Abstract
Cancerous inhibitor of protein phosphatase 2A (CIP2A) has been identified as a proto-oncogene that is overexpressed in various types of human cancers. CIP2A acts by inhibiting protein phosphatase 2A-dependent destabilization of c-Myc, resulting in increased cell proliferation. Here, we have characterized the proximal promoter region of the human CIP2A gene in cervical, endometrial and liver carcinoma cells. The 5′ flanking minimal proximal promoter of the CIP2A gene consists of putative binding sites for Ets1 and Elk1 in forward and reverse orientations. Here, we show that Ets1 and Elk1 binding is essential for CIP2A basal expression in several urogenital cancer cell lines. Interestingly, both Ets1 and Elk1 are required together for CIP2A expression, as siRNA knockdown of Ets1 and Elk1 together decreased CIP2A gene transcription, whereas knockdown of Ets1 or Elk1 alone had no effect. Moreover, ectopic expression of Ets1 and Elk1 together increased CIP2A expression. To gain physiological significance of the Ets1 and Elk1 regulation we observed, a panel of matched human cervical carcinoma samples was analyzed for the expression of CIP2A and Ets1 and/or Elk1. We found a direct correlation between the levels of CIP2A and the levels of Ets1 and Elk1. Our results suggest that the binding of Ets1 and Elk1 together to the proximal CIP2A promoter is absolutely required for CIP2A expression in cervical, endometrial and liver carcinoma cell lines. Thus, different factors regulate CIP2A expression in a cell-type specific manner. As previous work has shown a requirement for only Ets1 in prostate and gastric carcinomas, our results now indicate that CIP2A regulation is more complex than previously determined.
Introduction
Cancer is a complex disease that involves genetic alterations, which lead to deregulated growth and development.Citation1 Oncogenes play an important role in cancer progression.Citation2 Mutations due to genetic or epigenetic factors in oncogenes lead to a dominant gain-of-function, such as enhanced cell growth and division, and increased cell survival.Citation3 Cancerous inhibitor of protein phosphatase 2A (CIP2A) was discovered as a 90 kDa auto-antigen found to be co-expressed in hepatocellular carcinoma (HCC) patients along with a 62 kDa auto-antigen and was observed to be overexpressed in gastric cancer tissues.Citation4 CIP2A was initially characterized as an oncoprotein that inhibits protein phosphatase 2A (PP2A)-dependent dephosphorylation of the oncoprotein c-Myc at Serine 62 (S62), thus stabilizing c-Myc activity.Citation5 Several publications have demonstrated that interaction of CIP2A with c-Myc is an important aspect in the progression of different types of cancers, such as human cervical, gastric and breast cancer cells.Citation6-Citation8 Most recently, CIP2A has been shown to regulate death associated protein kinase (DAPK) via inhibition of PP2A.Citation9
There is a wealth of data showing that CIP2A expression is elevated in many different types of cancers. Shi et al.Citation10 showed increased expression of CIP2A in prostate cancer patients in comparison to patients with benign prostatic hyperplasia (BPH). Similarly, an increase in CIP2A expression has been observed in tissue samples obtained from gastric, breast, oral, prostate, cervical, esophageal squamous cell, non-small cell lung, colon, ovarian and colorectal cancer patients.11−19 The overexpression of CIP2A in breast, non-small cell lung and early stage tongue cancer has been correlated with poor prognosis, suggesting that CIP2A levels could serve as a marker for disease progression.Citation11,Citation16,Citation20
Coenen et al.Citation21 demonstrated CIP2A to be a novel mixed lineage leukemia translocation partner in acute myeloid leukemia (AML) patients. Increased expression of CIP2A has been identified in patients with AML and chronic myeloid leukemia (CML).Citation22,Citation23 High CIP2A levels in CML patients correlate with higher risk for blast crisis due to increased phosphorylation of c-Myc.Citation23 Increased CIP2A has been identified in fibroblast-like synoviocytes (FLS), which play an important role in rheumatoid arthritis (RA) progression, suggesting CIP2A as an ideal therapeutic target.Citation24
Considering the increased expression of CIP2A in different types of cancer and its important role in development of the disease through its inhibition of PP2A tumor suppressor activity, we identified and characterized the transcription factors that control its expression. In this study, we utilized functional deletion constructs and have shown that the region between -123 and -95 of the CIP2A gene promoter is essential for the basal transcription in human cervical (HeLa), liver (HepG2) and endometrial carcinoma (ECC-1) cells. Importantly, we have demonstrated that the transcription factors Ets1 and Elk1 are together required for regulating the transcription of the CIP2A gene in human cervical and endometrial carcinoma cells. Based on our results and those from others, we suggest that targeting both Ets1 and Elk1 may be a viable therapeutic strategy for treatment of endometrial and cervical cancers.
Results
Nucleotide sequence of CIP2A gene
The CIP2A nucleotide gene sequence (GenBank accession no AC092693.8) was used to construct 5′ deletion and full length 2.4 Kbp basal promoter clones. The first exon was found between +1 and +70 nucleotides (). The 5′ flanking region upstream of the transcription start site (TSS) +1 region was considered to harbor the promoter region for transcriptional regulation of CIP2A gene.
Figure 1. Transcription factor binding sites of the CIP2A proximal promoter. The 5′ flanking region of the CIP2A promoter is shown with the transcription start site (TSS) indicated at +1. The sequence has been numbered from the TSS. The following potential transcription factor binding sites were identified: 1, Glucocorticoid receptor α; 2, Retinoic acid receptor α; 3, Pax5; 4, Ets1; 5, Elk1; 6, NF-κB; 7, Sp1; and 8, AP-2—using the ALGEN-PROMO or Ali-baba 2.0 computational software. The region between −171 and −95 has Ets1/Elk1 palindromic binding sites, which are important for basal expression of human CIP2A.
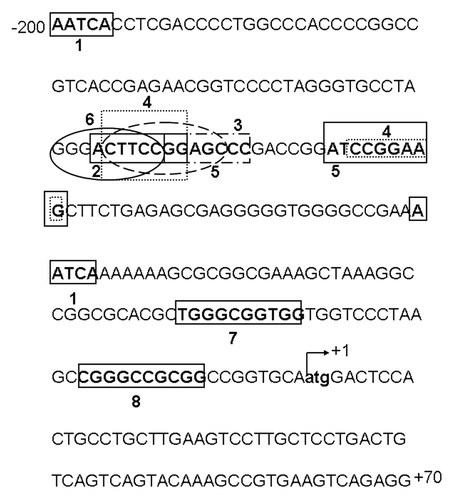
Characterization and Identification of CIP2A proximal promoter region
In order to identify functional transcription factor binding sites in the 5′ flanking region of CIP2A gene promoter, a series of PCR deletion clones were constructed in the pGL4 basic luciferase vector (). All promoter deletion clones were assayed for activity in human cervical carcinoma (HeLa) and liver hepatobalstoma (HepG2) cell lines. The fold change in relative luciferase activity (RLA) of individual deletion clones was compared with that of the pGL4 basic vector (negative control).
Figure 2. Identification of the CIP2A proximal promoter region. (A) and (D) Diagrammatic representation of the full length and sequentially deleted CIP2A promoter constructs used in this study. The transcription start site (TSS) is numbered as +1 and the constructs are numbered with reference to the TSS from 5′–3′ ends. (B) Human cervical carcinoma cells (HeLa), (C) liver hepatobalstoma cells (HepG2), and (E) endometrial carcinoma cells (ECC-1) were transfected with CIP2A promoter constructs shown in or and assayed for luciferase activity after 48 h as described in the Materials and Methods. Fold increase in relative luciferase activity (RLA) was compared with pGL4-basic (set as 1). Normalization in transfection efficiency was performed by co-transfection with pRL-TK (Renilla expression vector). The mean ± SD are from three different experiments, each performed in triplicate.
