Abstract
The generation of functional sperm in vitro has been a goal for almost a century. Until recently, researchers have only succeeded in reproducing the early steps of spermatogenesis. This is not surprising given that spermatogenesis is a complicated process that requires the coordinated efforts of germ cells and several somatic cells within the tubular structure of the testis. Finally—last year—Sato et al. reported the successful in vitro production of functional sperm, thereby potentially opening up a new era of reproductive biology. Here, we summarize the history of research directed toward reproducing steps of spermatogenesis in vitro, detail the seminal findings of Sato et al., and suggest ways that their approach can be applied toward clinical applications and addressing fundamental questions about the underlying mechanism of spermatogenesis.
Introduction
To become a functional sperm, an immature male germ cell undergoes a coordinated set of events that are collectively called spermatogenesis. This process occurs in tubules within the testis that harbor what is known as the seminiferous epithelium. The heart of the seminiferous epithelium is a somatic cell—the Sertoli cell—that is in intimate contact with all of the germ cells in the seminiferous epithelium, regardless of whether they are undergoing mitosis, meiosis, or maturation. Sertoli cells provide essential factors for spermatogenesis and evidence suggests that they communicate back and forth with germ cells to drive spermatogenesis. Other somatic cells also participate in spermatogenesis, including the peritubular myoid cells, which help form the seminiferous tubule structure, and the testosterone-producing Leydig cells, which are located in the interstitial area between tubules.Citation1
Given that spermatogenesis normally occurs in seminiferous tubules and that many somatic cell types participate in spermatogenesis, spermatogenesis has been difficult to reproduce in vitro. Nevertheless, this has not prevented researchers from trying! Indeed, as we summarize in this commentary, there have been scores of studies attempting to achieve this goal. Driving these attempts have been the large rewards. For example, if a robust and efficient in vitro spermatogenesis procedure could be created, this would have potential clinical applications for treating some cases of infertility and it could also greatly speed up the efforts to decipher the cellular and molecular mechanisms underlying spermatogenesis. Given these potential pay-offs, there was much fanfare when Sato et al. recently reported the first success in generating sperm from immature germ cells in vitro.Citation2 Using an organ culture method that relies on defined medium containing a putative spermatogenesis-promoting factor that also maintains embryonic stem (ES) cells, Sato et al. were able to generate elongated spermatids that were capable of fertilizing eggs and generating progeny. Here, we discuss the findings of Sato et al. and suggest basic science and clinical applications for their technique.
A Long Journey to Achieve in Vitro Spermatogenesis
Organ culture
Since the first report—in 1920—providing evidence for limited differentiation of male germ cells when cultured in vitro,Citation3 there have been many attempts to come up with ways to drive further differentiation of germ cells. For example, in 1937, it was reported that a clot composed of fowl plasma and embryo extract permitted some of the mitotically active spermatogonia within cultured newborn mouse testes to progress to the pachytene stage.Citation4 In the 1960s, the Steinberger group identified several measures that promoted long-term survival of cultured testes, including the addition of pyruvate to the culture medium as an energy source, reducing the incubation temperature to 31°C to better simulate the intratesticular temperature in vivo, and using a gas-liquid interphase method to increase oxygen exchange.Citation5 Using tritiated-thymidine to tag immature proliferating male germ cells (spermatogonia and preleptotene spermatocytes) present in rat testes explants, they demonstrated that some of these cells differentiated into pachytene spermatocytes after culture in vitro for 2 to 3 weeks.Citation6 They also achieved similar results using human testicular biopsies.Citation7 While encouraging, no germ cells beyond the pachytene stage of meiosis were observed by either Steinberger and colleagues or most other research groups that attempted to improve culture conditions during this early phase of the “in vitro spermatogenesis” field. An exception was one group that reported conditions that allowed tritiated thymidine-labeled human preleptotene spermatocytes to differentiate into spermatocytes that progressed to the end of meiosis: the telophase-II stage. In their protocol, testicular biopsies were cultured for 14 d in media containing deproteinized coconut milk, follicle-stimulating hormone (FSH) and luteinizing hormone (LH).Citation8 While potentially promising, a concern with regard to physiological relevance is that these investigators found that the speed of conversion of the pre-leptotene germ cells to telophase-II germ cells in vitro was more rapid than normally occurs in vivo.Citation9 A further concern is that only one out of 16 testicular biopsies were able to give rise to male germ cells that progressed to the telophase-II stage, thereby casting some doubt on the reproducibility of their culturing method.
