ, Rey O, Canon J, Ibarrondo FJ, Ackerson B, et al. Human immunodeficiency virus nucleocapsid protein polymorphisms modulate the infectivity of RNA packaging mutants. Virology 2002; 294:282 - 288
- Gladnikoff M, Shimoni E, Gov NS, Rousso I. Retroviral assembly and budding occur through an actin-driven mechanism. Biophys J 2009; 97:2419 - 2428
- Chen C, Jin J, Rubin M, Huang L, Sturgeon T, Weixel KM, et al. Association of Gag multimers with filamentous actin during equine infectious anemia virus assembly. Curr HIV Res 2007; 5:315 - 323
- Zennou V, Perez-Caballero D, Gottlinger H, Bieniasz PD. APOBEC3G incorporation into human immunodeficiency virus type 1 particles. J Virol 2004; 78:12058 - 12061
- Luo K, Liu B, Xiao Z, Yu Y, Yu X, Gorelick R, et al. Amino-terminal region of the human immunodeficiency virus type 1 nucleocapsid is required for human APOBEC3G packaging. J Virol 2004; 78:11841 - 11852
- Burnett A, Spearman P. APOBEC3G multimers are recruited to the plasma membrane for packaging into human immunodeficiency virus type 1 virus-like particles in an RNA-dependent process requiring the NC basic linker. J Virol 2007; 81:5000 - 5013
- Huthoff H, Towers GJ. Restriction of retroviral replication by APOBEC3G/F and TRIM5alpha. Trends Microbiol 2008; 16:612 - 619
- Guo F, Cen S, Niu M, Yang Y, Gorelick RJ, Kleiman L. The interaction of APOBEC3G with human immunodeficiency virus type 1 nucleocapsid inhibits tRNALys3 annealing to viral RNA. J Virol 2007; 81:11322 - 11331
- Bishop KN, Verma M, Kim EY, Wolinsky SM, Malim MH. APOBEC3G Inhibits Elongation of HIV-1 Reverse Transcripts. PLoS Pathogens 2008; 4:1000231
- Popov S, Popova E, Inoue M, Gottlinger HG. Human immunodeficiency virus type 1 Gag engages the Bro1 domain of ALIX/AIP1 through the nucleocapsid. J Virol 2008; 82:1389 - 1398
- Popov S, Popova E, Inoue M, Gottlinger HG. Divergent Bro1 domains share the capacity to bind human immunodeficiency virus type 1 nucleocapsid and to enhance virus-like particle production. J Virol 2009; 83:7185 - 7193
- Henriet S, Sinck L, Bec G, Gorelick RJ, Marquet R, Paillart JC. Vif is a RNA chaperone that could temporally regulate RNA dimerization and the early steps of HIV-1 reverse transcription. Nucleic Acids Res 2007; 35:5141 - 5153
- Boudier C, Storchak R, Sharma KK, Didier P, Follenius-Wund A, Muller S, et al. The mechanism of HIV-1 Tat-directed nucleic acid annealing supports its role in reverse transcription. J Mol Biol 2010; 400:487 - 501
- Romani B, Engelbrecht S, Glashoff RH. Functions of Tat: the versatile protein of human immunodeficiency virus type 1. J Gen Virol 2010; 91:1 - 12
- Malim MH, Emerman M. HIV-1 accessory proteins—ensuring viral survival in a hostile environment. Cell Host Microbe 2008; 3:388 - 398
- Zhang H, Pomerantz RJ, Dornadula G, Sun Y. Human immunodeficiency virus type 1 Vif protein is an integral component of an mRNP complex of viral RNA and could be involved in the viral RNA folding and packaging process. J Virol 2000; 74:8252 - 8261
- Bardy M, Gay B, Pebernard S, Chazal N, Courcoul M, Vigne R, et al. Interaction of human immunodeficiency virus type 1 Vif with Gag and Gag-Pol precursors: co-encapsidation and interference with viral protease-mediated Gag processing. J Gen Virol 2001; 82:2719 - 2733
- Huvent I, Hong SS, Fournier C, Gay B, Tournier J, Carriere C, et al. Interaction and co-encapsidation of human immunodeficiency virus type 1 Gag and Vif recombinant proteins. J Gen Virol 1998; 79:1069 - 1081
- Syed F, McCrae MA. Interactions in vivo between the Vif protein of HIV-1 and the precursor (Pr55(GAG)) of the virion nucleocapsid proteins. Arch Virol 2009; 154:1797 - 1805
- Akari H, Fujita M, Kao S, Khan MA, Shehu-Xhilaga M, Adachi A, et al. High level expression of human immunodeficiency virus type-1 Vif inhibits viral infectivity by modulating proteolytic processing of the Gag precursor at the p2/nucleocapsid processing site. J Biol Chem 2004; 279:12355 - 12362
- Kataropoulou A, Bovolenta C, Belfiore A, Trabatti S, Garbelli A, Porcellini S, et al. Mutational analysis of the HIV-1 auxiliary protein Vif identifies independent domains important for the physical and functional interaction with HIV-1 reverse transcriptase. Nucleic Acids Res 2009; 37:3660 - 3669
- Henriet S, Richer D, Bernacchi S, Decroly E, Vigne R, Ehresmann B, et al. Cooperative and specific binding of Vif to the 5′ region of HIV-1 genomic RNA. J Mol Biol 2005; 354:55 - 72
- Sova P, Volsky DJ, Wang L, Chao W. Vif is largely absent from human immunodeficiency virus type 1 mature virions and associates mainly with viral particles containing unprocessed Gag. J Virol 2001; 75:5504 - 5517
- Mazze FM, Degreve L. The role of viral and cellular proteins in the budding of human immunodeficiency virus. Acta Virol 2006; 50:75 - 85
- Yu XF, Dawson L, Tian CJ, Flexner C, Dettenhofer M. Mutations of the human immunodeficiency virus type 1 p6Gag domain result in reduced retention of Pol proteins during virus assembly. J Virol 1998; 72:3412 - 3417
- Fritz JV, Dujardin D, Godet J, Didier P, De Mey J, Darlix JL, et al. HIV-1 Vpr oligomerization but not that of Gag directs the interaction between Vpr and Gag. J Virol 2010; 84:1585 - 1596
- de Rocquigny H, Petitjean P, Tanchou V, Decimo D, Drouot L, Delaunay T, et al. The zinc fingers of HIV nucleocapsid protein NCp7 direct interactions with the viral regulatory protein Vpr. J Biol Chem 1997; 272:30753 - 30759
- Zhou J, Bean RL, Vogt VM, Summers M. Solution structure of the Rous sarcoma virus nucleocapsid protein: muPsi RNA packaging signal complex. J Mol Biol 2007; 365:453 - 467
- Yoshinaka Y, Luftig RB. Cleavage of Rauscher leukaemia virus (R-MuLV) Pr65Gag by the Moloney leukaemia virus (M-MuLV) proteolytic activity produces the R-MuLV-specific but not the M-MuLV-specific 40,000 dalton intermediate polypeptide. J Gen Virol 1981; 54:33 - 38