Abstract
Bile ducts play a crucial role in the formation and secretion of bile as well as excretion of circulating xenobiotic substances. In addition to its secretory and excretory functions, bile duct epithelium plays an important role in the formation of a barrier to the diffusion of toxic substances from bile into the hepatic interstitial tissue. Disruption of barrier function and toxic injury to liver cells appear to be involved in the pathogenesis of a variety of liver diseases such as primary sclerosing cholangitis, primary biliary cirrhosis and cholangiocarcinoma. Although the investigations into understanding the structure and regulation of tight junctions in gut, renal and endothelial tissues have expanded rapidly, very little is known about the structure and regulation of tight junctions in the bile duct epithelium. In this article we summarize the current understanding of physiology and pathophysiology of bile duct epithelium, the structure and regulation of tight junctions in canaliculi and bile duct epithelia and different mechanisms involved in the regulation of disruption and protection of bile duct epithelial tight junctions. This article will make a case for the need of future investigations toward our understanding of molecular organization and regulation of canalicular and bile duct epithelial tight junctions.
Liver is an essential organ with multiple functions. Secretion of bile, that is required for normal digestive function of the intestine, and excretion of circulating xenobiotic substances are two major functions of liver. Hepatocytes and cholangiocytes are two important cell types in the liver. The coordinated function of these cells is involved in the production and secretion of bile. Primary bile is produced and secreted by hepatocytes, which then undergoes modifications in its volume and composition in the ductules by epithelial cells or cholangiocytes during its transit in the network of biliary tree. Biliary tree is composed of interconnected ducts of increasing diameter from liver to duodenum. It is divided into intrahepatic bile ducts (IHBD) and extrahepatic hepatic ducts (EHBD). IHBD start at the canaliculi continues as bile ductules, interlobular ducts, area ducts, septal ducts and segmental bile ducts that join to form large IHBD. These ducts exhibit heterogeneous features based on morphological and histological analyses as well as their embryological origin. IHBD drain into EHBD system, which consists of right and left hepatic ducts, common hepatic duct, gall bladder, cystic duct and common bile duct.Citation1
Cholangiocytes were once thought as simple columnar epithelium, now gaining importance from physiological and pathological viewpoints. Although they constitute 4–5% of total liver cells, cholangiocytes contribute 40% of total daily bile secretion.Citation2 Complex nature of their biological heterogeneity along the axis of biliary tree emphasizes their critical role in a variety of fundamental processes of hepatic functions, and therefore forms a potential target for the development of many types of cholangiopathies.Citation3 Intrahepatic biliary epithelia vary from extrahepatic biliary epithelia in structure, function and embryological origin. Embryonic differentiation of EHBD takes place from hepatic endodermal cells of caudal region of embryonic hepatic diverticula; whereas IHBD originate from periportal hepatoblasts forming ductal plate that originate from cranial part of hepatic diverticula.Citation4 Likewise, pathogenesis of these two ductal systems shows diverging trends. In IHBD, small ducts are lined with small cholangiocytes and the larger ducts are lined with larger columnar epithelial cells, which allow them to meet the functional diversity, including secretion, excretion and barrier function.
Cholangiocytes, primarily involved in secretion of bicarbonate rich bile, are highly dynamic cells and are actively involved in biological processes such as transport of solutes and water in response to a variety of stimuli.Citation5 Cholangiocytes interact with other hepatic cells as well as microbes and hence exhibit heterogeneity in their form and function along the proximal to distal axis.Citation3 Therefore, liver is a target for the pathogenesis of immune-mediated, infectious, neoplastic, genetic, developmental, drug-induced and vasculature-related diseases.Citation6
Barrier Function of Bile Duct Epithelium
All epithelia form an interface between the external environment and the internal milieu, and assigned with the role to protect the tissues from external environment. Therefore, an important function of all epithelia is the formation of a selective barrier to the diffusion of molecules across the epithelium. This barrier, in most epithelia, is size selective and charge selective.Citation7 In the gut, epithelial barrier function prevents the diffusion of toxins, allergens and pathogens from the lumen into intestinal mucosa.Citation8 In lungs, the epithelium functions to guard the tissue from allergens and pathogens delivered in the inhaled air.Citation9 Similarly, renal tubular epithelial barrier function help prevent reabsorption of toxic substances from the glomerular filtrate.Citation10 Disruption of intestinal epithelial barrier function appears to be an essential event in the onset of many gastrointestinal diseases such as inflammatory bowel disease, celiac disease, enterocolitis, colon cancer and irritable bowel syndrome. Similarly, disruption of epithelial barrier function plays a crucial role in the pathogenesis of many pulmonary and renal diseases.Citation8-Citation10 The barrier function of bile duct epithelium has been established, but the nature of barrier function and its regulation are poorly understood.
Similar to other organ systems, liver maintains osmotic homeostasis.Citation11 Obstruction of common bile duct leads to disruption of osmotic pressure in the liver. Bile duct epithelium forms a transitional monolayer between the liver interstitial tissue and the lumen of bile ducts. Therefore, it must maintain a permeability barrier to prevent free flow of water from interstitial tissue into the hyperosmotic bile.Citation12 Bile duct epithelial barrier function was first showed by Lanthanum tracing experiments.Citation13 Bile duct epithelium blocks the diffusion of Lanthanum across the epithelium. Later it was shown that rat bile duct epithelium impedes permeability to inulin.Citation14 Bile duct epithelial barrier function was further characterized by measuring permeability to molecules of varying size by perfusing isolated bile duct segments in the anesthetized rats.Citation15 The perfusate-to-plasma concentration ratio was inversely proportional to the molecular weight of the substance, with lowest ratio for inulin and highest ratio for urea. This observation was further confirmed in guinea pigs by bile duct perfusion in vivo.Citation16 Perfusate-to-plasma concentration ratio was 1.02 for urea, while it was only 0.04 for inulin. Similar permeability barrier was demonstrated also in the EHBD.Citation17,Citation18
Bile duct epithelial cells have been successfully grown in vitro by cell culture and demonstrated their differentiation into a monolayer of polarized epithelial cells.Citation19 This cell monolayer assembled well-developed tight junctions and formed a good barrier for diffusion of horseradish peroxidase (HRP). A transepithelial electrical resistance of 137 Ohms.cm2 was achieved in this cell culture model of bile duct epithelium. Establishment of cholangiocytes in culture offered a new model system for the study of bile duct epithelium and enabled investigators not only to analyze the barrier property of bile duct epithelium, but also to investigate the regulation of bile duct epithelial barrier function. Subsequently, a primary rat intrahepatic cholangiocyte cell line was cloned that formed a polarized epithelial monolayer in culture.Citation20 This cell monolayer developed a barrier to the diffusion of inulin.Citation21,Citation22 Mouse cholangiocyte in culture also showed similar barrier function.Citation23 Two human cholangiocyte lines were later isolated, one non-malignant cholangiocytes immortalized by transfection of Simian virus-40 (SV40) large T antigen, and the second, malignant cholangiocarcinoma cell line.Citation24 The barrier function of the latter monolayer was found to be weak, and the barrier function of the former monolayer is untested. Similarly, mouse cholangiocytes immortalized by SV40 transfection exhibit leaky epithelium with weak barrier function.Citation25 All these cell lines will be very useful for future studies addressing the mechanisms involved in the regulation of bile duct epithelial barrier function.
