Abstract
The acrosome is a unique organelle that plays an important role at fertilization and during sperm morphogenesis and that is absent in globozoospermia, an inherited infertility syndrome in humans. At the light of recent experimental evidence, the acrosome is considered a lysosome-related organelle to whose biogenesis both the endocytic and biosynthetic pathways contribute. Vps54 is a vesicular sorting protein involved in the retrograde traffic; the recessive Vps54(L967Q) mutation in the mouse results in the wobbler phenotype, characterized by motor-neuron degeneration and male infertility. Here we have investigated the spatio-temporal occurrence/progression of the wobbler fertility disorder starting from mice at post-natal day 35, the day of the first event of spermiation. We show that the pathogenesis of wobbler infertility originates at the first spermiogenetic wave, affecting acrosome formation and sperm head elongation. Vps54(L967Q)-labeled vesicles, on the contrary of the wild type Vps54-labeled ones, are not able to coalesce into a larger vesicle that develops, flattens and shapes to give rise to the acrosome. Evidence that it is the malfunctioning of the endocytic traffic to hamper the development of the acrosome comes out from the study on UBPy. UBPy, a deubiquitinating enzyme, is a marker of acrosome biogenesis from the endocytic pathway. In wobbler spermatids UBPy-positive endosomes remain single, scattered vesicles that do not contribute to acrosome formation. As secondary defect of wobbler spermiogenesis, spermatid mitochondria are misorted; moreover, with the progression of the age/disease also Sertoli-germ cell adhesions are compromised suggesting a derailment in the endocytic route that underlies their restructuring.
Introduction
The wobbler mouse (wr), a spontaneous recessive mutant, was discovered by FalconerCitation1 as a mouse model of motor neuron disease. The wr phenotype is due to a homozygous missense mutation (L967Q) affecting the Vps54 gene that is located on chromosome 11.Citation2 Albeit widely employed to study spinal cord pathologies,Citation3–Citation5 the wr mouse is characterized by another disorder, namely male infertility, as noticed already in the first descriptions of the mutant.Citation6 This second aspect, however, has never been analyzed in detail even if defects in assembly of the sperm acrosomeCitation7,Citation8 and in sperm tail motilityCitation9 have been reported. The malformations of wobbler spermatozoa are apparently reminiscent of a human infertility syndrome known as globozoospermia (also called round-headed sperm syndrome). Globozoospermia is a rare but severe disorder in male infertility;Citation10 nowadays its specific etiology still remains an unsolved question, but a genetic component appears certain as some human family cases of globozoospermia ndicate.Citation11–Citation13 Knockout studies in mice have now identified many genes that produce an infertile male phenotype when disrupted. In particular, three genes, namely, CSNK2A2,Citation14 HRBCitation15 and GOPCCitation16 appear to reserve interest; the phenotypes of their respective null mutant mice are similar to human globozoospermia. However, no causative gene mutations have been identified in the orthologue human genes to date.Citation13
Vps54 codifies a vesicular protein for sorting, namely Vps54, which is involved as a component of the Golgi Associated Retrograde Protein (GARP) complex in the tethering of vesicles from endosomes to the Trans Golgi Network (TGN).Citation17,Citation18Vps54 knockout results in embryonic lethality, whereas wr mice are viable.Citation2 An A→T transversion at nucleotide 72 of exon 23 in the wr mouse changes evolutionarily (from human to yeast) conserved Leu967 to glutamine.Citation2 The different phenotypes of Vps54 disruption (lethal) and the wr mutant (viable) have been potentially explained by arguing that Vps54(L967Q) maintains the ability to assemble with the other GARP subunits thus allowing protein sorting.Citation19 Anyway, Vps54(L967Q) mutation compromises severely the functionality of both motor-neurons and spermatozoa.
The most prominent feature of globozoospermia is the lack of a true acrosome and, in the most severe cases, its complete absence. Hereupon, the name of the syndrome; the deficient development of the acrosome leads in fact to production of round-headed sperm.Citation20 The acrosome is considered to be indispensable at fertilization; consequently, its biogenesis is a crucial event for sperm fertilizing potential. This acidic vacuole, unique to male germ cells, has been described as a Golgi derivative,Citation21–Citation23 but recent experimental evidence indicates that there are at least two sources of vesicular transport to the forming acrosome, one derived from the biosynthetic pathway and one from the endocytic pathway.Citation24–Citation28 In particular, the deubiquitinating enzyme UBPy, now officially termed USP8, that functions in cultured cells as a key regulator of endosomal sorting,Citation29–Citation31 has been shown to be engaged in acrosome biogenesis;Citation28,Citation32 here UBPy, characterized by a MIT domain at its N-terminus, could promote both the sorting of selected cargo proteins and the microtubule-mediated endosomal vesicle trafficking to the acrosome.Citation28 Noteworthy, in the acrosomogenetic context also Vps54 has been found to label vesicular structures that evolve to form the acrosome.Citation28 To date, however, the Vps54(L967Q) variant has not yet been investigated in wr mice. It might be that Vps54(L967Q), although apparently active in trafficking between endosomes and the TGN when overexpressed in cultured transfected cells,Citation19 is not able to work correctly in the cells that are specifically affected by the wr recessive mutation, as indeed the lack of a real acrosome in wr spermatozoa implies.
In the present work, we have carried out a detailed histological analysis of wr spermatogenesis in line with the temporal progression of the pathology and have investigated about UBPy and Vps54(L967Q) during wr spermiogenesis, the cytodifferentiative phase when acrosomogenesis takes place.
Results
The chronology of progression of the wr neurological disease is subdivided into three successive phases, i.e., the presymptomatic (at 3rd–4th week of age), evolutionary (till the 12th week of age) and stabilization (3–5 months of age),Citation3 although slight differences, according to the wr strain examined, have been reported.Citation33 Motoneuronal death, however, is not a rapid, sudden event, but extends over time.Citation3 On its own, the completion of the first spermatogenic wave in the mouse requires approximately 1 month while the first event of spermiation, i.e., the release of spermatozoa from the seminiferous epithelium into the tubule lumen, occurs at day 35 post-partum.Citation34 It is so possible to establish whether male fertility disorder signs the wr phenotype even at the first round of spermatogenesis or successively during the progression of the evolutionary phase of the disease. With this purpose, we selected 3 time points, namely days 35 (first spermiation/early evolutionary phase), 44 (second spermatogenic wave/progressive evolutionary phase) and 77 (a going spermatogenesis/late evolutionary phase) post-partum, to carry on our analysis.
