Abstract
The C-terminal Domain of RNA polymerase II undergoes a cycle of phosphorylation which allows it to temporally couple transcription and transcription-associated processes. The discovery of hitherto unrecognized metazoan elongation phase CTD kinase activities expands our understanding of transcription. We discuss the circumstances that delayed the recognition of these kinase activities.
Introduction
Transcription of a eukaryotic gene by RNA polymerase II (RNAPII) entails the precise coordination of numerous events involving hundreds of proteins; for different genes the kinds of events and their timing often vary. In view of this complexity, it is not surprising that the recruitment and synchronization of transcription-associated factors and processes are dependent on a polymerase-attached multi-state signaling platform: the hyperphosphorylatable C-terminal repeat domain (CTD) of RNAPII. Our recent characterization of unidentified major elongation-phase CTD kinase activities in higher eukaryotes fills an important gap in the current understanding of CTD phosphorylation and function.Citation1 It also raises an interesting question: Why were these CTD kinase activities unrecognized until now?
The CTD of RNAPII and the CTD Kinases
The CTD of RNAPII is an intrinsically unstructured extension of the enzyme's largest subunit, Rpb1, and is predominantly composed of consecutive repeats of a seven amino acid consensus sequence Y1S2P3T4S5P6S7. One of the most striking characteristics of the CTD is the large number of heptad repeats, which varies from organism to organism and tends to correlate with genomic complexity: Saccharomyces cerevisiae has 26–27 repeats, Drosophila has 45 repeats and the RNAPIIs of humans and mice contain 52 repeats. Intriguingly, the CTD is dispensable for the catalytic function of RNAPII, but a certain fraction of its length is essential for viability in each organism.Citation2
The finding that newly synthesized RNA crosslinks exclusively to the hyperphosphorylated form of Rpb1, together with the discovery of mRNA processing-involved CTD binding proteins, supported the proposal that the CTD linked RNA processing to transcription. This proposal has been experimentally verified over the last two decades. We now know that the CTD, acting as a selective binding scaffold, coordinates transcription with a variety of nuclear processes via the recruitment of specific factors during appropriate stages of transcription.Citation2
The binding specificity of the CTD for particular factors is determined by a series of site-specific phosphorylation/dephosphorylation events. The best-studied of these events occur at Serines 2 and 5 of the heptad repeats, generating the canonical “phospho-CTD cycle.” Ser5P predominates at initiation, and then Ser2P increases during elongation, yielding a CTD that contains doubly-phosphorylated repeats (Ser2P + Ser5P) in the center of the gene. Toward the 3′ end of the gene Ser5P levels appear to decrease, leaving polymerases phosphorylated at Ser2 to terminate transcription. It should be noted that, even though it is a useful model, the “phosopho-CTD cycle” is oversimplified. There are exceptions to the usual patterns of phosphorylation, and other post translational modifications have also been described.Citation3
The phosphorylation states of the CTD, and therefore its binding properties, are modulated by the activity of CTD kinases and phosphatases () (reviewed in refs. Citation3 and Citation4). The phosphorylation of Ser5 residues during initiation and promoter clearance is executed by the TFIIH CTD kinase subunit (Kin28 in yeast and CDK7 in metazoa). RNAPII committed to productive elongation is further phosphorylated at Ser2 positions in the CTD, primarily by CTDK-I (consisting of Ctk1, a CDK homologue; Ctk2, a cyclin homologue and Ctk3, whose function is unknown), the principal elongation-phase CTD kinase in S. cerevisiae.Citation3 Although responsible for the bulk of Ser2 phosphorylation in vivo, Ctk1 is nonessential and coexists with the essential kinase Bur1 (consisting of the CDK homologue Bur1 and the cyclin Bur2), that also contributes to Ser2 phosphorylation during (early) elongation (more on this later).Citation5,Citation6 A similar pair of Ser2 elongation kinases is also present in the fission yeast Schizosaccharomyces pombe (Sp) where Lsk1, the S. pombe ortholog of Ctk1 coexists with SpCDK9, the S. pombe ortholog of Bur1.Citation7,Citation8 However, the paradigm of Ser2 CTD kinase pairs appeared not to hold in higher eukaryotes.