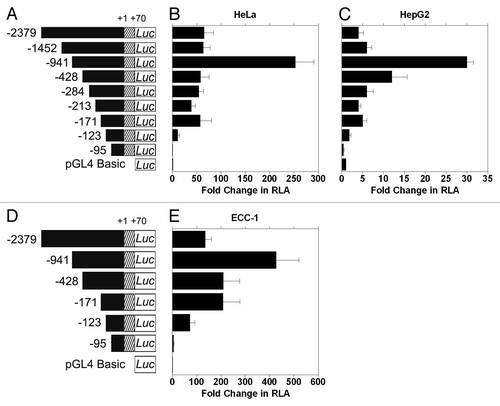
Initially, we found that the full length CIP2A promoter (-2379/+70) showed a 50-fold increase in RLA in HeLa () and a 5-fold increase in HepG2 (), compared with the basic vector, whereas the CIP2A -95/+70 clone showed no activity above background (; ). Further analyses of promoter function indicated that clone -123/+70 contained the minimal proximal promoter activity of the human CIP2A gene. Interestingly, the -941/+70 construct showed the highest increase in RLA ( and ; 253- and 30-fold for HeLa and HepG2, respectively). These data suggest that there may be enhancer and/or co-repressor binding sites upstream of the minimal proximal promoter, which we are currently investigating further. All data are summarized in and shown in -.
Table 1. Identification of human CIP2A basal proximal promoter
Due to our interest in studying female urogenital cancers, we then determined the minimal CIP2A promoter sequence housing activity in human endometrial (ECC-1) carcinoma cells. A subset of the deletion constructs () were analyzed. A 71- and 283-fold increase in RLA was observed with CIP2A -123/+70 and CIP2A -171/+70 clones, respectively (), in comparison with CIP2A -95/+70 clone, which displayed only a 5-fold increase in RLA when compared with pGL4. As was observed for HeLa and HepG2 cells, the CIP2A -941/+70 construct showed the highest activity, while the luciferase activity of the full-length construct -2379/+70 was similar to CIP2A -171/+70. Thus, the region between -171 and -95 contains the proximal promoter, and the region between -123 to -95 contains the minimal proximal promoter essential for CIP2A expression in human cervical, liver and endometrial carcinoma cells. The data are summarized in .
Identification of putative transcription factor binding sites
We analyzed the proximal promoter region (-171 to -95) of the human CIP2A gene for potential transcription factor binding sites using ALIBABA 2.0 or ALGEN-PROMO software. Binding sites for GRα (Glucocorticoid receptor α), RARα (Retinoic acid receptor α), Pax5, Ets1, Elk1, AP-2 and Sp1 were identified within -171 to +1 (). The binding sites for the Ets1 and Elk1 transcription factors were identified in reverse orientations in the region between -110/-118 and -127/-137.
Site-directed mutagenesis of transcription factor binding sites
Using PCR based site-directed mutagenesis, we introduced point mutations (underlined and in bold) or deletions (underlined and in bold italics) within several putative transcription factor binding sites (). The CIP2A −171/+70 construct was used for all of our mutagenesis studies. Mutant promoter sites were constructed as follows: point mutations for NF-κB (CIP2A Mut1), substitution and deletion mutants for the first Ets1/Elk1 binding site (CIP2A Mut2 and Mut3, respectively), point mutations for Pax5 (CIP2A Mut4), and deletion and substitution mutations for the second Ets1/Elk1 binding sites (CIP2A Mut5 and Mut6, respectively).
Figure 3. The Ets1 and Elk1 binding sites control transcription of CIP2A. (A) Six different mutants were constructed as explained in Materials and Methods. Mutated binding sites in the CIP2A promoter region are indicated in bold and are underlined. Deletions are indicated in bold, italics, and are underlined. CIP2A Mut1 targeted the binding sites for transcription factor NF-κB, CIP2A Mut2 targeted the binding site for transcription factor Ets1, CIP2A Mut3 was targeted toward the Elk1 binding site, and CIP2A Mut4 targeted the binding site for transcription factor Pax5, while CIP2A Mut5 and CIP2A Mut6 targeted the binding sites for Ets1 and Elk1. (B) HeLa and (C) ECC-1 cells were transfected with a mutant clone or the wild-type promoter (CIP2A -171/+70) and assayed for luciferase activity after 48 h as described in . Transfection efficiency was normalized by co-transfection with pRL-TK (Renilla expression vector). The mean ± SD are from three different experiments, each experiment performed in triplicate (***p < 0.001, **p < 0.01, *p < 0.05 with CIP2A −171/+70 compared with the control pGL4 basic vector and the mutant compared with CIP2A −171/+70 wild-type construct).
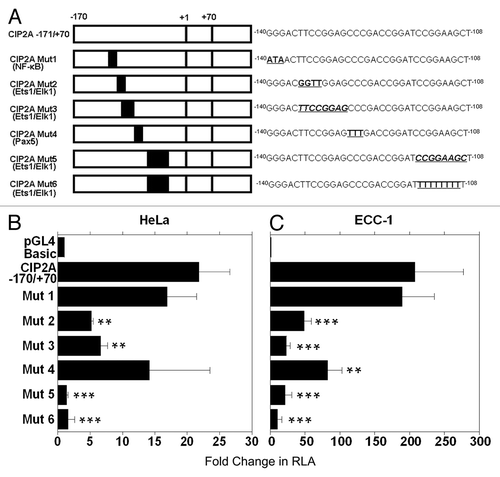
Interestingly, the CIP2A Mut2 (Ets1/Elk1) and CIP2A Mut3 (Ets1/Elk1) constructs displayed a 3 to 4-fold reduced promoter activity in the HeLa cells and a 7 to 16-fold reduction in promoter activity in the ECC-1 cells, when compared with the wild type CIP2A -171/+70 construct ( and ). In contrast, mutating the NF-κB binding site (CIP2A Mut1) did not affect the basal luciferase activity in the HeLa and ECC-1 cells. Mutation in the Pax5 binding site (CIP2A Mut4) showed cell-type specificity, as its loss in HeLa cells did not effect CIP2A transcription (), but decreased transcription by 2.5-fold in ECC-1 cells (). Importantly, when the HeLa and ECC-1 cells were transfected with CIP2A Mut5 (Ets1/Elk1, deleted binding site) a 9 to 16-fold loss in CIP2A promoter activity was observed. Similarly, the CIP2A Mut6 (Ets1/Elk1, nucleotide substitution) displayed a 13–39-fold decrease in luciferase activity compared with the wild type construct in HeLa and ECC-1 cells ( and ). These results suggest that the transcription factor binding sites for Ets1 and Elk1 together are critical for basal transcription of the CIP2A gene in human cervical and endometrial cancer cells.
In vitro binding of Ets1 and Elk1 to the CIP2A minimal proximal promoter region
Since our data suggested the requirement for Ets1 and Elk1 in driving CIP2A transcription, we next completed electrophoretic mobility shift assay (EMSA) to confirm our results. We synthesized a wild type (WT) probe harboring the Ets1 and Elk1 binding sites in the forward and reverse orientation from -138 to -107 of the CIP2A gene promoter with a consensus oligonucleotide for Ets1 and Elk1. Addition of the WT probe in the presence of nuclear extracts from ECC-1 cells displayed four protein-DNA complexes (, lane 2). Competition with 100-fold molar excess of unlabeled WT probe resulted in a complete inhibition of all four protein-DNA complexes (, lane 3). Competition with 100-fold molar excess of unlabeled mutant probe, in which the palindromic binding sites for Ets1 and Elk1 were mutated, did not abolish the fourth protein-DNA complex, though the first three complexes were inhibited (, lane 4).