Wobbler mice produce aberrant sperm already at the first spermatogenic wave.
As first parameter, we considered the weight/size of wr versus wild type male gonads, when mice were matched for age. A typical hallmark of the wr mutant is that from the 4th week of age both females and males are smaller in size than their control littermates and they remain smaller thereafter.Citation3 The average testicular weight in wr mice was found to be significantly lower than that in wild-type mice already starting at day 35 of age (). Moreover, epididymal sperm counts revealed that 35 days-old wr males produced remarkably fewer spermatozoa than wild-type mice (). The differences became still more remarkable progressively with the age (44 and 77 days-old mice).
The morphological analysis at the light microscope evidenced the aberrant phenotype of wr epididymal spermatozoa. Spermatozoa released at the first event of spermiation (n = 3 mice) displayed a globic head and an abnormal flagellar morphology (). Defective heads were essentially due to the lack of an acrosome; since this defect affects also the acquisition of the nuclear shaping,Citation35 wr sperm showed a round nucleus with apparently ill-condensed chromatin. Moreover, some spermatozoa were characterized by a globoid head, i.e., a large, vacuolated, round, acrosome-less head containing remnants of not discharged cytoplasm (). As to the flagellum, the most prominent and common anomaly was that tail mid-piece lacks the canonical mitochondrial sheath (); this last, when not at all absent, was found to be mislocalized ( and E) and/or engulfed in the head cytoplasm (). Live wr spermatozoa, examined by visual inspection with a light microscope, showed severely reduced tail motility.
Wobbler fertility disorder originates in spermiogenesis.
Histological analysis of 35 days-old testis sections showed that the distribution and number of mitotic (spermatogonia) and meiotic (spermatocytes) germ cells appeared to be similar in wr and wild-type seminiferous tubules and no obvious abnormality was observed in wr Sertoli cells (Sup. Fig. S1, A and B). The transformation of wr round spermatids into testicular spermatozoa (spermiogenesis) was, however, seriously compromised ( and B, and Sup. Fig. S1, B and C). Spermiogenesis, which collectively includes 16 steps,Citation36 implies extensive cell morphological changes like chromatin condensation and the formation of the acrosome, tail and residual body. Typically, 35 days-old mutant tubules were devoid of elongated spermatids as well as their lumen in the coincidence of the first event of spermiation was devoid of terminally differentiated spermatozoa (Sup. Fig. S1 B). Apparently, round spermatids were the only haploid cells present in the seminiferous epithelium because no germ cells exhibited sperm head elongation and sickle-like chromatin modeling (). All the 35-day-old testis sections examined showed differentiating spermatids with abnormal shape and/or orientation and a round nucleus ( and B); moreover, failure in removal of the anomalously vacuolated cytoplasm as residual body at spermiation was also often observed (). So, the pathogenesis of wr male infertility originates already at the first spermiogenetic wave; it is not due, however, to a spermiogenetic block or delay. Cells proximal to the lumen and/or cells released into the lumen at day 35 of age exhibited in fact a flagellum () as, on the other hand, the morphological analysis of epididymal spermatozoa revealed. Rather, wr male infertility results to be imputable to a general derailment in accomplishing crucial spermiogenetic events so that aberrant spermatozoa are produced. This phenotypical analysis was further strengthened by testis histology during progression of the evolutionary phase of the disease.
44 days-old wr seminiferous tubules exhibited a morphological pattern consistent with that described for 35 day-old mice ( and E, and Sup. Fig. S1, E and F). Accordingly the wr spermiogenetic defect, traditional stages (from I to XII in the mouse) of the epithelial cycle could not be easily recognizable. Instead of the precise positioning and orientation of spermatids that constitutes each stage along the seminiferous tubule in 44 day-old cross sections of control littermates (), putative elongating spermatids stayed together with round spermatids in the same region of wr seminiferous epithelium (). An hallmark of the three 44 days-old wr mice we examined was the clumping of round-shaped spermatids next to be released () and the fusion of aggregates of residual bodies containing clumped spermatids (Sup. Fig. S1 E).
Also in 77 days-old wr seminiferous tubules, differentiating spermatids failed to proceed correctly into further maturation steps ( and H and Sup. Fig. S1, H and I). However, here spermatogenesis was more seriously compromised if compared with 35- and 44-day wr testis (). Grossly, diameter of wr seminiferous tubules in cross sections, already reduced at 35 and 44 days of age, decreased still more significantly when compared with wild type littermates (). Whereas control seminiferous tubules showed normal spermatogenesis with single steps of round and elongated spermatids supported by Sertoli cells in an evenly spaced and orderly fashion ( and Sup. Fig. S1 G), in 77 day-old wr testis cell-cell contacts were heavily perturbed ( and H, and Sup. Fig. S1 H). In particular, Sertoli-germ cell junctions at the basal compartment, where mitotic and meiotic germ cells reside, were seriously compromised (). These cell contacts are essentially constituted by anchoring junctions formed by adherens junctions and desmosome-like junctions.Citation37 illustrates an electron micrograph showing the seminiferous epithelium as constituted by Sertoli cells and germ cells located on the tunica propria in 77 days-old wild type testis. and the boxed area that is enlarged in show a functional junction structure between a preleptotene spermatocyte and a Sertoli cell in 77 days-old wild type testis. The most striking difference between wild-type and wr 77 days-old testis at the basal compartment was the presence of multiple extensive gaps between Sertoli cells and germ cells in the mutant mouse (, asterisks). So, apparently, wr Sertoli cells are hampered to exert their essential role for both the survival and the proper maturation of germ cells. Consistently with the morphological observations, there was the estimation of the average number of primary spermatocytes present for cross-section. By examination of 20 cross sections, selected at random, of 77 days-old seminiferous epithelium a considerable reduction in the number of spermatocytes was found in the wr versus wild type testis (wr = 48.8 ± 6.2 versus wt = 85.6 ± 7.7).
The histological evaluation of the mutant epididymes at each selected time points confirmed that: (i) wr 35, 44 and 77 days-old epididymes lacked sperm and/or contained debris and few aberrant sperm in their lumen (Sup. Fig. S2, C–H); (ii) the cells primarily affected by the wobbler mutation are the germ cells since somatic cells of the columnar epithelial layer showed normal morphology with stereocilia that extend into the lumen (Sup. Fig. S2, C–F). In 77 day-old epididymes, however, some of these cells appeared altered and exhibited an anomalously vacuolated cytoplasm (Sup. Fig. S2, G and H).