Tight Junctions of Bile Duct Epithelium
Tight junctions, the specialized multi-protein complexes that are localized at the apical end of lateral membranes, seals the paracellular spaces.Citation26 Tight junctions exhibit selective permeability to molecules, including charge selectivity and size selectivity.Citation27-Citation29 A close examination of tight junctions by electron microscopy has revealed a unique ultra structural morphology. Transmission electron microscopy showed electron dense region at the apical end of lateral membranes of epithelial cells with regularly spaced electron dense punctates. Freeze fracture electron microscopy revealed tight junction morphology as anastomosing strands of beads.Citation30 Tight junction protein complex consists of transmembrane proteins such as occludin, claudins, junctional adhesion molecules, tricellulin and Marvel D3.Citation26-Citation29 Except the junctional adhesion molecules, all transmembrane proteins of tight junctions are tetraspanins with four transmembrane domains, and hence form two extracellular loops and one intracellular loop. The C-terminal domain in the cytosol forms a complex with adaptor proteins such as ZO-1, ZO-2 and ZO-3. These adaptor proteins interact with many other proteins and anchor themselves to the actin cytoskeleton. Interactions between different tight junction proteins and the cytoskeleton are essential for the normal assembly and maintenance of tight junction integrity. Tight junctions in different epithelia are disrupted by injurious factors such as, toxins, pathogens and inflammatory cytokines,Citation31-Citation33 while some protective factors such as growth factors, probiotics, and glutamine help preserve the tight junction integrity.Citation34-Citation38 Both disruption and assembly of tight junctions are regulated by a variety of signaling elements, including protein kinases, protein phosphatases and G-proteins.Citation39-Citation42
A clear barrier to the diffusion of macromolecules such as inulin, Lanthanum, HRP and dextran indicated the presence of tight junctions in the bile duct epithelium. Tight junctions are expected to be present at the bile canaliculi formed by hepatocytes as well as in the epithelia that line the intrahepatic biliary tree and the extrahepatic bile ducts. Tight junctions in bile canaliculi were first observed by electron microscopic studies. Freeze fracture electron microscopic images revealed tight junctions as regular belt of parallel strands around canalicular lumen.Citation43,Citation44 Transmission electron microscopy showed tight junctions in the bile ducts as electron dense regularly distributed punctates.Citation13 Tight junctions between hepatocytes form the canaliculi, while the intrahepatic bile duct lumen is lined with a monolayer of epithelial cells or cholangiocytes with tight junctions sealing the paracellular spaces (). The structure, molecular organization and regulation of bile duct epithelial tight junctions are poorly understood. The information currently available relates predominantly to the tight junctions of bile canaliculi. Very little is known about the tight junctions of intrahepatic and extrahepatic bile duct epithelium. Therefore, current understanding of molecular structure of bile duct epithelial tight junction predominantly rests on our understanding of molecular structure of canalicular tight junctions.
Figure 1. Electron microscopic image showing the cross section of bile duct. TJ, tight junction; N, nucleus; BDL, bile duct lumen; MV, microvilli. The image was obtained, with permission, from Electron Microscopic Atlas of Cells, Tissues and Organs by Dr. Holger Jastrow, University of Maintz, Germany.
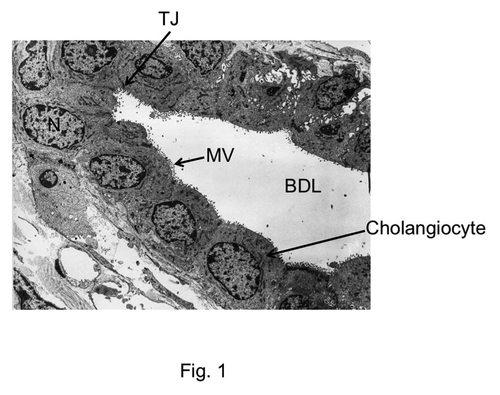
It was in 1989, Anderson and co-investigators first described the distribution of ZO-1, the first tight junction protein discovered, in the rat liver canaliculi.Citation45 Immunofluorescence staining revealed that ZO-1 is distributed in a linear and continuous manner along the margin of canalicular lumen. Later the same group of investigators visualized the distribution of occludin, the first discovered transmembrane protein of tight junctions, in bile canaliculi. Occludin was also found to be distributed in linear and continuous manner, and it is co-localized with ZO-1.Citation46 Immunolocalization of 7H6, another tight junction protein, closely correlated with the paracellular permeability barrier. Immunofluorescence staining of cryosections of rat liver indicated a linear distribution of 7H6 around the canalicular lumen.Citation47 Further studies demonstrated the expression of claudin 1, 2 and 3 in rat liver.Citation48 In zebra fish, the expression of claudin 15-like b protein was found to be essential for bile duct morphogenesis.Citation49 Mutation of this gene resulted in clustering of biliary epithelial cells and formation of disorganized biliary network. Expression of claudins 4, 5, 7 and 18 were detected in the normal or carcinomatous hepatocytes and cholangiocytes.Citation50-Citation52 The types of tight junction proteins detected in the tight junctions of canaliculi and bile ducts are listed in .
Table 1. Different tight junction proteins in canaliculi and bile ducts
Current information on the molecular structure of ductal epithelia has been derived from in vitro studies using cholangiocyte monolayers grown in culture. Monolayers of normal rat cholangiocytes (NRC1 cell line) in culture developed a good barrier function.Citation22 Development of barrier function was associated with a co-localization of occludin and ZO-1 at the intercellular junctions.Citation22 NRC1 cell monolayers also express claudin 1, 2, 3, 4 and 5, all found to be localized at the intercellular junctions.Citation21,Citation22,Citation53 Occludin and ZO-1 in NRC1 cell monolayers were found to be heavily phosphorylated on threonine residues.Citation22 Threonine phosphorylation of occludin is known to be associated with intact tight junctions, which undergoes dephosphorylation during disruption by hydrogen peroxide or calcium depletion.Citation54,Citation55
Regulation of Barrier Function
Damage to biliary epithelium is involved in the pathogenesis of several chronic cholestatic disorders and cholangiopathies. A most common event in bile duct associated liver disease is obstruction of intrahepatic or extrahepatic bile ducts. Experimental models of bile duct ligation for extrahepatic bile duct obstruction and estradiol treatment for induction of intrahepatic bile duct obstruction have been extensively used to understand the pathogenesis of biliary diseases. A major defect that mediates bile duct obstruction-induced biliary diseases is considered to be the disruption of bile duct epithelial barrier function.
Bile duct ligation in rats induced a 6-fold increase in inulin permeability of canaliculi.Citation14 Intrahepatic bile duct obstruction by estradiol treatment increased HRP secretion into bile by 2-fold, while extrahepatic bile duct ligation led to 6.5 fold increase in HRP secretion.Citation56 This indicates that the effect of extrahepatic bile duct obstruction has a greater effect on bile duct barrier function compared with estradiol-induced intrahepatic bile duct obstruction. This study also showed that the size selectivity of bile duct epithelium was reduced to 70–78 kDa molecular weight in estradiol-treated rats, while it was 256 kDa in bile duct ligated rats, indicating that the paracellular space was opened much wider in bile duct ligated animals, compared with that in estradiol-treated animals. The increase in paracellular permeability in bile duct ligated rats was confirmed by Lanthanum deposition in all canaliculi.Citation48
Bile duct barrier function is also increased by the administration of toxins and drugs. Exposure to Mirex or chlordecone causes a significant decrease in xenobiotic excretion, which was associated with the increased bile duct permeability.Citation57 Administration of α-naphthylisothiocyanate resulted in hepatobiliary dysfunction leading to cholestasis.Citation58 Increase in bile duct permeability appears to be responsible for the onset of cholestasis in this injury. Evidence indicates that physiological factors such as vasopressin and epinephrine also increase bile duct permeability to sucrose in rat liver.Citation59
Cell culture models of bile duct epithelium are excellent tools to study regulation of bile duct epithelial barrier function. Monolayers of intrahepatic bile duct epithelial cells develop a good barrier function when grown in cell culture. Incubation of these cell monolayers with tumor necrosis factor-α (TNFα) produced a significant increase in paracellular permeability to HRP without causing any loss of cell viability.Citation18 Most recently NRC1 cell monolayer was used as a cell culture model of the bile duct epithelium to study the regulation of barrier function. Exposure to E. coli lipopolysaccharide (LPS) significantly increased paracellular permeability.Citation22 Paracellular permeability in NRC1 cell monolayers was also significantly increased by administration of hydrogen peroxide.Citation21 Hydrogen peroxide-induced barrier dysfunction was attenuated by pretreatment of cell monolayers with epidermal growth factor (EGF).Citation21
Regulation of Bile Duct Epithelial Tight Junctions
Electron microscopic studies examined tight junction morphology in liver with and without bile duct ligation. Bile duct ligation resulted in disruption of intercellular junctions with formation of irregular canaliculi containing widened lumen.Citation44 Freeze fracture electron microscopy showed that bile duct ligation caused a reduction in the number of strands and appearance of discontinuous strands in tight junctions.Citation43,Citation60 Bile duct ligation also resulted in irregular strand network and formation of irregular loops in strands. These findings suggest an increased paracellular permeability and likely back flux of bile components into liver parenchyma. Transmission electron microscopy showed irregular distribution of electron dense punctates at tight junctions, increase in depth of tight junctions and wider distance between punctates.Citation13 Intrahepatic obstruction induced by estradiol treatment also resulted in irregular tight junction morphology with formation of loose network around canalicular lumen.Citation61
Immunofluorescence localization of ZO-1 indicated that bile duct ligation resulted in numerous discontinuous strands that were associated with irregular luminal space.Citation45 This observation suggests that molecular organization of tight junction was altered by bile duct ligation. Bile duct ligation caused accumulation of ZO-1 at the pericanalicular region as punctates, which was associated with an increased expression of ZO-1.Citation62 Unlike increased ZO-1 expression, occludin level was reduced by 50% at 2 d after bile duct ligation.Citation46 Also, unlike ZO-1, occludin distribution in bile duct ligated rat liver was at the tight junctions in a linear fashion. Localization of 7H6 was also altered by bile duct ligation. 7H6 was found to be discontinuously distributed outlining the bile duct canaliculi in bile duct ligated rat liver.Citation47 Similar disruption of 7H6 localization was seen in estradiol-treated rat liver; however, 7H6 was distributed more diffusively throughout the lobule. Expression of occludin was found to be increased in rat liver by bile duct ligation, while the expression of claudin 1, 2 and 3 was unaffected.Citation48 Oral administration of Lactobacillus plantarum, a probiotic, ameliorated bile duct ligation-induced disruption of tight junctions and increased the expression of occludin, claudin 4 and ZO-1.Citation63 Claudin-1 and ZO-2 localization was high in periportal cells of bile duct ligated rat liver, while other tight junction proteins were evenly distributed.Citation64 Bile duct ligation also increased the expression of ZO-1, ZO-2 and occludin, but the expression of claudins was unaffected.