Until recently it was believed that there is only one CTD Ser2 kinase in higher eukaryotes, P-TEFb (consisting of CDK9 and cyclinT).Citation3 P-TEFb plays key roles in various aspects of transcription, most notably in the release of polymerase from promoter-proximal pausing, and had been suggested to reconstitute the activity of both S. cerevisiae Ser2 CTD kinases, Bur1 and Ctk1.Citation9 This has been the overall view of the field for some time; however, there was extant evidence that this was not actually the case. Primarily stimulated by two evolutionary studies, work in our lab has now shown that the previously-unstudied Drosophila CDK12 (dCDK12) and little-studied human CDK12 and CDK13 (hCDK12/13) proteins are CTD elongation-phase kinases, the metazoan orthologs of yeast Ctk1.Citation1
A Short History of CTD Kinases
The first bona fide CTD kinase to be discovered was Ctk1, purified from yeast in 1989 and characterized in 1991 by Lee and Greenleaf.Citation10,Citation11 The next yeast CTD kinase was found in 1991 as a component of the RNAPII initiation machinery and was later identified as the Kin28 subunit of yeast TFIIH, providing an intuitive link between initiation and CTD phosphorylation.Citation12 In 1995, a genetic screen for suppressors of a CTD truncation further expanded the set of yeast CTD kinases with the discovery of Srb10 and Srb11, whose metazoan homologues are CDK8 and cyclin C.Citation13 These proteins were found to be part of the mediator complex, and appeared to play an inhibitory role in transcriptional initiation (reviewed in ref. Citation4). The discovery of Bur1 in 1993 by Prelich and Winston, and its subsequent identification as a CTD kinase in 2001 (more on this below) rounded out the collection of CTD kinases in S. cerevisiae.Citation14,Citation15
Most of the metazoan homologues of the yeast CTD kinases were discovered around the same time as their yeast counterparts. In 1994, CDK7 (then known as MO15) was identified as the mammalian counterpart (in terms of CTD kinase activity) to Kin28.Citation16 This was followed by the discovery of what was to become the principal Ser2 CTD kinase of higher eukaryotes with the 1995/1996 characterizations of P-TEFb by Marshall, Peng, Xie and Price.Citation17,Citation18 Using a Drosophila transcription system, Marshall and Price formulated an extremely successful model for RNAPII transcription in higher eukaryotes which stated that after initiation, RNAPII was “paused” near the promoter by the activity of negative transcription elongation factors (later identified as DSIF and NELF), which trapped the elongation complex in an arrested state.Citation19 P-TEFb (CDK9 + cyclin T) is required to overcome the influence of these negative elongation factors and allows the polymerase to enter productive elongation (reviewed in ref. Citation20). This crucial role for P-TEFb in metazoan transcription, coupled with the discovery, in 1997, that it is required for Tat-dependent elongation across the HIV genome, shifted the spotlight onto P-TEFb as the key player in Ser2 CTD phosphorylation and transcription elongation in higher eukaryotes.Citation21,Citation22 This burst of discoveries in the 1990s was followed by almost a decade of silence before the relationships between Bur1, Ctk1 and P-TEFb would be reexamined.
An Established Narrative
Perhaps one of the main reasons no one actively looked for Ctk1 homologues in higher eukaryotes is that there seemed to be no real necessity for them, as explained in the following two-part argument.