Culture of dissociated cells
Starting in the 1980s, there was a burst in publications attempting to reproduce spermatogenesis using dissociated testicular cells rather than organ culture. For example, it was reported that co-culture of immature rat germ cells with primary Sertoli cells for 11 d promoted their maturation into late pachytene spermatocytes.Citation10 In the 1990s, there were two reports providing evidence that immature germ cells could be pushed all the way to the haploid stage. These studies,Citation11,Citation12 which revolved around using immortalized Sertoli cell or germ cell lines, respectively, held great promise, but, to our knowledge, no follow-up studies using these techniques have been published. Later—in 2002— it was reported that immortalized mouse type-A spermatogonial cells in the presence of stem cell factor (SCF) are capable of undergoing differentiation into haploid spermatids in vitro.Citation13 However, no fully differentiated germ cells (e.g., tailed spermatozoa) were observed, it was not tested whether the round spermatids that are generated are functional competent for fertilization, and no follow-up studies have been subsequently published. In the human system, a study reported that dissociated cells from testicular biopsy samples from humans with premeiotic maturation arrest at the primary spermagocytes stage could be coaxed into becoming haploid germ cells after culture for 48 h.Citation14 Remarkably, the elongating spermatids generated in these in vitro cultures were functional competent, as oocytes injected with them were shown to form healthy babies when transplanted into hormonally receptive human females.Citation14 While promising, whether this approach could be safely used in the clinic is not clear since the time frame these investigators found was sufficient for differentiation of human meiotic germ cells into elongating spermatids in vitro was abnormally short (24–48 h); this multi-step process normally takes 20–22 d in vivo.Citation15
More recently, approaches have been identified that drive the differentiation of mature male germ cells from stem cells. In one approach, investigators found that culturing mouse spermatogonial stem cells (SSCs) with glial cell line-derived neurotrophic factor (GDNF) allowed the SSCs to grow for a long period of time and give rise to functional sperm when transplanted into germ cell-depleted testes of wild-type mice.Citation16,Citation17 This was a major breakthrough, as the transplanted mice were able to father progeny by natural conception. Another group reported that SSCs can be differentiated into early meiotic germ cells by simply culturing the SSCs in retinoic acid (RA).Citation18 It will be interesting to determine whether RA in combination with appropriate culture conditions can drive later steps of male germ cell differentiation. Other studies have reported the successful generation of haploid germ cells from human and mouse embryonic stem (ES) cells.Citation19,Citation20 One of these studies demonstrated that haploid cells generated from the mouse ES cells were capable of triggering blastocyst formation when injected into the cytoplasm of oocytes.Citation19 However, whether these blastocysts can generate progeny when injected into hormonally receptive female mice has not yet been reported.
In summary, the concerted efforts of many investigators over several decades has led to the development of culture conditions for promoting specific steps in spermatogenesis in vitro. However, the ultimate goal of generating functional sperm in vitro was not accomplished until, as described below, the Ogawa group revisited the old organ culture method using newly developed stem cell culture techniques and a media supplement that they discovered is essential for spermatid maturation.
Complete spermatogenesis in vitro
As a precursor to their groundbreaking work, the Ogawa group identified conditions for culturing mouse testes fragments from neonatal mice that allowed some of the male germ cells in them to undergo a significant degree of maturation.Citation21 To assess maturation, they made the testes cultures from transgenic mice that express green fluorescent protein (GFP) from either a mid-meiosis promoter or a late-meiosis promoter. This allowed them to detect even small numbers of cells that progressed to specific stages of meiosis. Using this and other methods, they detected some cells in the cultured testes chunks that completed meiosis and progressed to the round spermatid stage.
While a significant advance, Ogawa and coworkers were not able to detect any germ cells in the testes chunks that progressed past the round spermatid stage.Citation21 They considered the possibility that the fetal bovine serum in the culture medium was not supportive for this final stage of male germ cell maturation and thus they screened for other tissue culture supplements that might have such activity. This screen revealed that a supplement normally used to maintain ES cells—knock out serum replacement (KSR) media—was able to promote the maturation of male germ cells in cultured testes fragments to the elongated spermatid stage.Citation2 At least some of these elongated spermatids were functionally competent, as judged using intracytoplasmic sperm injection (ICSI), a method that involves injection of elongated spermatids or spermatozoa into mature oocytes, followed by in vitro culture of the fertilized oocytes to form two-cell embryos, and finally transfer of the embryos into the oviducts of pseudopregnant females. Importantly, Ogawa and colleagues found that the females implanted with these in vitro-generated spermatozoa-derived embryos delivered live and fertile offspring. Even the less differentiated spermatids generated in their organ culture system were capable of generating progeny, as they showed using a round spermatid injection (ROSI) technique. These investigators also tested whether their technique could be applied to a practical application: cryoprervation. In particular, they examined whether their system would support the proliferation and differentiation of germ cells present in cryopreserved neonatal testes tissues. Indeed, they found that when thawed and cultured in KSR-containing medium, some immature germ cells in frozen testes samples proliferated and formed elongated spermatids.