Failure in the acrosome biogenesis.
Acrosome biogenesis is a spermiogenetic event important for sperm biology not only during gamete interaction at fertilization, but also for sperm morphogenesis.Citation35 PAS staining is commonly used to visualize the changes of the developing acrosome during acrosomogenesis from round spermatids to elongating spermatids.Citation38 We thus used PAS staining to follow acrosome biogenesis in wr versus wild type mice. The progression of acrosomogenesis in the control littermates was easily detected: the few PAS-positive proacrosomal granules present in spermatids at steps 2–3 () coalesce into a single granule (steps 3–4) that then touches the nuclear surface and here flattens (spermatids at step 5) (). The proacrosomal vesicle continues to spread out over the nucleus through stages VI–VII until it covers more than a third of the nuclear surface (). In most of round spermatids at step 8 the PAS-positive acrosomal cap faces the basal membrane of the seminiferous tubule (). During the acrosomal phase (steps 9–12) the acrosome and the nucleus elongate ( and F), while chromatin begins to compact and hook-shaped nuclei of elongating spermatids at steps 12 become more darkly stained by hematoxylin ().
On the contrary, no evident acrosomal structure was detected in wr PAS-stained testes. In particular, already at the first spermatogenic wave round spermatids at steps 7–8 showed no well defined acrosomal cap but only PAS-positive granules scattered into the cytoplasm ( and H; compare with the respective wild type images, and E). PAS-positive granules sign also the acrosomal phase (). Consequently, an acrosomal structure was never detected at all analyzed stages. Owing to the spermiogenetic disorder, particularly hard in 77 days-old testis, in 44 and 77 days-old testes it was not possible to determine exactly the spermiogenetic step. Generally speaking, PAS-staining resulted only in a punctuate pink appearance diffuse in the cytoplasm (results not shown). Taken together, mutant spermatids do not develop an acrosome being PAS positive granules/proacrosomal vesicles prevented from coalescing into one single, larger vesicle that growths and shapes to give rise to the acrosome.
UBPy e Vps54(L967Q) in wobbler testis.
Recently it is emerged that the acrosome can be thought as a novel lysosome-related organelle to the biogenesis of which both the endocytic pathway and the biosynthetic pathway concur.Citation28 This interpretation sounds to be particularly hit at the light of the present evidence that wr spermatids, which express a mutated form of the vesicular endocytic sorting protein Vps54, are unable to form the acrosome. Being UBPy, the deubiquitinating enzyme that works as key endosomal regulator of protein sorting, a marker of acrosome biogenesis,Citation28,Citation32 we have carried out a study on UBPy in wr testis by immunofluorescence analysis. Previously, by immunohistochemistry UBPy has been shown to exhibit a diffuse, not polarized signal in wr round spermatids.Citation39 Here we have compared wr versus wild-type spermatids at different differentiation steps and matching the mice for age. summarizes the results. In seminiferous tubules at stage VII–VIII no UBPy-positive acrosomal structure was visualized in wr testis ( and B) whereas, on the contrary, control littermates were characterized by the brightly fluorescent UBPy immunostaining of the acrosomal cap and/or sickle-like acrosome ( and D). Rather, in wr spermatids and independently from the post-natal age/differentiation step UBPy immunolabeling resulted primarily in bright spots and/or fluorescent larger granules ( and B) that, however, never develop into the acrosome. Clearly, wr spermiogenesis suffers from defect in the vesicular traffic that in the wild type is engaged in the acrosome biogenesis. Consequently, we have searched for Vps54(L967Q) that so far has not yet been investigated in wr mice. In a previous work,Citation28 wild-type Vps54 was shown to follow the route of UBPy-labeled vesicles during acrosomogenesis. Here we show that in wr testis at each selected time point (35, 44 and 77 days post-partum) Vps54(L967Q) marks numerous punctuated vesicles that are and remain, scattered within the spermatid cytoplasm (, B, D, E, G and H) being not recruited, via the trafficking processes, to a polarized cell region. In other words, Vps54(L967Q)-labeled vesicles do not develop, on the contrary of the wild type Vps54-positive vesicles in the control littermates (, F and I), into an acrosomal structure either at the first spermatogenic wave or at the successive ones. It follows that in the cells phenotypically affected by the wr pathology, the missense mutation Vps54(L967Q) is not irrelevant, but it results in spermiogenetic derailment leading to the production of non functional sperm.
Discussion
The molecular nature of the wr gene defect, a point mutation in the Vps54 gene, has been recently identified.Citation2 This discovery has led to a resurgence of popularity of wr mice as an animal model for human motor neuron diseases.Citation40–Citation42 This, however, did not occur for the other wr disease, male infertility. So, albeit it was known that wr sperm lack a true acrosome,Citation7 no improvement in the understanding of why and how acrosomogenesis is disturbed has been till now obtained. Here we show that in wr mice spermiogenesis is compromised already at the first spermatogenic wave with the consequence that wr males are not only infertile, but sterile since they never produce functional spermatozoa; moreover, our data suggest that the vesicular sorting protein codified by the Vps54 gene could exert a critical role in acrosome biogenesis since as primary defect in spermatids expressing the Vps54(L967Q) variant the altered delivery of endocytic retrograde cargoes hampers the formation of the acrosome. As a secondary defect, since impaired acrosome biogenesis prevents correct shaping of the sperm nucleusCitation35,Citation43 and not viceversa,Citation7 the wr spermatid nuclei do not elongate and not acquire the characteristic sickle-like form, but remain round/irregularly shaped and with ill-condensed chromatin. As further disorder in the intracellular traffic, there is the mislocalization of sperm mitochondria. In wild-type mice during spermatid elongation mitochondria arrange and move along microtubule tracks to give rise to the mitochondrial sheath of the sperm tail mid-piece.Citation43 This event, apparently, does not take place definitely in wr spermatids and wr sperm, when released, are in fact characterized by the lack of the mitochondrial sheath at the tail mid-piece or by its mislocalization. This observation could explain, at least partially, the very poor motility of wr spermatozoa noticed already by Leestma and Sepsenwol.Citation9 Moreover, a further typical hallmark of wr males is that they produce few spermatozoa. Already at the 35th day of life, which in the mouse signs the first event of spermiation and in the wr mouse corresponds to the early evolutionary phase of the disease, wr males have significantly lower numbers of epididymal spermatozoa than their control littermates. The differences in sperm counts are still more pronounced with the progression of the age and, consequently, of the disease. A possible explanation of this phenomenon could be partially found through a detailed histological analysis of the wr testis. In 77 days-old wr testis, age that corresponds to the late evolutionary phase of the disease, spermatogenesis is not only disturbed at the post-meiotic phase, but also the cells residing at the basal compartment of the seminiferous epithelium show signs of sufferance and the number of primary spermatocytes is remarkably reduced in comparison to 77 days-old wild-type testis. Since the survival and proliferation of the germ cells, precursors of haploid spermatids, are guaranteed by the nourishing Sertoli cells,Citation44,Citation45 our finding that in adult wr testis Sertoli-germ cells contacts at the basal compartment are weakened, when not lost at all, could explain the observed decrease in meiotic cells and, consequently, in the next generations of spermatids. It is worth of mention in this regard to remember the crucial role of the endocytic traffic in the testis cell junction dynamic, restructuring and maintenance.Citation46 The histology of wobbler epididymes at each selected time point was consistent with the evaluations above.