Hepatitis C virus coat protein alters hepatic occludin localization, which is likely involved in the disruption of tight junctions.Citation65 Furthermore, expression of occludin, ZO-1 and E-cadherin were found to be downregulated in biopsies collected from patients with biliary tract cancer.Citation66 Such a downregulation of tight junction proteins may be involved in the loss of cell-cell adhesion and epithelial to mesenchymal transition.
In vitro studies using cell culture models of bile duct epithelium demonstrated that inflammatory mediators affect the integrity of tight junctions. Exposure of NRC1 cell monolayers to LPS resulted in redistribution of occludin, ZO-1 and claudin-4 from the intercellular junctions into the intracellular compartments,Citation22 indicating that LPS disrupted tight junctions. LPS treatment did not cause any loss of cell viability. Similarly, exposure to hydrogen peroxide caused redistribution of occludin, ZO-1 and claudin-3 from the intercellular junctions, indicating the disruption of tight junctions in bile duct epithelium by hydrogen peroxide.Citation21 EGF, an epithelial protective factor, preserves the barrier function of bile duct epithelium. Hydrogen peroxide-induced disruption of tight junctions in NRC1 cell monolayers was attenuated by pretreatment of cell monolayers with EGF.Citation21 Therefore, it is likely that under normal conditions, the tight junction integrity in the bile duct epithelium is a result of balance between influences by injurious factors and protective factors.
Cellular Mechanisms of Regulation of Bile Duct Epithelial Tight Junctions
Tight junctions in different epithelia are dynamically regulated by multiple regulatory mechanisms. Intestinal, renal and pulmonary epithelial tight junctions are targeted by inflammatory mediators such as cytokines, reactive oxygen species (ROS) and pathogens. Various injurious factors disrupt epithelial tight junctions by activating multiple intracellular signaling pathways. Protein kinases are a group of signaling molecules that are involved in both disruption and assembly of tight junctions. Activation of tyrosine kinases such as c-Src and tyrosine phosphorylation of tight junction proteins disrupts barrier function in the intestinal and renal epithelia.Citation40,Citation67 PKC isoforms such as PKCλ, PKCζ and PKCη are involved in the assembly of tight junctions,Citation42,Citation68,Citation69 while activation of PKCδ leads to disruption of tight junctions.Citation70 Proteins phosphatases such as PP2A and PP1 are involved in modulation of phosphorylation of tight junction proteins on threonine residues and regulation of tight junction integrity.Citation54,Citation55,Citation71 Other protein kinases involved in tight junction regulation include MLCK,Citation72,Citation73 Rho kinase,Citation74 protein kinase A,Citation75-Citation83 and AMP kinase.Citation84 Additionally, G-proteins such as Rho and Rab isoforms play crucial role in assembly and maintenance of tight junction integrity.Citation81-Citation83
In vivo studies in rats showed that bile duct ligation-induced tight junction disruption was associated with increased phosphorylation of ZO-2 64. This suggests that protein kinase and/or protein phosphatase-mediated cell signaling is involved in bile duct ligation-induced tight junction disruption. Conditional ablation of IKKα and IKKβ sensitizes liver to in vivo LPS challenge leading to severe jaundice and fatal cholangitis.Citation85 NFκB-mediated cell signaling is likely to be associated with bile duct epithelial tight junction regulation. While in vivo studies suggested the potential role of cell signaling pathways in regulation of bile duct epithelial barrier function, recent in vitro studies demonstrated the role of certain specific signaling pathways in regulation of bile duct epithelial tight junctions.
Disruption of tight junctions of NRC1 cell monolayers by LPS was attenuated by tyrosine kinase inhibitors such as genistein and PP2.Citation22 Knockdown of c-Src by specific siRNA attenuated LPS-induced tight junction disruption, demonstrating that c-Src is a signaling molecule associated with the LPS-induced tight junction disruption in the bile duct epithelium. LPS increased tyrosine phosphorylation of occludin and ZO-1, which was blocked by genistein or PP2.Citation22 The role of tyrosine phosphorylation of occludin in tight junction disruption has been established in the intestinal epithelium. Tyrosine phosphorylation of occludin on specific residues results in loss of its interaction with ZO-1 and therefore disassembly of tight junctions.Citation40,Citation86-Citation88 LPS in NRC1 cell monolayers also induced dephosphorylation of occludin on threonine residues.Citation22 This occludin dephosphorylation was attenuated by genistein and PP2, suggesting that a cross talk between tyrosine kinases and threonine kinases may regulate tight junction integrity in the bile duct epithelium. Occludin has been shown to be heavily phosphorylated on threonine residues in intact monolayers of Caco-2 and MDCK cells.Citation54,Citation89 Recent studies demonstrated that phosphorylation of occludin on specific threonine residues is required for its assembly into tight junctions.Citation42 The blockade of threonine-dephosphorylation in LPS-treated cell monolayers by PP2 suggested that Src kinase activity indirectly regulates kinase or phosphatases involved in threonine-phosphorylation of occludin. LPS-induced tight junction disruption in NRC1 cell monolayers involved toll-like receptors-4 (TLR-4) and LPS-binding protein (LBP).Citation22 Specific knockdown of TLR4 or LBP significantly attenuated LPS-induced tight junction disruption. Therefore, LPS-induced tight junction disruption involves c-Src-mediated tyrosine phosphorylation of occludin and ZO-1, and dephosphorylation of occludin on threonine residues. This LPS-induced signaling is mediated by LBP and TLR4. Further studies showed that LPS-induced tight junction disruption was blocked by ML-7, an MLCK-specific inhibitor, indicating that MLCK is also involved in the mechanism of LPS-induced tight junction disruption ().
Figure 2. Mechanism of LPS-induced tight junction disruption in rat cholangiocyte monolayer. LPS complexes with LBP and binds to TLR4 receptor. TLR4 activation leads to activation of c-Src and MLCK. c-Src phosphorylates tight junction proteins on tyrosine residues, while induced dephosphorylation of occludin on threonine residues. On the other hand MLCK activation leads to modulation of actomyosin belt. These two mechanisms synergistically destabilize the tight junctions and induce barrier dysfunction.