Part one depicts the relegation of Bur1 to the sidelines in terms of CTD phosphorylation activities. Shortly after the discovery of P-TEFb, homology searches revealed two candidates for the yeast counterpart of CDK9, Bur1 and Ctk1 (both with 43% sequence identity to CDK9).Citation22 Bur1 had been identified in a genetic screen for mutations that increase transcription from an UAS-less promoter and was shown to be an essential gene.Citation14 Despite the importance of Bur1 kinase, its substrates remained unidentified until 2001, when Murray et al. showed that the CTD (of Rpb1) co-immunoprecipitated with Bur1 and that the CTD of both the co-precipitated RNAPII and exogenously-added CTD fusion proteins could be phosphorylated by Bur1.Citation15 However, they identified the in vitro target site of Bur1 as Ser5 of the CTD repeats, whereas it was already known that the target site of P-TEFb was Ser2. The Bur1 result may be explained by what appears to be an artifact of CTD kinase assays: when assayed in vitro with an unphosphorylated substrate, CTD kinases often appear to change their target site specificity. For example, given an unphosphorylated substrate, Ctk1 kinase will begin by phosphorylating Ser5, before moving on to Ser2, its actual substrate site in vivo (making in vivo data a must in the characterization of CTD kinases).Citation23 Despite the apparent difference in substrate targets, the discovery of Bur1 CTD kinase activity provided functional evidence for the proposed evolutionary relationships between Bur1 and P-TEFb. However, these results were quickly followed by an in vivo study that used temperature-sensitive strains of Bur1 to dispute the claim that Bur1 is a CTD kinase.Citation24 Chromatin immunoprecipitation (ChIP) data showed that although Bur1 did associate with transcription elongation complexes and appeared to act as a transcriptional elongation factor, it had no effect on either Ser5 or Ser2 phosphorylation levels (after normalization to total polymerase). Relevant to the above commentary on in vitro assays, the same study found that Bur1 was able to phosphorylate about equally well both Ser5 and Ser2 positions in CTD fusion proteins.Citation24 Due to the ChIP data it was concluded that Bur1 is not a significant source of co-transcriptional RNAPII phosphorylation, and this view prevailed well into 2009. Shortly after the publication of reference Citation24, Pei and Shuman discovered that the S. pombe Bur1 ortholog (SpCDK9) phosphorylates the transcriptional elongation factor Spt5, a subunit of DSIF that had been previously shown to have genetic interactions with Bur1 in S. cerevisiae and to be phosphorylated by P-TEFb in higher eukaryotes.Citation25 The sequence similarities between metazoan P-TEFb and both Bur1 & Ctk1, together with the fact that Bur1 and Ctk1 share many genetic interactions (e.g., deletion of CTK1 is lethal in a bur1 mutant background), led to the proposal that P-TEFb may reconstitute the activities of both Bur1 and Ctk1 in higher eukaryotes.Citation9,Citation15 This “reconstitution hypothesis” became the predominant narrative of the transcription field and drew attention away from the existence of a separate metazoan Ctk1 homologue.
Part two of the argument for the superfluity of a metazoan ortholog of Ctk1 derives from the observation that inhibition or depletion of P-TEFb activity halts nearly all Ser2 CTD phosphorylation in several in vivo systems (e.g., Drosophila larval polytene chromosomes and mouse embryonic stem cells).Citation26,Citation27 Thus, models of CTD phosphorylation did not necessitate the existence of any major CTD kinases besides P-TEFb and CDK7. Because it seemed possible that other unknown kinases might also be affected by the P-TEFb inhibitor, Flavopiridol (FVP), we tested dCDK12 and hCDK13 for FVP sensitivity; however, both are much less sensitive than P-TEFb (data not shown), arguing against this interpretation. A more probable explanation for the loss of global Ser2 CTD phosphorylation upon P-TEFb inactivation relates to the previously-mentioned phenomenon of promoter-proximal pausing. Initially regarded as a rarity, promoter-proximal pausing has been shown to be a prevalent form of transcriptional control in metazoa (there is no evidence for promoter proximal pausing in yeast).Citation20 Thus, the inhibition of P-TEFb traps polymerases in promoter proximal positions within 100 bp downstream of the transcription start site and leads to a near global shutdown of pre-mRNA synthesis.Citation27,Citation28 A shutdown of global transcription would mask the activity of any downstream elongation phase CTD kinases, giving the illusion that all Ser2 CTD phosphorylation is mediated by P-TEFb. This phenomenon lacks a parallel in terms of the yeast kinases, as a strong reduction in Bur1 kinase activity does not necessarily reduce levels of elongating RNAPII, which continues to be phosphorylated on Ser2 by Ctk1.Citation5,Citation6
One additional possible reason for the lack of an earlier search for Ctk1 orthologs in higher eukaryotes is that P-TEFb became a factor of intense interest once its participation in HIV transcription was uncovered, overshadowing the idea that another metazoan CTD kinase might exist. As an illustration, a Pubmed search for “P-TEFb or Cdk9” currently yields over 650 results compared to about 30 for “Bur1” and 50 for “Ctk1.”