In follow-up work, Ogawa and colleagues tested whether they could use their technique to differentiate mature germ cells from SSCs.Citation22 They tested this by transplanting SSCs into the seminiferous tubules of germ cell-depleted testes (made germ cell deficient by either genetic or chemical means), followed by culture of fragments from these testes in the KSR-containing medium that they had shown promotes spermatogenesis in their earlier study.Citation2 Remarkably, they found that, under these conditions, the transplanted SSCs migrated into the basement membrane after only ~3 d of culture, began proliferating a few days later, and then, after almost two months, some of the cells differentiated into elongated spermatids. Some of the haploid spermatids were functionally competent, as assessed by ROSI. These investigators also performed a proof-of-principal study in which they asked whether their in vitro culture system was capable of correcting spermatogenic failure.Citation22 As a model system, they used steel mutant mice, which contain SSCs but lack later stage male germ cells because of a mutation in the gene encoding stem cell factor, a Sertoli-cell product essential for the proliferation and differentiation of spermatogonial cells. To determine whether they could drive the differentiation of SSCs from steel mutant testes using their organ culture system, they transplanted them into germ cell-deficient testes. They found that the transplanted SSCs could be successfully differentiated into haploid germ cells in their in vitro system. This result complemented an earlier study from Brinster and colleagues, which demonstrated restoration of spermatogenesis when SSCs from steel mutant testes are transplanted into germ cell-deficient testes in vivo.Citation23 Together, this earlier studyCitation23 and the more recent study from Ogawa and colleagues,Citation22 provide two independent paths—one in vitro and one in vivo—for assisted reproduction in large host animals and humans.
While the identification of culture conditions that permit immature male germ cells to mature into functional sperm is a major breakthrough, there are still several issues that require attention. Not surprisingly, efficiency is a major concern. Far fewer elongated spermatids and spermatozoa are produced per input immature male germ cell using the currently available in vitro systemCitation2 than in a normal testis in vivo. Ogawa and colleagues observed flagellated sperm in only 5 out of 11 cultured explants from neonatal testesCitation2 and in only 3 out of 17 cultured explants from SSC-transplanted testes.Citation22 Only the peripheral region of testes fragments exhibited advanced spermatogenesis in their system, while the center lost normal morphology and had large numbers of degenerating cells after 40 d of culture.Citation2 Furthermore, they found a gradual decrease in germ cell numbers over the 40-d culture period.Citation2 Thus, creative approaches to improve germ cell viability are required, such as the addition of an “in vitro vascular” system. Given that human application will typically require using testes from adolescent or adult individuals, a practical concern is whether explants from adult testes can maintain spermatogenesis using their culture conditions. In their mouse experiments, Ogawa and colleagues used testes from neonatal miceCitation2,Citation21 or postnatal (3-week-old) mice in the case of SSC-transplanted testes.Citation22 Another issue for human application is whether the spermatids generated from cryopreserved testes are functionally competent. In their mouse studies, Ogawa and colleagues did not test whether explants from cryopreserved testes can gave rise to functional spermatids, as judged, for example, by ISCI or ROSI.Citation2 In summary, the in vitro spermatogenesis approach described herein shows much promise. With improvements in efficiency and germ cell viability, along with altered conditions to permit usage of mature testes as input material, their technique is likely to be useful for a variety of basic science and clinical applications, as described below.
Future Applications
An efficient system for studying spermatogenesis
One of the technical challenges holding back our understanding of spermatogenesis has been the lack of an in vitro system that recapitulates spermatogenesis. Because of this deficit, many researchers have been forced to study mechanisms of spermatogenesis using in vivo systems. For example, to identify the role of specific genes in spermatogenesis, it has been necessary to generate genetically modified mice harboring targeted mutations in specific genes. While this is an essential approach to understand the role of genes in vivo, it is extremely time consuming and expensive. Furthermore, it is often difficult to use such mouse models to identify molecular mechanisms. Another approach to study spermatogenesis has been to use immortalized cell lines, but the number of cell lines available for such studies is limited—particularly in the case of germ cells—and how well such cell lines recapitulate events in normal cells is uncertain at best. Use of primary cells solves some of these problems, but they are not trivial to prepare or maintain. Furthermore, the culture conditions required for fully recapitulating spermatogenesis with primary cells have not been identified, nor is it clear whether testicular cells—even if freshly isolated—placed in isolation on a plastic surface act in a physiologically normal manner.
