Abstract
PLEKHA7 is a recently identified protein of the epithelial zonula adhaerens (ZA), and is part of a protein complex that stabilizes the ZA, by linking it to microtubules. Since the ZA is important in the assembly and disassembly of tight junctions (TJ), we asked whether PLEKHA7 is involved in modulating epithelial TJ barrier function. We generated clonal MDCK cell lines in which one of four different constructs of PLEKHA7 was inducibly expressed. All constructs were localized at junctions, but constructs lacking the C-terminal region were also distributed diffusely in the cytoplasm. Inducible expression of PLEKHA7 constructs did not affect the expression and localization of TJ proteins, the steady-state value of transepithelial resistance (TER), the development of TER during the calcium switch, and the flux of large molecules across confluent monolayers. In contrast, expression of three out of four constructs resulted both in enhanced recruitment of E-cadherin and associated proteins at the apical ZA and at lateral puncta adherentia (PA), a decreased TER at 18 h after assembly at normal calcium, and an attenuation in the fall in TER after extracellular calcium removal. This latter effect was inhibited when cells were treated with nocodazole. Immunoprecipitation analysis showed that PLEKHA7 forms a complex with the cytoplasmic TJ proteins ZO-1 and cingulin, and this association does not depend on the integrity of microtubules. These results suggest that PLEKHA7 modulates the dynamics of assembly and disassembly of the TJ barrier, through E-cadherin protein complex- and microtubule-dependent mechanisms.
Introduction
Zonulae occludentes (also called Tight Junctions: TJ) of vertebrate polarized epithelial and endothelial cells are crucial for the establishment and maintenance of barriers between body compartments.Citation1 TJ are topologically associated with zonulae adhaerentes (ZA) in the “apical junctional complex” (AJC) at the apicolateral border of polarized cells.Citation2 TJ and ZA are formed by specific transmembrane proteins (claudins, occludin/tricellulin, JAM-A for TJ, E-cadherin and nectins for ZA) that are linked intracellularly to cytoplasmic adaptor proteins, for example ZO-1, ZO-2, cingulin and polarity complex proteins at TJ, and β-catenin, p120-catenin, α-catenin, and afadin at ZA.Citation3-Citation5 In addition, specific cytoplasmic adaptor proteins of TJ and ZA are directly or indirectly linked to the actomyosin cytoskeleton, which forms a circumferential contractile belt underlying the ZA.Citation6-Citation8
Several lines of evidence show that the assembly and integrity of the ZA is essential for the establishment and maintenance of TJ. Antibodies against the extracellular domain of E-cadherin inhibit the assembly of TJ,Citation9 and studies both on cultured cells and in vivo confirm the crucial role of E-cadherin in the regulation of TJ barrier function.Citation10,Citation11 Modulation of the concentration of extracellular calcium, which controls cadherin-dependent adhesion, results in the modulation of the TJ barrier function, as determined by the measurement of the transepithelial electrical resistance (TER) of cultured cell monolayers in either “calcium switch” or calcium depletion assays.Citation12-Citation14 The circumferential actomyosin belt associated with the ZA is critically important in the physiological and pathological regulation of TJ barrier function,Citation15-Citation19 and disruption of E-cadherin-dependent adhesion affects the integrity of the TJ barrier through phosphorylation signals that control the contractility of the actomyosin cytoskeleton.Citation20
Recent studies have demonstrated that the E-cadherin complex is linked to the microtubule cytoskeleton, through a protein complex containing p120ctn, PLEKHA7, paracingulin (CGNL1) and nezha (CAMSAP3).Citation21-Citation23 Moreover, studies with drugs that inhibit microtubule polymerization show that the integrity of microtubules is required to maintain TJ barrier function in different types of epithelial and endothelial cells.Citation24-Citation26 Microtubules have also been implicated in the perturbation of the TJ barrier by enteric pathogens.Citation27 These observations raise a key question: does the protein complex that connects E-cadherin to microtubules regulate the TJ barrier?
PLEKHA7 links the microtubule cytoskeleton to the ZA, by binding to p120ctn and nezha.Citation21-Citation23 Unlike most other ZA proteins, including E-cadherin, p120ctn, α-catenin, and β-catenin, PLEKHA7 is not localized along the lateral membranes of polarized epithelial cells, but only at the apical circumferential ZA.Citation22 Depletion of the PLEKHA7 complex in Caco2 cells perturbs the organization of the ZA,Citation21 suggesting that it may indirectly affect the stability of the neighboring TJ. However, the role of PLEKHA7 in regulating TJ barrier function has not yet been investigated. PLEKHA7 is expressed in organs, such as kidney and intestine, where modulation of epithelial barrier function is critical for physiology.Citation22 Moreover, its involvement in the pathogenesis of glaucoma may depend on a hypothetical role in controlling the TJ barrier.Citation28 For these reasons, it is important to examine whether PLEKHA7 is implicated in the modulation of the TJ barrier. In the present paper we address this question, by studying the effects of exogenous expression of different PLEKHA7 constructs on the molecular organization and paracellular permeability of TJ of MDCK cells. Our results provide evidence that PLEKHA7 contributes to modulating the dynamic assembly and disassembly of the TJ barrier in a microtubule-dependent manner, through the junctional enrichment of E-cadherin complex proteins.
Results
PLEKHA7 constructs containing either N-terminal or central domains are targeted to junctions
We reasoned that if PLEKHA7 contributes to ZA stability, and ZA integrity affects TJ barrier function, exogenous expression of PLEKHA7 in cultured epithelial cells might influence the paracellular permeability barrier, in experimental models of junction assembly/disassembly induced either by calcium switch or calcium depletion, respectively. To test this hypothesis, we generated stable clones of MDCK-tet-off cells, where expression of exogenous human PLEKHA7 constructs was induced by removal of doxycycline (Dox) from the culture medium ().
Figure 1. Generation of stable MDCK tet-off cell clones with inducible expression of PLEKHA7 constructs. (A) Schematic diagrams showing the putative minimal regions of PLEKHA7 which can interact with afadin (residues 120–374 in human PLEKHA7), p120ctn (538–696), CGNL1 (620–769), or nezha (680–821),Citation21,Citation23,Citation29 and schematic structure of the PLEKHA7 constructs (Full-length FL, N-terminal = N-term., C-terminal = C-term., Central) used to generate stable clones of MDCK tet-off cells. Constructs were tagged N-terminally with YFP, and C-terminally with myc. Putative structural domains are indicated: WW (purple), PH (brown), Pro-rich (P, red), and coiled-coil (CC, blue, CC1 = residues 686–835, and CC2 = residues 1055–1095, based on Phyre2 analysis). Numbers indicate position of PLEKHA7 amino acid residues within constructs. (B) Immunoblotting analysis of lysates of MDCK cell lines (two clones for each construct shown) cultured either in the presence (+) or in the absence (-) of doxycycline, using antibodies against the myc tag (to detect exogenous PLEKHA7), E-cadherin (E-cad.), cingulin (CGN) and tubulin (loading control). Numbers on the right indicate the migration of pre-stained markers (kDa). (C) Confocal immunofluorescent analysis of induced cultures of stable clones showing the localization of exogenous PLEKHA7 constructs (green, YFP) and α-tubulin (red), on the apical plane of focus (ZO-1 labeling is shown for control cells, panel E). Arrows = junctional labeling; arrowheads = cytoplasmic labeling; circle = cytoplasmic dots; n = nuclear labeling. (D) Table summarizing the subcellular localization of each YFP-tagged construct, based on immunofluorescence on paraformaldehyde-fixed cells. (E) Immunofluorescent labeling of control Tet-off cells showing tubulin and ZO-1 in the apical plane of focus. Bar = 10 μm.
