Abstract
Ubiquitylation has emerged as an omnipresent factor at all levels of transcriptional regulation. A recent study that describes the yeast transcriptional activator Met4 as a functional component of the very same ubiquitin ligase that regulates its own activity highlights the close relation between transcription and the ubiquitin proteasome system.
Introduction
Ubiquitylation is a cascade of enzymatic reactions that lead to the covalent modification of proteins with the highly conserved protein ubiquitin. Once one ubiquitin is attached to substrate proteins (monoubiquitylation) additional ubiquitin moieties can be attached to other lysine residues in the substrate (multiubiquitylation), or to substrate-linked ubiquitin to form a polyubiquitin chain (polyubiquitylation).Citation1 Ubiquitylation serves as a protein mark with distinct signaling functions. Best known for signaling of degradation by the proteasome, many other functions for protein ubiquitylation have also been described including intracellular transport, protein interactions and protein activity.Citation2 Given the host of cellular processes that are regulated by ubiquitin it is hardly surprising that many levels of transcriptional control are governed by ubiquitylation in a proteolytic and proteolysis-independent fashion.Citation3 Here we will discuss the role of ubiquitylation in transcription in general and take a closer look at recent findings that transcription factors themselves can be active components of the ubiquitylation machinery to control protein complex dynamics.Citation4
Ubiquitylation and Chromatin Reorganization
In eukaryotic cells, DNA is packaged in compact chromatin and this organization appears to hinder RNA polymerase II (RNAPII) mediated transcription. To overcome this obstacle, chromatin needs to be reorganized to allow the transcription machinery access to target DNA sequences. The precise mechanism by which this reorganization is achieved is not known but emerging evidence indicates that it is accomplished by several posttranslational modifications on histones, leading to the recruitment of chromatin-remodeling factors.Citation5,Citation6 Both the histone cores and the histone tails are subject to a great diversity of posttranslational modifications including but not limited to acetylation, methylation, phosphorylation and ubiquitylation. It was known for more than three decades that histone H2A is monoubiquitylated,Citation7 but only recently strong evidence has been reported that monoubiquitylation of H2A has a repressive role.Citation8,Citation9 In contrast, monoubiquitylation of the core histone H2B stimulates activating methylations of histone H3 (H3K4 and H3K79) and might play a direct role in stimulating RNA polymerase II. H2B monoubiquitylation has also been associated with silencing, underscoring the diverse roles for histone ubiquitylation in transcription.Citation10,Citation11
Ubiquitylation and RNAPII
Ubiquitylation and degradation of Rbp1, the largest subunit of RNAPII, was first observed as part of the transcription-coupled DNA repair pathway.Citation12 More recent work extended the role for Rbp1 ubiquitylation to resolve stalled RNAPII during general transcription pausing independent of DNA damage.Citation13 For example, RNAPII inhibition or a block of transcriptional elongation will induce Rbp1 ubiquitylation and subsequent proteasomal degradation.Citation14 The mechanism appears similar to DNA damage induced RNAPII clearance, and is a last resort to resolve stalled RNAPII.
Ubiquitylation and mRNA Metabolism
Maturation, nuclear export and stability of mRNA transcripts are tightly controlled and critical roles for ubiquitylation in these processes are emerging. Ubiquitylation has been known for some time to be important for splicing in vitroCitation15 and recently, an ubiquitylation/deubiquitylation cycle has been described to control the dynamics of the spliceosome complex.Citation16 Central to this regulation are the spliceosomal Prp19 complex (NTC), which functions as an E3 ubiquitin ligase, and the deubiquitylating enzyme Usp4Sart3. Together they regulate reversible ubiquitylation of the U4 snRNP component Prp3, which orchestrates rearrangement of both snRNA and protein components within the spliceosome during progression through the splicing cycle.Citation16 Several studies, mainly in the yeast model, have linked the ubiquitin-proteasome system to the nuclear export of mRNAs.Citation17 Particularly, the two HECT ubiquitin ligases, Rsp5 and Tom1, as well as the cullinRING ligase SCFMdm30 are connected with the transport of mRNAs from nucleus to cytoplasm. In most cases detailed mechanisms and critical substrates remain to be elucidated, but the crucial role for the E3 ligase Rsp5 seems to be ubiquitylation and subsequent degradation of the nuclear export factor Hpr1.Citation18 Less is known about the relationship between mRNA degradation and the ubiquitin proteasome system. However, already over a decade ago, it was shown that the rapid decay of AU-rich cytokine and proto-oncogene mRNAs requires both ubiquitin conjugation and proteasome activity.Citation19
Ubiquitylation and Transcription Factors
Many transcription factors, including yeast Gcn4 and Gal4 as well as mammalian Myc, Jun, E2-F and p53, are unstable proteins. Their protein levels are usually maintained low through targeted degradation by the ubiquitin-proteasome system (UPS) to allow rapid response to environmental stimuli. A surprising and seemingly paradoxical concept has emerged in which ubiquitylation and degradation of many transcriptional activators at promoter sites is necessary for efficient transcription.Citation3,Citation20,Citation21 This tight spatial and temporal connection between transcription factor activation and degradation could be crucial for sensitivity to regulatory signals and prevents hyperactivation.