It is realized by now that the endocytic traffic at the plasma membrane/endosome level is an important regulator of spermatogenesisCitation47,Citation48 and, in particular, of acrosomogenesis.Citation28,Citation49 Recently, Vps54 has been shown to be involved in acrosome biogenesis, labeling the acrosome until its full maturation.Citation28 Vps54, a component of the GARP complex together with Vps52 and Vps53, is an ubiquitous protein in mammalian cells; however, its mutated variant Vps54(L967Q) seems to affect only motor neurons and sperm cells.Citation2 Why are these cell types particularly hit by the mutation? We hypothesize that functionality of neurons and developing male germ cells is strictly dependent on the function of selected tethering complexes and related, ubiquitin-dependent, sorting of protein cargoes. As to this point, it is of interest to remember here that different domains of Vps54 confer separate functions to the protein. Whereas the N-terminus is important for Vps54 assembly to the GARP complex, the evolutionarily conserved C-terminus is essential to mediate Vps54 localization to the early endosome.Citation17 Tethering complexes, to transport protein cargo intermediates to the appropriate downstream compartment, must recognize and associate to specific membrane factors on both the donor and recipient compartment. It might be that the wr mutation prevents the recognition and/or association of Vps54(L967Q) with spermatid-specific UBPy-labeled early endosomes whereas this does not occur with the wild type Vps54 or even, taking into account the possible existence of neutral polymorphisms,Citation50 with another variant of Vps54 not mutated at the C-terminus. Clearly, our immunofluorescence studies carried out on both wr and wild type testis show that whereas in the control littermates UBPy-labeled and Vps54-labeled vesicular structures coalesce contributing to the development of the acrosomal cap and acrosome, in wr spermatids both UBPy-labeled and Vps54(L967Q)-labeled vesicular structures do not develop into the acrosome, but they remain as scattered vesicles into the cell cytoplasm.
In conclusion, our study on wr spermatogenesis provides experimental evidence that the wr mouse may be a valuable model for human globozoospermia, a syndrome that is due to a genetic backgroundCitation10 and is characterized by sperm possessing a round nucleus, lack of an acrosome and a disorganized tail mid-piece. Although three mouse genesCitation14–Citation16 have been reported as possible responsible of globozoospemia, to date no causative gene mutations have been identified in their human orthologues. Viceversa, Dam et al.Citation13 have identified the first example of human autosomal gene defect, namely a homozygous point mutation in the gene SPATA16, as causative of globozoospemia. The SPATA16 mutation predicts an amino acid change of a highly conserved residue (R283Q) at the C-terminal end of the SPATA16 TPR domain.Citation13 When expressed by transfection experiments into mouse spermatogenic cells, a GFP-fused wild type SPATA16 protein was found to shift from the spermatid Golgi area to the acrosome.Citation51 It might be that in vivo SPATA16 is effectively involved in the vesicle trafficking addressed to the formation of the acrosome. Clearly, the existence of several candidate genes suggests genetic heterogeneity in globozoospermia. It is likely that not even a random gene mutation of a single gene, but rather a mutation at a key position of each gene that codifies a protein essential for acrosomogenesis could be causative of globozoospermia. It follows that to understand globozoospermia it is necessary to know finely and exactly how acrosomogenesis occurs so to develop possibly an acrosome biogenesis-proteome. Again, Wobbler mice could represent a valuable tool to this purpose.
Materials and Methods
Animals.
Wobbler mice and healthy littermates (NFR strain, NIH, Animal Resources, Bethesda, MD) were provided us by Drs. Tiziana Mennini and Paolo Bigini (“Mario Negri” Institute for Pharmacological Research, Milano, Italy) and were bred at Charles River Italia (Calco, Lecco, Italy). Mice were housed at a temperature of 21 ± 1°C with relative humidity of 55 ± 10% and 12 h light/dark cycle. Procedures involving animals and their care were conducted according international laws and policies (EEC Council Directive 86/609, OJ L 358, 1, December 12, 1987; NIH Guide for the Care and Use of laboratory animals, US National Research Council 1996). Genotypization of the progeny from mating heterozygous male and female mice was carried out as described in reference Citation51. Only homozygous wobbler (wr/wr) and control (+/+) males were used. Mice were sacrificed by carbon dioxide asphyxiation at selected time points (post-natal days 35, 44 and 77, considering P 0 the day of birth).
Sperm counts and analyses.
Sperm counts were made on epididymal sperm released from a single cauda epididymis of each animal per selected time point. The tissue, minced with scissor, was incubated in 0.5 ml of sperm motility buffer (135 mM NaCl, 5 mM KCl, 1 mM MgSO4, 2 mM CaCl2, 30 mM HEPES pH 7.4, 10 mM sodium lactate, 1 mM sodium pyruvate, 20 mg/ml bovine serum albumin, 25 mM NaHCO3) and sperm were allowed to swim out for 30 min at room temperature. Sperm motility was visually monitored under phase-contrast microscopy. Numbers of epididymal sperm were determined by hemocytometer counts either undiluted or diluted 10-fold. All counts were made in duplicate and averaged. Data were presented as mean ± SD and analyzed using Student's t test.
Sperm morphology was examined on a Leica DMRB microscope (Leica AG, Heerbrug, Switzerland) equipped with standard filter set for blue fluorescence by photographing unfixed spermatozoa that were stained with 2 µg/ml 4′,6′-diamidino-2-phenylindole (DAPI) (Sigma-Aldrich; #D9542) to visualize sperm nuclei.