The ability to recapitulate spermatogenesis in organ cultureCitation2 solves many of these problems, but it also brings up new challenges. One challenge is how to detect germ cell maturation in an in vitro organ culture system. One approach to assay germ cell development is to use a battery of reporters (expressing different versions of GFP) under the control of promoters that drive gene expression in different stages of germ cells. This advancement, coupled with live organ imaging technology using multiphoton fluorescence microscopy, would allow germ cell development to be visualized in real time (Fig. 1). Another challenge is to work out how to efficiently deliver vectors into the cells in organ culture in order to manipulate the expression of genes of interest and thereby address their functional role. Two delivery approaches are electroporation and viral infection. Electroporation has been successfully used to introduce genes into the testis in vivo,Citation24 but it causes extensive tissue damage, limiting its use for many applications. Also, electroporation typically only delivers genes transiently, so it is not generally suitable for determining functions occurring over long periods of time. Lentiviruses have the intrinsic advantage of being able to integrate into the genome and thus infection of cells with lentiviruses can provide long-term gene expression. Indeed, lentiviruses have been used to successfully express genes for long periods of time in cells in the seminiferous tubule in vivo.Citation25,Citation26 However, a potential drawback of lentiviruses is that they may not be able to efficiently infect most of the cells in organ culture. Indeed, one study found that lentiviruses infect only Sertoil cells, not germ cells, when injected into seminiferous tubules.Citation25 In contrast, a recent report showed that lentiviruses can deliver transgenes into germ cells when injected into seminiferous tubules and that transgene expression in germ cells persisted for up to 3 mo.Citation26 Given the contrasting results of these two studies, the question of whether or not lentiviruses efficiently infect germ cells in testes organ culture is an issue that needs to be revisited. For somatic cell types outside of the seminiferous tubules—peritubular myoid cells and Leydig cells—it is likely that they will be highly susceptible to lentiviral infection since these cells are accessible to the culture media in cultured testes fragments. Thus, lentiviral vectors can probably be effectively used to test the role of genes in these two somatic cell types. In the case of germ cells, an alternative approach is to introduce the vector or small interfering (si) RNA of interest into SSCs, followed by transplantation of these SSCs into testes and then in vitro culture of explants generated from these testes. While this “in vivo-in vitro” approach has not yet been tried, in principal it should be feasible as in vivo transplantation has been used to identify genes involved in SSC self-renewal or differentiation.Citation18,Citation27
How might in vitro approaches be specifically applied to studying spermatogenesis? By coupling in vitro culture approaches with live tissue imaging, the vector/siRNA-expressing cells can be monitored, allowing for earlier detection of defects than might be observed in vivo (Fig. 1). To specifically study the cells in which a given gene has been manipulated in explant cultures, one could use two different reporters. As an example application, a vector that co-expresses a siRNA against the gene of interest and red fluorescent protein (RFP) could be introduced into testes explants from a transgenic mouse containing a transgene that expresses GFP from a promoter active in the germ cell stage of interest. Following delivery of the vector, the testis fragments would be cultured as describedCitation2 and monitored for GFP and RFP expression. If, for example, knockdown of the gene of interest by the siRNA causes a defect in progression to the stage monitored by GFP, there will be less RFP+/GFP+ double-positive cells compared with testes fragments delivered negative-control siRNAs. If cell type- or stage-specific expression of the siRNA is required, this can be achieved by using the appropriate promoter to drive its expression.Citation28
Not only is it likely to be much easier to examine the role of genes using in vitro spermatogenesis procedures than generating and characterizing knockout mice, but in vitro procedures may provide opportunities that are not readily feasible with the latter. For example, analysis of the redundant role of members of clustered gene families cannot be easily accomplished using a standard knockout approach. This is because the recombination frequencies of closely linked genes are too low to permit feasible generation of double knockout progeny mice from single knockout mice. In contrast, by introducing multiple siRNAs in testes explants, one can relatively easily examine the independent and redundant roles of gene clusters. Another advantage is that one could feasibly perform genome-wide screening for factors involved in spermatogenesis using siRNA libraries ().