Aside from the classic paradigm of ubiquitin-dependent degradation of transcription factors, the ubiquitin system is also involved in a limited processing rather than in complete destruction of the target transcription factor. For example, the yeast transcription factor Spt23 is anchored to the outer membrane of the endoplasmic reticulum and activation in response to fatty acid limitation is initiated by Spt23 ubiquitylation followed by limited proteolysis to clip off the membrane anchor.Citation22 The mature Spt23 is released from the membrane and can translocate to the nucleus to activate expression of genes involved in fatty acid biosynthesis. Regulation of transcription factor localization by ubiquitylation is a common concept. A classical example is NFκB signaling. NFκB is restrained to the cytosol by binding to its inhibitor IκB. Inflammation induces ubiquitylation and degradation of IκB allowing translocation of NFκB into the nucleus, where it activates gene transcription.Citation23 The tumor suppressor protein and transcriptional activator p53 demonstrates a more complex regulation by ubiquitylation. The p53 ubiquitin ligase Mdm2 can either mono or polyubiquitylate p53. Polyubiquitylation induces p53 degradation by the proteasome and monoubiquitylation signals for its nuclear export.Citation24 Both ubiquitylation events thus inactivate the nuclear function of p53, but monoubiquitylation protects cytosolic roles of p53.
The many aspects of transcription factor regulation by ubiquitin have been extensively studied, but research has often been focused on individual proteins. Yet, gene expression is generally controlled by multi-ubunit protein complexes consisting of activators, DNA-binding components and other co-factors. Recent work on regulation of sulfur metabolism in yeast has highlighted the importance of ubiquitylation in regulation of the dynamics and composition of transcription complexes. Below we will introduce this system and discuss how the interplay between proteolysis-dependent and independent ubiquitylation controls this transcription network.
Regulation of Biosynthesis of Sulfur Containing Metabolites by the Met4 Transcription Complex
In Saccharomyces cerevisiae, the transcription factor Met4 regulates a subset of genes referred to as MET genes.Citation25 MET genes encode proteins required for the biosynthesis of sulfur containing metabolites such as methionine, cysteine, glutathione and S-adenosylmethionine (SAM).Citation26 Limiting intracellular methionine levels as well as heavy metal stress (cadmium or arsenic), trigger Met4 activation and subsequent MET gene expression.Citation27 This pathway connects sulfur-containing metabolite balance to cell cycle regulation, because full activation of Met4 induces a cell cycle arrest to maintain cellular and genetic integrity during nutrient limitation or heavy metal stress. The products of MET genes rewire the metabolic pathways to restore intracellular levels of sulfur metabolites and once achieved, Met4 gets inactivated through ubiquitylation by the E3 ubiquitin ligase, SCFMet30.Citation28,Citation29 Regulation of the transcriptional activator Met4 by ubiquitylation represents an interesting example for the diversity of ubiquitin signaling. Inactivation of Met4 is achieved through attachment of a single K-48 linked ubiquitin chain to an invariant lysine residue at position 163 in Met4.Citation30 Despite carrying this canonical degradation signal ubiquitylated Met4 is generally not targeted for degradation by the proteasome.Citation30–Citation32 The K48-ubiquitin chain serves as a direct modulator of Met4 activity in a proteolysis-independent manner and demonstrates the versatility of the ubiquitin signal (). The interesting mode of Met4 regulation raises several questions. How does ubiquitylated Met4 escape degradation by the proteasome? How does Met4 ubiquitylation repress Met4? And, why inactivate by proteolysis-independent ubiquitylation rather than degradation? A series of experiments identified two internal ubiquitin binding domains (UBD) within the N-terminal region of Met4. These tandem-UBDs specifically bind to the polyubiquitin chain attached to Met4 and shield it from recognition by the 26S proteasome.Citation33,Citation34 Mutations in these domains convert Met4 in a proteasome substrate. Interestingly, the tandem-UBD domain of Met4 is transferable and can shield proteasome substrates to convert them into stable ubiquitylated proteins.Citation34 The identification of the N-terminal UBDs also suggested an attractive model for Met4 repression by ubiquitylation through induction of a conformational switch by the interaction between tandem-UBD and the ubiquitin chain. Surprisingly, this model was disproven and the detailed mechanism for Met4 inactivation by ubiquitylation remains to be discovered. Lastly, the rationale for Met4 inactivation by non-proteolytic ubiquitylation versus simple degradation by the proteasome led to two important conceptual insights. First, proteolysis-independent inactivation maintains a pool of dormant Met4 that can be rapidly activated by deubiquitylation. Such a kinetic advantage in activation of MET genes was obvious by comparing nutrient or heavy metal