Histology and testis staging.
Testes and a single epididymis from each animal were removed, weighed and immediately immersed in Bouin's fixative overnight at 4°C to be then embedded in paraffin wax, and sectioned at a thickness of 5 µm. For routine histology, sections were stained with hematoxylin/eosin according to standard protocols. For staging of seminiferous tubule cross-sections, these were stained with the Periodic Acid-Schiff (PAS) technique and hematoxylin as a counter stain according to the manufacturer's protocol (Sigma-Aldrich, # 395B). At least 60 testis cross sections from each wobbler and control littermate (three testes from three wobbler mice were examined with a total of about 180 sections per time point per hematoxylin/eosin staining) were randomly selected and the light microscopy images were acquired using a Leica DC 150 camera coupled to a personal computer. All the sections that display normal spermiogenesisCitation36,Citation38 were rated in the category (+) whereas the sections with disturbed/anomalous spermiogenesis were rated in the category (−). Staging of PAS-stained seminiferous tubules was performed on light microscopy images according to the classification of Ahmed and De Rooij.Citation38 Paired comparisons of the diameter values of testis cross-sections between 77 days-old wr and wild-type littermates were made for statistical significance by Student's t test. Observations for counts of primary spermatocytes were made on Bouin-fixed and hematoxylin/eosin-stained 5-µm testis sections from 77 days-old mice, examined at 40× magnification on a Leica microscope. Images of cross-sections, randomly selected, of seminiferous tubules were acquired using the Leica DC 150 camera coupled to a personal computer. Twenty cross-sections were examined from each wr and wild type testis; cytological determination of the hematoxylin-stained spermatocytes was according to Ahmed and De Rooij.Citation38
Immunofluorescence.
After blocking of nonspecific binding sites in 3% bovine serum albumin (Sigma-Aldrich; A9647) in Hepes-buffered saline for 1 h at room temperature, deparaffinized testis sections (5 µm thick) from wr and control littermate mice were incubated with the indicated primary antibody, that is, anti-UBPyCitation53 (dilution 1:300) or anti-Vps54Citation18 (1:100), followed by anti-rabbit Alexa Fluor conjugated (Invitrogen; #A11011, #A11008) secondary antibodies as described in reference Citation28. Nuclei counterstaining to determine nuclear shape and chromatin condensation was carried out with DAPI (2 µg/ml). In samples used as control, primary antibodies were omitted.
Testis sections were examined on a Leica DMRB microscope equipped with standard filter sets for green (Alexa 488), red (Alexa 568) and blue (DAPI) fluorescence. Fluorescence images were captured with a Leica DCF480 charge-coupled device camera (Leica Microsystems) by using Imaging software (Microsoft Corporation) and were elaborated with Adobe Photoshop (Mountain View).
Transmission electron microscopy.
Whole testes were immersed in 3% glutaraldehyde in 0.1 M phosphate buffer (pH 7.2–7.4). After 15 min the samples were cut into small pieces (2–3 mm3) and placed in the same fresh fixative for 4 hrs at room temperature. Specimens were postfixed in 2% osmium tetroxide in 0.1 M phosphate buffer (pH 7.2–7.4) for 2 h at 4°C, dehydrated in a graded ethanol series, and embedded in Epon/Spurr resin (Electron Microscopy Sciences, #14300). Ultrathin sections (70 nm) were cut using a diamond knife on a Reichert Ultracut ultramicrotome, mounted on Cu/Rh grids (200 mesh), contrasted with uranyl acetate and lead citrate and examined with a Zeiss 902 transmission electron microscope (80 kV).
Abbreviations
wr | = | wobbler |
PAS | = | periodic acid Schiff |
Figures and Tables
Figure 1 Morphology of wr spermatozoa. Spermatozoa were collected from the cauda epididymes of 35 days-old wr (A–F) and wild-type (G) mice. Nuclei were counterstained with DAPI. Note the rounded (globic, (A–E) and globoid, (F)) heads lacking an acrosome and the deformed, mislocalized mitochondrial sheath (C–E) of the wr sperm. Scale bar, 5 µm.
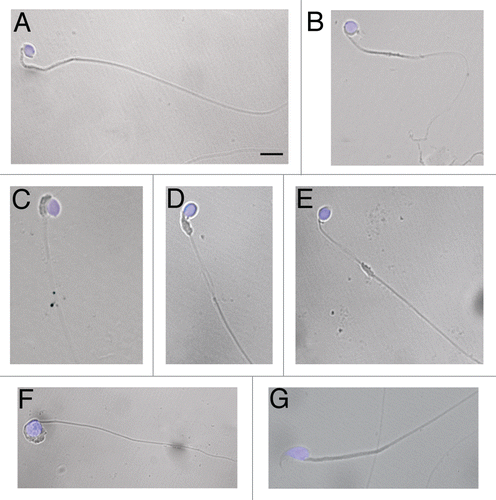
Figure 2 Histology of 35 (A–C), 44 (D–F) and 77 (G–I) days-old testis. Representative cross sections of the seminiferous epithelium of wr (left and middle columns) and wild-type (right column) mice. Black arrowheads and arrows point to round spermatids and elongated spermatids/spermatozoa, respectively. White arrows point to the clumping of round-headed spermatozoa next to be released (D) and to aggregates of residual bodies containing clumped spermatids (G). Asterisk signs the loosening/loss of Sertoli-germ cell contacts at the basal compartment in wr (H) versus wild type littermate (I). Note the round heads/nuclei and vacuolated cytoplasm of wr spermatids/spermatozoa in contrast to the hook-shaped heads devoid of remnants of cytoplasm of the wild-types. Scale bar, 10 µm.