Figure 4. In vitro binding of Ets1 and Elk1 to the proximal promoter region of the CIP2A gene. Electrophoretic mobility shift assay (EMSA) was performed with nuclear extracts (9 µg) ECC-1 cells that were incubated with the wild type (WT) probe (-138 to -107) from human CIP2A gene as described in Materials and Methods. In competition experiments, a 100-fold molar excess of the designated probes were utilized to demonstrate the specificity of each binding reaction. Arrows indicate the formation of specific protein–DNA complexes. The experiment was repeated twice with similar results.
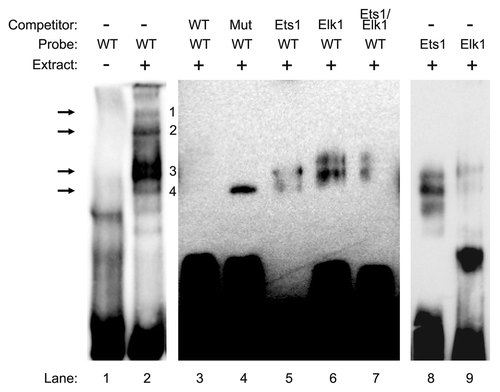
In order to confirm the loss of the fourth DNA-protein complex as the binding site for Ets1 and Elk1, a 100-fold excess molar competition was performed with the Ets1 and Elk1 consensus sequences, which led to the loss of the fourth protein-DNA complex (, lanes 5–6). Moreover, the pattern of protein-DNA complexes observed with the labeled Ets1 and Elk1 consensus probe were similar to those observed with the WT probe of the CIP2A gene (, compare lane 2 with lanes 8 and 9). These results indicate that transcription factors Ets1 and Elk1 bind to the -138 to -107 region of the CIP2A gene and regulate its transcription in endometrial carcinoma cells.
We next performed a gel-super shift analysis to further confirm our results. As a negative control, pre-immune IgG was utilized (, lane 3) and no shift was detected. Addition of Ets1 antibody to the nuclear extract from ECC-1 cells in the presence of the WT probe caused a shift in the protein DNA complex (, lane 4). Interestingly, in the presence of Elk1 antibody, the intensity of the protein-DNA complex was greatly enhanced rather than a shift (, lane 5). In the presence of both Ets1 and Elk1 antibodies, we detected a shift and an increase in the intensity of the protein-DNA complex (, lane 6). These results confirm that Ets1 and Elk1 bind to the palindromic sequence (-138 to -107) in the CIP2A promoter.
Figure 5. Confirmation of Ets1 and Elk1 binding to the proximal promoter of the CIP2A gene. Nuclear extracts (9 μg) from ECC-1 were incubated with WT probe (−138 to−107) from the CIP2A gene along with 2 μg of antibody directed toward Ets1, Elk1, or Ets1 and Elk1 together. The negative control consisted of rabbit-pre-immune control IgG. The formation of DNA–protein complexes is designated by solid arrows. The experiment was repeated twice with similar results.
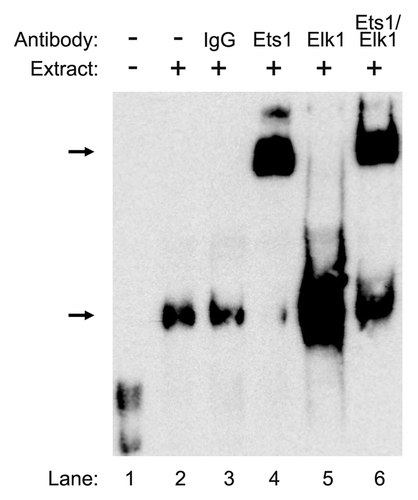
In vivo association of Ets1 and Elk1 with the CIP2A gene promoter
To confirm our in vitro data, we used chromatin immunoprecipitation (ChIP) analyses and quantitative PCR to assess the direct in vivo association of Ets1 and Elk1 with the CIP2A gene promoter. Cross-linked protein-DNA was immunoprecipitated with antibodies against Ets1, Elk1 or pre-immune IgG. Two primer sets were used to examine the specificity of Ets1 and Elk1 binding (). Region 1 contains the two binding sites for Ets1 and Elk1, while region 2 has no binding sites for the transcription factors. We found that Ets1 immunoprecipitation (IP) resulted in a significant 3-fold increase in binding to the CIP2A promoter at region 1, while IP with the Elk1 antibody resulted in a significant 2-fold increase in binding to the CIP2A promoter compared with IgG in HeLa cells (). Similarly Ets1 and Elk1 IP from ECC-1 cells showed a significant 2-fold increase in binding to CIP2A promoter occupancy compared with control IgG (). In contrast, the Ets1 and Elk1 immunoprecipitates did not amplify CIP2A promoter fragment in the region between -2379 to -2101 in either cell line ( and ). These results demonstrate that Ets1 and Elk1 associate with CIP2A gene promoter in vivo in cervical and endometrial carcinoma cells.
Figure 6. ChIP analysis of Ets1 and Elk1 binding to the CIP2A promoter. (A) Schematic representation of the Ets1 and Elk1 binding sites within the proximal promoter region. The primers utilized for amplification of DNA region are noted in . ChIP qPCR analysis of Ets1 and Elk1 association with the CIP2A promoter for region 1 in (B) HeLa and (C) ECC-1 and region 2 for (D) HeLa and (E) ECC-1 are shown. Mouse IgG serves as a negative control. Region 1 is specific for the region containing the Ets1 and Elk1 binding sites in the CIP2A promoter, while region 2 is a distal part of the CIP2A gene that is devoid of Ets1 and Elk1 binding sites. The fold enrichment from IgG, Ets1 and Elk1 immunoprecipitation are shown and were calculated relative to input as % input. The fold change in occupancy was calculated by setting the fold enrichment of IgG to 1. The results are from two different experiments, each experiment performed in duplicate (***p < 0.001, **p < 0.01, *p < 0.05 with Ets1, Elk1 compared with the control IgG).
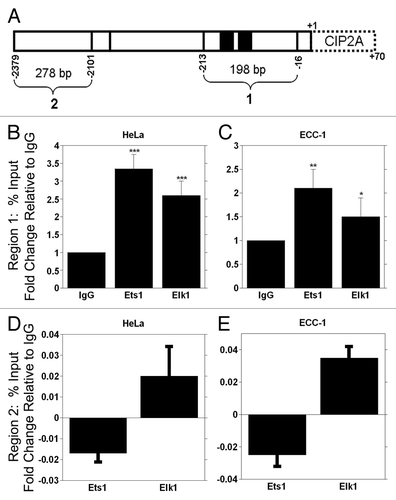
Functional analysis of Ets1 and Elk1 in CIP2A expression
Since our studies indicated that Ets1 and Elk1 transcription factors together are required for regulating basal CIP2A expression, we completed siRNA analyses in order to substantiate the specificity of Ets1 and Elk1 for CIP2A expression. HeLa cells were transfected with siRNA specific for ETS1, ELK1, or ETS1 and ELK1 together. Both siRNAs displayed specificity for the target mRNA, as ETS1 mRNA levels were altered only upon ETS1 siRNA treatment and ELK1 mRNA was only affected with ELK1 siRNA treatment, as determined by qRT-PCR analysis ( and ). A significant 40% decrease in CIP2A mRNA expression level was observed when HeLa cells were transfected with siRNA toward ETS1 and ELK1 together (), whereas no effect on CIP2A mRNA expression levels were observed when siRNA against ETS1 or ELK1 alone was used.