stress response of cells expressing either wild-type Met4 (non-proteolytic regulation) or Met4 lacking the UBDs (regulated by proteolysis).Citation33,Citation34 Second, more recently we discovered an additional and perhaps more significant reason for non-proteolytic regulation of Met4. In addition to its function as a transactivator, Met4 is a substrate recruitment factor in the SCFMet30-Met4 ubiquitin ligase, which requires Met4 to be available even when it is inactive as a transcription factor.Citation4,Citation35 To appreciate the significance of this dual function of Met4 it is important to introduce Met4 co-factors. Due to the lack of an intrinsic DNA binding activity Met4 requires auxiliary factors with DNA-binding capacity. Met31, Met32, Met28 and Cbf1 bind to Met4 and form a functional transcription complex. Among these cofactors, Met31, Met32 and Cbf1 are the DNA binding cofactors, whereby Met31 and Met32 are redundant for MET gene expression.Citation36 All auxiliary factors are required for the biosynthesis of sulfur-containing metabolites, but only Met32 is involved in induction of cell cycle arrest during nutrient and heavy metal stress ().
A Transcriptional Activator as a Component of the SCFMet30-Met4 Ubiquitin Ligase
All Met4 co-factors are relatively unstable proteins, but particularly Met32 showed very rapid degradation and was almost completely absent from cells unless they experienced nutrient or heavy metal stress.Citation4 These observations prompted further investigation of the Met32 degradation pathway because of its role in connecting cell cycle arrest with nutrient availability. Indeed, stabilization of Met32 induced G1-phase cell cycle arrest even in the absence of nutrient stress. Ubiquitylation and degradation of Met32 depended on the SCFMet30 ubiquitin ligase, the same E3 ligase complex that ubiquitylates Met4. As an interesting note, these results highlight the dependence of ubiquitin signaling on down-stream factors for signal interpretation because here the same ligase ubiquitylates two proteins whereby one of them (Met32) is degraded by the proteasome and the other (Met4) is directly regulated in a proteolysis-independent fashion. E3 ubiquitin ligases mediate substrate specificity by directly binding to their substrates. Not surprisingly, SCFMet30 binding to its substrate Met4 has been extensively documented both in vivo and in vitro.Citation4,Citation28,Citation29,Citation32 Unexpectedly, despite strong genetic evidence for SCFMet30-dependent ubiquitylation and degradation of Met32, a physical interaction between the ubiquitin ligase and its substrate Met32 could not be detected. This conundrum was solved when the transcriptional activator Met4 was added to the system, because it mediated recruitment of Met32 to the E3 ligase.Citation4 The transactivator Met4 is thus a bona fide component of the SCFMet30-Met4 ubiquitin ligase that mediates substrate selection and stimulates ubiquitylation of Met32. Although similar biochemical evidence is lacking for other Met4 co-factors, genetic analyses suggest that SCFMet30-Met4 is the ligase responsible for ubiquitylation and degradation of other Met4 co-factors, namely Cbf1 and Met31. Degradation of the remaining known co-factor Met28 is independent of SCFMet30, and its ubiquitin ligase remains to be identified. Collectively, these findings expose the E3 ligase SCFMet30 as the master regulator of the Met4 transcription complex. SCFMet30 controls transactivator function of Met4 by proteolysis-independent ubiquitylation and regulates stability of the DNA-binding co-factors in the complex (). What is more, by employing its substrate Met4 as substrate adaptor, SCFMet30 coordinates Met4 inactivation with degradation of its co-factors. The significance of this dual function of Met4 as an E3 ligase component and a transcriptional activator is particularly evident considering that Met32 is the mediator of cell cycle arrest in response to nutrient or heavy metal stress. Both stress conditions block SCFMet30, which leads simultaneously to active deubiquitylated Met4 and stabilized Met32 and thus synchronizes the transcriptional response with cell cycle progression. An additional layer of ubiquitin-based control adds further to the complexity of this system. A so far unidentified ubiquitin ligase selectively targets excess co-factors for degradation by the proteasome.Citation4 This pathway is constitutive and unresponsive to stress conditions. Interestingly, co-factors bound to Met4 are protected from this degradation pathway. Consequently, nutrient and heavy metal stress, which both block SCFMet30 result in selective stabilization of Met4-bound co-factors, while the unbound DNA-binding factors are removed by proteasomal degradation. Together these different ubiquitylation pathways ensure accumulation of the active and completely assembled Met4 transcription complex during stress conditions, whereas formation of partially assembled unproductive complexes is prevented (). Furthermore, a system as the one described here for the Met4 complex is exceptionally well suited for fine-tuning, as activation and stabilization of individual components in the complex is linked at the single-molecule level.