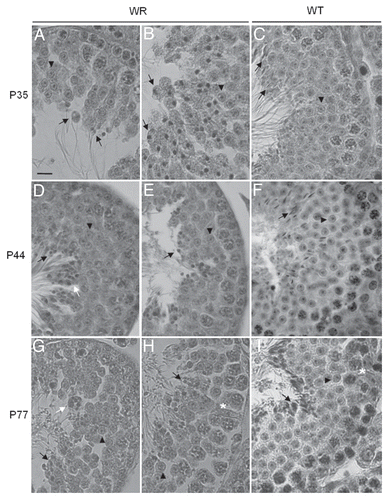
Figure 3 Loosening of anchoring junctions between Sertoli cells and germ cells at the basal compartment in 77 days-old wr testis. (A) Electron micrograph showing a representative cross-section of a seminiferous tubule from 77 days-old wild-type testis. This micrograph also illustrates the intimate relationship between the seminiferous epithelium (composed of Sertoli cells and germ cells) and the basement membrane (BM) of the tunica propria. (B) A magnification of (A) showing the intimate relationship between a Sertoli cell (SC) and a preleptotene spermatocyte (PS). (C) Enlargement of inset shown in (B): black arrowheads indicate the intact germ cell-Sertoli cell junctional complex. (D, F and H) Electron micrographs illustrating an altered architecture of the seminiferous epithelium at the basal compartment in 77 days-old wr testis. Abnormal and extensive intercellular spaces (asterisks) are found between Sertoli cells and spermatocytes (SP) and Sertoli cells and spermatogonia (SG). (E) Magnified view of the boxed area shown in (D). (G) Magnified view of the boxed area shown in (F). (I) Magnified view of the boxed area shown in (H) illustrating a desmosome-like junction, partially still intact, between a spermatogonium and a Sertoli cell. Scale bars, 2 µm (A, F and H); 1 µm (B–E and G); 0.5 µm (I).
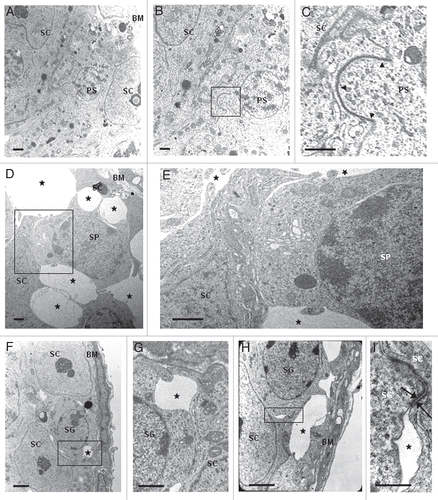
Figure 4 PAS-hematoxylin staining of wild-type (A–F) and wr (G–I) mouse testis to detect different phases of acrosomogenesis. Representative cross-sections of seminiferous tubuli are shown. Stages of the seminiferous epithelial cycle are marked with Roman numerals. PAS-positive (pink) proacrosomal granules, acrosomal caps and acrosomes are indicated by arrows. In contrast to the wild type (D–F), no evident acrosomal caps and acrosomes were detected, independently from the acrosomogenetic step, in the wr testis (G–I). PAS-staining resulted only in punctuate spots (arrows) scattered into the wr spermatid cytoplasm. Scale bar, 10 µm.
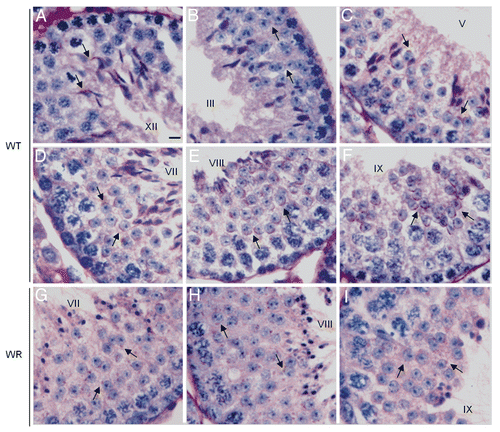
Figure 5 Localization of UBPy in testis from wr mice. Sections of wr (A and B) and wild-type (C and D) testes at stage VII of the seminiferous epithelial cycle were immunofluorescently labeled with antibodies to UBPy (red); DAPI (blue) was used to stain nuclei. Note the diffuse punctuate staining of UBPy in wr spermatids; in particular, the enlargement in (B) shows that no spermatid at step 7 exhibits the brightly fluorescent UBPy-immunostaining of the acrosomal cap characteristic of the wild-type step 7 spermatids (enlargement in D). Scale bars, 20 µm (A), 26 µm (C) and 10 µm (B and D).
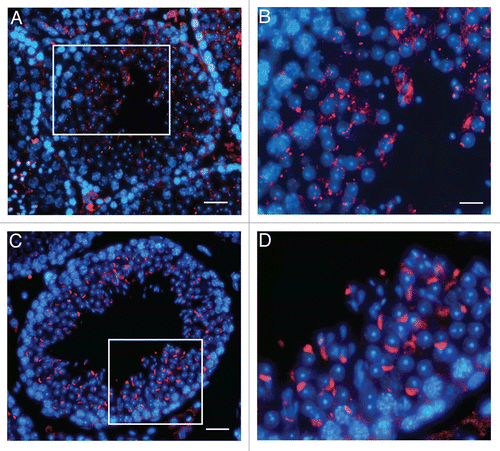
Figure 6 Localization of Vps54(L967Q) by immunofluorescence analysis in wr testis at different ages (P35, P44 and P77). Stages of the seminiferous epithelial cycle are marked with Roman numerals. Sections of wr (left and middle columns)) and wild-type littermate (right column) testes were labeled with antibodies to Vps54 (green); DAPI (blue) was used to stain nuclei. Compare the Vps54(L967Q) and Vps54 immunostainings. Vps54(L967Q) marks punctuated vesicles scattered within the spermatid cytoplasm, independently from the acrosomogenetic step. These vesicles do not develop into a polarized acrosomal cap (A and B, G and H) and acrosome (D and E) as, on the contrary, it occurs for the Vps54-labeled vesicles in the respective stages of the wild-type littermate spermatids/spermatozoa (C, I and F, respectively). Scale bars, 10 µm (A, D and G); 5 µm (B, C, F and I); 6 µm (E and H).
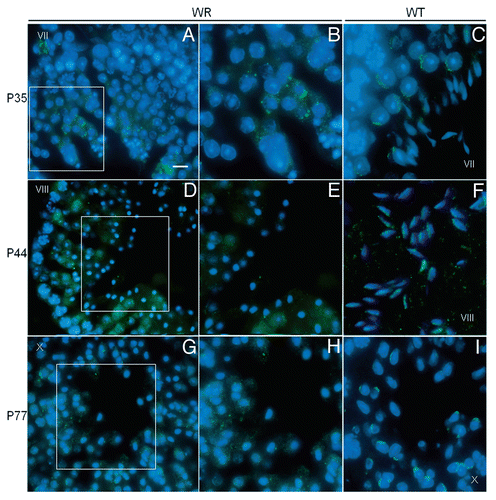
Table 1 Testis weights, epididymal sperm counts and seminiferous tubule diameters of age-matched wobbler and wild-type male mice
Additional material
Download Zip (3.7 MB)Acknowledgements
The Authors thank Dr. T. Mennini and Dr. P. Bigini (“Mario Negri” Institute for Pharmacological Research, Milano, Italy) for supplying the wobbler strain and Dr. F. Stenner (Zurich Hospital, Switzerland) for Vps54 antibody. This work was supported by PRIN 2008 and PUR 2009 to G.B.