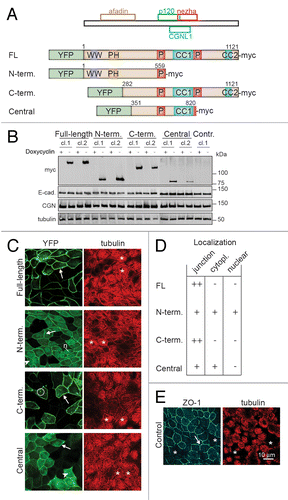
To design the constructs, we took into account the putative regions of PLEKHA7 which have been implicated in the interaction with different protein partners, namely afadin, p120ctn, CGNL1 and nezha (CAMSAP3) ().Citation21,Citation23,Citation29 The Full-length (FL) construct contained all the putative interactor binding sites, the « N-terminal » (N-term.) construct contained the afadin-binding, and part of the p120ctn-interacting sequences. The C-terminal (C-term.) construct contained part of the afadin-binding sequence, and the sequences involved in the interaction with p120ctn, CGNL1 and nezha. The « Central » construct lacked both the afadin-binding region, and additional sequences downstream of the first coiled-coil region, but it comprised the p120ctn, CGNL1, and part of the nezha-binding domains. (). Constructs were tagged N-terminally with YFP, to directly visualize their expression in induced cells ().
Immunoblotting analysis showed that the exogenous proteins were expressed at very low or undetectable levels in the presence of Dox, and were strongly upregulated following Dox removal (). Expression of exogenous PLEKHA7 constructs did not result in detectable changes in the levels of expression of ZA and TJ proteins, including E-cadherin, cingulin (), α-catenin, β-catenin, p120ctn, paracingulin, and ZO-1 (data not shown). Immunofluorescence analysis confirmed that fluorescent exogenous protein was very low or undetectable in cells grown in the presence of Dox (, and data not shown).
Figure 2. Exogenous expression of full-length, N-terminal, C-terminal, but not Central PLEKHA7 constructs enhances ZA recruitment and PA clustering of E-cadherin. Confocal immunofluorescence analysis of clonal lines of MDCK cells showing labeling for exogenous constructs (green) and E-cadherin, either in the apical plane of focus (ZO-1, gray, left panels), which contains ZA (zonula adhaerens), or in the basal plane (nuclei, blue DAPI, right panels), which contains PA (puncta adhaerentia). (A) Full-length, uninduced (FL+DOX); (B) Full-length, induced (FL); (C) N-terminal, induced (N-TERM); (D) C-terminal, induced (C-TERM; (E) Central, induced. Matched arrows indicate matched normal junctional labeling (using ZO-1 as a reference fore the apical plane), and matched arrowheads indicate matched decreased junctional labeling (B, C, D, apical plane) or diffuse lateral labeling (A and E, basal). Within each panel, arrows indicate stronger labeling than arrowheads. The basal plane was typically 10–15 μm below the apical plane. Bar = 10 μm.
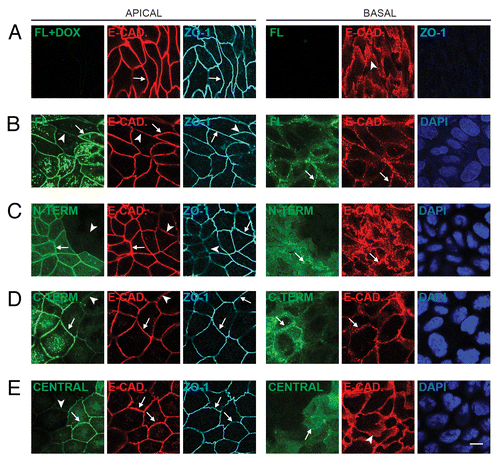
Next, we examined the localization of the exogenous constructs by immunofluorescence. All constructs were targeted to cell-cell junctions (arrows in , and ), indicating that they all contained sequences that are sufficient for the junctional recruitment of PLEKHA7. The Full-length and C-terminal constructs showed a selective accumulation at junctions, and little or no cytoplasmic labeling (). In contrast, the N-terminal and Central constructs showed not only an accumulation at junctions, but also a finely diffuse cytoplasmic labeling (arrowheads in and , see also Figs. S1–S3). In the case of the N-terminal construct, labeling was also detected in the nuclei (« n » in , , see also Figs. S1–S3). Cytoplasmic dots were often detected in cells expressing low/medium amounts of exogenous proteins, and additional granular cytoplasmic labeling was detected in cells expressing high levels of exogenous proteins (circles in Fig. S1, see also Fig. S2, S3), suggesting that exogenous PLEKHA7 proteins may ectopically associate with centrosomes, primary cilia, and the minus ends of cytoplasmic microtubules.
Since PLEKHA7 is part of a complex that is associated with microtubules, we asked whether the organization of microtubules is affected by exogenous expression of PLEKHA7 constructs. Tubulin staining in confluent MDCK monolayers was concentrated in the apical plane of focus, containing the TJ marker ZO-1 (arrow in ), whereas it was much weaker in the sub-apical and basal planes, below ZO-1 (asterisks in , see also Fig. S3). In cells expressing exogenous PLEKHA7 constructs, we selected regions of the monolayer where neighboring cells expressed different levels of exogenous protein, to directly compare tubulin staining. No difference was observed in the distribution of microtubule labeling between cells expressing either low or high amounts of exogenous PLEKHA7 constructs, either under normal conditions (matched asterisks in ) or after treatment with nocodazole (Fig. S3). This indicated that PLEKHA7 exogenous proteins did not induce a detectable reorganization of the microtubule cytoskeleton, nor did they induce an accumulation of ectopic microtubules along cell-cell junctions.
Exogenous expression of full-length, N-terminal and C-terminal, but not central PLEKHA7 constructs enhances the zonular accumulation and lateral clustering of E-cadherin complex proteins
We next examined whether expression of exogenous constructs of PLEKHA7 modified the distribution of proteins of the cadherin complex, which includes E-cadherin, p120-ctn, and β-catenin. All of these proteins are localized in polarized epithelial cells at two distinct localizations: at the apical circumferential belt (zonula adhaerens, ZA), where endogenous PLEKHA7 is also detected, and at the lateral puncta adhaerentia (PA), where PLEKHA7 is not detected.Citation22 We examined regions of the monolayer where neighboring cells expressed different levels of exogenous protein, to ask whether increased exogenous (PLEKHA7) protein correlated with increased labeling for either E-cadherin, p120ctn, and β-catenin. Images were taken both at the apical, zonular ZA plane, using ZO-1 as a marker (, apical), and at a more basal plane which did not contain ZO-1, but contained prominent nuclear labeling (, basal). In confluent cultures grown in the presence of Dox, which inhibits transgene expression, no labeling either for the full-length construct (), or for any of other exogenous constructs (data not shown) was detectable in either plane of focus. E-cadherin labeling in the apical plane was distributed along along circumferential ZA, similar to ZO-1 (arrows in ). In a more basal plane of focus, E-cadherin was distributed diffusely and along discontinuous spots throughout the lateral membrane, corresponding to PA (arrowhead in , basal). Upon induction of expression, labeling for the exogenous proteins was detected not only at cell-cell junctions in the ZA plane of focus, but also at cell-cell contact areas in the basal planes of focus, throughout the lateral membrane (, , basal). This indicated that although PLEKHA7 is localized in normal tissues in situ exclusively at the ZA,Citation22 when exogenously expressed it can also be recruited to lateral PA. Close examination of E-cadherin labeling in the apical plane of focus, using ZO-1 as a marker, clearly showed increased zonular E-cadherin labeling in cells expressing higher levels of exogenous Full-length, N-terminal, and C-terminal constructs (compare arrows/stronger labeling with arrowheads/weaker labeling in , apical). In contrast, no increase of E-cadherin labeling at the ZA was observed in cells expressing the Central construct (, apical). Furthermore, in the basal plane of focus of expressing cells, E-cadherin was no longer distributed diffusely in PA along the lateral membrane, but was accumulated in fewer, more brighly labeled spots, which precisely co-localized with exogenous PLEKHA7 (matching arrows in , basal, see Fig. S1E for a scheme). Again, this lateral clustering effect was observed with the Full-length, N-terminal, and C-terminal constructs (), but not with the Central construct, where cells showed diffuse lateral E-cadherin labeling, similarly to cells grown in the presence of Dox (arrowhead in , basal). Essentially identical observations were made when examining the localization of additional protein components of the E-cadherin complex, namely p120-ctn, α-catenin, and β-catenin: in cells expressing Full-length, N-terminal, and C-terminal, but not Central PLEKHA7 constructs, zonular labeling of the ZA protein was increased, and the more basal, lateral PA labeling was clustered, and co-localized with clustered exogenous PLEKHA7 (Fig. S1A-D and data not shown).