The Ctk1/CDK12/CDK13 Undertow and the Characterization of CDK12 as a CTD Kinase
A cursory search through the literature reveals that post early 2000, most publications list P-TEFb as the homologue of both Bur1 and Ctk1; however, there was strong evidence that the CTD kinase story was incomplete. A comparative genomics paper published in 2000 by Liu and Kipreos examined the evolutionary relationships of CDKs in budding yeast, fission yeast, humans, Drosophila and C. elegans.Citation29 As expected, Bur1 was found to be most closely related to human P-TEFb (CDK9); however, Ctk1 was actually found to be most closely related to two little-studied human proteins, known then as CrkRS and CHED (subsequently renamed CDK12 and CDK13, respectively). Drosophila contained only one Ctk1 counterpart, an uncharacterized protein (CG7597), which we have now renamed Drosophila CDK12 (dCDK12). The evolutionary analysis did not go completely unnoticed: the 2001 paper which first characterized Bur1 as a CTD kinase cited Liu and Kipreos in its discussion and made the point that multiple P-TEFb like CTD kinases might be present not only in yeast, but in other organisms.Citation15 In the same year, CrkRS (hCDK12) was even shown to have CTD kinase activity in vitro.Citation30 Another comparative genomics paper published by Guo and Stiller in 2004 further strengthened the evolutionary evidence, independently coming to the same conclusions regarding the relationships between P-TEFb, Bur1, CDK12/13 and Ctk1.Citation31 Based on these evolutionary relationships, our lab began characterizing CDK12 and CDK13.
The final pieces of the puzzle fell together in 2009 when, as was implied by the evolutionary analyses, two independent groups showed that Bur1 has an in vivo Ser2 CTD kinase activity.Citation5,Citation6 These findings also correlated well with the paradigms discovered in the fission yeast Sp. During 2007–2009 Lsk1, the Sp ortholog of Ctk1, was shown to be responsible for the bulk of Ser2 CTD phosphorylation while SpCDK9, the Bur1 ortholog in Sp, was found to phosphorylate both the CTD and the Spt5 subunit of DSIF.Citation7,Citation8 It is interesting to note that the contribution of Bur1 to CTD Ser2 phosphorylation appears to be greatest near the promoter (due to the recruitment of Bur1 to the Ser5 phosphorylated CTD via a phospho-CTD-interacting domain in the C-terminus of Bur1); this may serve to prime the CTD for further phosphorylation by Ctk1 and is in accordance with the proposed homologies (Bur1↔CDK9 and Ctk1↔CDK12/13).Citation1,Citation5,Citation8,Citation23
Combining the evolutionary and functional evidence from previous studies with our recent results led us to conclude that CDK12 is indeed a bona fide CTD kinase, the metazoan ortholog of yeast Ctk1. (The in vivo function of CDK13 remains somewhat elusive).Citation1
What's Next for the Field?
Besides our initial characterizations, there have been no other studies of CDK12 and CDK13 in the context of transcription elongation and CTD phosphorylation. This leaves many important questions unanswered including, but surely not limited to, the following: Where exactly, and when, do these kinases add phosphates along the CTD? Why are there two Ctk1 homologues in humans? What is the actual function of CDK13? Do these kinases act at specific subsets of genes? What are the genome-wide relationships between P-TEFb and CDK12/13?
Further studies of CDK12 and CDK13 in the next few years should answer these and other questions and should greatly expand our knowledge of both CTD phosphorylation and RNAPII transcription. Importantly, the identification of these elongation-phase Ser2 CTD kinases in metazoa enables studies of the link between CTD phosphorylation patterns and transcription elongation-associated processes, such as splicing, in higher eukaryotes. These studies should lead to many fascinating discoveries in the coming years.