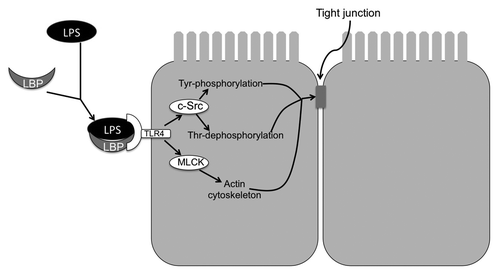
Hydrogen peroxide-induced tight junction disruption in NRC1 cell monolayers was associated with a rapid increase in the level of phosphorylated myosin light chain, (MLC) indicating the activation of MLCK by hydrogen peroxide.Citation21 Inhibition of MLCK by ML-7 attenuated hydrogen peroxide-induced tight junction disruption. This indicates that MLCK activation is a common mechanism involved in both LPS and hydrogen peroxide-induced tight junction disruption in NRC1 cell monolayers. Similar to LPS, hydrogen peroxide also rapidly activated c-Src, and inhibition of Src kinase by PP2 blocked hydrogen peroxide-induced tight junction disruption.Citation21 This study showed that activation of c-Src and MLCK are independent of each other. Therefore, two distinct signaling pathways are activated by hydrogen peroxide to synergistically induce tight junction disruption. Src activation modulates phosphorylation status of tight junction proteins, while MLCK activation modulates the integrity of actomyosin belt. Both these mechanisms have been previously shown to disrupt tight junctions in the intestinal epithelium. In support of the role of MLCK, the study showed that hydrogen peroxide disrupts the actin cytoskeleton mainly at the actomyosin belt region.Citation21
EGF-mediated protection of tight junctions of NRC1 cell monolayers from hydrogen peroxide was associated with the prevention of hydrogen peroxide-induced tyrosine-phosphorylation of occludin, ZO-1, E-cadherin and β-catenin.Citation21 EGF-mediated protection of tight junctions from hydrogen peroxide was blocked by the inhibition of phospholipase Cγ (PLCγ) and protein kinase C (PKC). Therefore, PLCγ and PKC isoforms are likely involved in the mechanism of EGF-mediated tight junction protection in the bile duct epithelium. PLCγ and PKC isoforms have been previously shown to be involved in EGF-mediated tight junction protection in Caco-2 cell monolayers.Citation90 c-Src and MLCK-mediated disruption of tight junctions by hydrogen peroxide as well as PLCγ and PKC-mediated protection of tight junctions by EGF was also demonstrated in Mz-Ch1 cell monolayers, a human cholangiocyte cell line.Citation21 Therefore, c-Src and MLCK activations appear to be involved in tight junction disruption, and PLCγ and PKC isoforms are involved in the protection of tight junctions in bile duct epithelium ().
Figure 3. Mechanism of hydrogen peroxide-induced tight junction disruption and protection by epidermal growth factor in rat cholangiocyte monolayer. Hydrogen peroxide (H2O2) activates c-Src and MLCK. c-Src phosphorylates tight junction proteins on tyrosine residues. On the other hand, MLCK activation leads to modulation of actomyosin belt. These two mechanisms synergistically destabilize tight junctions and induce barrier dysfunction.
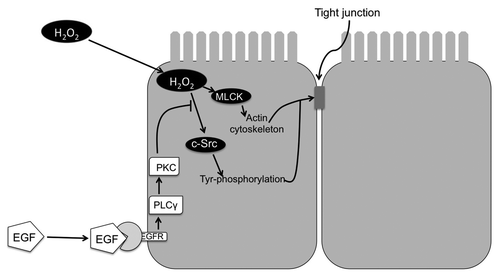
Tight Junction Dysfunction in Biliary Diseases
Hepatocyte tight junction integrity is essential for the maintenance of structure and function of canaliculi, while tight junctions of the bile duct epithelium is required for the maintenance of epithelial cell polarity and unidirectional movement of bile into the lumen of bile ducts. Loss of tight junction integrity may lead to structural deformity of canaliculi and bile ducts and regurgitation of bile components into liver interstitial tissue. Back flux of bile components in the interstitial tissue may cause injury to hepatocytes and vascular endothelial cells. Such a loss of barrier function and bile-induced cell injury is a likely mechanism involved in the onset of many biliary diseases.Citation91
Altered function of biliary tract is a significant source of morbidity and graft failure in patients with liver transplantation.Citation92 In fact, chronic hepatic allograft rejection is associated biliary epithelial atrophy.Citation93 One of the mechanisms of liver transplantation failure is ischemia-reperfusion type of injury to the transplanted liver. Evidence indicates that ischemia-reperfusion induces alteration in bile canalicular F-actin microfilaments in hepatocytes.Citation94 Ischemia-reperfusion generates reactive oxygen species, the factors that are likely involved in tight junction disruption and bile duct injury in the transplanted liver. Significant evidence indicates that bile duct is damaged after cold storage. Distribution of tight junction protein 1 and claudin-1 is altered by cold storage and more so after reperfusion.Citation95
A significant body of evidence indicates that pathogenesis of cholestatic diseases such as PBC,Citation96 PSCCitation97 and biliary atresia involves disruption of tight junctions and biliary dysfunction. A tight correlation between cholestasis and leaky tight junctions was recognized almost 2 decades ago.Citation98 In PBC, the level of 7H6 is diminished in bile duct epithelium, which was reversed by treatment of these patients with ursodeoxy cholic acid.Citation99 On the other hand, in PSC, 7H6 disappeared from the hepatocyte tight junctions. Disruption of hepatocyte tight junctions was confirmed in experimental models of cholestasis.Citation100 Claudin 1 deficiency is attributed as one of the main defects in neonatal ichthyosis and sclerosing cholangitis syndrome.Citation101
Incidence of intrahepatic, extrahepatic and gall bladder carcinoma is increasing worldwide. Integrity of bile duct epithelial tight junctions appears to be compromised in hepatocellular tumors and cholangiocarcinoma. Occludin, ZO-1 and E-cadherin levels are downregulated in both intrahepatic and extrahepatic carcinomas.Citation66 Carcinogenesis has profound effect on tight junctions. Claudin-7 is undetectable in normal hepatocytes, but it is expressed in bile duct epithelial cells. The level of claudin-7 is increased in cholangiocarcinomas, but not in hepatocellular carcinomas.Citation102 Therefore, claudin-7 may be helpful in distinguishing cholangiocarcinoma from hepatocellular carcinomas. Claudin 3, 4 and 7 are undetectable in fibrolamellar hepatocellular carcinomas, but their expression is high in cholangiocellular carcinomas.Citation50 Focal and diffuse expression of claudin-5 was detected in hepatocellular carcinoma. Tricellulin was downregulated in all tumors compared with normal tissue. Claudin-18 is absent in normal liver, but it is expressed in biliary neoplasms, such as intrahepatic and extrahepatic carcinomas and papillary neoplasms.Citation51 Claudin-4 is overexpressed in cholangiocarcinomas and knockdown of claudin-4 reduces the expansion and metastasis of tumors .Citation52 Therefore, expression of tight junction proteins is altered in both hepatocellular and cholangiocellular carcinomas. Disruption of tight junctions may not only be involved in the carcinogenic transformation of cells, but also a likely mechanism involved in tumor metastasis. Expression of claudin isoforms may indeed offer a potential tool in distinguishing hepatocellular carcinomas from cholangiocellular carcinomas. Various biliary diseases that are associated with altered tight junctions in canaliculi and bile ducts are listed in .
Table 2. Alteration of tight junction proteins in biliary diseases
In summary, it is clear that maintenance of normal tight junction integrity in both hepatocytes that form canaliculi and cholangiocytes that form bile duct epithelium is of extreme importance for the normal functioning of bile ducts in bile formation and preservation of homeostasis in the hepatic interstitial tissue. Disruption of tight junction integrity in canaliculi and bile ducts plays a crucial role in the pathogenesis of biliary diseases such as ischemic cholangitis, cholestatic diseases such as PBC and PSC, and hepatocellular and cholangiocarcinoma. However, very little is understood regarding the structure and regulation of tight junctions in liver. Current information on bile duct is predominantly based on studies on the tight junctions of canaliculi. The information on tight junctions of bile duct epithelium is only handful and recent. Therefore, future studies on understanding the molecular components of bile duct epithelial tight junctions and the regulatory mechanisms that disrupt tight junctions or protect junctions from injurious factors are extremely important to understand the mechanisms associated with the pathogenesis of various biliary diseases and development of novel therapeutics to treat these diseases.
Acknowledgments
Supported by Mussette and Alan Morgan Jr. Foundation for the study of PSC; NIH grants DK55532 and AA12307.