Figure 3. Exogenous expression of full-length PLEKHA7 enhances the ZA recruitment of paracingulin, but not cingulin, ZO-1, occludin and afadin. Confocal immunofluorescence analysis of MDCK cells expressing the Full-length construct cells with labeling for exogenous protein (green) and either paracingulin (CGNL1, (A)), cingulin (CGN, (B)) occludin (OCCL, (D)), or afadin (AFAD), (E)) in red. ZO-1 labeling in triple-stained cells is shown in gray in apical, left panels, and in basal plane (B). See legend to for arrow/arrowheads description. Bar = 10 μm.
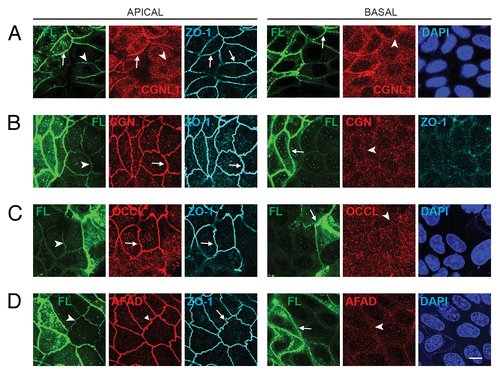
In summary, these observations showed that exogenous expression of all PLEKHA7 constructs, except for the Central construct, results in enhanced ZA recruitment of E-cadherin-complex proteins, and their clustering along the lateral cell-cell contact regions (Fig. S1E).
Exogenous expression of PLEKHA7 constructs does not affect the localization of zonular membrane and cytoplasmic components of the apical junctional complex, with the exception of paracingulin
The ZA, together with the TJ, forms the « apical junctional complex » (AJC), which is characterized by proteins whose distribution is exclusively «zonular », e.g., in a continuous circumferential belt. These include 1) specific TJ and membrane proteins, such as cingulin, occludin and ZO-1; 2) paracingulin, which is localized both at TJ and ZA, and 3) afadin and PLEKHA7, which, unlike most proteins of the E-cadherin complex, are localized at the ZA, but not at lateral PA.Citation22,Citation23 So, we asked whether expression of exogenous PLEKHA7 constructs influenced the junctional recruitment and subcellular localization of zonular AJC proteins.
We found that paracingulin labeling in the apical plane of focus was increased in cells expressing higher levels of exogenous Full-length, N-terminal, and C-terminal, but not Central constructs (, apical, and Fig. S2). Unlike E-cadherin complex proteins, we did not observe a significant increase of lateral paracingulin labeling in cells expressing the Full-length, N-terminal, and C-terminal constructs, indicating that PLEKHA7 does not induce the ectopic lateral recruitment of CGNL1 (, basal, and Fig. S2). In contrast to CGNL1, the zonular labeling for cingulin, ZO-1, occludin, and afadin was not affected by exogenous expression of PLEKHA7 constructs, and no labeling was detected at junctions in more basal planes of focus, where exogenous PLEKHA7 constructs were still detected (, and Fig. S2).
Exogenous expression of PLEKHA7 does not affect either the establishment of the TJ paracellular permeability barrier to ions during the calcium switch, or the barrier to large solutes
The observation that exogenous expression of specific PLEKHA7 constructs promotes the accumulation of E-cadherin complex proteins at the ZA raises the possibility that E-cadherin-dependent modulation of TJ barrier function may be affected in these cells. To test this hypothesis, we first measured the development of the transepithelial electrical resistance (TER) of the different clonal lines in the « calcium switch » assay, where junction re-assembly is rapidly induced by adding calcium to cultures incubated for 16–18 h in the absence of extracellular calcium. In this assay, the TER profile of MDCK cells shows a characteristic peak between 4 and 8 h after the switch.Citation30,Citation31 Clonal lines were assayed either in the presence (uninduced) or absence (induced) of Dox. All clonal lines showed a peak of TER between 4 and 8 h, with a resistance value between 200–400 ohm.cm2. We observed no significant difference when comparing the profiles of each pair of cultures, either in the presence or the absence of Dox (). Immunofluorescent analysis of the localization of ZA and TJ proteins at different times during the calcium switch confirmed that there was no detectable difference in the dynamics of junction assembly, whether the exogenous PLEKHA proteins were expressed or not (data not shown).
Figure 4. Expression of exogenous PLEKHA7 constructs does not affect TER development during the calcium switch in MDCK cells. Transepithelial electrical resistance (TER, ohm.cm2) profiles of representative clonal lines of MDCK cells expressing YFP (Control) or YFP-tagged constructs of PLEKHA7 (see legends), either in the absence (red) or presence (blue) of doxycycline, during the calcium switch.
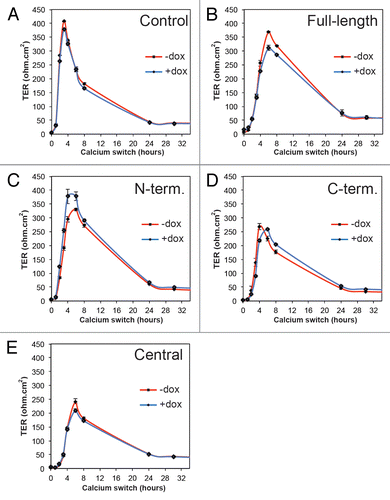
Next, we asked whether the barrier to large solutes is affected in cells expressing exogenous PLEKHA7 constructs, by measuring the flux of fluorescently labeled dextran. In normal calcium medium all clones showed a very low permeability to dextran, which was similar to that of control cells, and was not dependent, for each clone, on whether the exogenous protein was repressed or induced (plus or minus Dox) (). One hour after calcium removal, the flux was increased 10-fold to 30-fold, depending on the clone, and independently of the presence of Dox ().
Figure 5. Exogenous expression of PLEKHA7 constructs does not affect paracellular permeability to large solutes. Histogram showing paracellular permeability to 3 kDa dextran of clonal MDCK lines expressing PLEKHA7 constructs, and control MDCK Tet-off cells, either at normal extracellular calcium concentration (NC, value at 2 h), or after extracellular calcium depletion (LC, value at 30 min after calcium depletion), either in the presence (+DOX, uninduced) or in the absence (-DOX, induced) of doxycycline.
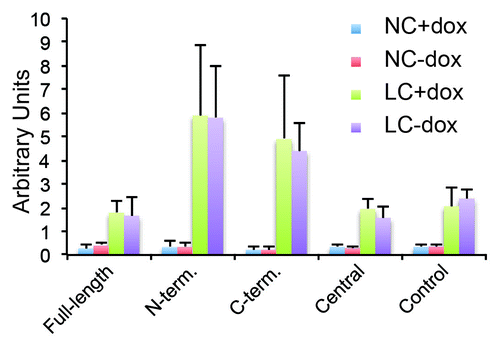
Taken together, these observations show that inducible expression of exogenous constructs of PLEKHA7 does not significantly affect either the establishment of the TJ barrier to ions in the calcium switch, or the flux to large molecules in confluent monolayers at steady-state.
Exogenous expression of specific PLEKHA7 constructs affects the dynamics of TJ barrier establishment at normal calcium, and protects from disruption of the TJ barrier by extracellular calcium removal in a microtubule-dependent manner
When junctions are assembled during the calcium switch, assembly is rapid, because it occurs from a pool of pre-synthesized junctional proteins, which become stabilized at the cell membrane with fast kinetics, following calcium readdition. However, junction assembly can also be obtained following trypsinization of monolayers and assembly at normal calcium (NC). This assembly is slower, since it requires the synthesis and junctional delivery of protein components of junctions. To study the effect of exogenous expression of PLEKHA7 constructs on junction assembly at NC, clonal cell lines grown either in the presence, or in the absence of Dox were trypsinized, and seeded into transwells at confluent density in NC medium, and the TER was measured 18 h later. The TER value of clones expressing the full-length, N-terminal, and C-terminal constructs, but not the Central construct, was significantly lower than the values of uninduced cells (), suggesting that expression of these exogenous proteins perturbs the development of the TJ barrier at normal calcium. When the TER was measured at steady-state, e.g., 48 h or later after the beginning of junction assembly at normal calcium, all clones showed a similar TER, and within each clone there was no difference between the uninduced and induced cells (), confirming that exogenous expression of PLEKHA7 contructs does not affect TER at steady-state.