Human spermatogenesis in vitro
Studying mouse spermatogenesis will no doubt provide many insights into human spermatogenesis, but ultimately, for the purposes of human health, it will be crucial to develop systems to decipher precisely how this process occurs in humans. This need is underscored by the extremely high rate of evolution of the male reproductive tract and the genes that function in male reproduction.Citation29,Citation30 This high rate of evolution casts a doubt on the mouse being an appropriate model for many aspects of human spermatogenesis.36 There are both ethical and practical concerns with studying spermatogenesis in humans in vivo and thus it is essential to instead develop in vitro approaches to study this process. In principal, the procedure developed by Ogawa and colleaguesCitation2 could be applied to human testis biopsies to achieve this goal. However, it is currently unknown whether the culturing conditions that permit complete spermatogenesis to occur in mice explants can be applied to human explants. It might be more challenging to accomplish this goal with human explants, as human spermatogenesis takes much longer in vivo (~64 d) than does mouse spermatogenesis (~35 d).Citation9 Yet, if it can be accomplished, such a human testes culture system could then be used to examine the functional role of genes suspected to be involved in human spermatogenesis by the approaches described above for the mouse system ().
Infertility treatment
In vitro spermatogenesis procedures have several potential applications in the clinic, including: (1) restoration of fertility in juvenile patients requiring chemotherapy, (2) generation of functional sperm from induced pluripotent stem (iPS) cells, and (3) gene therapy approaches to generate fertile sperm from infertile men.
In the first application, the goal is to provide juvenile cancer patients who have undergone high-dose chemotherapy with a source of functional spermatids when they are ready to father children. The number of long-term survivors of childhood cancer has increased greatly in recent years, highlighting the need for identifying methods to recover the fertility of these patients. One means to achieve this is to isolate SSCs from these patients prior to chemotherapy and then use them for “auto-transplantation” in vivo (). This seems feasible given the recent success of long-term culture of human SSCs from cryopreserved testis biopsy.Citation31 To judge the spermatogenic potential of such SSC cells, they can be transplanted into testicular explant biopsies cultured in vitro using the culture method developed by the Ogawa group (). A challenge with the “SSC approach” is to reduce its inherent risks. For example, SSCs have the potential to become pluripotent stem cells that generate teratomas after transplantation.Citation32 It is possible that this risk can be judged by injecting the SSCs into testes explants and assaying for tumors, but whether the latter support tumor growth remains to be seen. An alternative approach is to skip the SSC isolation step and instead directly use testes biopsies from patients for in vitro culture. This requires that testes explant culture conditions are discovered to support complete human spermatogenesis, which may not be trivial to achieve given that human spermatogenesis requires more time than mouse spermatogenesis, at least in vivo.Citation9 Moreover, it may be necessary—in the case of very young individuals—to provide external endocrine signals to trigger spermatogenesis in cryopreserved juvenile testis biopsies since human spermatogenesis typically does not initiate until after 10 y of age. If indeed human spermatogenesis can be safely reproduced in vitro, then in principal it should be possible to use either the round or elongated spermatids generated from cryopreserved juvenile testis biopsies to successfully fertilize eggs by either ISCI or ROSI, respectively ().
Figure 2. Potential clinical applications of the in vitro testis culture system. Blue arrows indicate germ cell transplantation in vivo or in vitro. SSCs, spermatogonial stem cells; iPS cells, induced pluripotent stem cells; PGCs, primordial germ cells; IVF, in vitro fertilization.
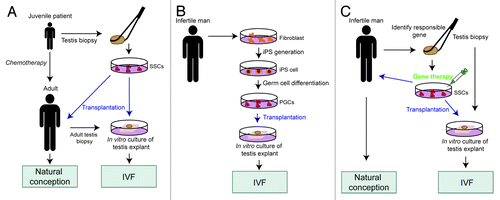
The discovery of methods to generate ES-like cells from fully differentiated cells—iPS cellsCitation33,Citation34—has opened up the possibility of another means to generate functional spermatids in vitro. The beauty of using iPS cells is that no testicular tissues from the donor are required. In the way we envisage this approach, iPS cells generated from the individual would first be coaxed down the early male germ cell lineage using in vitro methods that have already been developed for human ES cells.Citation20,Citation33 Following purification of the male germ cells that have partially differentiated in vitro from the iPS cells, they would be pushed further toward final maturity by transplanting them into testicular explants from another individual. This latter step—which would involve in vitro culture of testicular explants by the methods described herein by Ogawa and colleaguesCitation2—is necessary because current in vitro methods for differentiating male germ cells from human pluripotent cells are extremely inefficient and generate few cells that progress beyond the haploid stage.Citation20,Citation33 Following in vitro culture of the explants, the mature germ cells would be isolated and used to fertilize human eggs by ICSI or ROSI (Fig. 2B). While a potentially powerful method for certain clinical applications, it should be stressed that this iPS/testes explant approach is fanciful at present. Even though conditions for differentiating human ES and iPS cells down the haploid male germ-cell lineage have been established,Citation20,Citation33 the functionality of the differentiated spermatids produced from these cultures has never been tested. Another potential challenge is that the recipient heterologous testes explants for the donor iPS-derived male germ cells would need to be treated to kill the progenitor germ cells in them so that only the donor germ cells provided would progress to maturity. While methods to do this have been established (e.g., using busulfan), this step adds another layer of complexity to this approach. Despite these concerns, this iPS/testes explant approach is worth attempting to establish, as it has the potential to fill niches that are currently empty in assisted reproduction practice. For example, this approach would allow cancer patients given high-dose chemotherapy the ability to father children even if they did not bank frozen testicular biopsies prior to chemotherapy treatment ().
