Abstract
Spermatogenesis is a series of cellular processes that leads to the development of motile, elongate sperm cells. Mitotic expansion of spermatogenic stem cells is followed by two meiotic cell divisions that yield haploid round spermatids which then transform from a spherical form into an elongate, highly polarized form. In Drosophila, spermatogenesis takes place within encapsulating cysts that contain spermatogenic cells. Spermatogenic cysts were isolated and grown in culture over the course of 96 hours. Cultures were treated with buthionine sulfoximine (BSO), glutathione (GSH), insulin, and GSH+insulin in order to test the effects of these agents on cyst viability. The addition of glutathione and exogenous insulin to cultured spermatogenic cysts each appeared to have a positive effect on early spermatogenic cyst survival in vitro at some timepoints. The addition of GSH+insulin together had no significant effect on early spermatogenic cyst survival in vitro. Oxidative stress induced by BSO resulted in a significant decrease and/or complete loss of specific early spermatogenic cyst types and the abnormal development of elongating cysts in culture. This culture system offers the opportunity for high-resolution analysis of spermatogenic processes not previously possible.
Introduction
Spermatogenesis in Drosophila species proceeds within paired, blind-ended, tubular or ellipsoid testes. In both flies and mammals, a stem cell regeneration system provides a continuous supply of precursor sperm cells. The sperm stem cell niche in flies resides at the apical end of the testes and consists of three types of cells: germline stem cells (GSCs), cyst progenitor cells (or cyst stem cells, CySCs) and hub cells.Citation1–Citation5 The hub cells constitute a signaling center responsible for maintaining the stem cell populations in an undifferentiated state.Citation2,Citation6–Citation8 In Drosophila melanogaster, GSCs and CySCs are arranged in a rosette around the hub cells.Citation9 The mitotic division of a GSC results in a gonialblast committed to differentiation and a GSC that remains in contact with the hub. The cyst progenitor cells also divide mitotically to produce a stem cell and a cell that will terminally differentiate into a cyst cell—a pair of which will encapsulate the germline cells throughout spermatogenesis.Citation5,Citation10 Although the roles of the cysts cells is not fully characterized, it is known that germ cell survival relies upon signaling from the cyst cells.Citation11 Spermatogenesis proceeds within cysts from the apical end of the testes towards the basal end. As in mammals, the fly gonialblast proliferates mitotically before entering into meiosis. In D. pseudoobscura, five mitotic divisions result in 32 diploid clones called spermatogonia.Citation12 The spermatogonia then rapidly progress through pre-meiotic S-phase and enter an extended G2 cell cycle phase in which they grow up to approximately 25 times in volume.Citation2,Citation13 At this stage, the cells are termed primary spermatocytes. Primary spermatocytes undergo the first meiotic division to create 64 secondary spermatocytes. The second meiotic division gives rise to 128 haploid round spermatids. Round spermatids undergo a final, post-meiotic stage of maturation termed spermiogenesis, where the nuclei transform into the species-specific elongate shape and the sperm tails are assembled.Citation14–Citation16 During all stages of spermatogenesis and spermiogenesis, the spermatogenic cells remain interconnected by cytoplasmic bridges. The final stage of spermiogenesis involves each individual cell being enrobed within a individual plasma membrane in a process called individualization.Citation16,Citation17 The cells then coil at the basal end of the testis, burst from the encapsulating cyst and relocate to the seminal vesicle for storage until mating.
In a previous study, we successfully isolated and cultured spermatogenic cysts from the pupal-stage testes of D. pseudoobscura.Citation18 This culture system offers a means to study the cellular spermatogenic differentiation processes at high resolution in vitro. The testes of D. pseudoobscura can be easily distinguished in the later pupal stages due to intense pigmentation, and are easily handled due to their ellipsoid morphology. The survival of cysts to the elongated and motile form was consistently achieved in vitro. However, the degeneration of early spermatogenic cysts (spermatogonia and primary spermatocyte) was frequently observed in the culture system. Previously, Niki et al.Citation19 established an in vitro system to analyze factors that regulate the division and differentiation of GSCs in D. melanogaster ovaries. Kawamoto et al. reported the differentiation of primary spermatocytes and dissociated spermatogonia into motile spermatids in vitro. Exogenous insulin and glutathione (GSH) were included in the culture media in these systems. Insulin can act as a mitogen for various cell types in culture, and an insulin receptor and its signaling cascade are well conserved in Drosophila.Citation21 Drosophila insulin-like peptides are known to promote the proliferation and growth of somatic cells, and the division of GSCs in females.Citation22 Glutathione is a three amino acid peptide that is highly conserved in animals,Citation23 plants,Citation24 microbes.Citation25 Its synthesis, reduction and oxidation is key to controlling reactive oxidative species (ROS) in cells. The regeneration of oxidized GSH to the active, reduced form via either the glutathione reductase pathway or a thioredoxin systemCitation26 is well-characterized, with the latter existing in D. melanogaster.Citation27,Citation28 In mammals, GSH is involved in the protection of both males and female gametes against oxidative damage.Citation29
The purpose of the current work was to study the effects of exogenous insulin and GSH on spermatogenic cyst viability in vitro, especially the early spermatogenic cysts that are susceptible to degradation in culture. Additionally, we sought to investigate the effects of oxidative stress on these cyst types using the reagent buthionine sulfoximine (BSO). BSO disrupts the synthesis of GSH by inhibiting the enzyme gamma-glutamylcysteine synthaseCitation30,Citation31 which is responsible for catalyzing the synthesis reaction to create the dipeptide glutamate-cysteine prior to the addition of glycine to complete the tripeptide. This system is present in DrosophilaCitation30 and BSO has been shown to be effective for producing oxidative stress conditions in cultured Drosophila cells.Citation31 In the current work, in untreated cultures as well as those treated with GSH-only, insulin-only, and GSH+insulin, the survival of cysts to the elongated, motile form was consistently achieved. Statistical analysis and performance factor analysis showed that cultures treated with GSH only demonstrated a slightly higher survival rate of spermatogonia and primary spermatocyte cysts over 96 hours in culture as compared to untreated controls. The addition of insulin only to the culture medium had a similar effect on early cyst survival, while spermatogenic cysts in cultures treated with GSH+insulin were comparable in performance with untreated control cultures after 96 hours in vitro. Degeneration analysis of primary spermatocyte cysts indicated that the addition of GSH and insulin to culture had little or no effect on the degeneration of PS cysts over time. The induction of oxidative stress by the addition of BSO resulted in significant decrease and/or complete loss of early spermatogenic cysts by 48 hours in culture. Additionally, BSO treated cysts at the elongation stage of spermiogenesis exhibited abnormalities that indicated that oxidative stress disrupts the elongation process.