Figure 6. Exogenous expression of Full-length, N-terminal and C-terminal but not Central PLEKHA7 constructs attenuates barrier disruption by extracellular calcium removal, in a microtubule-dependent manner. (A) TER values (expressed as % of value of uninduced, +dox cultures) of clonal lines of MDCK cells after trypsinization and incubation of confluent monolayers at normal extracellular calcium for either 18 h (NC + dox 18hr, NC-dox 18hr) or 64 h (NC + dox 64hr, NC-dox 64hr). The values at 64 h were the average of two experiments. (B) TER values (expressed as % of value after overnight incubation in NC) of clonal lines of MDCK cells under different conditions: normal calcium (NC) or 30 min low calcium treatment (LC), uninduced or induced (+dox, -dox), treated or untreated with nocodazole (DMSO, noco). For cultures maintained at normal calcium, the TER values were expressed as % of the value before treatment with either DMSO or nocodazole. For cultures undergoing calcium depletion (only the 30 min time point is shown), the TER values were expressed as % of value at time 0, after treatment with either DMSO or nocodazole, and just prior to calcium removal. * = P < 0.05.
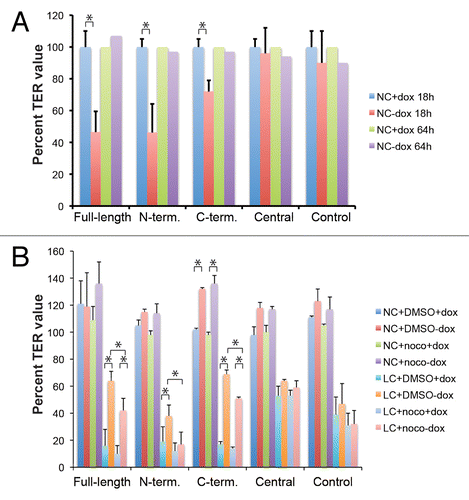
Next, we used the « calcium depletion » assay to examine whether exogenous expression of PLEKHA7 constructs can stabilize cadherin-based complexes, and thus partially protect the TJ barrier from disruption following extracellular calcium removal. Removal of extracellular calcium results in the inhibition of homophylic cadherin-mediated adhesion, and subsequent actomyosin cytoskeleton-mediated opening of the TJ.Citation14,Citation20 To explore the role of microtubule integrity in this assay, we studied the behavior of monolayers treated either with nocodazole, or with vehicle (DMSO). Following this treatment, cultures were incubated for 30 min either in medium with normal calcium (NC, with either DMSO or nocodazole), or with low calcium medium (LC, with either DMSO or nocodazole), this latter to disassemble junctions. Incubation of uninduced cultures in LC medium and DMSO resulted in a fall in TER, to 10–20% of initial values (, LC+DMSO+dox). However, in induced clones expressing either Full-length, N-terminal, or C-terminal constructs, the TER was significantly higher after calcium removal (, LC+DMSO-dox). When nocodazole was added to the cultures, it did not modify significantly the size of the fall in TER in the presence of Dox (, LC+noco+dox). However, in the absence of Dox, where the TER was higher due to transgene expression, addition of nocodazole resulted in a significant fall in TER, down to levels similar to those of uninduced cultures, in cells expressing either the Full-length, N-terminal, or C-terminal constructs (, LC+noco-dox). In contrast, lines expressing the Central construct did not display significant changes in the size of the fall in TER, with or without induction, or with or without nocodazole, when compared with Control cells. These results indicate that exogenous expression of PLEKHA7 constructs containing either all the N-terminal or all the C-terminal regions protects epithelial monolayers from TJ barrier disruption following extracellular calcium removal, and this protective effect is dependent upon the integrity of microtubules.
To further understand the cellular basis of the phenotype, we examined by immunofluorescence the effect of nocodazole on the organization of microtubules and the distribution of exogenous PLEKHA7 constructs. Microtubules were abundant in the apical plane of focus and scarce in the basal plane, and we could not detect any major change in their organization when comparing control cells, and cells expressing different levels of exogenous PLEKHA7 proteins (Fig. S3, see also ). Nocodazole treatment resulted in an overall decrease in tubulin labeling, a decrease in the number of microtubules, and the appearance of fewer, more brightly stained microtubules, detectable especially in the apical plane of focus (Fig. S3). No significant difference could be observed between control cells, and cells expressing exogenous PLEKHA7 proteins. Treatment with nocodazole did not affect the junctional labeling for the exogenous PLEKHA7 constructs, except for a fragmentation of junctional labeling in the basal plane of focus, which was observed in cells expressing either the N-terminal or the Central constructs (arrow in Fig. S3, N-term). In the case of the Full-length and C-terminal constructs, we observed an increase in the number of cytoplasmic « dots » (circles in Fig. S3, Full-length), which could occasionally be imaged at microtubule ends (magnified inset in Fig. S3, C-term), suggesting that the exogenous proteins may be recruited to the minus ends of the microtubules generated following nocodazole treatment.
PLEKHA7 forms a microtubule-independent complex with TJ proteins
To explore whether PLEKHA7 might modulate TJ barrier function by an interaction with TJ proteins, we asked whether PLEKHA7 forms complexes with TJ proteins. PLEKHA7 is known to interact with paracingulin, which is not exclusively localized at TJ, but also at the ZA.Citation23 However, complex formation between PLEKHA7 and TJ proteins, such as cingulin and ZO-1, has not been reported so far. Immunoblot analysis of PLEKHA7 immunoprecipitates from lysates of intestinal epithelial cells showed that not only paracingulin, but also cingulin and ZO-1, are detected in PLEKHA7 immunoprecipitates (). Next, we asked whether the integrity of microtubules is required for the formation of the complex between PLEKHA7 and TJ proteins, by carrying out immunoprecipitation from lysates of cells treated either with DMSO or nocodazole. We did not detect significant differences in the amount of ZO-1 () and cingulin (not shown) in PLEKHA7 immunoprecipitates, with or without nocodazole, indicating that the integrity of microtubules does not perturb the ability of PLEKHA7 to form a complex with TJ proteins.
Figure 7. PLEKHA7 forms a complex with TJ proteins. (A) Immunoblot analysis of PLEKHA7 immunoprecipitates from lysates of confluent human intestinal colon carcinoma cells (Caco2) with antibodies against PLEKHA7, ZO-1, cingulin and paracingulin. (B) Immunoblot analysis of PLEKHA7 immunoprecipitates, with antibodies against either PLEKHA7 or ZO-1, from lysates of Caco2 cells treated with either DMSO or nocodazole for 1 h prior to lysis. Numbers on the right indicate migration of molecular size markers (kDa).
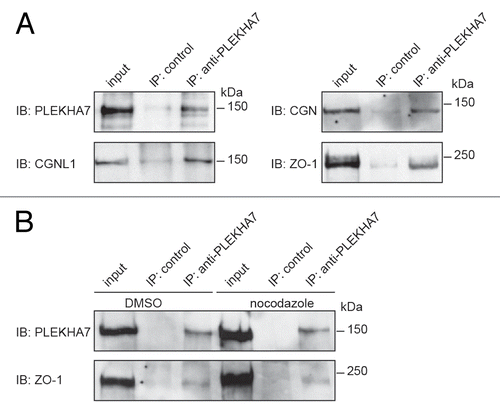
Discussion
Here we provide the first evidence for a role of the ZA protein PLEKHA7 in modulating epithelial TJ barrier function, through a mechanism which we propose to depend on the stabilization of the E-cadherin complex by microtubules. Although exogenous expression of PLEKHA7 constructs in MDCK monolayers did not affect either the development of the TJ barrier to ions during the calcium switch, or the steady-state TER, or the steady-state flux of large solutes, specific contructs slowed TER development at normal calcium, and attenuated the fall in TER of confluent monolayers following extracellular calcium depletion.