Figure 7. Functional analysis of Ets1 and Elk1 in regulating CIP2A transcription in HeLa cells. Cells were transiently transfected with 100 nM of ETS1, ELK1, or ETS1 and ELK1 siRNA together or non-targeting siRNA (Control) as the negative control and (A) ETS1, (B) ELK1 and (C) CIP2A mRNA expression analyzed by qRT-PCR 24 h after transfection. (D) western blot analysis of CIP2A, Ets1, Elk1 and GAPDH protein expression levels in HeLa cells tranfected with scrambled siRNA or siRNA toward ETS1, ELK1 or ETS1 and ELK1 together after 72 h.
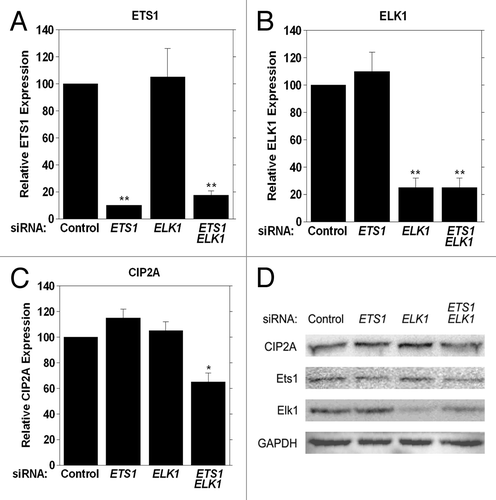
We determined endogenous CIP2A protein levels when ETS1, ELK1, or ETS1 and ELK1 were targeted with siRNA in HeLa cells 72h after siRNA transfection to determine if a decrease in mRNA expression correlated with a decrease in CIP2A protein level. As shown in , a decrease in CIP2A protein level was observed when cells were treated with both ETS1 and ELK1 siRNA together, while there was no change in CIP2A protein level in the presence of either ETS1 or ELK1 siRNA alone. Thus, a loss in Ets1/Elk1 expression (and protein level) causes a decrease in CIP2A protein level, suggesting a direct role for Ets1 and Elk1 together in the coordinate regulation of CIP2A expression.
To corroborate our observations, we completed an add-back assay to confirm the specificity for Ets1 and Elk1 in basal expression of CIP2A. We transfected HeLa cells with either non-targeting siRNA (, lane 1) or siRNA against the 3′ UTR regions of ETS1 and ELK1, effectively depleting the cells of endogenous Ets1 and Elk1 protein (, lane 2). We co-transfected cells with either empty vector (, lanes 1 and 2) or vectors overexpressing ETS1 or ELK1 or ETS1 and ELK1 together (, lane 3, 4, 5), which were resistant to ETS1 and ELK1 3′ UTR siRNA, and analyzed cell lysates by western blot. A significant decrease in CIP2A protein levels was observed in HeLa cells upon treatment with both ETS1 and ELK1 3′-UTR siRNA (, lane 2) when compared with non-targeting siRNA (, lane1). Furthermore, ectopic expression of either ETS1 or ELK1 alone did not rescue CIP2A expression in HeLa cells (, lanes 3, 4) treated with ETS1 and ELK1 3′ UTR siRNA. Importantly, the loss of CIP2A protein was rescued in HeLa cells when ETS1 and ELK1 were overexpressed together (, lane 5) in the presence of ETS1 and ELK1 3′ UTR siRNA. Importantly, CIP2A mRNA was significantly decreased when cells were treated with ETS1 and ELK1 3′ UTR siRNA (, compare 1 and 2) when analyzed by qRT-PCR, and CIP2A expression was not recovered when ETS1 or ELK1 were overexpressed individually (, compare 3 and 4 to 1). Importantly, CIP2A expression was returned to normal levels when both ETS1 and ELK1 were overexpressed (, compare 5 to 1). These results confirm the specificity of Ets1 and Elk1 transcription factors in regulating the basal transcription of CIP2A.
Figure 8. Ectopic expression of ETS1 and ELK1 together rescues CIP2A expression from 3′ UTR siRNA treatment. HeLa cells were transiently transfected with 100 nM of ETS1 and ELK1 3′ UTR siRNA together or non-targeting (NT) siRNA as the negative control. ETS1 and ELK1 cDNA were cloned into pCDN4-His Max Topo expression vector (Invitrogen, K864–20) utilizing the primers mentioned in , generating Ets1-Topo and Elk1-Topo. Sequences of the clones were verified and 1 µg was utilized for ectopic expression in HeLa cells following 3′ UTR siRNA treatment. Cells transfected with the empty vector served as a negative control. (A) western blot analysis of CIP2A, Ets1, Elk1 and GAPDH protein expression levels was performed 72 h after transfection and (B) qRT-PCR was conducted at 48 h after transfection to confirm rescue of CIP2A mRNA. **p < 0.01.
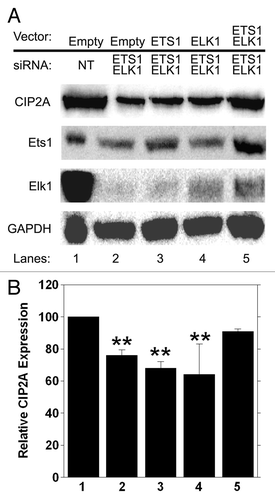
Table 4. Primers utilized for construction of Ets1, Elk1 mammalian expression vectors
Expression levels of CIP2A, Ets1 and Elk1 in human cervical tumor samples
To begin to asses the physiological relevance of our results, we examined CIP2A, Ets1 and Elk1 protein levels in human cervical tumor samples. Six different matched pairs of cervical tumor and normal adjacent tissue (NAT) were utilized for western analyses to determine the protein levels of CIP2A, Ets1, Elk1 and actin. Strikingly, we observed an increase in the protein expression levels of CIP2A in all the six tumor samples compared with the NAT samples (). Four of the six tumor samples also showed marked increase in Elk1 () protein and all five tumors tested displayed increased Ets1 () protein when compared with normal tissue samples. Importantly, all six tumor samples showed an increase in at least one transcription factor. Together, these results strongly suggest that Ets1 and Elk1 regulate the basal transcription of CIP2A in human cervical tumors.
Figure 9. Analysis of CIP2A, Ets1 and Elk1 protein expression levels in human cervical tumor samples. Six different matched pairs of human cervical tumor samples with adjacent normal tissue were obtained and protein extracted as mentioned in Materials and Methods. Patient samples are described in . The expression levels of (A) CIP2A, (B) Elk1, (C) Ets1 and Actin (as loading control) protein were observed by western blotting.
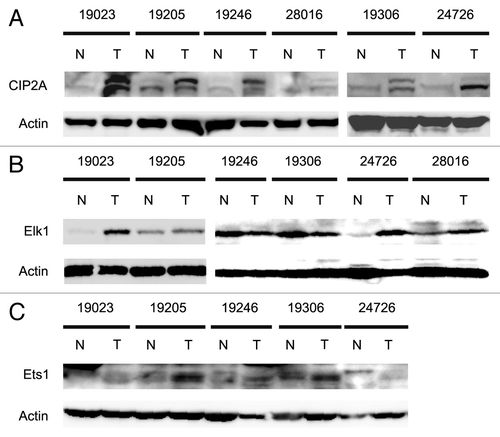
Discussion
Studies from Junttila et al.Citation6 first described the role of CIP2A as an oncogene interacting with protein phosphatase 2A (PP2A) and c-Myc. Recently, multiple publications have also described increased expression of CIP2A in various types of cancer cells and tumor samples, including gastric, breast, prostate, cervical, ovarian and colorectal cancer and a variety of other cancers.Citation7,Citation8,Citation11-Citation24 These observations suggest a direct correlation between CIP2A overexpression, cancer progression, aggressivity and poor prognosis in cancer patients.Citation11,Citation16
We are the first to elucidate the transcriptional regulation of CIP2A in female urogenital cancers. In the present study, we have functionally characterized the human CIP2A gene basal proximal and minimal proximal promoter in ECC-1 and HeLa cell lines. We provide evidence that the GGAA binding transcription factors, Ets1 and Elk1, together are involved in the basal regulation of CIP2A expression in human cervical and endometrial carcinoma cells.