Results
Cyst cultures.
Whole testes with enclosed cysts are shown in and B. When initially isolated from pupal testes, cysts in advanced post-elongation stages of spermiogenesis (individualization and coiling; and C), were never observed. At the initiation of culture, each well contained an average of approximately 400 total cysts for insulin and GSH treated cultures and controls. For BSO treated cultures and controls, the starting average was approximately 1,000 cysts per well. The method for cyst isolation for BSO cultures gave a better yield of cysts and also gave slightly different ratios of cyst types present at the initiation of culture (). Cyst maturation was observed after 24 hours in all cultures as indicated by the presence of the cystic bulge on some cysts and initial coiling of cysts. and B show examples of individualizing and coiling cysts seen after 72 hours in culture. Additionally, after the first 24 hours in culture, the number of spermatogonia and primary spermatocyte cysts declined steeply in GSH, insulin and GSH/insulin controls ( and ). In almost all cases, the number of secondary spermatocytes and round spermatids (SS+RS) increased in number after 24 hours in culture, indicating the differentiation of primary spermatocytes ( and ). In GSH, insulin and GSH+insulin treated cultures, the increase in the number of elongating cysts after 24 hours ( and ) can be attributed to the cyst isolation method. Clumps of elongating cysts dissociate during the first few hours in culture. As previously reported in reference Citation18, by 96 hours in culture, most of the surviving cysts were fully developed and motility was often observed in both treated and untreated cultures.
Glutathione-only treatment.
Over the course of 96 hours in culture, GSH treated cultures showed increases in meiotic cysts (secondary spermatocytes and round spermatids) and cysts at the elongation stage of development (pooled data; and and ). After 24 hours, there was a significant decrease in the percentage and raw number of mitotic (spermatogonia) cysts and pre-meiotic (primary spermatocyte) cysts ( and and ). After 48 hours, the percentage of mitotic and premeiotic cysts continued to decrease, while the percentage of meiotic and coiling cysts increased. GSH treated cultures showed an increase in secondary spermatocyte + round spermatid cysts after 24, 48 and 72 hours in culture (). Interestingly, after 48 hours the percentage of coiling cysts was higher in the untreated cultures as compared to the treated cultures (pooled data; ). This trend continued up to 96 hours. Statistical analysis of three GSH-treated cultures indicated a significant effect on spermatogonia and primary spermatocyte cyst survival after 24, 48, 72 and 96 hours in culture (p < 0.05; and ). However, the starting percentage of primary spermatocytes was also significantly different at the initiation of GSH culture (). This discrepancy was negated by pooling the starting percentages of PS and SG into one category (early spermatogenic cysts; ). Performance factor ratios for spermatogonia and primary spermatocyte cyst percentages relative to untreated controls were computed on pooled data (). GSH effect on performance of early cyst survival relative to untreated controls approximately doubled by 96 hours in culture (). The degeneration analysis of primary spermatocytes for GSH showed a significant difference relative to untreated controls at 72 hours in culture ().
Insulin-only treatment.
As with GSH treated cultures, insulin treated cultures showed a significant decrease in the percentage of spermatogonia and primary spermatocyte cysts after 24 hours in culture ( and and ). Secondary spermatocyte and round spermatid cyst percentage increased up to 48 hours in culture, then declined until 96 hours. After 72 hours the untreated cultures had a higher percentage of coiled cysts over insulin treated cultures (). The raw number of secondary spermatocytes + round spermatids increased after 24 hours in culture and declined thereafter ().
Statistical analysis of the three insulin treated cultures indicated a significant effect of treatment on early cyst survival at 72 and 96 hours (p < 0.05; and ). Performance factor analysis of pooled insulin culture data indicated that insulin treatment improved spermatogonia survival by approximately by a factor of 2 and primary spermatocyte survival by a factor of 1.7 (). Degeneration analysis of primary spermatocytes indicated no significant difference relative to untreated controls after 96 hours in culture ().