Concluding Remarks
Regulation of the yeast Met4 transcription complex reveals the complexity and flexibility of the ubiquitin-proteasome system as it evolved to coordinate regulation of components within the transcription complex. We have only started to appreciate the importance of ubiquitylation in modulation of transcription factor complexes. The yeast Met4 system taught us how ubiquitylation events synchronize individual components in the complex to the activation and repression cycles. Work on the vertebrate transcription complexes assembled by LIM homeodomain (LIM-HD) proteins demonstrated the importance of ubiquitylation in co-factor exchange while preserving promoter occupancy.Citation37 The same system was used to show how a series of co-factor interactions protects the complex from ubiquitylation and degradation and thus allows the selective assembly of stable transcription complexes in response to developmental cues.Citation38,Citation39 These studies on transcription factor complexes and their regulation by the ubiquitin proteasome system have impact beyond the field of transcription. Multisubunit protein complexes rather than individual proteins govern most cellular and developmental processes. The assembly of functional protein complexes and their dynamics in response to various signals is thus intrinsic to our understanding of living organisms. The ubiquitin proteasome system is likely a major contributor to these processes.
Figures and Tables
Figure 1 Regulation of sulfur metabolite homeostasis by ubiquitin-controlled transcription programs. (A) The ubiquitin ligase SCFMet30 links nutrient availability and heavy metal stress to cell cycle control and transcriptional response. The transcriptional activator Met4 is maintained in an inactive state by ubiquitylation. Ubiquitin-binding domains in the amino-terminal region of Met4 shield the ubiquitin chain from proteasome recognition and degradation. Nutrient or heavy metal stress block Met4 ubiquitylation and deubiquitylation converts the dormant pool of inactive Met4 into an active transcription factor. Met4 together with several co-factors induces a transcriptional program to restore sulfur metabolite homeostasis and to protect from heavy metal stress. A cell cycle arrest that specifically requires Met32 is also induced to protect cellular and genomic integrity during stress. (B) The transcriptional activator Met4 is substrate and substrate adapter of the ubiquitin ligase SCFMet30-Met4 and controls transcription complex dynamics. The transactivator function of Met4 is inhibited by SCFMet30-mediated ubiquitylation. Met4 ubiquitylation does not regulate its function as substrate adapter of the ubiquitin ligase SCFMet30-Met4, which leads to ubiquitylation and degradation of the DNA-binding co-factors Met31, Met32 and Cbf1. Stress signals block SCFMet30 resulting in simultaneous activation of Met4 and stabilization of the Met4 bound co-factors to assemble the active Met4 complex. A constitutive second degradation pathway independent of SCFMet30-Met4 selectively removes unbound co-factors. Repression after disappearance of the stress signal induces ubiquitin-dependent co-factor degradation and Met4 inactivation.