Our work shows that the minimal proximal promoter of CIP2A lies between -123/+70 (). Both Ets1 and Elk1 bind to this palindromic region in order to regulate CIP2A expression ( and ). Previously, a head-to-head Ets1 binding site separated by 4 bp in the stromelysin-1 gene was identified to be essential in basal transcription of the promoter.Citation25 Moreover, the p53 promoter is regulated by Ets1 binding to palindromic Ets-binding sites.Citation26 The presence of palindromic Ets1 binding sites enhances an association between Ets1/Ets1 homodimers by creating a stable interaction in the DNA binding domain, which overcomes the auto-inhibitory domain in the Ets-DNA binding region.Citation27 The Ets-DNA binding domain is flanked by N- and C-terminal inhibitory regions that cause auto-inhibition and impair DNA binding.Citation28
We observed a decrease in CIP2A luciferase activity in ECC-1 cells when the Mut4 construct was expressed (); this construct harbors a mutation in the Pax5 binding site. However, no significant decrease in CIP2A promoter activity was seen when compared with the minimal proximal promoter region (-123/+70, data not shown), which suggests that Pax5 is not the major transcription factor regulating CIP2A in ECC-1 cells. The decreased luciferase activity due to expression of CIP2A Mut4 might be due to cooperative interactions between Pax5 and Elk1 as characterized in earlier studies.Citation29 Previous work has demonstrated that Pax5 recruits Elk1 and Net transcription factors to form a functional ternary complex in the B-cell specific promoter. Structural studies with Pax5 and Ets1 have shown a change in the Ets1 nucleotide binding motif from GGAA to GGAG upon interaction with Pax5.Citation30,Citation31 Additionally, a recent observation by Khanna et al.Citation32 has described mutations in the overlapping Ets1 binding sites within the CIP2A gene promoter, which result in decreased promoter activity in human gastric adenocarcinoma (AG1478) cells. Thus, it is possible that different regions of the CIP2A gene regulate basal transcription in different cell types.
The importance of Ets1 and Elk1 in controlling the basal transcription of CIP2A gene was obtained using EMSA () and gel super-shift () assays. Interestingly, we did not detect a shift when incubating nuclear extracts with the anti-Elk1 antibody; rather, we detected an increase in the intensity of the protein-DNA complex (, lane 5). The increase in intensity also indicates a positive interaction of the antibody with protein-DNA complex, as previous observations by Kurachi et al.Citation53 have shown a similar increase in protein-DNA complex in the presence of anti-Ets1 antibody. It could be possible that a small shift in the presence of anti-Elk1, as observed by Yang et al.,Citation54 was masked by an increased protein-DNA interaction. These results confirm the binding of Ets1 and Elk1 transcription factor(s) in the region between -138 and -107 of the CIP2A gene promoter in endometrial carcinoma cells.
Using ChIP analyses, we showed that Ets1 and Elk1 bind to the CIP2A promoter in HeLa and ECC-1 cells ( and ). We are the first to demonstrate that Elk1 regulates CIP2A expression, and to suggest that Elk1 and Ets1 coordinately regulate expression in human cervical and endometrial cancer cells. Based on these results, we believe that any attempt to therapeutically decrease CIP2A expression through inhibition of specific transcription factors will be guided by what type of cancer is being treated. In the case of cervical and endometrial cancers, two inhibitors may be required to decrease CIP2A expression efficiently.
We have shown that Ets1 and Elk1 together control the basal transcription of CIP2A ( and ) in female urogenital cancers. It has been observed that knockdown of the Ets1 transcription factor alone decreases CIP2A protein levels in human gastric (AG1478) and prostate (PC-3 and LNCaP) carcinoma cells.Citation32 We confirmed these results in PC-3 cells (data not shown), suggesting that there is cell-type specific regulation of CIP2A. The siRNA rescue experiment () further demonstrates the necessity for Ets1 and Elk1 together in cell-type specific regulation of the CIP2A gene. Thus, Ets1 and Elk1 are essential for basal transcription of CIP2A in endometrial and cervical carcinoma cell lines. The differences in our results from those of others can be explained by cell-type specific regulation of gene expression, such as previously reported for AKR1C1 transcription. Sp1 was found to regulate AKR1C1 transcription in human colon cancer cells resistant to methotrexate,Citation33 while the same gene was regulated by nuclear factor-Y in human ovarian, liver and lung carcinoma cells.Citation34
The E-26-specific (Ets) family is comprised of 29 transcription factors involved in regulating and inhibiting the expression of various genes involved in several biological processes, such as apoptosis, development, differentiation, proliferation and transformation.Citation35-Citation37 The Ets DNA binding domain binds to the GGA(A/T) core motif referred to as the Ets binding site (EBS).Citation38 In the present study, the GGAA core binding motif was identified in the CIP2A promoter region in a palindromic orientation and found to be fully functional. Ets like kinase 1 (Elk1) is a ternary complex factor (TCF) initially identified to regulate the c-fos promoter in cooperation with serum response elements (SRE).Citation39,Citation40 The oligonucleotide consensus sequence CCGGAAGTR shows more affinity for Elk1 binding in association with a second transcription factor SRE, which binds to the consensus sequence CC(A/T)6GG.Citation41,Citation42 Microarray analysis by Boros et al.Citation43,Citation44 identified a large group of promoters that function through Elk1 independently of the SRE binding region. We show, however, that the regulation of CIP2A by Elk1 is SRE-independent but does require Ets1 promoter binding.
Since the Ets families of proteins share the EBS binding motif, it is challenging to identify the specific transcription factor involved in gene regulation based solely on binding to this element. Moreover, the Ets family proteins have a varied tissue distribution: Ets1, Erg, Fli-1, ETV4, Elf1, ESE-1 and PU.1 are found in lymphoid organs, brain, vascular endothelial cells, hematopoietic cells, kidney, liver, epithelial cells, B cells, macrophages and neutrophils, while Ets2, GABPα, TEL and TCFs are found to be ubiquitous.Citation36 Based on our results and the data of others, it is evident that the complex distribution of Ets family members may play a role in regulating the tissue-specific expression of CIP2A. Microarray analysis demonstrated the possibility of overlapping promoter binding patterns for the Ets transcription factors Ets1, Elf1 and GABPα.Citation45 Similarly, our data demonstrate that both Ets family members, Ets1 and Elk1, cooperatively bind and are required for CIP2A expression in human cervical and endometrial carcinoma cells. Interestingly, Ets1 and Elk1 together have recently been identified to regulate the expression of dipeptidyl-peptidase III (DPP-III) gene, which is involved in ovarian cancer aggressivity.Citation46
CIP2A aids in the development of drug resistance in cancer cells. Recent reports have correlated the overexpression of CIP2A with development of doxorubicin resistance in breast cancer cells (MDA-MB-231)Citation47 and overexpression of CIP2A in hepatocellular carcinoma cells (HCC) PLC5 leads to resistance to bortezomib.Citation48 The development of drug resistance and increased CIP2A expression can be correlated with Ets1 expression levels, as previous reports have observed a direct relationship between Ets1 expression and cisplatin and gemcitabine resistance in human ovarian and pancreatic carcinoma cells.Citation49,Citation50 Our results show a correlation in the protein expression levels of CIP2A, Ets1 and Elk1 in six matched pairs of human cervical tumor samples. Increased CIP2A protein correlated with an increase in the Elk1 and/or Ets1 expression levels (). Importantly, each tumor sample showed increased expression of either Ets1 or Elk1 alone or together. The combined identification of CIP2A expression and expression of transcription factors regulating CIP2A expression may prove to be a prognostic marker in human cervical carcinoma.