Finally, we propose that the in vitro spermatogenesis procedure could be used for personalized fertility therapy. For example, if an infertile male had a known mutation in germ cells that was responsible for his infertility, this mutation could be corrected in male germ cell progenitors in vitro, followed by their expansion and differentiation in testes explants so that spermatids could be harvested for in vitro fertilization procedures (). Optimally, a targeting vector would be used to correct the mutation in the endogenous gene. This would involve selecting for rare homologous recombinants in which the wild-type sequences from the targeting vector have replaced the mutant sequences. A technically simpler approach would be to provide a wild-type copy of the gene that randomly integrates into the genome, but this insertion event could lead to a deleterious mutation in another gene and would also irreversibly alter the genome organization of the human germ line. So, we consider an endogenous gene targeting approach the only viable “gene therapy” approach for male germ cells. An advantage of this approach is its affects can be tested in vitro before using it in vivo (). Of course, such an approach will raise ethical concerns given that it involves passing on a manipulated gene to future generations. But as long as the only manipulation is a “positive one” that converts a mutant gene to a wild-type form, one can make a case for its utility outweighing such ethical concerns. In contrast, currently available assisted reproductive technologies are likely to be having the opposite effect. By allowing individual with infertility to have children, these technologies are allowing mutant genes that negatively affect fertility to be passed on to future generations. It is worth noting that there is already precedent for foreign gene transfer through the human germ line. In particular, to improve the efficiency of in vitro fertilization of oocytes from aged women with poor ovarian reserve, these oocytes were injected with the cytoplasm of oocytes from younger women with good ovarian reserve.Citation35 Since the cytoplasm includes the mitochondria and its associated genome, this is an example where a portion of the human genome—the mitochondrial portion—was purposefully altered in future generations. Clearly, there is likely to be a long debate about whether it is appropriate for the betterment of humankind to correct mutations in human male germ cells that are used for fertilization. Since we are getting closer to the point where this will be technically feasible, perhaps it is time to start debating this issue in earnest.
Closing Remarks
After almost a century of effort, we are now in a new phase of male germ cell studies in which all steps of spermatogenesis can be recapitulated in vitro. This has opened up a plethora of new opportunities to better understand the underlying mechanisms of spermatogenesis and to treat male infertility.
Abbreviations: | ||
siRNA | = | small interfering RNA |
ISCI | = | intracytoplasmic sperm injection |
ROSI | = | round spermatid injection |
SSCs | = | spermatogonial stem cells |
iPS cell | = | induced pluripotent stem cell |
IVF | = | in vitro fertilization |
KSR | = | knockout serum replacement |
PGCs | = | primordial germ cells |
Disclosure of Potential Conflicts of Interest
The authors have nothing to declare.
Financial Support
This work was supported by NIH grants R01-HD053808 and R01-HD45595.