PLEKHA7 is a key component of the molecular complex that links the epithelial ZA to the minus ends of non-centrosomal microtubules.Citation21 Non-centrosomal microtubules are abundant in epithelial cells, and are stabilized by capture and anchoring at cortical sites.Citation32 In addition to PLEKHA7, several other junctional/cortical proteins, including desmoplakin, APC, and cingulin have been implicated in microtubule anchoring to epithelial junctions.Citation21,Citation33-Citation35 However, there is so far no evidence that any of the above proteins is involved in regulating the TJ permeability barrier. For example, cingulin depletion or exogenous expression in MDCK cells, and cingulin knockout, either in embryoid bodies or mice, does not result in changes in either the development or the steady-state function of the TJ barrier, and does not affect the organization of the ZA.Citation30,Citation36-Citation38 Although previous studies have shown that a correct organization and anchoring of microtubules is required for the maturation of an efficient TJ barrier,Citation24-Citation26,Citation39 the molecular mechanisms of this regulation were unknown. Our observations identify PLEKHA7 as the first junctional protein, among those involved in microtubule anchoring, whose expression levels can modulate the TJ barrier function.
We observed a correlation between the ability of exogenous PLEKHA7 constructs to enhance the accumulation of E-cadherin complex proteins at the ZA and promote their clustering along lateral PA, with the ability of the same constructs to slow down the development of a normal TER at normal extracellular calcium, and attenuate the fall in TER induced by depletion of extracellular calcium. These observations point to a mechanistic implication of the E-cadherin complex in the phenotypes that we observed. Specifically, the observation that the fall in TER in the calcium depletion model in these clones was enhanced by disrupting microtubule integrity is consistent with the role of PLEKHA7 as a protein that stabilizes the E-cadherin protein complex, through connecting it to microtubules.Citation21 We propose that in cells that express specific exogenous contructs of PLEKHA7, the increase in the amount of E-cadherin complex proteins and PLEKHA7 at the ZA results in strengthened anchoring to the microtubule cytoskeleton, a more stable ZA, and a less dynamic remodeling, delivery and endocytic removal of ZA proteins.Citation26,Citation40,Citation41 Additional studies will be required to test these hypotheses.
Afadin has a key role in the proper formation and actin anchoring of the ZA,Citation42-Citation44 and in TJ assembly and function.Citation45-Citation47 However, since exogenous expression of PLEKHA7 constructs did not affect afadin recruitment at the ZA, we speculate that the effect of PLEKHA7 constructs on the TJ barrier does not occur through afadin. In contrast, the effect of PLEKHA7 constructs on the accumulation of paracingulin confirms the notion that paracingulin is a component of the complex that links microtubules to the ZA, and is recruited by PLEKHA7 to the ZA.Citation23 Finally, although we show that PLEKHA7 is detectable in a complex with specific TJ proteins such as cingulin and ZO-1, the apparent lack of effect of PLEKHA7 constructs on TJ protein organization argues against a direct role of PLEKHA7 in modulating TJ barrier function through actions on the cytoplasmic scaffolding complex of TJ. Therefore, the functional significance of PLEKHA7 interaction with afadin, cingulin, and ZO-1 in the regulation of TJ barrier function remains unclear.
There is an apparent discrepancy in our observation that exogenous expression of PLEKHA7 constructs slowed TER development when junction assembly was performed at normal extracellular calcium, but not when it was performed by the calcium switch. This could be due to the fact that junction assembly by the calcium switch is rapid, and relies on the fusion of endocytic vesicles containing the pre-assembled junctional complexes with the plasma membrane upon calcium re-addition.Citation48,Citation49 Excess PLEKHA7 may not interfere with this process, since the junctional machineries are already pre-assembled, and the actin rather than the microtubule cytoskeleton plays a major role, as indicated by the role of Rac1 in TER development in this model.Citation31 In contrast, junction assembly at normal calcium after trypsinization has slower dynamics, involves de novo protein synthesis, and may require a precise spatial organization of microtubules, to correctly deliver newly synthesized ZA and TJ proteins to the plasma membrane.Citation13,Citation50-Citation52 Exogenous PLEKHA7 proteins appeared to be associated with microtubule minus ends, and future studies should address the role of PLEKHA7 in microtubule dynamics and trafficking. However, since at steady-state all clones showed a similar TER value, regardless of induction of exogenous protein expression, both in assembly by calcium switch and at normal calcium, we conclude that PLEKHA7 exogenous expression only subtly affects the dynamics of the establishment and disruption of the TJ barrier, but not its integrity at steady-state. Preliminary observations on intestinal epithelial cells also show that PLEKHA7 depletion has a modest inhibitory effect on the establishment and maintenance of the TJ barrier (our unpublished observations). The phenotype of PLEKHA7-depleted cells will be investigated in future studies.
Our results also provide new information about structure-function relationships in PLEKHA7. Although afadin was reported to be important for PLEKHA7 junctional recruitment,Citation29 we observed that the Central construct, lacking all of the putative afadin binding sequence, was still targeted to junctions. This would indicate that the interaction with p120ctn, which occurs in the central region, is sufficient for PLEKHA7 recruitment to both ZA and PA. Conversely, the observation that both the N-terminal and Central constructs displayed a cytoplasmic localization suggests that the presence of both afadin-binding and p120ctn/CGNL1/nezha binding regions is essential for a stable association of PLEKHA7 with the ZA. The association of the N-terminal construct with p120ctn is also, presumably, responsible for the E-cadherin enrichment at ZA and PA, since this construct is in principle lacking the CGNL1 and nezha interaction sequences. We cannot exclude that additional protein interactions may help endogenous PLEKHA7 or exogenous constructs to be targeted to ZA and PA, and future studies will address this question.
Our results, providing the first evidence for a role of PLEKHA7 in modulating the dynamics of the TJ barrier, may help to understand the molecular mechanisms through which PLEKHA7 participates in the pathogenesis of primary angle closure glaucomaCitation28,Citation53 and hypertension.Citation54-Citation56 For example, PLEKHA7 expression levels may influence the response of epithelial tissues to physiological or pathological stimuli that modulate the paracellular barrier. Similarly, mutations in the PLEKHA7 locus, which might affect the expression or interactions of PLEKHA7, could influence the barrier function of epithelial cells involved in these diseases, by interfering with the stabilizing role of the E-cadherin complex on the TJ barrier.
In summary, our results show that expression of specific exogenous PLEKHA7 constructs affects the dynamics of assembly and disassembly of the epithelial TJ barrier, and indicate that the E-cadherin protein complex and microtubules are mechanistically implicated in this modulation. These observations provide a framework for future studies on PLEKHA7 function in normal and diseased tissues.
Materials and Methods
Antibodies and plasmids
Antibodies were (species-antigen, dilution for immunofluorescence-IF with methanol fixation unless otherwise stated, and immunoblotting-IB): rabbit PLEKHA7 (1:10,000 IBCitation22), rabbit cingulin (1:5000 IF-MeOH, 1:100 IF-PFA, 1:2000 IBCitation57), rabbit paracingulin (1:250 IF, 1:10,000 IBCitation31), mouse p120ctn (15D2, 1:250 IF, 1:2000 IB, from A. Reynolds), mouse E-cadherin (1:500 IF, 1:2000 IB, BD610181), rabbit α-catenin (1:100 IF, 1:1000 IB, BD610193), rabbit β-catenin (1:200 IF, Sigma C2206), mouse α-tubulin (1:150 IF, 1:1000 IB, Zymed 32–2500), rabbit anti-afadin (1:100 IF, Sigma A0224), rat ZO-1 (1:50 IF, a gift from D. Goodenough, Harvard University), rabbit occludin (1:50 IF, Zymed 71–1500), mouse myc (9E10 hybridoma culture supernatant, undiluted for IB). The constructs for inducible expression of YFP-PLEKHA7-myc were obtained by cloning either the full-length human PLEKHA7 sequence (residues 1–1121), or the truncated constructs (ΔCterm: 1–559, ΔNterm: 282–1121, Central: 351–820) into pBluescript (Xba-HindIII sites, except for Central, BamH1-EcoRI/XhoI), and subsequently into the NotI-ClaI sites of a previously prepared TRE2-Hyg vectorCitation58 where NotI is downstream of a YFP cassette and ClaI upstream of a myc tag.