Materials and Methods
Cell Culture. The human cervical carcinoma cell line HeLa was grown in DMEM supplemented with 10% fetal calf serum (Invitrogen, 16140071) and penicillin-streptomycin (Sigma, P433) at a final concentration of 100 µg/ml. The hepatocellular carcinoma cells HepG2 were maintained in DMEM (Invitrogen, 11995065) with 10% FCS and gentamicin (Invitrogen, 15710064) at a final concentration of 10 µg/ml. The endometrial carcinoma cells ECC-1 were grown in RPMI-1640 (Invitrogen, 11875093) supplemented with 5% FCS. All cells were grown at 37°C in a 5% CO2 incubator.
Human cervical carcinoma tissue samples
Six matched pair of human cervical carcinoma tissue samples were purchased from ILS Bio, LLC (Chestertown, MD) and were analyzed for protein levels of CIP2A, Ets1, and Elk1. Sample descriptions are provided in .
Table 2. Pathological characteristics of human cervical carcinoma tissue samples
Construction of CIP2A luciferase reporter vector and 5′ deletion analysis
The BAC clone RP11–161J9 (Rosewell Parker Cancer Institute Human BAC library) that harbors the 5′ flanking region of CIP2A gene (GenBank accession no AC092693.8) was utilized to design the primers for construction of CIP2A promoter-luciferase plasmids. DNA isolated from HeLa cells was used as templates to generate PCR fragments using Taq polymerase (Takara, RR350A), which were cloned into the reporter vector at the NheI-XhoI (New England Biolabs, R0131, R0146) poly-cloning sites by incorporating the corresponding restriction sties in the forward and reverse primers. The full length construct -2379/+70 luciferase promoter consisting of an approximately 2.4 kbp region upstream and 70 bp downstream of the transcription start site (TSS) was cloned into the pGL4.10 [luc2] vector (Promega, E6651). PCR amplified promoter regions -1452/+70, -941/+70, -428/+70, -284/+70, -213/+70, -171/+70, -123/+70 and -95/+70 were cloned in NheI-XhoI sites of pGL4-basic vector. The nucleotide sequence of the clones was verified by sequencing.
Transient transfection and luciferase assay
Cells were seeded in 6-well plates at a density of 5x105 cells/well for HeLa, HepG2 and 8x105 for Ecc1 cells, 24 h before transfection. Transfection was performed utilizing Lipofectamine 2000 reagent (Invitrogen, 11668019) following manufactures recommendations. In each experiment, 2 μg of control vector (pGL4-basic without CIP2A promoter insert, empty vector) or the reporter vector (CIP2A full length promoter fragment or sequentially deleted CIP2A PCR fragments in pGL4-basic vector) was co-transfected along with 250 ng of pRL-TK (Renilla luciferase, Promega, E2241) as an internal control. Following incubation with DNA for 4 h cells were feed with 2 mL of fresh growth medium for an additional 44 h. Luciferase assay was performed as described by Pallai et al.Citation34 utilizing a 384 well robotic plate reader (Envison, Perkin Elmer).
Identification of potential putative transcription factor binding sites in CIP2A gene promoter
Potential transcription factor binding sites within the CIP2A gene promoter were screened using ALGEN-PROMOCitation51,Citation52 or ALIBABA 2.0 programs (www.gene-regulation.com).
Site-directed Mutagenesis
The −171/+70 CIP2A proximal promoter fragment was used to generate mutant clones of the CIP2A promoter. The Quickchange lightning site-directed mutagenesis kit (Stratagene, 210518) was utilized to generate mutants CIP2A Mut1-Mut6. Primers for introduction of point mutations or deletions were designed as instructed by the manufacturer. The nucleotide sequence of the mutated clones was verified by sequencing. The promoter activity of the mutated clones was assayed by transient transfection and luciferase assay as described above.
Electrophoretic mobility shift assay (EMSA) and gel super-shift
Nuclear extracts were prepared from ECC-1 cells. 1x107 cells were seeded in 75 cm2 flasks 24 h before nuclear proteins were extracted utilizing the nuclear complex CO-IP kit (Active Motif, 54001) as instructed by the manufacturer. The wild type and mutant probes were synthesized as double stranded oligonucleotides (Integrated DNA technology) from the -138 to -107 region of the CIP2A gene promoter. Consensus oligonucleotides for Ets1 and Elk1 were synthesized based on the sequence data from Santa Cruz Biotechnology (Santa Cruz, CA) and Panomics (Affymetrix, CA). The sequences of the probes utilized were: WT -5′-GACTTCCGGAGCCCGACCGGATCCGGAAGCTT-3′; Mutant - 5′-GAAAATTTAAGCCCGACCGGATAAATTTACTT-3′ (mutated bases shown in bold text); Et1s- 5′-GATCTCGAGCAGGAAGTTCGA-3′; Elk1 - 5′- TTTGCAAAATGCAGGAATTGTTTTCACAGT-3′. All the probes were labeled with biotin using the Biotin 3′-end DNA labeling kit (Thermo Scientific, 89818) according to the manual. Nine micrograms of the nuclear extract was utilized for the binding reactions. The EMSA binding reactions were performed at room temperature for 30 min and consisted of the nuclear extract in 1 × binding buffer (50% glycerol, 100 mM MgCl2, 1μg/μl Poly (dI–dC), 1% NP-40, 1 M KCl, 200 mM EDTA and 5 µM DNA probe). The mixture was run on 8% non-denaturing polyacrylamide gels in 0.5 × Tris Borate-EDTA buffer at 170 V. The protein–DNA complexes were then transferred to Hybond-N+ nylon membrane using the Trans-Blot semi-dry method (Bio-Rad, CA) and cross-linked using the Spectrolinker XL-1000 UV cross-linker (Spectronics Corporation, NY). Detection of biotin-labeled DNA was performed using the LightShift chemiluminescent EMSA kit (Thermo Scientific, 20148) and visualized by exposure to a charge-couple device camera (GE ImageQuant LAS 4000).
For competition EMSA, 100-fold molar excess of the cold, mutant or consensus oligonucleotides were added to the EMSA binding reaction. For the gel-Supershift assay, following the incubation of the nuclear extracts with the 29 bp WT CIP2A promoter fragment for 30 min, 5 μg of Ets1 antibody (Abcam, ab124282), 5 μg of Elk1 antibody (Epitomics, 1277–1) and/or the two antibodies (anti-Ets1 and Elk1) were added to the binding reaction and the mixture incubated at RT for an additional 30 min. The pre-immune IgG (Millipore, 12–371) was utilized as negative control in the Supershift assay. The mixture was fractionated on 5% non-denaturing polyacrylamide gel. Transfer and detection was performed as described above.
Ets1/Elk1 siRNA knockdown, siRNA rescue, and CIP2A expression analysis
Human siRNA specific for Ets1 (Dharmacon, J-003887–06, J-003887–08), Elk1 (Dharmacon, J-003885–06, J-003885–08) or Ets1/Elk1 together were utilized. siGENOME non-targeting siRNA pool 1 (Dharmacon, D-001206–13) was utilized as negative control. The human cervical carcinoma cell line HeLa were seeded at a density of 5x105 cells/well and were transiently transfected with 100 nM of each of the targeted siRNA or in combination utilizing Lipofectamine 2000 (Invitrogen). RNA and protein were isolated 24 h and 72 h after transfection for CIP2A expression levels.