GSH+insulin treatment.
After 24 hours, cultures treated with GSH and insulin combined showed an increase in the percentage and number of secondary spermatocyte, round spermatid and post-meiotic cysts present, while (as with all other conditions reported) the percentage and number of mitotic and pre-meiotic cysts decreased (pooled data; and and ). The percentage of coiling cysts increased in a manner similar to untreated controls up to 96 hours (). For all time points, statistical analysis showed that the percentages of early cysts in GSH+insulin treated cultures were not significantly different from untreated cultures (p > 0.05; and ).
Performance factor analysis showed that the ratio of spermatogonia cysts in GSH+insulin treated cultures compared to those in untreated cultures was less than 1.0 after 24, 72 and 96 hours, indicated that the combination had no effect on spermatogonia cyst survival (). The performance factor for primary spermatocyte cyst survival was slightly better, reaching 1.5 by 72 hours in culture, but declining to1.28 by 96 hours. Degeneration analysis of primary spermatocytes showed no significant difference relative to untreated controls ().
BSO treatment.
By 24 hours in culture, the percentage and number of primary spermatocyte cysts dropped sharply, and by 48 hours in culture the percentage dropped to zero ( and and ). By 24 hours, the difference in percentage of primary spermatocyte cysts present in culture compared to untreated controls was statistically significant (p < 0.05; ). Performance factor analysis of pooled results was below 1 for both spermatogonia cysts and primary spermatocyte cysts by 96 hours in culture (). Interestingly, BSO-treated cysts at the elongation stage of spermiogenesis showed abnormal elongation () and in some cases, disorganization of elongating sperm heads (). In some cases, the sperm nuclei of pre-elongation round spermatids also appeared disorganized ().
Discussion
In treated and untreated cultures, cyst maturation as well as motility was consistently achieved. However, we sought to determine the effect of exogenous GSH and insulin treatments on the overall survival of spermatogenic cysts, and the survival of early cysts specifically. Cysts at the spermatogonia and primary spermatocyte stage frequently degenerated in culture as observed in a previous study.Citation18 Analysis of the datasets generated in the current work was complicated by the fact that, for each cyst type, it was not always possible to determine if cyst numbers were changing due to degeneration, differentiation or both. However, early cyst survival as analyzed by calculating the percentages of SG and PS over time may be reasonable given that the SG category consisted of 8, 16 and 32 cell stages (1, 2 and 4 cell stages could not be discerned), and PS have an extended growth phase. Cyst degeneration could also be calculated in the case of primary spermatocyte as the increased number of cysts in the next successive developmental stage (SS+RS) could only be derived from differentiating primary spermatocytes. It was assumed the remaining decline in PS cyst number was attributable to degeneration, but again, this cannot be definitively stated as it was not possible to determine the extent of cyst contribution from the developing SG stage. Despite these difficulties, our analyses appear to indicate that the addition of GSH and/or insulin to cultures had a slight positive effect on the survival of early spermatogenic cysts as compared to untreated controls and as compared to cultures treated both insulin and GSH together. Interestingly, we did not detect any significant effect on early spermatogenic cyst survival or primary spermatocyte degeneration in cultures treated with GSH+insulin; no synergistic effect over time when both agents were used was observed. A possible explanation may be derived from the structure of GSH and insulin. GSH contains a thiol group that participates in the reduction of other molecules. The bovine insulin used in our study contains three disulphide linkages produced by cysteine amino acid residues. Davidson and HirdCitation33 investigated the reaction of glutathione with the disulphide bonds of purified proteins, including bovine serum albumin, pepsin and insulin. These workers showed that the three disulphide bonds of bovine insulin are reduced by glutathione. Proteolysis increased the reactivity of the proteins analyzed because the disulphide bonds within them became more accessible to glutathione. Native proteins under physiological conditions do not react with glutathione because the disulphide bonds are structurally protected by steric hindrance or hydrophobic regions surrounding the bonds.Citation33 Our present study suggests that GSH and insulin interaction inactivated both agents so that survival of early cysts was not affected by their addition. Other culture additives have been used in germ cell culture systems and may prove useful for the one described in the current work. These include fly extract,Citation19,Citation20 larval extract,Citation20 and ecdysone.Citation37
Oxidative stress produced by treatment with BSO had a significant effect on the degeneration of primary spermatocyte cysts, with this group completely disappearing from culture by 48 hours. Primary spermatocytes are highly metabolically active cells, growing in size by approximately 25 times and producing mRNAs necessary for later stages of spermiogenesisCitation13 and therefore may be more susceptible to oxidative stress and free radicals as compared to other cell types. Additionally, abnormal morphologies were observed in elongating and pre-elongating cysts in BSO-treated cultures. The reason for this is unknown, however, elongating cysts are also metabolically active in that the sperm axoneme is being assembled and the nucleus is reorganizing and elongating.Citation15,Citation16 The cyst cells themselves are also actively growing in order to accommodate the elongating sperm within. Oxidative stress resulting from a build-up of free radicals could be expected to have an effect on elongating cysts. The BSO-treated and BSO-control cultures themselves also behaved slightly differently than GSH/insulin cultures. The elongating cysts in BSO control cultures declined at a lower rate than those in GSH/insulin treated cultures and controls. Additionally, BSO control cultures did not exhibit an increase in SS+RS cysts after 24 hours in culture. We may speculate that these effects could be attributed to the different ratios of cyst types and the higher overall concentration of cysts obtained by the method used to isolate BSO-treated and BSO controls. This is not an unreasonable speculation given that overall culture health requires a minimum concentration of cysts at the initiation of culture.Citation18 It is unknown what factors account for this effect.