Cell culture, transfection, and other techniques
Culture of MDCKII (Madin-Darby Canine Kidney) Tet-off epithelial cells (Clontech) and Caco2 cells, transfection, selection, isolation of stable clones, immunoblotting and immunofluorescence (cold methanol fixation) were as described previously.Citation58,Citation59 Stable clones of MDCK-tet-off cells full-length or truncated constructs of PLEKHA7 were incubated either in the presence or in the absence of doxycyclin (40 ng/ml) for 72 h, prior to experimental manipulations. Treatment with nocodazole 1 h at 37 °C was at final concentrations of either 0.2 μM (TER experiments) or 33 μM (immunofluorescence), by dilution from a concentrated stock, in DMSO. Three clonal lines were generated for each construct, and their behavior was similar. Representative examples of experiments performed with one of the three lines is shown.
Calcium switch assay
For the calcium switch assay, 2x104 cells were plated into 6.5 mm Transwell filters (0.2 ml apical compartment, 0.7 ml basal compartment), and allowed to grow for 6 days, changing the medium every 2 days, to reach TER steady-state. The cells were then washed 3x with PBS without Ca, and the medium was replaced with Ca2+-free medium (SMEM+EGTACitation30). Following overnight incubation (16–20 h), the Transepithelial Electrical Resistance (TER) was measured (t = 0), then the medium was replaced with normal calcium medium (calcium-switch), and the TER was measured at different times (1, 2, 3, 4, 8, 24, 30 h) after the switch. TER was meausred using a Millipore ERS-CELL voltohmeter.Citation14
Assembly at normal calcium, and calcium depletion assays
Cells were incubated either with or without dox for 3 days, and then trypsinized and seeded on Transwells filters (3x105 cells per filter). The TER was measured after overnight (18 h) incubation, and at subsequent times (22 h, 26 h, 42 h, 64 h, 90 h), until the TER value reached steady-state (at around 50 h). The average values under induced conditions (-dox) were expressed as a percent of the values under uninduced conditions (+dox), that were taken as 100%. To test the effect of calcium depletion, cells after overnight assembly at normal calcium were incubated either with solvent (DMSO, final 0.01%) or nocodazole (0.2 μM, in DMSO) for 1 h. Next, the TER was measured again (time = 0), and expressed as percentage of the value before the treatment. Cells were then either left in NC medium (with either DMSO or nododazole), or washed 3x with PBS without Ca, and incubated with Ca2+-free medium, containing either DMSO or nocodazole. The TER was then measured 30 min later, and expressed as a percent of the value before incubation with DMSO/nocodazole. The 30 min time point was chosen because at this time TER of MDCK monolayers is typically reduced by > 70% compared with the initial value.Citation14 The TER values at 30 min were ratioed to the TER values at time = 0, to express them as a percent of initial value.
Measurement of paracellular flux
For flux assays, confluent monolayers in duplicate 6.5 mm Transwell filters were cultured in normal medium for 24 h, washed with pre-warmed Hank’s Buffer (Invitrogen 14025), and incubated in Hank’s Buffer for 30 min at 37 °C. The Buffer in the apical chamber was aspirated, and the filter transferred into a new well, containing 0.7 ml Hanks’ Buffer. 50 μl 3 kDa FITC-Dextran (Invitrogen D3305, stock 1 mg/ml) was added to the apical compartment. Cells were incubated at 37 °C for 2 h, and 0.1 ml of the basal compartment solution was removed, and replaced by 0.1 ml Hank’s Buffer. For calcium depletion experiments, after aspiration cells were rinsed 3X with calcium-free PBS, and incubated in calcium-free PBS, containing the tracer. Fluorescence of the basal compartment sample was measured with a spectrophotometer, and the amount of dextran (ng/ml) in each sample was determined by interpolation with a standard curve. The apparent permeability was calculated using the formula: Permapp = (ng/ml basal) x 106/Area well x Initial concentration x Time (sec).
Immunofluorescence
For immunofluorescence analysis, we routinely used a cold methanol fixation protocol.Citation30 For labeling of microtubules, cells were washed twice with PBS, fixed in 4% paraformaldehyde (PFA), 1% Triton X-100 in Microtubule Stabilizing Buffer (MTSB, 1 mM EGTA, 4% PEG8000, 0.1M Pipes, pH 6.9) for 10 min at room temperature (RT), followed by incubation in 4% PFA in MTSB for 30 min RT, washing with PBS, blocking in 10 mM glycine, 1% bovine serum albumin (10 min RT), and washing with PBS. Incubation with primary and secondary antibodies (diluted in PBS) were performed either at 30 °C (45 min) or at 37° (30 min). Secondary antibodies (1:400, Jackson Laboratories) were labeled either with either Cy3 (anti-mouse or anti-rabbit) or Cy5 (anti-rat). Confocal images were acquired using a LSM-700 Zeiss confocal microscope.
Immunoprecipitation and immunoblotting
For immunoprecipitation, cells were lysed in IP lysis buffer (150 mM NaCl, 20 mM TRIS-HCl pH 7.5, 1% NP40, 1 mM EDTA), lysates were clarified by centrifugation (13,000 rpm for 15 min), 20 μl of Dynabeads Protein G (Invitrogen) were washed three times with PBS containing 5% BSA and 1% NP40, antibodies (5 μl of rabbit either anti-PLEKHA7 or preimmune serumCitation22) were incubated for 1 h at 4 °C with Dynabeads, subsequently lysates were incubated overnight at 4 °C and washed with IP lysis buffer. Proteins were separated on 8% polyacrylamide gels, transferred onto nitrocellulose (0. 45 μm) (100 V for 80 min at 4 °C), and blots were incubated with primary antibody, followed by secondary HRP-labeled antibody (1:10,000, Amersham), and chemiluminescence revelation.
Statistical analysis
Unless otherwise stated, TER and flux data represent the mean of at least three separate experiments, each with duplicate wells for each experimental condition. Data were analyzed for statistical significance (P < 0.05) using the Student’s T-test, the comparisons being made each time either between the –Dox (induced) and +Dox (uninduced), or between DMSO vs. nocodazole condition.
Additional material
Download Zip (13.5 MB)Disclosure of Potential Conflicts of Interest
No potential conflicts of interest were disclosed.
Acknowledgments
This study was supported by grants from the Swiss Cancer League (KFS-2813-08-2011) and the Swiss National Science Foundation (31003A_135730/1), and by the State of Geneva. We are grateful to all colleagues who generously provided us with plasmids and reagents.
References
- Powell DW. Barrier function of epithelia. Am J Physiol 1981; 241:G275 - 88; PMID: 7032321
- Farquhar MG, Palade GE. Junctional complexes in various epithelia. J Cell Biol 1963; 17:375 - 412; http://dx.doi.org/10.1083/jcb.17.2.375; PMID: 13944428
- Shin K, Fogg VC, Margolis B. Tight junctions and cell polarity. Annu Rev Cell Dev Biol 2006; 22:207 - 35; http://dx.doi.org/10.1146/annurev.cellbio.22.010305.104219; PMID: 16771626
- Guillemot L, Paschoud S, Pulimeno P, Foglia A, Citi S. The cytoplasmic plaque of tight junctions: a scaffolding and signalling center. Biochim Biophys Acta 2008; 1778:601 - 13; http://dx.doi.org/10.1016/j.bbamem.2007.09.032; PMID: 18339298
- Meng W, Takeichi M. Adherens junction: molecular architecture and regulation. Cold Spring Harb Perspect Biol 2009; 1:a002899; http://dx.doi.org/10.1101/cshperspect.a002899; PMID: 20457565
- Madara JL. Relationships between the tight junction and the cytoskeleton. In: Cereijido M, ed. Tight junctions. Boca Raton, FL: CRC Press, 1992:105-20.