References
- Falconer DS. Wobbler Mouse. Newsletter 1956; 15:23
- Schmitt-John T, Drepper C, Mussmann A, Hahn P, Kuhlmann M, Thiel C, et al. Mutation of Vps54 causes motor neuron disease and spermiogenesis defect in the wobbler mouse. Nat Genet 2005; 37:1213 - 1215
- Boillée S, Peschanski M, Junier MP. The wobbler mouse: a neurodegeneration jigsaw puzzle. Mol Neurobiol 2003; 28:65 - 106
- Diana V, Ottolina A, Botti F, Fumagalli E, Calcagno E, De Paola M, et al. Neural precursor-derived astrocytes of wobbler mice induce apoptotic death of motor neurons through reduced glutamate uptake. Exp Neurol 2010; 225:163 - 172; PMID: 20558160
- Bastone A, Fumagalli E, Bigini P, Perini P, Bernardinello D, Cagnotto A, et al. Proteomic profiling of cervical and lumbar spinal cord reveals potential protective mechanisms in the wobbler mouse, a model of motor neuron degeneration. J Proteome Res 2009; 8:5229 - 5240; PMID: 19764823
- Duchen LW, Strich SJ. An hereditary motor neuron disease with progressive denervation of muscle in the mouse: The mutant “wobbler”. J Neurol Neurosur Ps 1968; 31:535 - 542
- Heimann P, Laage S, Jockusch H. Defect of sperm assembly in a neurological mutant of the mouse, wobbler (WR). Differentiation 1991; 47:77 - 83
- Augustin M, Heimann P, Rathke S, Jockusch H. Spinal muscular atrophy gene wobbler of the mouse: evidence from chimeric spinal cord and testis for cell-autonomous function. Dev Dyn 1997; 209:286 - 295
- Leestma JE, Sepsenwol S. Sperm tail axoneme alterations in the Wobbler mouse. J Reprod Fertil 1980; 58:267 - 270
- Dam AHDM, Feenstra I, Westphal JR, Ramos L, van Golde RJT, Kremer JAM. Globozoospermia revisited. Hum Reprod Update 2007; 13:63 - 75
- Kilani Z, Ismail R, Ghunaim S, Mohamed H, Hughes D, Brewis I, et al. Evaluation and treatment of familial globozoospermia in five brothers. Fertil Steril 2004; 82:1436 - 1439
- Christensen GL, Ivanov IP, Atkins JF, Campbell B, Carrell DT. Identification of polymorphisms in the Hrb, GOPC and Csnk2a2 genes in two men with globozoospermia. J Androl 2006; 27:11 - 15
- Dam A, Koscinski I, Kremer J, Moutou C, Jaeger A, Oudakker A, et al. Homozygous mutation in SPATA16 is associated with male infertility in human globozoospermia. Am J Hum Genet 2007; 81:813 - 820; PMID: 17847006
- Xu X, Toselli PA, Russell LD, Seldin DC. Globozoospermia in mice lacking the casein kinase ii alpha2 catalytic subunit. Nat Genet 1999; 23:118 - 121
- Kang-Decker N, Mantchev GT, Juneja SC, McNiven MA, van Deursen JMA. Lack of Acrosome Formation in Hrb-Deficient Mice. Science 2001; 294:1531; http://dx.doi.org/10.1126/science.1063665
- Yao R, Ito C, Natsume Y, Sugitani Y, Yamanaka H, Kuretake S, et al. Lack of acrosome formation in mice lacking a Golgi protein, GOPC. Proc Natl Acad Sci USA 2002; 99:11211 - 11216; http://dx.doi.org/10.1073/pnas.162027899
- Quenneville NR, Chao TY, McCaffery JM, Conibear E. Domains within the GARP Subunit Vps54 Confer Separate Functions in Complex Assembly and Early Endosome Recognition. Mol Biol Cell 2006; 17:1859 - 1870
- Liewen H, Meinhold-Heerlein I, Oliveira V, Schwarzenbacher R, Luo G, Wadle A, et al. Characterization of the human GARP (Golgi associated retrograde protein) complex. Exp Cell Res 2005; 306:24 - 34
- Pérez-Victoria FJ, Mardones GA, Bonifacino JS. Requirement of the Human GARP Complex for Mannose 6-phosphate-receptor-dependent Sorting of Cathepsin D to Lysosomes. Mol Biol Cell 2008; 19:2350 - 2362
- Schirren CG, Holstein AF, Schirren C. Uber die morphogenese rund-kopfiger spermatozoen des menschen. Andrologia 1971; 3:117 - 125
- Tang XM, Lalli MF, Clermont Y. A cytochemical study of the Golgi apparatus of the spermatid during spermiogenesis in the rat. Am J Anat 1982; 163:283 - 294
- Burgos MH, Gutierrez LS. The Golgi complex of the early spermatid in guinea pig. Anat Rec 1986; 216:139 - 145
- Martinez MJ, Geuze HJ, Ballesta J. Evidence for a nonlysosomal origin of the acrosome. J Histochem Cytochem 1996; 44:313 - 320
- Moreno RD, Ramalho-Santos J, Chan EK, Wessel GM, Schatten G. The Golgi apparatus segregates from the lysosomal/acrosomal vesicle during rhesus spermiogenesis: structural alterations. Dev Biol 2000; 219:334 - 349
- Sun-Wada GH, Imai-Senga Y, Yamamoto A, Murata Y, Hirata T, Wada Y, Futai M. A proton pump ATPase with testis-specific E1-subunit isoform required for acrosome acidification. J Biol Chem 2002; 277:18098 - 18105
- Moreno RD, Palomino J, Schatten G. Assembly of spermatid acrosome depends on microtubule organization during mammalian spermiogenesis. Dev Biol 2006; 293:218 - 227
- Moreno RD, Alvarado CP. The mammalian acrosome as a secretory lysosome: new and old evidence. Mol Reprod Dev 2006; 73:1430 - 1434
- Berruti G, Ripolone M, Ceriani M. USP8, a regulator of endosomal sorting, is involved in mouse acrosome biogenesis through interaction with the spermatid ESCRT-0 complex and microtubules. Biol Reprod 2010; 82:930 - 939