One microgram of the RNA was used in the reverse transcription reaction along with 4 U of Omniscript reverse transcriptase (Qiagen, 205110), 1 μM oligo-dT primer (Qiagen, 79237), 0.5 mM dNTP (Qiagen, 205110), 10 U of RNase inhibitor (Qiagen, 129916) and 1 × RT buffer (Qiagen, 205110). Reverse transcription was performed at 37°C for 1 h with a final incubation at 93°C for 5 min for inactivation of reverse transcriptase. Two microliters of the product was used in the real-time PCR reaction. The Quantitect SYBR green PCR kit (Qiagen, 204163) was utilized and PCR was performed according to the manufacturer's instructions using the Stratagene MxPro 3000 real time RT cycler (Agilent Technologies). Each PCR reaction consisted of 50% (v/v) of 2 X SYBR green master mixes and 0.2 μM gene-specific forward and reverse primers. Quantification of glyceraldehyde-6-phosphate dehydrogenase was used to normalize the relative expression levels of CIP2A, ETS1 and ELK1 mRNA. Each experiment was performed in duplicates and repeated at least twice.
For the siRNA rescue experiment, 5x105 HeLa cells seeded in 6 well plates were transiently transfected with ETS1 3′-UTR siRNA and ELK1 3′-UTR siRNA (Dharmacon). siGENOME non-targeting siRNA pool 1 (Dharmacon) was utilized as the negative control. Transfections were performed with 100 nM of the targeted 3′-UTR siRNA in combination or the non-targeting pool with Lipofectamine RNAiMax (Invitrogen) as described by the manufacturer. Forty-eight hours after 3′-UTR siRNA treatment, cells were transiently transfected with 1 µg of Ets1-Topo and Elk1-Topo mammalian expression vector encoding respective cDNAs utilizing Lipofectamine RNAiMax (Invitrogen). HeLa cells transfected with empty vector served as a negative control. Protein was extracted 72 h after transfection for CIP2A analysis. Rabbit anti-CIP2A (Novus, NB100–68264), rabbit anti-Ets1 (Abcam, ab124282), rabbit-anti-Elk1 (Epitomics, 1277–1) and rabbit ant-GAPDH (Abcam, ab9385) were utilized for western blotting.
Chromatin immunoprecipitation assay
Chromatin immunoprecipitation was performed using 1–2x107 HeLa or ECC-1 cells, which were treated with 37% formaldehyde (Sigma, F1635) at 1% final concentration, v/v for 10 min at room temperature to cross-link proteins to DNA. After cross-linking the cells were washed twice with 1 × ice-cold PBS containing protease inhibitor cocktail (Sigma, P8340). The cells were collected and centrifuged at 700 × g for 2 min, resuspended in 1 ml of SDS-lysis buffer with protease inhibitor cocktail. The cells were then sonicated twice with a bioruptor (Diagenode, UCD200) at high power, with 30 sec on/off pulse for 15 min. The cell lysate was centrifuged at 10,000 × g for 15 min at 4°C and the supernatant was further subjected to enzymatic digestion utilizing micrococcal nuclease (New England Biolabs, M0247) for 15 min at 37°C. The enzyme activity was inactivated by adding 0.5 M ETDA and incubated on ice for 10 min. Twenty-five microliters of the DNA fraction was kept aside as input for PCR. The remaining DNA fraction was pre-cleared using a mixture of 35 μl of protein G and protein A agarose beads each (50% slurry, Millipore, 16–201, 16–157) for 2 h at 4°C. Immunoprecipitation was performed by adding antibodies toward Ets1 (Abcam, ab124282), Elk1 (Epitomics, 1277–1) or mouse IgG (Millipore, 12–371) as the negative control. The immunocomplex was precipitated by incubation with 70 μl of protein A/G agarose beads for 2 h at 4°C. The protein/DNA complex was eluted using 200 μl of elution buffer (1% SDS, 0.1 M NaHCO3) from the beads. Cross-linking of protein–DNA was reversed by adding 10 μl of 5 M NaCl at 65°C for 2–3h. The DNA was purified using spin columns (Promega, A9281) and 5 μl of the DNA was used in the qPCR reaction for amplification of 198 bp or 298 bp of the CIP2A promoter region (). qPCR reactions were performed utilizing the primers in .
Table 3. Primers utilized for chromatin immunoprecipitation
Western Blot Analysis
Protein was isolated from cells by washing the cells in 1X ice-cold PBS and resuspending in 250 μl of RIPA buffer supplemented with protease inhibitor cocktail (Sigma, P8340). The cells were then sonicated with the bioruptor (Diagenode, UCD200) at high power, with 30 sec on/off pulse for 10 min. The cell lysate was centrifuged at 10,000 × g for 30 min at 4°C and 50 μg of protein was fractioned on 10% or 12% SDS-PAGE and transferred to a 0.45 μm nitrocellulose membrane, blocked at 4°C overnight in 5% non-fat milk in TBS and incubated with rabbit anti-CIP2A (Novus, NB100–68264), rabbit anti-Elk1 (Novus, NB110–56953), rabbit anti-Ets1 (Novus, NBL1–10355) and rabbit anti-GAPDH (Abcam, ab9485) at 4°C overnight. Blots were then washed with 1X TBST and incubated with anti-rabbit secondary HRP at room temperature for 1h. Detection of signal was performed by adding chemiluminescent substrate (Millipore, WBKLS0500) and visualized by exposure to a charge-couple device camera (GE ImageQuant LAS 4000).
Statistical Analysis
Statistical analysis was performed for calculating the significant differences in luciferase activity between constructs, effect of siRNA in knock-down of CIP2A mRNA expression and effect of Ets1, Elk1 knock-down in CIP2A mRNA by one way randomized analysis of variance (ANOVA) and Newman-Keuls test with significance level of p < 0.05.
Abbreviations: | ||
CIP2A | = | cancerous inhibitor of protein phosphatase 2A |
PP2A | = | protein phosphatase 2A |
Ets1 | = | E-twenty six |
Elk1 | = | Ets like kinase1 |
RLA | = | relative luciferase activity |
TSS | = | transcription start site |
Acknowledgments
We would like to thank Dr. E. Mordechai, Dr. M. Adelson, Dr. J.T. Nickels and Dr. M. Webb for helpful discussions regarding this work. We further thank Genesis Biotechnology Group, LLC for financial support of this work.
Disclosure of Potential Conflicts of Interest
The authors have no conflicts of interest.
Funding
This work was supported by Genesis Biotechnology Group, LLC.
References
- Hanahan D, Weinberg RA. The hallmarks of cancer. Cell 2000; 100:57 - 70; http://dx.doi.org/10.1016/S0092-8674(00)81683-9; PMID: 10647931
- Bishop JM, Weinberg RA, eds. Scientific American molecular oncology. New York: Scientific American, Inc., 1996.