Seven genes encoding insulin-like peptides (ILPs) have been identified in D. melanogaster genome.Citation34 These peptides are synthesized in clusters of medial neurosecretory cells in the Drosophila brain, but are expressed in various areas of the fruit fly. For example, DILP7 is expressed in neurons that innervate the female reproductive tract.Citation34 An ILP receptor (insulin receptor, InR) and its downstream signaling cascade are well conserved in D. melanogaster. To understand the biological effects of insulin on cell growth and differentiation, Fernandez et al. genetically cloned Drosophila homologs of the insulin signaling pathway. They reported that loss of function mutations in the inr gene lead to embryonic lethality. Ueishi et al. reported that GSC maintenance and spermatocyte growth was impaired or reduced by inhibited insulin signaling in Drosophila mutants. Inhibition of insulin signaling resulted in a lower abundance of germline cells in Drosophila testes. In addition, spermatocytes growth was compromised in mutants that lacked insulin signaling, suggesting that insulin signaling plays a role in inducing the growth seen in primary spermatocytes. Our analyses indicate that exogenous insulin had a small positive effect on the survival of early cysts in culture at 72 and 96 hours in culture.
Our results for the culture of cysts isolated from D. pseudoobscura testes resemble germ cell development and differentiation in early reports of in vitro spermatogenic cyst culture of other Drosophila species, with some important differences. Cross and ShellenbargerCitation32 and LiebrichCitation35 reported a low yield of fully elongated cysts, and motility was never observed in D. melanogaster and D. hydei cultures, respectively. Fowler and JohannissonCitation36 did not observe sperm motility in culture using Drosophila hydei.
Recent studies have successfully investigated in vitro sperm culture in D. melanogaster. Noguchi and MillerCitation17 used this system with adult testes starting material to analyze the role of actin in the individualization process in D. melanogaster. The use of adult testes yielded a large number of cysts undergoing individualization and coiling. However, cysts at advanced stages of development at the onset of culture are not favorable for in vitro analysis of the molecular mechanisms involved in nuclear transformation, which occurs from the round spermatid stage through the elongation stage. Our studies utilized pupal testes in order to isolate a large number of earlier stage cysts because we are particularly interested in the transformation of the sperm nucleus. Kawamoto et al. used larval testes from D. melanogaster as a starting material for the study of Drosophila spermatogenesis in vitro. They showed that sperm development and motility can be achieved in culture using cysts from testes from early and late larva. In our hands, the isolation of cysts from larval testes was unsuccessful because the testes are delicate at this stage, and thus easily damaged. Additionally, the testes are not pigmented in the larval stage and are therefore difficult to locate in the larval abdomen.
Cross and ShellenbargerCitation32 reported that in vitro sperm coiling in D. melanogaster cyst cultures did not always follow the completion of individualization as in vivo. Additionally, the initiation of in vitro coiling occurred at variable stages of the individualization process. They also reported the in vitro development from meiosis to a coiled state was less than one-half the estimated in vivo time. Our results showed that in cultures treated with GSH 23.7% of cysts were at the coiled stage, while 28.7% of cysts were in the coiled stage in untreated cultures by 96 hours (pooled data). Development of cysts at the coiled stage in untreated cultures also out-performed cultures treated with insulin at 96 hours by a difference of 10.5%. This indicates that in untreated cultures, cysts progress to the coiling stage at a much faster rate. However in cultures treated with GSH+insulin, there was only a 0.7% difference in percentage of coiling cysts relative to untreated controls. This suggests that the addition of GSH and insulin independently retards the cyst coiling process in vitro. The reason for this apparent retardation of coiling is unknown.
Materials and Methods
Chemicals.
Jazz Mix Drosophila medium was purchased from Fisher. Shields and Sang M3 medium, fetal calf serum, penicillin/streptomycin, insulin from bovine pancreas, glutathione and buthionine sulfoximine were purchased from Sigma-Aldrich.
Fly stock and cultures.
Fly stocks were obtained from the University of California San Diego Drosophila Species Stock Center. All flies were cultured in our laboratory on Jazz Mix Drosophila medium at 22°C.
Culture medium.
The culture media used was based on previous studies in reference Citation18 and Citation32. Powdered Shields and Sang M3 Insect medium without bicarbonate was reconstituted according to manufacturer's instructions and supplemented with 10% fetal calf serum and 1% penicillin/streptomycin cocktail according to previous studies in reference Citation18–Citation20. The serum was heat-inactivated at 50°C for 30 minutes. Treated cultures were supplemented with either 10 µg/ml insulin from bovine pancreas, 0.6 mg/ml glutathione, or both insulin and GSH together. Insulin and GSH concentrations were as in Niki et al.Citation19 and Kawamoto et al. BSO treated cultures were treated with 1 mM BSO. Supplemented culture medium was prepared with GSH-only, insulin-only, GSH+insulin, and BSO on the day of culture initiation. Untreated control cultures for insulin/GSH experiments were grown in supplemented medium that lacked insulin and/or GSH. BSO control cultures were supplemented with 0.6 mg/ml glutathione.