Disclosure of Potential Conflicts of Interest
No potential conflicts of interest were disclosed
References
- Alvaro D, Mancino MG, Onori P, Franchitto A, Alpini G, Francis H, et al. Estrogens and the pathophysiology of the biliary tree. World J Gastroenterol 2006; 12:3537 - 45; PMID: 16773710
- Syal G, Fausther M, Dranoff JA. Advances in cholangiocyte immunobiology. Am J Physiol Gastrointest Liver Physiol 2012; 303:G1077 - 86; http://dx.doi.org/10.1152/ajpgi.00227.2012; PMID: 22961800
- LaRusso NF. Morphology, physiology, and biochemistry of biliary epithelia. Toxicol Pathol 1996; 24:84 - 9; http://dx.doi.org/10.1177/019262339602400112; PMID: 8839285
- Shiojiri N. Development and differentiation of bile ducts in the mammalian liver. Microsc Res Tech 1997; 39:328 - 35; http://dx.doi.org/10.1002/(SICI)1097-0029(19971115)39:4<328::AID-JEMT3>3.0.CO;2-D; PMID: 9407543
- Strazzabosco M. Transport systems in cholangiocytes: their role in bile formation and cholestasis. Yale J Biol Med 1997; 70:427 - 34; PMID: 9626763
- Roberts SK, Yano M, Ueno Y, Pham L, Alpini G, Agre P, et al. Cholangiocytes express the aquaporin CHIP and transport water via a channel-mediated mechanism. Proc Natl Acad Sci U S A 1994; 91:13009 - 13; http://dx.doi.org/10.1073/pnas.91.26.13009; PMID: 7528928
- Anderson JM, Van Itallie CM. Physiology and function of the tight junction. Cold Spring Harb Perspect Biol 2009; 1:a002584; http://dx.doi.org/10.1101/cshperspect.a002584; PMID: 20066090
- Camilleri M, Madsen K, Spiller R, Greenwood-Van Meerveld B, Verne GN. Intestinal barrier function in health and gastrointestinal disease. Neurogastroenterol Motil 2012; 24:503 - 12; http://dx.doi.org/10.1111/j.1365-2982.2012.01921.x; PMID: 22583600
- Ogasawara N, Kojima T, Go M, Takano K, Kamekura R, Ohkuni T, et al. Epithelial barrier and antigen uptake in lymphoepithelium of human adenoids. Acta Otolaryngol 2011; 131:116 - 23; http://dx.doi.org/10.3109/00016489.2010.520022; PMID: 21062118
- Li J, Ananthapanyasut W, Yu AS. Claudins in renal physiology and disease. Pediatr Nephrol 2011; 26:2133 - 42; http://dx.doi.org/10.1007/s00467-011-1824-y; PMID: 21365189
- Opie EL, Rothbard MB. Osmotic homeostasis maintained by mammalian liver, kidney, and other tissues. J Exp Med 1953; 97:483 - 97; http://dx.doi.org/10.1084/jem.97.4.483; PMID: 13052814
- Esteller A. Physiology of bile secretion. World J Gastroenterol 2008; 14:5641 - 9; http://dx.doi.org/10.3748/wjg.14.5641; PMID: 18837079
- Koga A, Todo S. Morphological and functional changes in the tight junctions of the bile canaliculi induced by bile duct ligation. Cell Tissue Res 1978; 195:267 - 76; http://dx.doi.org/10.1007/BF00236724; PMID: 737720
- Accatino L, Contreras A, Berdichevsky E, Quintana C. The effect of complete biliary obstruction on bile secretion. Studies on the mechanisms of postcholestatic choleresis in the rat. J Lab Clin Med 1981; 97:525 - 34; PMID: 6259265
- Smith ND, Boyer JL. Permeability characteristics of bile duct in the rat. Am J Physiol 1982; 242:G52 - 7; PMID: 7058899
- Tavoloni N. Permeation patterns of polar nonelectrolytes across the guinea pig biliary tree. Am J Physiol 1984; 247:G527 - 36; PMID: 6437250
- Tavoloni N, Jones MJ, Berk PD. Postnatal development of bile secretory physiology in the dog. J Pediatr Gastroenterol Nutr 1985; 4:256 - 67; http://dx.doi.org/10.1097/00005176-198504000-00018; PMID: 3989625
- Mano Y, Ishii M, Okamoto H, Igarashi T, Kobayashi K, Toyota T. Effect of tumor necrosis factor alpha on intrahepatic bile duct epithelial cell of rat liver. Hepatology 1996; 23:1602 - 7; PMID: 8675183
- Okamoto H, Ishii M, Mano Y, Igarashi T, Ueno Y, Kobayashi K, et al. Confluent monolayers of bile duct epithelial cells with tight junctions. Hepatology 1995; 22:153 - 9; http://dx.doi.org/10.1002/hep.1840220125; PMID: 7601408
- Vroman B, LaRusso NF. Development and characterization of polarized primary cultures of rat intrahepatic bile duct epithelial cells. Lab Invest 1996; 74:303 - 13; PMID: 8569194
- Guntaka SR, Samak G, Seth A, LaRusso NF, Rao R. Epidermal growth factor protects the apical junctional complexes from hydrogen peroxide in bile duct epithelium. Lab Invest 2011; 91:1396 - 409; http://dx.doi.org/10.1038/labinvest.2011.73; PMID: 21606925
- Sheth P, Delos Santos N, Seth A, LaRusso NF, Rao RK. Lipopolysaccharide disrupts tight junctions in cholangiocyte monolayers by a c-Src-, TLR4-, and LBP-dependent mechanism. Am J Physiol Gastrointest Liver Physiol 2007; 293:G308 - 18; http://dx.doi.org/10.1152/ajpgi.00582.2006; PMID: 17446308
- Hanada S, Harada M, Koga H, Kawaguchi T, Taniguchi E, Kumashiro R, et al. Tumor necrosis factor-alpha and interferon-gamma directly impair epithelial barrier function in cultured mouse cholangiocytes. Liver Int 2003; 23:3 - 11; http://dx.doi.org/10.1034/j.1600-0676.2003.01707.x; PMID: 12640721
- Harnois DM, Que FG, Celli A, LaRusso NF, Gores GJ. Bcl-2 is overexpressed and alters the threshold for apoptosis in a cholangiocarcinoma cell line. Hepatology 1997; 26:884 - 90; http://dx.doi.org/10.1002/hep.510260413; PMID: 9328309
- Fukushima K, Ueno Y, Inoue J, Kanno N, Shimosegawa T. Filopodia formation via a specific Eph family member and PI3K in immortalized cholangiocytes. Am J Physiol Gastrointest Liver Physiol 2006; 291:G812 - 9; http://dx.doi.org/10.1152/ajpgi.00250.2005; PMID: 16782697
- Mitic LL, Van Itallie CM, Anderson JM. Molecular physiology and pathophysiology of tight junctions I. Tight junction structure and function: lessons from mutant animals and proteins. Am J Physiol Gastrointest Liver Physiol 2000; 279:G250 - 4; PMID: 10915631
- Van Itallie CM, Anderson JM. Claudins and epithelial paracellular transport. Annu Rev Physiol 2006; 68:403 - 29; http://dx.doi.org/10.1146/annurev.physiol.68.040104.131404; PMID: 16460278
- Mitic LL, Anderson JM. Molecular architecture of tight junctions. Annu Rev Physiol 1998; 60:121 - 42; http://dx.doi.org/10.1146/annurev.physiol.60.1.121; PMID: 9558457
- Anderson JM, Van Itallie CM. Physiology and function of the tight junction. Cold Spring Harb Perspect Biol 2009; 1:a002584; http://dx.doi.org/10.1101/cshperspect.a002584; PMID: 20066090
- Madara JL. Maintenance of the macromolecular barrier at cell extrusion sites in intestinal epithelium: physiological rearrangement of tight junctions. J Membr Biol 1990; 116:177 - 84; http://dx.doi.org/10.1007/BF01868675; PMID: 2380981
- Ulluwishewa D, Anderson RC, McNabb WC, Moughan PJ, Wells JM, Roy NC. Regulation of tight junction permeability by intestinal bacteria and dietary components. J Nutr 2011; 141:769 - 76; http://dx.doi.org/10.3945/jn.110.135657; PMID: 21430248