References
- Griswold MD. The central role of Sertoli cells in spermatogenesis. Semin Cell Dev Biol 1998; 9:411 - 6; http://dx.doi.org/10.1006/scdb.1998.0203; PMID: 9813187
- Sato T, Katagiri K, Gohbara A, Inoue K, Ogonuki N, Ogura A, et al. In vitro production of functional sperm in cultured neonatal mouse testes. Nature 2011; 471:504 - 7; http://dx.doi.org/10.1038/nature09850; PMID: 21430778
- Champy C. Quelques resultats de la methode de culture des tissus. Arch Zool Exp Gen 1920; 60:461 - 500
- Martinovitch PN. Development in vitro of the mammlian gonad. Nature 1937; 139:413; http://dx.doi.org/10.1038/139413a0
- Steinberger A, Steinberger E, Perloff WH. Mammalian Testes in Organ Culture. Exp Cell Res 1964; 36:19 - 27; http://dx.doi.org/10.1016/0014-4827(64)90156-9; PMID: 14222741
- Steinberger A, Steinberger E. Differentiation of Rat Seminiferous Epithelium in Organ Culture. J Reprod Fertil 1965; 9:243 - 8; http://dx.doi.org/10.1530/jrf.0.0090243; PMID: 14273758
- Steinberger E. Maintenance of adult human testicular tissue in culture. Anat Rec 1967; 157:327 - 8
- Ghatnekar R, Lima-de-faria A, Rubin S, Menander K. Development of juman male meiosis in vitro. Hereditas 1974; 78:265 - 72; http://dx.doi.org/10.1111/j.1601-5223.1974.tb01448.x; PMID: 4463180
- Clermont Y. The cycle of the seminiferous epithelium in man. Am J Anat 1963; 112:35 - 51; http://dx.doi.org/10.1002/aja.1001120103; PMID: 14021715
- Tres LL, Kierszenbaum AL. Viability of rat spermatogenic cells in vitro is facilitated by their coculture with Sertoli cells in serum-free hormone-supplemented medium. Proc Natl Acad Sci USA 1983; 80:3377 - 81; http://dx.doi.org/10.1073/pnas.80.11.3377; PMID: 6407012
- Rassoulzadegan M, Paquis-Flucklinger V, Bertino B, Sage J, Jasin M, Miyagawa K, et al. Transmeiotic differentiation of male germ cells in culture. Cell 1993; 75:997 - 1006; http://dx.doi.org/10.1016/0092-8674(93)90543-Y; PMID: 7504588
- Hofmann MC, Hess RA, Goldberg E, Millán JL. Immortalized germ cells undergo meiosis in vitro. Proc Natl Acad Sci USA 1994; 91:5533 - 7; http://dx.doi.org/10.1073/pnas.91.12.5533; PMID: 8202522
- Feng LX, Chen Y, Dettin L, Pera RA, Herr JC, Goldberg E, et al. Generation and in vitro differentiation of a spermatogonial cell line. Science 2002; 297:392 - 5; http://dx.doi.org/10.1126/science.1073162; PMID: 12077424
- Tesarik J, Bahceci M, Ozcan C, Greco E, Mendoza C. Restoration of fertility by in-vitro spermatogenesis. Lancet 1999; 353:555 - 6; http://dx.doi.org/10.1016/S0140-6736(98)04784-9; PMID: 10028987
- Heller CH, Clermont Y. Kinetics of the Germinal Epithelium in Man. Recent Prog Horm Res 1964; 20:545 - 75; PMID: 14285045
- Kubota H, Avarbock MR, Brinster RL. Growth factors essential for self-renewal and expansion of mouse spermatogonial stem cells. Proc Natl Acad Sci USA 2004; 101:16489 - 94; http://dx.doi.org/10.1073/pnas.0407063101; PMID: 15520394
- Kanatsu-Shinohara M, Miki H, Inoue K, Ogonuki N, Toyokuni S, Ogura A, et al. Long-term culture of mouse male germline stem cells under serum-or feeder-free conditions. Biol Reprod 2005; 72:985 - 91; http://dx.doi.org/10.1095/biolreprod.104.036400; PMID: 15601913
- Dann CT, Alvarado AL, Molyneux LA, Denard BS, Garbers DL, Porteus MH. Spermatogonial stem cell self-renewal requires OCT4, a factor downregulated during retinoic acid-induced differentiation. Stem Cells 2008; 26:2928 - 37; http://dx.doi.org/10.1634/stemcells.2008-0134; PMID: 18719224
- Geijsen N, Horoschak M, Kim K, Gribnau J, Eggan K, Daley GQ. Derivation of embryonic germ cells and male gametes from embryonic stem cells. Nature 2004; 427:148 - 54; http://dx.doi.org/10.1038/nature02247; PMID: 14668819
- Kee K, Angeles VT, Flores M, Nguyen HN, Reijo Pera RA. Human DAZL, DAZ and BOULE genes modulate primordial germ-cell and haploid gamete formation. Nature 2009; 462:222 - 5; http://dx.doi.org/10.1038/nature08562; PMID: 19865085