In vitro culture of isolated cysts.
Pupae were harvested five to seven days after pupation began. They were soaked in 70% ethanol for 15 minutes and dissected in 1x phosphate buffered saline (PBS) with 1% penicillin/streptomycin on a sterilized bench. After dissection, three testes were washed twice in 1x PBS and once in 50 µl supplemented culture media. The testes were then ruptured in supplemented culture media with forceps. For GSH, insulin and GSH+insulin treated cultures, the released cysts were dissociated by gently pipetting up and down several times. The dissociated cysts were then transferred, using a pipette, into a sterile 24-well culture plate with fresh culture media under the laminar flow hood. Each well contained 950 µl of supplemented M3 media and 50 µl of cysts. For BSO treated cultures, the released cysts were allowed to dissociate on their own (without pipetting up and down) by incubating at room temperature in culture medium for 30 minutes prior to transfer to the culture plate. All cyst cultures were incubated at room temperature (22°C) without CO2 for 96 hours. After 48 hours in culture, 200 µl of supplemented media was added to each culture. A total of three experiments each were performed for GSH-only, insulinonly, GSH+insulin and BSO cultures. Cultured cysts were monitored daily and images were collected using an inverted Leica DMIL microscope equipped with a Leica DFC 300FX cooled CCD camera.
Phase contrast and brightfield imaging of whole testes.
Whole testes were dissected from pupa in 1x PBS and mounted under a coverslip using paper ring spacer. The testes in were imaged using a 10x phase contrast objective and by collecting six overlapping images. The images were made into a panoramic montage using the Photomerge function in Photoshop (CS5). The testis image in was produced by collecting brightfield images from three successive focal depths. A three-dimensional montage was created using the Do Stack function in CombineZP (http://www.hadleyweb.pwp.blueyonder.co.uk/).
Quantification of cyst survival, degeneration and statistical analysis.
Cysts were quantified by manually counting viable cysts present at each stage of development every 24 hours over the course of 96 hours in culture. Viable cysts were defined as those that had normal morphology and lacked any kind of degeneration such as bursting of cysts or internal degradation of spermatogenic cells. The cysts were categorized in developmental stages based on the number of cells per cyst, morphology and size of the cysts (see ). The survival rates of the cysts were quantified based on how many viable cysts were present in culture per experiment each day. The duration of manual counting of cysts for quantification for treated and untreated cultures was approximately thirty minutes. This time was kept as short as possible to minimize the exposure of cysts to light. Statistical significance was determined using unpaired, one-tailed Student's t-test (GraphPad Prism). Data for each treatment condition were also pooled and analyzed using Tableau (Tableau Software). We computed the ratios of the pooled percentages of spermatogonia and primary spermatocytes for the different treatments versus the pooled data for the untreated controls. The ratios are normalized to 1 for Day 0 (initiation of culture). Degeneration counts for primary spermatocytes were calculated by determining the total number of lost PS cysts over the course of each 24 hour period, then subtracting the increase in secondary spermatocyte and round spermatid (SS+RS) cysts. This calculation was performed for primary spermatocytes only because an increase in the number of cysts at the next stage of development (SS+RS) was observed (at least at early timepoints), indicating that the change in PS number was due to both degeneration and differentiation at some timepoints.
Hoechst staining of cultured cysts.
Cysts were gently removed from the culture plate with a pipet and deposited into a well on a coverslip. Hoechst 33342 (Sigma) was added to a final concentration of 5 µg/ml and the preparations were imaged on an Olympus FV1000 confocal laser scanning microscope.
Figures and Tables
Figure 1 Spermatogenesis in D. pseudoobscura pupal testes. (A) Paired ellipsoid testes (T), seminal vesicles (SV) and accessory glands (AG). The stem cell niche is assumed to be in the apex (A) of the D. pseudoobscura testis based on what is known about the placement of the stem cell niche in D. melanogaster. Sperm coiling occurs in the basal end (B) and mature sperm are stored in the seminal vesicle in adults. Phase contrast image. Bar = 100 µm. (B) Single testis showing enclosed cysts. Arrow indicates elongating cysts (E) which span almost the entire length of the testis as they mature. Brightfield image. Bar = 100 µm. (C) Diagram of spermatogenic cyst maturation in D. pseudoobscura. H, hub cell; CySC, cyst stem cell or cyst progenitor cell; GSC, germline stem cell, G, gonialblast; PS, primary spermatocyte; SS, secondary spermatocyte, RS, round spermatid. The number of spermatogenic cells encapsulated by cyst cells is indicated in parentheses.
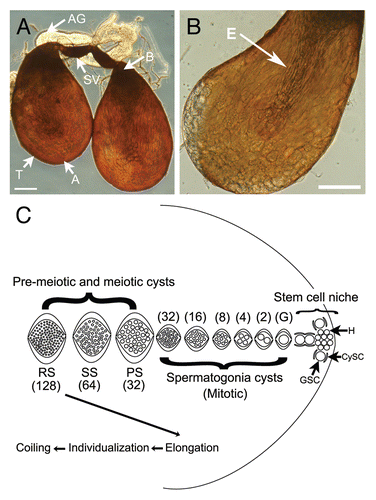
Figure 2 Brightfield images showing spermatogenic cysts after 72 hours in GSH-treated culture. (A) Overall view showing most stages of spermatogenic cyst development. (B) Individualizing cysts with cystic bulges (arrow). (C) Coiling cysts (arrow). Bars = 100 µm. SG, spermatogonia; PS, primary spermatocyte; SS/RS, secondary spermatocyte/round spermatid; E, elongating; IC, individualizing cyst with cystic bulge; CC, coiling cyst; D, degenerating cyst. SS/RS were scored together as it was often difficult to distinguish between them.