- Mooseker MS. Organization, chemistry, and assembly of the cytoskeletal apparatus of the intestinal brush border. Annu Rev Cell Biol 1985; 1:209 - 41; http://dx.doi.org/10.1146/annurev.cb.01.110185.001233; PMID: 3916317
- Van Itallie CM, Fanning AS, Bridges A, Anderson JM. ZO-1 stabilizes the tight junction solute barrier through coupling to the perijunctional cytoskeleton. Mol Biol Cell 2009; 20:3930 - 40; http://dx.doi.org/10.1091/mbc.E09-04-0320; PMID: 19605556
- Gumbiner B, Simons K. A functional assay for proteins involved in establishing an epithelial occluding barrier: identification of a uvomorulin-like polypeptide. J Cell Biol 1986; 102:457 - 68; http://dx.doi.org/10.1083/jcb.102.2.457; PMID: 3511070
- Tunggal JA, Helfrich I, Schmitz A, Schwarz H, Günzel D, Fromm M, Kemler R, Krieg T, Niessen CM. E-cadherin is essential for in vivo epidermal barrier function by regulating tight junctions. EMBO J 2005; 24:1146 - 56; http://dx.doi.org/10.1038/sj.emboj.7600605; PMID: 15775979
- Capaldo CT, Macara IG. Depletion of E-cadherin disrupts establishment but not maintenance of cell junctions in Madin-Darby canine kidney epithelial cells. Mol Biol Cell 2007; 18:189 - 200; http://dx.doi.org/10.1091/mbc.E06-05-0471; PMID: 17093058
- Martinez-Palomo A, Meza I, Beaty G, Cereijido M. Experimental modulation of occluding junctions in a cultured transporting epithelium. J Cell Biol 1980; 87:736 - 45; http://dx.doi.org/10.1083/jcb.87.3.736; PMID: 6780571
- Gonzalez-Mariscal L, Chávez de Ramírez B, Cereijido M. Tight junction formation in cultured epithelial cells (MDCK). J Membr Biol 1985; 86:113 - 25; http://dx.doi.org/10.1007/BF01870778; PMID: 4032460
- Citi S. Protein kinase inhibitors prevent junction dissociation induced by low extracellular calcium in MDCK epithelial cells. J Cell Biol 1992; 117:169 - 78; http://dx.doi.org/10.1083/jcb.117.1.169; PMID: 1556151
- Turner JR, Rill BK, Carlson SL, Carnes D, Kerner R, Mrsny RJ, Madara JL. Physiological regulation of epithelial tight junctions is associated with myosin light-chain phosphorylation. Am J Physiol 1997; 273:C1378 - 85; PMID: 9357784
- Turner JR, Angle JM, Black ED, Joyal JL, Sacks DB, Madara JL. PKC-dependent regulation of transepithelial resistance: roles of MLC and MLC kinase. Am J Physiol 1999; 277:C554 - 62; PMID: 10484342
- Hecht G, Pestic L, Nikcevic G, Koutsouris A, Tripuraneni J, Lorimer DD, Nowak G, Guerriero V Jr., Elson EL, Lanerolle PD. Expression of the catalytic domain of myosin light chain kinase increases paracellular permeability. Am J Physiol 1996; 271:C1678 - 84; PMID: 8944652
- Tinsley JH, De Lanerolle P, Wilson E, Ma W, Yuan SY. Myosin light chain kinase transference induces myosin light chain activation and endothelial hyperpermeability. Am J Physiol Cell Physiol 2000; 279:C1285 - 9; PMID: 11003609
- Clayburgh DR, Barrett TA, Tang Y, Meddings JB, Van Eldik LJ, Watterson DM, Clarke LL, Mrsny RJ, Turner JR. Epithelial myosin light chain kinase-dependent barrier dysfunction mediates T cell activation-induced diarrhea in vivo. J Clin Invest 2005; 115:2702 - 15; http://dx.doi.org/10.1172/JCI24970; PMID: 16184195
- Citi S, Volberg T, Bershadsky AD, Denisenko N, Geiger B. Cytoskeletal involvement in the modulation of cell-cell junctions by the protein kinase inhibitor H-7. J Cell Sci 1994; 107:683 - 92; PMID: 8006081
- Meng W, Mushika Y, Ichii T, Takeichi M. Anchorage of microtubule minus ends to adherens junctions regulates epithelial cell-cell contacts. Cell 2008; 135:948 - 59; http://dx.doi.org/10.1016/j.cell.2008.09.040; PMID: 19041755
- Pulimeno P, Bauer C, Stutz J, Citi S. PLEKHA7 is an adherens junction protein with a tissue distribution and subcellular localization distinct from ZO-1 and E-cadherin. PLoS One 2010; 5:e12207; http://dx.doi.org/10.1371/journal.pone.0012207; PMID: 20808826
- Pulimeno P, Paschoud S, Citi S. A role for ZO-1 and PLEKHA7 in recruiting paracingulin to tight and adherens junctions of epithelial cells. J Biol Chem 2011; 286:16743 - 50; http://dx.doi.org/10.1074/jbc.M111.230862; PMID: 21454477
- Yap AS, Stevenson BR, Abel KC, Cragoe EJ Jr., Manley SW. Microtubule integrity is necessary for the epithelial barrier function of cultured thyroid cell monolayers. Exp Cell Res 1995; 218:540 - 50; http://dx.doi.org/10.1006/excr.1995.1189; PMID: 7796888
- Birukova AA, Smurova K, Birukov KG, Usatyuk P, Liu F, Kaibuchi K, Ricks-Cord A, Natarajan V, Alieva I, Garcia JG, et al. Microtubule disassembly induces cytoskeletal remodeling and lung vascular barrier dysfunction: role of Rho-dependent mechanisms. J Cell Physiol 2004; 201:55 - 70; http://dx.doi.org/10.1002/jcp.20055; PMID: 15281089
- Ivanov AI, McCall IC, Babbin B, Samarin SN, Nusrat A, Parkos CA. Microtubules regulate disassembly of epithelial apical junctions. BMC Cell Biol 2006; 7:12; http://dx.doi.org/10.1186/1471-2121-7-12; PMID: 16509970
- Tomson FL, Viswanathan VK, Kanack KJ, Kanteti RP, Straub KV, Menet M, Kaper JB, Hecht G. Enteropathogenic Escherichia coli EspG disrupts microtubules and in conjunction with Orf3 enhances perturbation of the tight junction barrier. Mol Microbiol 2005; 56:447 - 64; http://dx.doi.org/10.1111/j.1365-2958.2005.04571.x; PMID: 15813736
- Vithana EN, Khor CC, Qiao C, Nongpiur ME, George R, Chen LJ, Do T, Abu-Amero K, Huang CK, Low S, et al. Genome-wide association analyses identify three new susceptibility loci for primary angle closure glaucoma. Nat Genet 2012; 44:1142 - 6; http://dx.doi.org/10.1038/ng.2390; PMID: 22922875
- Kurita S, Yamada T, Rikitsu E, Ikeda W, Takai Y. Binding between the junctional proteins afadin and PLEKHA7 and implication in the formation of adherens junction in epithelial cells. J Biol Chem 2013; 288:29356 - 68; http://dx.doi.org/10.1074/jbc.M113.453464; PMID: 23990464
- Guillemot L, Citi S. Cingulin regulates claudin-2 expression and cell proliferation through the small GTPase RhoA. Mol Biol Cell 2006; 17:3569 - 77; http://dx.doi.org/10.1091/mbc.E06-02-0122; PMID: 16723500
- Guillemot L, Paschoud S, Jond L, Foglia A, Citi S. Paracingulin regulates the activity of Rac1 and RhoA GTPases by recruiting Tiam1 and GEF-H1 to epithelial junctions. Mol Biol Cell 2008; 19:4442 - 53; http://dx.doi.org/10.1091/mbc.E08-06-0558; PMID: 18653465
- Bartolini F, Gundersen GG. Generation of noncentrosomal microtubule arrays. J Cell Sci 2006; 119:4155 - 63; http://dx.doi.org/10.1242/jcs.03227; PMID: 17038542
- Lechler T, Fuchs E. Desmoplakin: an unexpected regulator of microtubule organization in the epidermis. J Cell Biol 2007; 176:147 - 54; http://dx.doi.org/10.1083/jcb.200609109; PMID: 17227889
- McCartney BM, Näthke IS. Cell regulation by the Apc protein Apc as master regulator of epithelia. Curr Opin Cell Biol 2008; 20:186 - 93; http://dx.doi.org/10.1016/j.ceb.2008.02.001; PMID: 18359618