- Utech M, Mennigen R, Bruewer M. Endocytosis and recycling of tight junction proteins in inflammation. J Biomed Biotechnol 2010; 2010:484987; http://dx.doi.org/10.1155/2010/484987; PMID: 20011071
- Edelblum KL, Turner JR. The tight junction in inflammatory disease: communication breakdown. Curr Opin Pharmacol 2009; 9:715 - 20; http://dx.doi.org/10.1016/j.coph.2009.06.022; PMID: 19632896
- Amasheh M, Andres S, Amasheh S, Fromm M, Schulzke JD. Barrier effects of nutritional factors. Ann N Y Acad Sci 2009; 1165:267 - 73; http://dx.doi.org/10.1111/j.1749-6632.2009.04063.x; PMID: 19538315
- Seth A, Basuroy S, Sheth P, Rao RK. L-Glutamine ameliorates acetaldehyde-induced increase in paracellular permeability in Caco-2 cell monolayer. Am J Physiol Gastrointest Liver Physiol 2004; 287:G510 - 7; http://dx.doi.org/10.1152/ajpgi.00058.2004; PMID: 15331350
- Ahrne S, Hagslatt ML. Effect of lactobacilli on paracellular permeability in the gut. Nutrients 2011; 3:104 - 17; http://dx.doi.org/10.3390/nu3010104; PMID: 22254077
- Basuroy S, Seth A, Elias B, Naren AP, Rao R. MAPK interacts with occludin and mediates EGF-induced prevention of tight junction disruption by hydrogen peroxide. Biochem J 2006; 393:69 - 77; http://dx.doi.org/10.1042/BJ20050959; PMID: 16134968
- Seth A, Yan F, Polk DB, Rao RK. Probiotics ameliorate the hydrogen peroxide-induced epithelial barrier disruption by a PKC- and MAP kinase-dependent mechanism. Am J Physiol Gastrointest Liver Physiol 2008; 294:G1060 - 9; http://dx.doi.org/10.1152/ajpgi.00202.2007; PMID: 18292183
- Dörfel MJ, Huber O. Modulation of tight junction structure and function by kinases and phosphatases targeting occludin. J Biomed Biotechnol 2012; 2012:807356; http://dx.doi.org/10.1155/2012/807356; PMID: 22315516
- Elias BC, Suzuki T, Seth A, Giorgianni F, Kale G, Shen L, et al. Phosphorylation of Tyr-398 and Tyr-402 in occludin prevents its interaction with ZO-1 and destabilizes its assembly at the tight junctions. J Biol Chem 2009; 284:1559 - 69; http://dx.doi.org/10.1074/jbc.M804783200; PMID: 19017651
- Rao R. Occludin phosphorylation in regulation of epithelial tight junctions. Ann N Y Acad Sci 2009; 1165:62 - 8; http://dx.doi.org/10.1111/j.1749-6632.2009.04054.x; PMID: 19538289
- Suzuki T, Elias BC, Seth A, Shen L, Turner JR, Giorgianni F, et al. PKC eta regulates occludin phosphorylation and epithelial tight junction integrity. Proc Natl Acad Sci U S A 2009; 106:61 - 6; http://dx.doi.org/10.1073/pnas.0802741106; PMID: 19114660
- De Vos R, Desmet VJ. Morphologic changes of the junctional complex of the hepatocytes in rat liver after bile duct ligation. Br J Exp Pathol 1978; 59:220 - 7; PMID: 656322
- Metz J, Aoki A, Merlo M, Forssmann WG. Morphological alterations and functional changes of interhepatocellular junctions induced by bile duct ligation. Cell Tissue Res 1977; 182:299 - 310; http://dx.doi.org/10.1007/BF00219766; PMID: 922807
- Anderson JM, Glade JL, Stevenson BR, Boyer JL, Mooseker MS. Hepatic immunohistochemical localization of the tight junction protein ZO-1 in rat models of cholestasis. Am J Pathol 1989; 134:1055 - 62; PMID: 2719075
- Fallon MB, Brecher AR, Balda MS, Matter K, Anderson JM. Altered hepatic localization and expression of occludin after common bile duct ligation. Am J Physiol 1995; 269:C1057 - 62; PMID: 7485446
- Kawaguchi T, Sakisaka S, Sata M, Mori M, Tanikawa K. Different lobular distributions of altered hepatocyte tight junctions in rat models of intrahepatic and extrahepatic cholestasis. Hepatology 1999; 29:205 - 16; http://dx.doi.org/10.1002/hep.510290115; PMID: 9862868
- Takakuwa Y, Kokai Y, Sasaki K, Chiba H, Tobioka H, Mori M, et al. Bile canalicular barrier function and expression of tight-junctional molecules in rat hepatocytes during common bile duct ligation. Cell Tissue Res 2002; 307:181 - 9; http://dx.doi.org/10.1007/s00441-001-0489-4; PMID: 11845325
- Cheung ID, Bagnat M, Ma TP, Datta A, Evason K, Moore JC, et al. Regulation of intrahepatic biliary duct morphogenesis by Claudin 15-like b. Dev Biol 2012; 361:68 - 78; http://dx.doi.org/10.1016/j.ydbio.2011.10.004; PMID: 22020048
- Patonai A, Erdélyi-Belle B, Korompay A, Somorácz A, Straub BK, Schirmacher P, et al. Claudins and tricellulin in fibrolamellar hepatocellular carcinoma. Virchows Arch 2011; 458:679 - 88; http://dx.doi.org/10.1007/s00428-011-1077-y; PMID: 21503766
- Shinozaki A, Shibahara J, Noda N, Tanaka M, Aoki T, Kokudo N, et al. Claudin-18 in biliary neoplasms. Its significance in the classification of intrahepatic cholangiocarcinoma. Virchows Arch 2011; 459:73 - 80; http://dx.doi.org/10.1007/s00428-011-1092-z; PMID: 21607649
- Bunthot S, Obchoei S, Kraiklang R, Pirojkul C, Wongkham S, Wongkham C. Overexpression of claudin-4 in cholangiocarcinoma tissues and its possible role in tumor metastasis. Asian Pac J Cancer Prev 2012; 13:Suppl 71 - 6; PMID: 23480768
- Grosse B, Cassio D, Yousef N, Bernardo C, Jacquemin E, Gonzales E. Claudin-1 involved in neonatal ichthyosis sclerosing cholangitis syndrome regulates hepatic paracellular permeability. Hepatology 2012; 55:1249 - 59; http://dx.doi.org/10.1002/hep.24761; PMID: 22030598
- Seth A, Sheth P, Elias BC, Rao R. Protein phosphatases 2A and 1 interact with occludin and negatively regulate the assembly of tight junctions in the CACO-2 cell monolayer. J Biol Chem 2007; 282:11487 - 98; http://dx.doi.org/10.1074/jbc.M610597200; PMID: 17298946
- Sheth P, Samak G, Shull JA, Seth A, Rao R. Protein phosphatase 2A plays a role in hydrogen peroxide-induced disruption of tight junctions in Caco-2 cell monolayers. Biochem J 2009; 421:59 - 70; http://dx.doi.org/10.1042/BJ20081951; PMID: 19356149
- Rahner C, Stieger B, Landmann L. Structure-function correlation of tight junctional impairment after intrahepatic and extrahepatic cholestasis in rat liver. Gastroenterology 1996; 110:1564 - 78; http://dx.doi.org/10.1053/gast.1996.v110.pm8613064; PMID: 8613064
- Curtis LR, Hoyt D. Impaired biliary excretion of taurocholate associated with increased biliary tree permeability in mirex- or chlordecone-pretreated rats. J Pharmacol Exp Ther 1984; 231:495 - 501; PMID: 6209385
- Kossor DC, Meunier PC, Handler JA, Sozio RS, Goldstein RS. Temporal relationship of changes in hepatobiliary function and morphology in rats following alpha-naphthylisothiocyanate (ANIT) administration. Toxicol Appl Pharmacol 1993; 119:108 - 14; http://dx.doi.org/10.1006/taap.1993.1049; PMID: 8470113
- Ballatori N, Truong AT. Cholestasis, altered junctional permeability, and inverse changes in sinusoidal and biliary glutathione release by vasopressin and epinephrine. Mol Pharmacol 1990; 38:64 - 71; PMID: 2115113