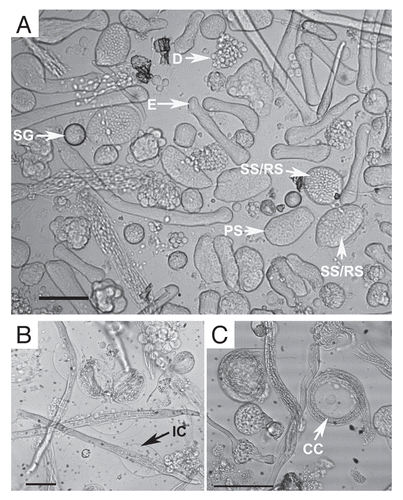
Figure 3 Percentages of each cyst type in pooled data for each treatment type vs. untreated controls. (A) GSH-only, (B) Insulin-only, (C) GSH+insulin, (D) BSO. For each timepoint, the control data bar is on the left and the experimental bar is on the right.
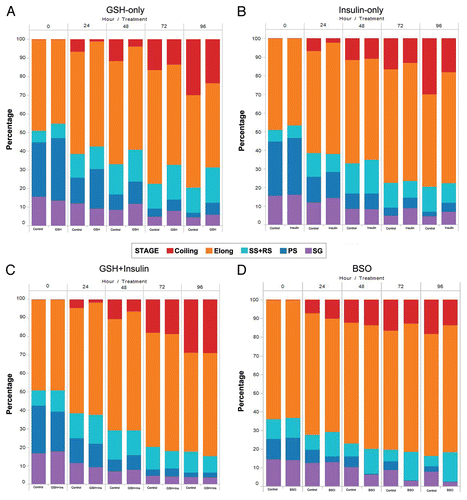
Figure 4 Line graphs showing the change in the raw number of each cyst type for treated and control cultures from the initiation of culture to 96 hours. (A) GSH-only, (B) Insulin-only, (C) GSH-Insulin, (D) GSH/Insulin control, (E) BSO, (F) BSO control.
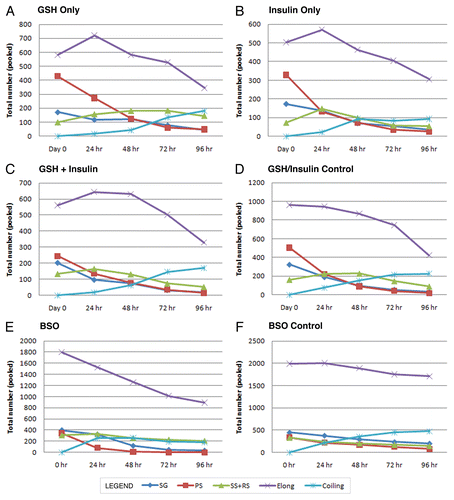
Figure 5 Degeneration counts for primary spermatocytes (PS) in GSH, insulin and GSH+insulin treated cultures. Statistically significant differences are indicated by an asterisk (*). Bars are means ± SEM. *p < 0.05 (Student's t-test). Degeneneration was calculated by determining the total number of lost PS cysts over the course of each 24 hour period then subtracting the increase in secondary and round spermatid cysts. (A) 0 to 24 hours, (B) 24 to 48 hours, (C) 48 to 72 hours, (D) 72 to 96 hours.
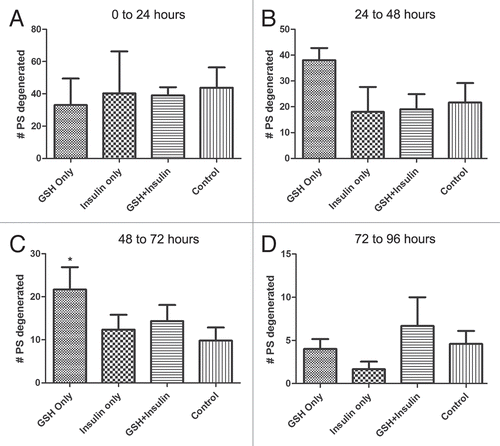
Figure 6 Performance factors for spermatogonia and primary spermatocyte cysts over 96 hours in culture. The ratios are normalized to start at 1 for Day 0 (initiation of culture). Top parts are spermatogonia cysts; bottom parts are primary spermatocyte cysts.
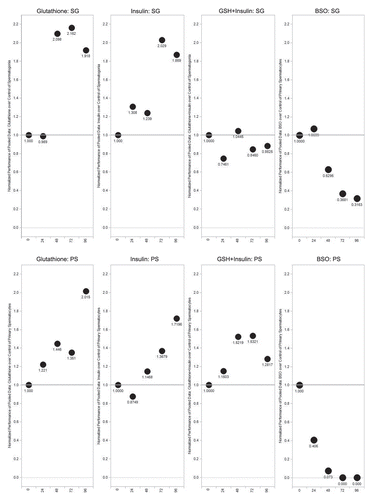
Figure 7 Percentages of spermatogonia and primary spermatocytes in culture vs. untreated controls over 96 hours. Statistically significant differences are indicated by an asterisk (*). Bars are means ± SEM. *p < 0.05 (Student's t-test). (A) GSH-only, (B) Insulin-only. n = 3 for each treatment; n = 5 for untreated controls. (C) GSH+Insulin, (D) BSO. n = 3 for each treatment; n = 5 for untreated controls.