Abbreviations
CTD | = | C-terminal domain |
Ser5P | = | phosphorylated Ser5 (within the CTD heptad repeat) |
Ser2P | = | phosphorylated Ser2 (within the CTD heptad repeat) |
RNAPII | = | RNA polymerase II |
TFIIH | = | transcription factor IIH |
P-TEFb | = | positive transcription elongation factor b (consisting of CDK9 + cyclin T) |
CDK | = | cyclin dependent kinase |
DSIF | = | DRB sensitivity inducing factor (Spt4 + Spt5) |
NELF | = | negative elongation factor |
UAS | = | upstream activating sequence |
S. cerevisiae | = | Saccharomyces cerevisiae |
S. pombe or Sp | = | Schizosaccharomyces pombe |
Figures and Tables
Figure 1 An updated model of the “Phoshpo-CTD cycle” featuring major kinase activities and CTD-associated proteins in both yeast (y) and metazoa (m). RNA Polymerase II is illustrated at four positions within a gene, with the phosphorylation state of the CTD indicated by color. The phosphorylation status of the CTD dictates which sets of factors bind to the CTD, and proteins bound by a specific CTD phosphorylation state are shown in the appropriate color (examples of associated factors at each stage of transcription are given below the gene). Major CTD kinase activities are indicated for both yeast and metazoa and include the newly characterized metazoan ortholog of yeast Ctk1, mCDK12. Although mCDK9 and mCDK12 kinase activities are illustrated as having distinct boundaries, it seems likely that the activities overlap during early elongation (whether this is really the case remains to be determined). CTD phosphatases work in coordination with the CTD kinases throughout the “Phospho-CTD cycle” in order to shape the phosphorylation status of the CTD (reviewed in references Citation2 and Citation3).
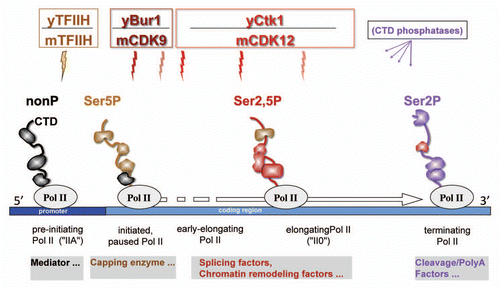
Acknowledgements
We thank D. Price, H. Phatnani and T. Winsor for critical reading of the manuscript. We also apologize to the researchers whose work could not be referenced due to space limitations. This work was supported by NIH grant GM040505 and Duke School of Medicine Bridging Funds to A.L.G.
References
- Bartkowiak B, Liu P, Phatnani HP, Fuda NJ, Cooper JJ, Price DH, et al. CDK12 is a transcription elongation-associated CTD kinase, the metazoan ortholog of yeast Ctk1. Genes Dev 2010; 24:2303 - 2316
- Phatnani HP, Greenleaf AL. Phosphorylation and functions of the RNA polymerase II CTD. Genes Dev 2006; 20:2922 - 2936
- Buratowski S. Progression through the RNA polymerase II CTD cycle. Mol Cell 2009; 36:541 - 546
- Meinhart A, Kamenski T, Hoeppner S, Baumli S, Cramer P. A structural perspective of CTD function. Genes Dev 2005; 19:1401 - 1415
- Qiu H, Hu C, Hinnebusch AG. Phosphorylation of the Pol II CTD by KIN28 enhances BUR1/BUR2 recruitment and Ser2 CTD phosphorylation near promoters. Mol Cell 2009; 33:752 - 762
- Liu Y, Warfield L, Zhang C, Luo J, Allen J, Lang WH, et al. Phosphorylation of the transcription elongation factor Spt5 by yeast Bur1 kinase stimulates recruitment of the PAF complex. Mol Cell Biol 2009; 29:4852 - 4863
- Karagiannis J, Balasubramanian MK. A cyclin-dependent kinase that promotes cytokinesis through modulating phosphorylation of the carboxy terminal domain of the RNA Pol II Rpb1p sub-unit. PLoS One 2007; 2:433
- Viladevall L, St. Amour CV, Rosebrock A, Schneider S, Zhang C, Allen JJ, et al. TFIIH and P-TEFb coordinate transcription with capping enzyme recruitment at specific genes in fission yeast. Mol Cell 2009; 33:738 - 751
- Wood A, Shilatifard A. Bur1/Bur2 and the Ctk complex in yeast: the split personality of mammalian P-TEFb. Cell Cycle 2006; 5:1066 - 1068
- Lee JM, Greenleaf AL. A protein kinase that phosphorylates the C-terminal repeat domain of the largest subunit of RNA polymerase II. Proc Natl Acad Sci USA 1989; 86:3624 - 3628