- Gohbara A, Katagiri K, Sato T, Kubota Y, Kagechika H, Araki Y, et al. In vitro murine spermatogenesis in an organ culture system. Biol Reprod 2010; 83:261 - 7; http://dx.doi.org/10.1095/biolreprod.110.083899; PMID: 20393168
- Sato T, Katagiri K, Yokonishi T, Kubota Y, Inoue K, Ogonuki N, et al. In vitro production of fertile sperm from murine spermatogonial stem cell lines. Nat Commun 2011; 2:472; http://dx.doi.org/10.1038/ncomms1478; PMID: 21915114
- Ogawa T, Dobrinski I, Avarbock MR, Brinster RL. Transplantation of male germ line stem cells restores fertility in infertile mice. Nat Med 2000; 6:29 - 34; http://dx.doi.org/10.1038/71496; PMID: 10613820
- Yomogida K, Yagura Y, Nishimune Y. Electroporated transgene-rescued spermatogenesis in infertile mutant mice with a sertoli cell defect. Biol Reprod 2002; 67:712 - 7; http://dx.doi.org/10.1095/biolreprod.101.001743; PMID: 12193376
- Ikawa M, Tergaonkar V, Ogura A, Ogonuki N, Inoue K, Verma IM. Restoration of spermatogenesis by lentiviral gene transfer: offspring from infertile mice. Proc Natl Acad Sci USA 2002; 99:7524 - 9; http://dx.doi.org/10.1073/pnas.072207299; PMID: 12032316
- Kim TS, Choi HS, Ryu BY, Gang GT, Kim SU, Koo DB, et al. Real-time in vivo bioluminescence imaging of lentiviral vector-mediated gene transfer in mouse testis. Theriogenology 2010; 73:129 - 38; http://dx.doi.org/10.1016/j.theriogenology.2009.07.028; PMID: 19837451
- Oatley JM, Avarbock MR, Telaranta AI, Fearon DT, Brinster RL. Identifying genes important for spermatogonial stem cell self-renewal and survival. Proc Natl Acad Sci USA 2006; 103:9524 - 9; http://dx.doi.org/10.1073/pnas.0603332103; PMID: 16740658
- Rao MK, Pham J, Imam JS, MacLean JA, Murali D, Furuta Y, et al. Tissue-specific RNAi reveals that WT1 expression in nurse cells controls germ cell survival and spermatogenesis. Genes Dev 2006; 20:147 - 52; http://dx.doi.org/10.1101/gad1367806; PMID: 16418481
- Harcourt AH, Harvey PH, Larson SG, Short RV. Testis weight, body weight and breeding system in primates. Nature 1981; 293:55 - 7; http://dx.doi.org/10.1038/293055a0; PMID: 7266658
- Maclean JA 2nd, Chen MA, Wayne CM, Bruce SR, Rao M, Meistrich ML, et al. Rhox: a new homeobox gene cluster. Cell 2005; 120:369 - 82; http://dx.doi.org/10.1016/j.cell.2004.12.022; PMID: 15707895
- Sadri-Ardekani H, Mizrak SC, van Daalen SK, Korver CM, Roepers-Gajadien HL, Koruji M, et al. Propagation of human spermatogonial stem cells in vitro. JAMA 2009; 302:2127 - 34; http://dx.doi.org/10.1001/jama.2009.1689; PMID: 19920237
- Kanatsu-Shinohara M, Inoue K, Lee J, Yoshimoto M, Ogonuki N, Miki H, et al. Generation of pluripotent stem cells from neonatal mouse testis. Cell 2004; 119:1001 - 12; http://dx.doi.org/10.1016/j.cell.2004.11.011; PMID: 15620358
- Panula S, Medrano JV, Kee K, Bergström R, Nguyen HN, Byers B, et al. Human germ cell differentiation from fetal- and adult-derived induced pluripotent stem cells. Hum Mol Genet 2011; 20:752 - 62; http://dx.doi.org/10.1093/hmg/ddq520; PMID: 21131292
- Park TS, Galic Z, Conway AE, Lindgren A, van Handel BJ, Magnusson M, et al. Derivation of primordial germ cells from human embryonic and induced pluripotent stem cells is significantly improved by coculture with human fetal gonadal cells. Stem Cells 2009; 27:783 - 95; http://dx.doi.org/10.1002/stem.13; PMID: 19350678
- Barritt JA, Brenner CA, Malter HE, Cohen J. Mitochondria in human offspring derived from ooplasmic transplantation. Hum Reprod 2001; 16:513 - 6; http://dx.doi.org/10.1093/humrep/16.3.513; PMID: 11228222
- Swanson WJ, Vacquier VD. The rapid evolution of reproductive proteins. Nat Rev Genet 2002; 3:137 - 44; http://dx.doi.org/10.1038/nrg733; PMID: 11836507