Figure 8 Percentages of early spermatogenic cysts (spermatogonia (SG) + primary spermatocyte (PS)) cysts present over 96 hours in treated and untreated cultures. SG and PS percentages were pooled for GSH, Insulin and GSH+Insulin. (A) Initiation of culture, (B) 24 hours, (C) 48 hours, (D) 72 hours, (E) 96 hours. *p < 0.05 (Student's t-test). Means ± SEM. n = 3 for each treatment; n = 5 for untreated controls.
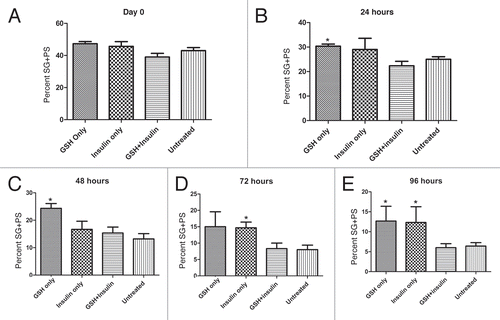
Figure 9 Brightfield images of cultured cysts with fluorescence signal from nuclei (arrows) overlaid. (A) Examples of normal elongating cysts after 24 hours in GSH-treated culture. (B–E) abnormal cysts present after 72 hours in BSO-treated cultures. Several different types of abnormally elongating cysts were observed. In some cases, the nuclei appeared to begin elongation, but became disorganized (large arrows, B and C), while the elongation of the tail portion of the cysts was retarded. Some cysts at the pre-elongation, round spermatid stage also exhibited disorganized nuclei (small arrow, C). (D and E) show cysts with normally elongating nuclei (arrows), but abnormally developed tail regions of the cysts.
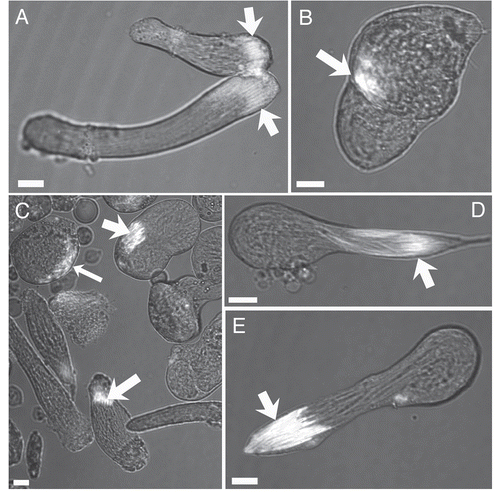
Table 1 Changes in the raw numbers of cysts after 24 hours (0 → 24), 48 (24 → 48), 72 (48 → 72) and 96 (72 → 96) hours
Acknowledgments
We gratefully acknowledge Allan Blake, Carolyn Bentivegna, Heping Zhou, Monicah Njogu, Crystal Pristell, Zain Alvi and Vikram Basava (SHU) for many helpful discussions, as well as two anonymous reviewers for insightful comments on the manuscript. We gratefully thank Jennifer Goonetilleke and Juan Franco (SHU) for the testes dissections in and B. We thank the SHU Department of Biological Sciences for funding this work.
References
- White-Cooper H. Studying how flies make sperm-investigating gene function in Drosophila testes. Mol Cell Endocr 2009; 306:367 - 373
- White-Cooper H. Molecular mechanisms of gene regulation during Drosophila spermatogenesis. Reproduction 2010; 139:11 - 21
- Le Bras S, Van Doren M. Development of the male germline stem cell niche in Drosophila. Dev Biol 2006; 294:92 - 103
- Leatherman JL, DiNardo S. Germline self-renewal requires cyst stem cells, while stat regulates niche adhesion in Drosophila testes. Nat Cell Biol 2010; 12:806 - 811
- Fuller MT. Bate M, Martinez-Arias A. Spermatogenesis. The Development of Drosophila 1993; New York Cold Spring Harbor Press 71 - 147
- Kiger AA, Jones DL, Schulz C, Rogers MB, Fuller MT. Stem cell self-renewal specified by JAK-STAT activation in response to a support cell cue. Science 2001; 294:2542 - 2545
- Tulina N, Matunis E. Control of stem cell self-renewal in Drosophila spermatogenesis by JAK-STAK signaling. Science 2001; 294:2546 - 2549
- Singh SR, Zheng Z, Wang H, Oh S, Chen X, Hou SX. Competitiveness for the niche and mutual dependence of the germline and somatic stem cells in the Drosophila testis are regulated by the JAK/STAT signaling. J Cell Physiol 2010; 223:500 - 510
- Hardy KW, Tokuyasu KT, Lindsley DL, Garavito M. The germinal proliferation center in the testis of Drosophila melanogaster. J Ultrastruct Res 1979; 69:180 - 190
- Cheng J, Tivaboonchai A, Yamashita YM, Hunt AJ. Asymmetric division of cyst stem cells in Drosophila testis is ensured by anaphase spindle repositioning. Development 2011; 138:831 - 837
- Boutananaev AM, Mikhaylova LM, Nurminsky DI. Upregulation of the Ku heterodimer in Drosophila testicular cyst cells. FEBS Lett 2007; 581:1707 - 1715
- Sharer L, Da Lage J, Joly D. Evolution of testicular architecture in the Drosophilidae: A role for sperm length. BMC Evol Biol 2008; 8:143 - 152
- Fuller MT. Genetic control of cell proliferation and differentiation in Drosophila spermatogenesis. Sem Cell Devel Biol 1998; 9:433 - 444