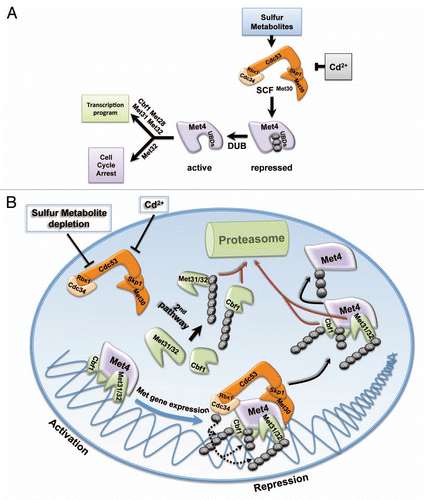
Acknowledgments
Work in the Kaiser laboratory is supported by National Institutes of Health Grants GM66164 and GM66164AS1 (to P.K.). I.O. is a recipient of a National Institutes of Health Ruth Kirschstein Postdoctoral Fellowship T32 (CA-113265).
References
- Hershko A, Ciechanover A. The ubiquitin system. Annu Rev Biochem 1998; 67:425 - 479
- Kerscher O, Felberbaum R, Hochstrasser M. Modification of proteins by ubiquitin and ubiquitin-like proteins. Annu Rev Cell Dev Biol 2006; 22:159 - 180
- Muratani M, Tansey WP. How the ubiquitin-proteasome system controls transcription. Nat Rev Mol Cell Biol 2003; 4:192 - 201
- Ouni I, Flick K, Kaiser P. A transcriptional activator is part of an SCF ubiquitin ligase to control degradation of its cofactors. Mol Cell 2010; 40:954 - 964
- Smith E, Shilatifard A. The chromatin signaling pathway: diverse mechanisms of recruitment of histone-modifying enzymes and varied biological outcomes. Mol Cell 2010; 40:689 - 701
- Li B, Carey M, Workman JL. The role of chromatin during transcription. Cell 2007; 128:707 - 719
- Goldknopf IL, Taylor CW, Baum RM, Yeoman LC, Olson MO, Prestayko AW, et al. Isolation and characterization of protein A24, a “histone-like” non-histone chromosomal protein. J Biol Chem 1975; 250:7182 - 7187
- Zhou W, Zhu P, Wang J, Pascual G, Ohgi KA, Lozach J, et al. Histone H2A monoubiquitination represses transcription by inhibiting RNA polymerase II transcriptional elongation. Mol Cell 2008; 29:69 - 80
- Wang H, Wang L, Erdjument-Bromage H, Vidal M, Tempst P, Jones RS, et al. Role of histone H2A ubiquitination in Polycomb silencing. Nature 2004; 431:873 - 878
- Osley MA, Fleming AB, Kao CF. Histone ubiquitylation and the regulation of transcription. Results Probl Cell Differ 2006; 41:47 - 75
- Weake VM, Workman JL. Histone ubiquitination: triggering gene activity. Mol Cell 2008; 29:653 - 663
- Beaudenon SL, Huacani MR, Wang G, McDonnell DP, Huibregtse JM. Rsp5 ubiquitin-protein ligase mediates DNA damage-induced degradation of the large subunit of RNA polymerase II in Saccharomyces cerevisiae. Mol Cell Biol 1999; 19:6972 - 6979
- Daulny A, Tansey WP. Damage control: DNA repair, transcription and the ubiquitin-proteasome system. DNA Repair 2009; 8:444 - 448
- Somesh BP, Reid J, Liu WF, Sogaard TM, Erdjument-Bromage H, Tempst P, et al. Multiple mechanisms confining RNA polymerase II ubiquitylation to polymerases undergoing transcriptional arrest. Cell 2005; 121:913 - 923
- Bellare P, Small EC, Huang X, Wohlschlegel JA, Staley JP, Sontheimer EJ. A role for ubiquitin in the spliceosome assembly pathway. Nat Struct Mol Biol 2008; 15:444 - 451
- Song EJ, Werner SL, Neubauer J, Stegmeier F, Aspden J, Rio D, et al. The Prp19 complex and the Usp4Sart3 deubiquitinating enzyme control reversible ubiquitination at the spliceosome. Genes Dev 2010; 24:1434 - 1447
- Durairaj G, Garg P, Bhaumik SR. Nuclear export of mRNA and its regulation by ubiquitylation. RNA Biol 2009; 6:531 - 535
- Gwizdek C, Hobeika M, Kus B, Ossareh-Nazari B, Dargemont C, Rodriguez MS. The mRNA nuclear export factor Hpr1 is regulated by Rsp5-mediated ubiquitylation. J Biol Chem 2005; 280:13401 - 13405
- Laroia G, Cuesta R, Brewer G, Schneider RJ. Control of mRNA decay by heat shock-ubiquitin-proteasome pathway. Science 1999; 284:499 - 502
- Thomas D, Tyers M. Transcriptional regulation: Kamikaze activators. Curr Biol 2000; 10:341 - 343