- McGill JM, Gettys TW, Basavappa S, Fitz JG. GTP-binding proteins regulate high conductance anion channels in rat bile duct epithelial cells. J Membr Biol 1993; 133:253 - 61; http://dx.doi.org/10.1007/BF00232024; PMID: 7687295
- De Vos R, Desmet V. Morphology of liver cell tight junctions in ethinyl estradiol induced cholestasis. Pathol Res Pract 1981; 171:381 - 8; http://dx.doi.org/10.1016/S0344-0338(81)80111-2; PMID: 7279787
- Fallon MB, Mennone A, Anderson JM. Altered expression and localization of the tight junction protein ZO-1 after common bile duct ligation. Am J Physiol 1993; 264:C1439 - 47; PMID: 8333499
- Zhang M, Wang XQ, Zhou YK, Ma YL, Shen TY, Chen HQ, et al. Effects of oral Lactobacillus plantarum on hepatocyte tight junction structure and function in rats with obstructive jaundice. Mol Biol Rep 2010; 37:2989 - 99; http://dx.doi.org/10.1007/s11033-009-9866-y; PMID: 19816788
- Maly IP, Landmann L. Bile duct ligation in the rat causes upregulation of ZO-2 and decreased colocalization of claudins with ZO-1 and occludin. Histochem Cell Biol 2008; 129:289 - 99; http://dx.doi.org/10.1007/s00418-007-0374-7; PMID: 18197414
- Benedicto I, Molina-Jiménez F, Barreiro O, Maldonado-Rodríguez A, Prieto J, Moreno-Otero R, et al. Hepatitis C virus envelope components alter localization of hepatocyte tight junction-associated proteins and promote occludin retention in the endoplasmic reticulum. Hepatology 2008; 48:1044 - 53; http://dx.doi.org/10.1002/hep.22465; PMID: 18802961
- Németh Z, Szász AM, Somorácz A, Tátrai P, Németh J, Gyorffy H, et al. Zonula occludens-1, occludin, and E-cadherin protein expression in biliary tract cancers. Pathol Oncol Res 2009; 15:533 - 9; http://dx.doi.org/10.1007/s12253-009-9150-4; PMID: 19184677
- Basuroy S, Sheth P, Kuppuswamy D, Balasubramanian S, Ray RM, Rao RK. Expression of kinase-inactive c-Src delays oxidative stress-induced disassembly and accelerates calcium-mediated reassembly of tight junctions in the Caco-2 cell monolayer. J Biol Chem 2003; 278:11916 - 24; http://dx.doi.org/10.1074/jbc.M211710200; PMID: 12547828
- Andreeva AY, Krause E, Müller EC, Blasig IE, Utepbergenov DI. Protein kinase C regulates the phosphorylation and cellular localization of occludin. J Biol Chem 2001; 276:38480 - 6; http://dx.doi.org/10.1074/jbc.M104923200; PMID: 11502742
- Banan A, Zhang LJ, Shaikh M, Fields JZ, Farhadi A, Keshavarzian A. Theta-isoform of PKC is required for alterations in cytoskeletal dynamics and barrier permeability in intestinal epithelium: a novel function for PKC-theta. Am J Physiol Cell Physiol 2004; 287:C218 - 34; http://dx.doi.org/10.1152/ajpcell.00575.2003; PMID: 14985240
- Mullin JM, Kampherstein JA, Laughlin KV, Clarkin CE, Miller RD, Szallasi Z, et al. Overexpression of protein kinase C-delta increases tight junction permeability in LLC-PK1 epithelia. Am J Physiol 1998; 275:C544 - 54; PMID: 9688609
- Nunbhakdi-Craig V, Machleidt T, Ogris E, Bellotto D, White CL 3rd, Sontag E. Protein phosphatase 2A associates with and regulates atypical PKC and the epithelial tight junction complex. J Cell Biol 2002; 158:967 - 78; http://dx.doi.org/10.1083/jcb.200206114; PMID: 12196510
- Turner JR. ‘Putting the squeeze’ on the tight junction: understanding cytoskeletal regulation. Semin Cell Dev Biol 2000; 11:301 - 8; http://dx.doi.org/10.1006/scdb.2000.0180; PMID: 10966864
- Cunningham KE, Turner JR. Myosin light chain kinase: pulling the strings of epithelial tight junction function. Ann N Y Acad Sci 2012; 1258:34 - 42; http://dx.doi.org/10.1111/j.1749-6632.2012.06526.x; PMID: 22731713
- Terry S, Nie M, Matter K, Balda MS. Rho signaling and tight junction functions. Physiology (Bethesda) 2010; 25:16 - 26; http://dx.doi.org/10.1152/physiol.00034.2009; PMID: 20134025
- Leve F, de Souza W, Morgado-Díaz JA. A cross-link between protein kinase A and Rho-family GTPases signaling mediates cell-cell adhesion and actin cytoskeleton organization in epithelial cancer cells. J Pharmacol Exp Ther 2008; 327:777 - 88; http://dx.doi.org/10.1124/jpet.108.140798; PMID: 18791066
- Kawedia JD, Jiang M, Kulkarni A, Waechter HE, Matlin KS, Pauletti GM, et al. The protein kinase A pathway contributes to Hg2+-induced alterations in phosphorylation and subcellular distribution of occludin associated with increased tight junction permeability of salivary epithelial cell monolayers. J Pharmacol Exp Ther 2008; 326:829 - 37; http://dx.doi.org/10.1124/jpet.107.135798; PMID: 18550693
- Köhler K, Louvard D, Zahraoui A. Rab13 regulates PKA signaling during tight junction assembly. J Cell Biol 2004; 165:175 - 80; http://dx.doi.org/10.1083/jcb.200312118; PMID: 15096524
- Mruk DD, Lau AS, Conway AM. Crosstalk between Rab GTPases and cell junctions. Contraception 2005; 72:280 - 90; http://dx.doi.org/10.1016/j.contraception.2005.03.013; PMID: 16181972
- Köhler K, Zahraoui A. Tight junction: a co-ordinator of cell signalling and membrane trafficking. Biol Cell 2005; 97:659 - 65; http://dx.doi.org/10.1042/BC20040147; PMID: 16033326
- Stevenson BR, Keon BH. The tight junction: morphology to molecules. Annu Rev Cell Dev Biol 1998; 14:89 - 109; http://dx.doi.org/10.1146/annurev.cellbio.14.1.89; PMID: 9891779
- Ivanov AI, Samarin SN, Bachar M, Parkos CA, Nusrat A. Protein kinase C activation disrupts epithelial apical junctions via ROCK-II dependent stimulation of actomyosin contractility. BMC Cell Biol 2009; 10:36; http://dx.doi.org/10.1186/1471-2121-10-36; PMID: 19422706
- Samarin SN, Ivanov AI, Flatau G, Parkos CA, Nusrat A. Rho/Rho-associated kinase-II signaling mediates disassembly of epithelial apical junctions. Mol Biol Cell 2007; 18:3429 - 39; http://dx.doi.org/10.1091/mbc.E07-04-0315; PMID: 17596509
- Walsh SV, Hopkins AM, Chen J, Narumiya S, Parkos CA, Nusrat A. Rho kinase regulates tight junction function and is necessary for tight junction assembly in polarized intestinal epithelia. Gastroenterology 2001; 121:566 - 79; http://dx.doi.org/10.1053/gast.2001.27060; PMID: 11522741
- González-Mariscal L, Tapia R, Chamorro D. Crosstalk of tight junction components with signaling pathways. Biochim Biophys Acta 2008; 1778:729 - 56; http://dx.doi.org/10.1016/j.bbamem.2007.08.018; PMID: 17950242
- Luedde T, Heinrichsdorff J, de Lorenzi R, De Vos R, Roskams T, Pasparakis M. IKK1 and IKK2 cooperate to maintain bile duct integrity in the liver. Proc Natl Acad Sci U S A 2008; 105:9733 - 8; http://dx.doi.org/10.1073/pnas.0800198105; PMID: 18606991
- Atkinson KJ, Rao RK. Role of protein tyrosine phosphorylation in acetaldehyde-induced disruption of epithelial tight junctions. Am J Physiol Gastrointest Liver Physiol 2001; 280:G1280 - 8; PMID: 11352822