- Lee JM, Greenleaf AL. CTD kinase large subunit is encoded by CTK1, a gene required for normal growth of Saccharomyces cerevisiae. Gene Expr 1991; 1:149 - 167
- Feaver WJ, Gileadi O, Li Y, Kornberg RD. CTD kinase associated with yeast RNA polymerase II initiation factor b. Cell 1991; 67:1223 - 1230
- Liao SM, Zhang J, Jeffery DA, Koleske AJ, Thompson CM, Chao DM, et al. A kinase-cyclin pair in the RNA polymerase II holoenzyme. Nature 1995; 374:193 - 196
- Prelich G, Winston F. Mutations that suppress the deletion of an upstream activating sequence in yeast: involvement of a protein kinase and histone H3 in repressing transcription in vivo. Genetics 1993; 135:665 - 676
- Murray S, Udupa R, Yao S, Hartzog G, Prelich G. Phosphorylation of the RNA polymerase II carboxy-terminal domain by the Bur1 cyclin-dependent kinase. Mol Cell Biol 2001; 21:4089 - 4096
- Feaver WJ, Svejstrup JQ, Henry NL, Kornberg RD. Relationship of CDK-activating kinase and RNA polymerase II CTD kinase TFIIH/TFIIK. Cell 1994; 79:1103 - 1109
- Marshall NF, Peng J, Xie Z, Price DH. Control of RNA polymerase II elongation potential by a novel carboxyl-terminal domain kinase. J Biol Chem 1996; 271:27176 - 27183
- Marshall NF, Price DH. Purification of P-TEFb, a transcription factor required for the transition into productive elongation. J Biol Chem 1995; 270:12335 - 12338
- Marshall NF, Price DH. Control of formation of two distinct classes of RNA polymerase II elongation complexes. Mol Cell Biol 1992; 12:2078 - 2090
- Nechaev S, Adelman K. Pol II waiting in the starting gates: Regulating the transition from transcription initiation into productive elongation. Biochim Biophys Acta 2011; 1809:34 - 45
- Mancebo HS, Lee G, Flygare J, Tomassini J, Luu P, Zhu Y, et al. P-TEFb kinase is required for HIV Tat transcriptional activation in vivo and in vitro. Genes Dev 1997; 11:2633 - 2644
- Zhu Y, Pe'ery T, Peng J, Ramanathan Y, Marshall N, Marshall T, et al. Transcription elongation factor P-TEFb is required for HIV-1 tat transactivation in vitro. Genes Dev 1997; 11:2622 - 2632
- Jones JC, Phatnani HP, Haystead TA, MacDonald JA, Alam SM, Greenleaf AL. C-terminal repeat domain kinase I phosphorylates Ser2 and Ser5 of RNA polymerase II C-terminal domain repeats. J Biol Chem 2004; 279:24957 - 24964
- Keogh MC, Podolny V, Buratowski S. Bur1 kinase is required for efficient transcription elongation by RNA polymerase II. Mol Cell Biol 2003; 23:7005 - 7018
- Pei Y, Shuman S. Characterization of the Schizosaccharomyces pombe Cdk9/Pch1 protein kinase: Spt5 phosphorylation, autophosphorylation and mutational analysis. J Biol Chem 2003; 278:43346 - 43356
- Ni Z, Schwartz BE, Werner J, Suarez JR, Lis JT. Coordination of transcription, RNA processing and surveillance by P-TEFb kinase on heat shock genes. Mol Cell 2004; 13:55 - 65
- Rahl PB, Lin CY, Seila AC, Flynn RA, McCuine S, Burge CB, et al. c-Myc regulates transcriptional pause release. Cell 2010; 141:432 - 445
- Chao SH, Price DH. Flavopiridol inactivates P-TEFb and blocks most RNA polymerase II transcription in vivo. J Biol Chem 2001; 276:31793 - 31799
- Liu J, Kipreos ET. Evolution of cyclin-dependent kinases (CDKs) and CDK-activating kinases (CAKs): differential conservation of CAKs in yeast and metazoa. Mol Biol Evol 2000; 17:1061 - 1074
- Ko TK, Kelly E, Pines J. CrkRS: a novel conserved Cdc2-related protein kinase that colocalises with SC35 speckles. J Cell Sci 2001; 114:2591 - 2603
- Guo Z, Stiller JW. Comparative genomics of cyclin-dependent kinases suggest co-evolution of the RNAP II C-terminal domain and CTD-directed CDKs. BMC Genomics 2004; 5:69