- Salghetti SE, Caudy AA, Chenoweth JG, Tansey WP. Regulation of transcriptional activation domain function by ubiquitin. Science 2001; 293:1651 - 1653
- Hoppe T, Matuschewski K, Rape M, Schlenker S, Ulrich HD, Jentsch S. Activation of a membrane-bound transcription factor by regulated ubiquitin/proteasome-dependent processing. Cell 2000; 102:577 - 586
- Palombella VJ, Rando OJ, Goldberg AL, Maniatis T. The ubiquitin-proteasome pathway is required for processing the NFκB1 precursor protein and the activation of NFκB. Cell 1994; 78:773 - 785
- Li M, Brooks CL, Wu-Baer F, Chen D, Baer R, Gu W. Mono- versus polyubiquitination: differential control of p53 fate by Mdm2. Science 2003; 302:1972 - 1975
- Lee TA, Jorgensen P, Bognar AL, Peyraud C, Thomas D, Tyers M. Systematic dissection of combinatorial control by the Met4 transcriptional complex. Mol Biol Cell 2010; 21:456 - 469
- Thomas D, Surdin-Kerjan Y. Metabolism of sulfur amino acids in Saccharomyces cerevisiae. Microbiol Mol Biol Rev 1997; 61:503 - 532
- Kaiser P, Su NY, Yen JL, Ouni I, Flick K. The yeast ubiquitin ligase SCFMet30: connecting environmental and intracellular conditions to cell division. Cell Div 2006; 1:16
- Kaiser P, Flick K, Wittenberg C, Reed SI. Regulation of transcription by ubiquitination without proteolysis: Cdc34/SCF(Met30)-mediated inactivation of the transcription factor Met4. Cell 2000; 102:303 - 314
- Rouillon A, Barbey R, Patton EE, Tyers M, Thomas D. Feedback-regulated degradation of the transcriptional activator Met4 is triggered by the SCF(Met30) complex. EMBO J 2000; 19:282 - 294
- Flick K, Ouni I, Wohlschlegel JA, Capati C, McDonald WH, Yates JR, et al. Proteolysis-independent regulation of the transcription factor Met4 by a single Lys 48-linked ubiquitin chain. Nat Cell Biol 2004; 6:634 - 641
- Kuras L, Rouillon A, Lee T, Barbey R, Tyers M, Thomas D. Dual regulation of the met4 transcription factor by ubiquitin-dependent degradation and inhibition of promoter recruitment. Mol Cell 2002; 10:69 - 80
- Chandrasekaran S, Deffenbaugh AE, Ford DA, Bailly E, Mathias N, Skowyra D. Destabilization of binding to cofactors and SCFMet30 is the rate-limiting regulatory step in degradation of polyubiquitinated Met4. Mol Cell 2006; 24:689 - 699
- Flick K, Raasi S, Zhang H, Yen JL, Kaiser P. A ubiquitin-interacting motif protects polyubiquitinated Met4 from degradation by the 26S proteasome. Nat Cell Biol 2006; 8:509 - 515
- Tyrrell A, Flick K, Kleiger G, Zhang H, Deshaies RJ, Kaiser P. Physiologically relevant and portable tandem ubiquitin-binding domain stabilizes polyubiquitylated proteins. Proc Natl Acad Sci USA 2010; 107:19796 - 19801
- Yan J, Xiong Y. Targeted ubiquitylation: the prey becomes predator. Mol Cell 2010; 40:853 - 855
- Blaiseau PL, Thomas D. Multiple transcriptional activation complexes tether the yeast activator Met4 to DNA. EMBO J 1998; 17:6327 - 6336
- Ostendorff HP, Peirano RI, Peters MA, Schluter A, Bossenz M, Scheffner M, et al. Ubiquitination-dependent cofactor exchange on LIM homeodomain transcription factors. Nature 2002; 416:99 - 103
- Gungor C, Taniguchi-Ishigaki N, Ma H, Drung A, Tursun B, Ostendorff HP, et al. Proteasomal selection of multiprotein complexes recruited by LIM homeodomain transcription factors. Proc Natl Acad Sci USA 2007; 104:15000 - 15005
- Xu Z, Meng X, Cai Y, Liang H, Nagarajan L, Brandt SJ. Single-stranded DNA-binding proteins regulate the abundance of LIM domain and LIM domain-binding proteins. Genes Dev 2007; 21:942 - 955