- Row PE, Prior IA, McCullough J, Clague MJ, Urbe S. The ubiquitin isopeptidase UBPY regulates endosomal ubiquitin dynamics and is essential for receptor downregulation. J Biol Chem 2006; 281:12618 - 12624
- Mizuno E, Kobayashi K, Yamamoto A, Kitamura N, Komada M. A deubiquitinating enzyme UBPY regulates the level of protein ubiquitination on endosomes. Traffic 2006; 7:1017 - 1031
- Alwan HA, van Leeuwen JE. UBPY-mediated epidermal growth factor receptor (EGFR) deubiquitination promotes EGFR degradation. J Biol Chem 2007; 19:1658 - 1669
- Berruti G, Martegani E. The deubiquitinating enzyme mUBPY interacts with the sperm specific molecular chaperone MSJ-1: the relation with the proteasome, acrosome and centrosome in mouse male germ cells. Biol Reprod 2005; 72:14 - 21
- Ulbrich M, Schmidt V, Ronsiek M, Mussmann A, Bartsch J, Augustin M, et al. Genetic modifiers that aggravate the neurological phenotype of the wobbler mouse. Neuroreport 2002; 13:535 - 539
- Huang X, Guo X, Shen J, Wang Y, Chen L, Xie J, et al. Construction of a proteome profile and functional analysis of the proteins involved in the initiation of mouse spermatogenesis. J Proteome Res 2008; 7:3435 - 3446
- Lin YN, Roy A, Yan W, Burns KH, Matzuk MM. Loss of Zona Pellucida binding proteins in the acrosomal matrix disrupts acrosome biogenesis and sperm morphogenesis. Mol Cell Biol 2007; 27:6794 - 6805
- Oakberg EF. A description of spermiogenesis in the mouse and its use in analysis of the cycle of the seminiferous epithelium and germ cell renewal. Am J Anat 1956; 99:391 - 413; http://dx.doi.org/10.1002/aja.1000990303
- Yan HH, Mruk DD, Lee WM, Cheng CY. Ectoplasmic specialization: a friend or a foe of spermatogenesis?. Bioessays 2007; 29:36 - 48
- Ahmed EA, De Rooij DG. Staging of mouse seminiferous tubule cross-sections. Methods Mol Biol 2009; 558:263 - 277
- Chianese R, Scarpa D, Berruti G, Cobellis G, Pierantoni R, Fasano S, et al. Expression and localization of the deubiquitinating enzyme mUBPy in wobbler mouse testis during spermiogenesis. Gen Comp End 2010; 166:2289 - 2295
- Dennis JS, Citron BA. Wobbler mice modeling motor neuron disease display elevated transactive response DNA binding protein. Neuroscience 2009; 158:745 - 750
- Bastone A, Fumagalli E, Bigini P, Perini P, Bernardinello D, Cagnotto A, et al. Proteomic profiling of cervical and lumbar spinal cord reveals potential protective mechanisms in the wobbler mouse, a model of motor neuron degeneration. J Prot Res 2009; 8:5229 - 5240
- Meyer M, Gonzalez Deniselle MC, Garay LI, Gargiulo Monachelli G, Lima A, Roig P, et al. Stage dependent effects of progesterone on motoneurons and glial cells of wobbler mouse spinal cord degeneration. Cell Mol Neurobiol 2010; 30:123 - 135; http://dx.doi.org/10.1007/s10571-009-9437-8
- Kierszenbaum AL, Tres LL. The acrosome-acroplaxome-manchette complex and the shaping of the spermatid head. Arch Histol Cytol 2004; 67:271 - 284
- Kopera IA, Bilinska B, Cheng CY, Mruk DD. Sertoli-germ cell junctions in the testis: A review of recent data. Phil Trans R Soc B 2010; 365:1593 - 1605; http://dx.doi.org/10.1098/rstb.2009.0251
- Aivatiadou E, Mattei E, Cerini M, Tilia L, Berruti G. Impaired fertility and spermiogenetic disorders with loss of cell adhesion in male mice expressing an interfering Rap1 mutant. Mol Biol Cell 2007; 18:1530 - 1542
- Su L, Mruk DD, Lee WM, Cheng CY. Differential effects of testosterone and TGFβ3 on endocytic vesicle-mediated protein trafficking events at the blood-testis barrier. Exp Cell Res 2010; 316:2945 - 2960; PMID: 20682309
- Zhu G, Salazar G, Zlatic SA, Fiza B, Doucette MM, Heilman CJ, et al. SPE-39 family proteins interact with the HOPS complex and function in lysosomal delivery. Mol Biol Cell 2009; 20:1223 - 1240
- Rainey MA, George M, Ying G, Akakura R, Burgess DJ, Siefker E, et al. The endocytic recycling regulator EHD1 is essential for spermatogenesis and male fertility in mice. BMC Dev Biol 2010; 10:37 - 43; PMID: 20359371
- Li S, Qiao Y, Di Q, Le X, Zhang L, Zhang X, et al. Interaction of SH3P13 and DYDC1 protein: a germ cell component that regulates acrosome biogenesis during spermiogenesis. Eur J Cell Biol 2009; 88:509 - 520
- Meisler MH, Russ C, Montgomery KT, Greenway M, Ennis S, Hardiman O, et al. Evaluation of the Golgi trafficking protein VPS54 (wobbler) as a candidate for ALS. Amyotroph Lateral Scler 2008; 9:141 - 148
- Lu L, Lin M, Xu M, Zhou ZM, Sha JH. Gene functional research using polyethylenimine-mediated in vivo gene transfection into mouse spermatogenic cells. Asian J Androl 2006; 8:53 - 59
- Rathke-Hartlieb S, Schmidt VC, Jockusch H, Schmitt-John T, Bartsch JW. Spatiotemporal progression of neurodegeneration and glia activation in the wobbler neuropathy of the mouse. NeuroReport 1999; 10:3411 - 3416
- Gnesutta N, Ceriani M, Innocenti M, Mauri I, Zippel R, Sturani E, et al. Cloning and characterization of mouse UBPY, a deubiquinating enzyme that interacts with the ras guanine nucleotide exchange factor CD25(Mm)/Ras-GRF1. J Biol Chem 2001; 276:39448 - 39454