- Yano T, Matsui T, Tamura A, Uji M, Tsukita S. The association of microtubules with tight junctions is promoted by cingulin phosphorylation by AMPK. J Cell Biol 2013; 203:605 - 14; http://dx.doi.org/10.1083/jcb.201304194; PMID: 24385485
- Guillemot L, Hammar E, Kaister C, Ritz J, Caille D, Jond L, Bauer C, Meda P, Citi S. Disruption of the cingulin gene does not prevent tight junction formation but alters gene expression. J Cell Sci 2004; 117:5245 - 56; http://dx.doi.org/10.1242/jcs.01399; PMID: 15454572
- Guillemot L, Schneider Y, Brun P, Castagliuolo I, Pizzuti D, Martines D, Jond L, Bongiovanni M, Citi S. Cingulin is dispensable for epithelial barrier function and tight junction structure, and plays a role in the control of claudin-2 expression and response to duodenal mucosa injury. J Cell Sci 2012; 125:5005 - 14; http://dx.doi.org/10.1242/jcs.101261; PMID: 22946046
- Paschoud S, Citi S. Inducible overexpression of cingulin in stably transfected MDCK cells does not affect tight junction organization and gene expression. Mol Membr Biol 2008; 25:1 - 13; http://dx.doi.org/10.1080/09687680701474009; PMID: 18097951
- Yap AS, Manley SW. Microtubule integrity is essential for apical polarization and epithelial morphogenesis in the thyroid. Cell Motil Cytoskeleton 2001; 48:201 - 12; http://dx.doi.org/10.1002/1097-0169(200103)48:3<201::AID-CM1009>3.0.CO;2-C; PMID: 11223951
- Rodriguez OC, Schaefer AW, Mandato CA, Forscher P, Bement WM, Waterman-Storer CM. Conserved microtubule-actin interactions in cell movement and morphogenesis. Nat Cell Biol 2003; 5:599 - 609; http://dx.doi.org/10.1038/ncb0703-599; PMID: 12833063
- Birkenfeld J, Nalbant P, Yoon SH, Bokoch GM. Cellular functions of GEF-H1, a microtubule-regulated Rho-GEF: is altered GEF-H1 activity a crucial determinant of disease pathogenesis?. Trends Cell Biol 2008; 18:210 - 9; http://dx.doi.org/10.1016/j.tcb.2008.02.006; PMID: 18394899
- Mandai K, Nakanishi H, Satoh A, Obaishi H, Wada M, Nishioka H, Itoh M, Mizoguchi A, Aoki T, Fujimoto T, et al. Afadin: A novel actin filament-binding protein with one PDZ domain localized at cadherin-based cell-to-cell adherens junction. J Cell Biol 1997; 139:517 - 28; http://dx.doi.org/10.1083/jcb.139.2.517; PMID: 9334353
- Ikeda W, Nakanishi H, Miyoshi J, Mandai K, Ishizaki H, Tanaka M, Togawa A, Takahashi K, Nishioka H, Yoshida H, et al. Afadin: A key molecule essential for structural organization of cell-cell junctions of polarized epithelia during embryogenesis. J Cell Biol 1999; 146:1117 - 32; http://dx.doi.org/10.1083/jcb.146.5.1117; PMID: 10477764
- Sawyer JK, Choi W, Jung KC, He L, Harris NJ, Peifer M. A contractile actomyosin network linked to adherens junctions by Canoe/afadin helps drive convergent extension. Mol Biol Cell 2011; 22:2491 - 508; http://dx.doi.org/10.1091/mbc.E11-05-0411; PMID: 21613546
- Ooshio T, Kobayashi R, Ikeda W, Miyata M, Fukumoto Y, Matsuzawa N, Ogita H, Takai Y. Involvement of the interaction of afadin with ZO-1 in the formation of tight junctions in Madin-Darby canine kidney cells. J Biol Chem 2010; 285:5003 - 12; http://dx.doi.org/10.1074/jbc.M109.043760; PMID: 20008323
- Tanaka-Okamoto M, Hori K, Ishizaki H, Itoh Y, Onishi S, Yonemura S, Takai Y, Miyoshi J. Involvement of afadin in barrier function and homeostasis of mouse intestinal epithelia. J Cell Sci 2011; 124:2231 - 40; http://dx.doi.org/10.1242/jcs.081000; PMID: 21652626
- Birukova AA, Tian X, Tian Y, Higginbotham K, Birukov KG. Rap-afadin axis in control of Rho signaling and endothelial barrier recovery. Mol Biol Cell 2013; 24:2678 - 88; http://dx.doi.org/10.1091/mbc.E13-02-0098; PMID: 23864716
- Kartenbeck J, Schmid E, Franke WW, Geiger B. Different modes of internalization of proteins associated with adhaerens junctions and desmosomes: experimental separation of lateral contacts induces endocytosis of desmosomal plaque material. EMBO J 1982; 1:725 - 32; PMID: 6821357
- Kartenbeck J, Schmelz M, Franke WW, Geiger B. Endocytosis of junctional cadherins in bovine kidney epithelial (MDBK) cells cultured in low Ca2+ ion medium. J Cell Biol 1991; 113:881 - 92; http://dx.doi.org/10.1083/jcb.113.4.881; PMID: 2026652
- Fesenko I, Kurth T, Sheth B, Fleming TP, Citi S, Hausen P. Tight junction biogenesis in the early Xenopus embryo. Mech Dev 2000; 96:51 - 65; http://dx.doi.org/10.1016/S0925-4773(00)00368-3; PMID: 10940624
- Ligon LA, Holzbaur EL. Microtubules tethered at epithelial cell junctions by dynein facilitate efficient junction assembly. Traffic 2007; 8:808 - 19; http://dx.doi.org/10.1111/j.1600-0854.2007.00574.x; PMID: 17550375
- Bellett G, Carter JM, Keynton J, Goldspink D, James C, Moss DK, Mogensen MM. Microtubule plus-end and minus-end capture at adherens junctions is involved in the assembly of apico-basal arrays in polarised epithelial cells. Cell Motil Cytoskeleton 2009; 66:893 - 908; http://dx.doi.org/10.1002/cm.20393; PMID: 19479825
- Day AC, Luben R, Khawaja AP, Low S, Hayat S, Dalzell N, Wareham NJ, Khaw KT, Foster PJ. Genotype-phenotype analysis of SNPs associated with primary angle closure glaucoma (rs1015213, rs3753841 and rs11024102) and ocular biometry in the EPIC-Norfolk Eye Study. Br J Ophthalmol 2013; 97:704 - 7; http://dx.doi.org/10.1136/bjophthalmol-2012-302969; PMID: 23505305
- Levy D, Ehret GB, Rice K, Verwoert GC, Launer LJ, Dehghan A, Glazer NL, Morrison AC, Johnson AD, Aspelund T, et al. Genome-wide association study of blood pressure and hypertension. Nat Genet 2009; 41:677 - 87; http://dx.doi.org/10.1038/ng.384; PMID: 19430479
- Hong KW, Jin HS, Lim JE, Kim S, Go MJ, Oh B. Recapitulation of two genomewide association studies on blood pressure and essential hypertension in the Korean population. J Hum Genet 2010; 55:336 - 41; http://dx.doi.org/10.1038/jhg.2010.31; PMID: 20414254
- Lin Y, Lai X, Chen B, Xu Y, Huang B, Chen Z, Zhu S, Yao J, Jiang Q, Huang H, et al. Genetic variations in CYP17A1, CACNB2 and PLEKHA7 are associated with blood pressure and/or hypertension in She ethnic minority of China. Atherosclerosis 2011; 219:709 - 14; http://dx.doi.org/10.1016/j.atherosclerosis.2011.09.006; PMID: 21963141
- Cardellini P, Davanzo G, Citi S. Tight junctions in early amphibian development: detection of junctional cingulin from the 2-cell stage and its localization at the boundary of distinct membrane domains in dividing blastomeres in low calcium. Dev Dyn 1996; 207:104 - 13; http://dx.doi.org/10.1002/(SICI)1097-0177(199609)207:1<104::AID-AJA10>3.0.CO;2-0; PMID: 8875080
- Paschoud S, Citi S. Inducible overexpression of cingulin in stably transfected MDCK cells does not affect tight junction organization and gene expression. Mol Membr Biol 2008; 25:1 - 13; http://dx.doi.org/10.1080/09687680701474009; PMID: 18097951
- Paschoud S, Guillemot L, Citi S. Distinct domains of paracingulin are involved in its targeting to the actin cytoskeleton and regulation of apical junction assembly. J Biol Chem 2012; 287:13159 - 69; http://dx.doi.org/10.1074/jbc.M111.315622; PMID: 22315225