- Tokuyasu KT. Dynamics of spermiogenesis in Drosophila melanogaster. VI. Significance of “onion” nebenkern formation. J Ultrastruct Res 1975; 53:93 - 112
- Tokuyasu KT. Dynamics of spermiogenesis in Drosophila melanogaster. IV. Nuclear transformation. J Ultrastruct Res 1974; 48:284 - 303
- Tokuyasu KT. Dynamics of spermiogenesis in Drosophila melanogaster. I. Individualization process. Z Zellforsch Mikrosk Anat 1972; 124:479 - 506
- Noguchi T, Miller KG. A role for actin dynamics in individualization during spermatogenesis in Drosophila melanogaster. Development 2003; 130:1805 - 1816
- Njogu M, Ricketts P, Klaus AV. Spermatogenic cyst and organ culture in Drosophila pseudoobscura. Cell Tissue Res 2010; 341:453 - 464
- Niki Y, Yamaguchi T, Mahowald AP. Establishment of stable cell lines of Drosophila germ-line stem cells. Proc Nat Acad Sci 2006; 103:16325 - 16330
- Kawamoto T, Kawai K, Kodama T, Yokokura T, Niki Y. Autonomous differentiation of Drosophila spermatogonia in vitro. Devel Growth Differ 2008; 50:623 - 632
- Fernandez R, Tabarini D, Azpiazu N, Frasch M, Schlessinger J. The Drosophila insulin receptor homolog: A gene essential for embryonic development encodes two receptor isoforms with different signaling potential. EMBO J 1995; 14:3373 - 3384
- Ueishi S, Shimizu H, Inoue Y. Male germline stem cell division and spermatocyte growth require insulin signaling in Drosophila. Cell Struct Func 2009; 34:61 - 69
- Anderson ME. Gluthione: an overview of biosynthesis and modulation. Chem-Biol Interact 1998; 112:1 - 14
- Blokhina O, Birolainen E, Fagerstedt V. Antioxidants, oxidative damage and oxygen deprivation stress: a review. Ann Botany 2003; 91:179 - 194
- Allocati N, Federici L, Masulli M, Di Ilio C. Glutathione transferases in bacterial. FEBS J 2009; 276:58 - 75
- Koharyova M, Kollarova M. Oxidative stress and thioredoxin system. Gen Physiol Biophys 2008; 27:71 - 84
- Kanzok SM, Fechner A, Bauer H, Ulschmid JK, Muller H, Botella-Munoz J, et al. Substitution of the thioredoxin system for glutathione reductase in Drosophila melanogaster. Science 2001; 291:642 - 646
- Missirlis F, Phillips JP, Jackle H. Cooperative action of antioxidant defense systems in Drosophila. Current Biology 2001; 11:1272 - 1277
- Luberda Z. The role of glutathione in mammalian gametes. Reprod Biol 2005; 5:6 - 17
- Saunders RDC, McLellan LI. Molecular cloning of Drosophila gamma-glutamylcysteine synthetase by functional complementation of a yeast mutant. FEBS Lett 2000; 467:337 - 340
- Fraser JA, Saunders RDC, McLellan LI. Drosophila melanogaster glutamate-cysteine ligase activity is regulated by a modifier subunit with a mechanism of action similar to that of the mammalian form. J Biol Chem 2002; 277:1158 - 1165
- Cross DP, Shellenbarger DL. The dynamics of Drosophila melanogaster spermatogenesis in in vitro cultures. J Embryol Exp Morphol 1979; 53:345 - 351
- Davidson BE, Hird FJB. The reactivity of the disulphide bonds of purified proteins in relationship to primary structure. Biochem J 1967; 104:473 - 479
- Gronke S, Clarke DF, Broughton S, Andrews TD, Partridge L. Molecular evolution and functional characterization of Drosophila insulin-like peptides. PLoS Genet 2010; 6:1000857; http://dx.doi.org/10.1371/journal.pgen.1000857
- Liebrich W. In vitro spermatogenesis in Drosophila I. Development of isolated spermatocyte cysts from wild-type Drosophila hydei. Cell Tissue Res 1981; 220:251 - 262
- Fowler G, Johannisson R. Kurstak E, Maramorosch K. Single-cyst in vitro spermatogenesis in Drosophila hydei. Invertebrate Tissue Culture, Applications in Medicine, Biology and Agriculture 1976; New York Academic Press 161 - 172
- Bianco A, Poukkula M, Cliffe A, Mathieu J, Luque CM, Fulga TA, Rorth P. Two distinct modes of guidance signalling during collective migration of border cells. Nature 2007; 448:362 - 366