- Basuroy S, Sheth P, Kuppuswamy D, Balasubramanian S, Ray RM, Rao RK. Expression of kinase-inactive c-Src delays oxidative stress-induced disassembly and accelerates calcium-mediated reassembly of tight junctions in the Caco-2 cell monolayer. J Biol Chem 2003; 278:11916 - 24; http://dx.doi.org/10.1074/jbc.M211710200; PMID: 12547828
- Kale G, Naren AP, Sheth P, Rao RK. Tyrosine phosphorylation of occludin attenuates its interactions with ZO-1, ZO-2, and ZO-3. Biochem Biophys Res Commun 2003; 302:324 - 9; http://dx.doi.org/10.1016/S0006-291X(03)00167-0; PMID: 12604349
- Sakakibara A, Furuse M, Saitou M, Ando-Akatsuka Y, Tsukita S. Possible involvement of phosphorylation of occludin in tight junction formation. J Cell Biol 1997; 137:1393 - 401; http://dx.doi.org/10.1083/jcb.137.6.1393; PMID: 9182670
- Suzuki T, Seth A, Rao R. Role of phospholipase Cgamma-induced activation of protein kinase Cepsilon (PKCepsilon) and PKCbetaI in epidermal growth factor-mediated protection of tight junctions from acetaldehyde in Caco-2 cell monolayers. J Biol Chem 2008; 283:3574 - 83; http://dx.doi.org/10.1074/jbc.M709141200; PMID: 17991733
- Lazaridis KN, Strazzabosco M, Larusso NF. The cholangiopathies: disorders of biliary epithelia. Gastroenterology 2004; 127:1565 - 77; http://dx.doi.org/10.1053/j.gastro.2004.08.006; PMID: 15521023
- Porayko MK, Kondo M, Steers JL. Liver transplantation: late complications of the biliary tract and their management. Semin Liver Dis 1995; 15:139 - 55; http://dx.doi.org/10.1055/s-2007-1007271; PMID: 7660167
- Wiesner RH, Batts KP, Krom RA. Evolving concepts in the diagnosis, pathogenesis, and treatment of chronic hepatic allograft rejection. Liver Transpl Surg 1999; 5:388 - 400; http://dx.doi.org/10.1002/lt.500050519; PMID: 10477840
- Benkoël L, Dodero F, Hardwigsen J, Campan P, Botta-Fridlund D, Lombardo D, et al. Effect of ischemia-reperfusion on bile canalicular F-actin microfilaments in hepatocytes of human liver allograft: image analysis by confocal laser scanning microscopy. Dig Dis Sci 2001; 46:1663 - 7; http://dx.doi.org/10.1023/A:1010693218680; PMID: 11508665
- Brunner SM, Junger H, Ruemmele P, Schnitzbauer AA, Doenecke A, Kirchner GI, et al. Bile duct damage after cold storage of deceased donor livers predicts biliary complications after liver transplantation. J Hepatol 2013; 58:1133 - 9; http://dx.doi.org/10.1016/j.jhep.2012.12.022; PMID: 23321317
- Nakanuma Y, Tsuneyama K, Gershwin ME, Yasoshima M. Pathology and immunopathology of primary biliary cirrhosis with emphasis on bile duct lesions: recent progress. Semin Liver Dis 1995; 15:313 - 28; http://dx.doi.org/10.1055/s-2007-1007284; PMID: 8578317
- Wiesner RH. Current concepts in primary sclerosing cholangitis. Mayo Clin Proc 1994; 69:969 - 82; http://dx.doi.org/10.1016/S0025-6196(12)61822-9; PMID: 7934194
- Anderson JM. Leaky junctions and cholestasis: a tight correlation. Gastroenterology 1996; 110:1662 - 5; http://dx.doi.org/10.1053/gast.1996.v110.agast961662; PMID: 8613080
- Sakisaka S, Kawaguchi T, Taniguchi E, Hanada S, Sasatomi K, Koga H, et al. Alterations in tight junctions differ between primary biliary cirrhosis and primary sclerosing cholangitis. Hepatology 2001; 33:1460 - 8; http://dx.doi.org/10.1053/jhep.2001.25086; PMID: 11391535
- Landmann L. Cholestasis-induced alterations of the trans- and paracellular pathways in rat hepatocytes. Histochem Cell Biol 1995; 103:3 - 9; http://dx.doi.org/10.1007/BF01464469; PMID: 7736278
- Paganelli M, Stephenne X, Gilis A, Jacquemin E, Henrion Caude A, Girard M, et al. Neonatal ichthyosis and sclerosing cholangitis syndrome: extremely variable liver disease severity from claudin-1 deficiency. J Pediatr Gastroenterol Nutr 2011; 53:350 - 4; http://dx.doi.org/10.1097/MPG.0b013e3182169433; PMID: 21865982
- Jakab C, Kiss A, Schaff Z, Szabó Z, Rusvai M, Gálfi P, et al. Claudin-7 protein differentiates canine cholangiocarcinoma from hepatocellular carcinoma. Histol Histopathol 2010; 25:857 - 64; PMID: 20503174
- Németh Z, Szász AM, Somorácz A, Tátrai P, Németh J, Gyorffy H, et al. Zonula occludens-1, occludin, and E-cadherin protein expression in biliary tract cancers. Pathol Oncol Res 2009; 15:533 - 9; http://dx.doi.org/10.1007/s12253-009-9150-4; PMID: 19184677
- Patonai A, Erdélyi-Belle B, Korompay A, Somorácz A, Straub BK, Schirmacher P, et al. Claudins and tricellulin in fibrolamellar hepatocellular carcinoma. Virchows Arch 2011; 458:679 - 88; http://dx.doi.org/10.1007/s00428-011-1077-y; PMID: 21503766
- Hadj-Rabia S, Baala L, Vabres P, Hamel-Teillac D, Jacquemin E, Fabre M, et al. Claudin-1 gene mutations in neonatal sclerosing cholangitis associated with ichthyosis: a tight junction disease. Gastroenterology 2004; 127:1386 - 90; http://dx.doi.org/10.1053/j.gastro.2004.07.022; PMID: 15521008
- Nagtzaam IF, van Geel M, Driessen A, Steijlen PM, van Steensel MA. Bile duct paucity is part of the neonatal ichthyosis-sclerosing cholangitis phenotype. Br J Dermatol 2010; 163:205 - 7; PMID: 20645982
- Németh Z, Szász AM, Tátrai P, Németh J, Gyorffy H, Somorácz A, et al. Claudin-1, -2, -3, -4, -7, -8, and -10 protein expression in biliary tract cancers. J Histochem Cytochem 2009; 57:113 - 21; http://dx.doi.org/10.1369/jhc.2008.952291; PMID: 18854598
- Brunner SM, Rümmele P, Schlitt HJ, Fichtner-Feigl S. Ischemia-reperfusion injury disrupts architecture of tight junction protein 1 in the common bile duct. Z Gastroenterol 2010; 48:V3_02; http://dx.doi.org/10.1055/s-0029-1246472
- Carlton VE, Harris BZ, Puffenberger EG, Batta AK, Knisely AS, Robinson DL, et al. Complex inheritance of familial hypercholanemia with associated mutations in TJP2 and BAAT. Nat Genet 2003; 34:91 - 6; http://dx.doi.org/10.1038/ng1147; PMID: 12704386
- Laurila JJ, Karttunen T, Koivukangas V, Laurila PA, Syrjälä H, Saarnio J, et al. Tight junction proteins in gallbladder epithelium: different expression in acute acalculous and calculous cholecystitis. J Histochem Cytochem 2007; 55:567 - 73; http://dx.doi.org/10.1369/jhc.6A7155.2007; PMID: 17283368
- Lódi C, Szabó E, Holczbauer A, Batmunkh E, Szíjártó A, Kupcsulik P, et al. Claudin-4 differentiates biliary tract cancers from hepatocellular carcinomas. Mod Pathol 2006; 19:460 - 9; http://dx.doi.org/10.1038/modpathol.3800549; PMID: 16439986
- Shinozaki A, Shibahara J, Noda N, Tanaka M, Aoki T, Kokudo N, et al. Claudin-18 in biliary neoplasms. Its significance in the classification of intrahepatic cholangiocarcinoma. Virchows Arch 2011; 459:73 - 80; http://dx.doi.org/10.1007/s00428-011-1092-z; PMID: 21607649