- Isaacs W, Kainu T. Oncogenes and tumor suppressor genes in prostate cancer. Epidemiol Rev 2001; 23:36 - 41; http://dx.doi.org/10.1093/oxfordjournals.epirev.a000794; PMID: 11588853
- Soo Hoo L, Zhang JY, Chan EKL. Cloning and characterization of a novel 90 kDa ‘companion’ auto-antigen of p62 overexpressed in cancer. Oncogene 2002; 21:5006 - 15; http://dx.doi.org/10.1038/sj.onc.1205625; PMID: 12118381
- Junttila MR, Westermarck J. Mechanisms of MYC stabilization in human malignancies. Cell Cycle 2008; 7:592 - 6; http://dx.doi.org/10.4161/cc.7.5.5492; PMID: 18256542
- Junttila MR, Puustinen P, Niemelä M, Ahola R, Arnold H, Böttzauw T, et al. CIP2A inhibits PP2A in human malignancies. Cell 2007; 130:51 - 62; http://dx.doi.org/10.1016/j.cell.2007.04.044; PMID: 17632056
- Li W, Ge Z, Liu C, Liu Z, Björkholm M, Jia J, et al. CIP2A is overexpressed in gastric cancer and its depletion leads to impaired clonogenicity, senescence, or differentiation of tumor cells. Clin Cancer Res 2008; 14:3722 - 8; http://dx.doi.org/10.1158/1078-0432.CCR-07-4137; PMID: 18559589
- Côme C, Laine A, Chanrion M, Edgren H, Mattila E, Liu X, et al. CIP2A is associated with human breast cancer aggressivity. Clin Cancer Res 2009; 15:5092 - 100; http://dx.doi.org/10.1158/1078-0432.CCR-08-3283; PMID: 19671842
- Guenebeaud C, Goldschneider D, Castets M, Guix C, Chazot G, Delloye-Bourgeois C, et al. The dependence receptor UNC5H2/B triggers apoptosis via PP2A-mediated dephosphorylation of DAP kinase. Mol Cell 2010; 40:863 - 76; http://dx.doi.org/10.1016/j.molcel.2010.11.021; PMID: 21172653
- Shi FD, Zhang JY, Liu D, Rearden A, Elliot M, Nachtsheim D, et al. Preferential humoral immune response in prostate cancer to cellular proteins p90 and p62 in a panel of tumor-associated antigens. Prostate 2005; 63:252 - 8; http://dx.doi.org/10.1002/pros.20181; PMID: 15538718
- Khanna A, Böckelman C, Hemmes A, Junttila MR, Wiksten JP, Lundin M, et al. MYC-dependent regulation and prognostic role of CIP2A in gastric cancer. J Natl Cancer Inst 2009; 101:793 - 805; http://dx.doi.org/10.1093/jnci/djp103; PMID: 19470954
- Katz J, Jakymiw A, Ducksworth MK, Stewart CM, Bhattacharyya I, Cha S, et al. CIP2A expression and localization in oral carcinoma and dysplasia. Cancer Biol Ther 2010; 10:694 - 9; http://dx.doi.org/10.4161/cbt.10.7.12895; PMID: 21068540
- Vaarala MH, Väisänen MR, Ristimäki A. CIP2A expression is increased in prostate cancer. J Exp Clin Cancer Res 2010; 29:136 - 9; http://dx.doi.org/10.1186/1756-9966-29-136; PMID: 20964854
- Liu J, Wang X, Zhou G, Wang H, Xiang L, Cheng Y, et al. Cancerous inhibitor of protein phosphatase 2A is overexpressed in cervical cancer and upregulated by human papillomavirus 16 E7 oncoprotein. Gynecol Oncol 2011; 122:430 - 6; http://dx.doi.org/10.1016/j.ygyno.2011.04.031; PMID: 21575984
- Qu W, Li W, Wei L, Xing L, Wang X, Yu J. CIP2A is overexpressed in esophageal squamous cell carcinoma. Med Oncol 2012; 29:113 - 8; http://dx.doi.org/10.1007/s12032-010-9768-9; PMID: 21140243
- Dong QZ, Wang Y, Dong XJ, Li ZX, Tang ZP, Cui QZ, et al. CIP2A is overexpressed in non-small cell lung cancer and correlates with poor prognosis. Ann Surg Oncol 2011; 18:857 - 65; http://dx.doi.org/10.1245/s10434-010-1313-8; PMID: 20842459
- Böckelman C, Lassus H, Hemmes A, Leminen A, Westermarck J, Haglund C, et al. Prognostic role of CIP2A expression in serous ovarian cancer. Br J Cancer 2011; 105:989 - 95; http://dx.doi.org/10.1038/bjc.2011.346; PMID: 21897396
- Teng HW, Yang SH, Lin JK, Chen WS, Lin TC, Jiang JK, et al. CIP2A is a predictor of poor prognosis in colon cancer. J Gastrointest Surg 2012; 16:1037 - 47; http://dx.doi.org/10.1007/s11605-012-1828-3; PMID: 22328001
- Böckelman C, Koskensalo S, Hagström J, Lundin M, Ristimäki A, Haglund C. CIP2A overexpression is associated with c-Myc expression in colorectal cancer. Cancer Biol Ther 2012; 13:289 - 95; http://dx.doi.org/10.4161/cbt.18922; PMID: 22310977
- Böckelman C, Hagström J, Mäkinen LK, Keski-Säntti H, Häyry V, Lundin J, et al. High CIP2A immunoreactivity is an independent prognostic indicator in early-stage tongue cancer. Br J Cancer 2011; 104:1890 - 5; http://dx.doi.org/10.1038/bjc.2011.167; PMID: 21610708
- Coenen EA, Zwaan CM, Meyer C, Marschalek R, Pieters R, van der Veken LT, et al. KIAA1524: A novel MLL translocation partner in acute myeloid leukemia. Leuk Res 2011; 35:133 - 5; http://dx.doi.org/10.1016/j.leukres.2010.08.017; PMID: 20943269
- Wang J, Li W, Li L, Yu X, Jia J, Chen C. CIP2A is over-expressed in acute myeloid leukemia and associated with HL60 cells proliferation and differentiation. Int Jnl Lab Hem 2011; 33:290 - 8; http://dx.doi.org/10.1111/j.1751-553X.2010.01288.x
- Lucas CM, Harris RJ, Giannoudis A, Copland M, Slupsky JR, Clark RE. Cancerous inhibitor of PP2A (CIP2A) at diagnosis of chronic myeloid leukemia is a critical determinant of disease progression. Blood 2011; 117:6660 - 8; http://dx.doi.org/10.1182/blood-2010-08-304477; PMID: 21490338
- Lee J, Park EJ, Hwang JW, Oh JM, Kim H, Bae EK, et al. CIP2A expression is associated with synovial hyperplasia and invasive function of fibroblast-like synoviocytes in rheumatoid arthritis. Rheumatol Int 2012; 32:2023 - 30; http://dx.doi.org/10.1007/s00296-011-1927-6; PMID: 21479604
- Baillat D, Bègue A, Stéhelin D, Aumercier M. ETS-1 transcription factor binds cooperatively to the palindromic head to head ETS-binding sites of the stromelysin-1 promoter by counteracting autoinhibition. J Biol Chem 2002; 277:29386 - 98; http://dx.doi.org/10.1074/jbc.M200088200; PMID: 12034715
- Baillat D, Laitem C, Leprivier G, Margerin C, Aumercier M. Ets-1 binds cooperatively to the palindromic Ets-binding sites in the p53 promoter. Biochem Biophys Res Commun 2009; 378:213 - 7; http://dx.doi.org/10.1016/j.bbrc.2008.11.035; PMID: 19022222
- Babayeva ND, Wilder PJ, Shiina M, Mino K, Desler M, Ogata K, et al. Structural basis of Ets1 cooperative binding to palindromic sequences on stromelysin-1 promoter DNA. Cell Cycle 2010; 9:3054 - 62; http://dx.doi.org/10.4161/cc.9.14.12257; PMID: 20686355
- Garvie CW, Pufall MA, Graves BJ, Wolberger C. Structural analysis of the autoinhibition of Ets-1 and its role in protein partnerships. J Biol Chem 2002; 277:45529 - 36; http://dx.doi.org/10.1074/jbc.M206327200; PMID: 12221090
- Fitzsimmons D, Hodsdon W, Wheat W, Maira SM, Wasylyk B, Hagman J. Pax-5 (BSAP) recruits Ets proto-oncogene family proteins to form functional ternary complexes on a B-cell-specific promoter. Genes Dev 1996; 10:2198 - 211; http://dx.doi.org/10.1101/gad.10.17.2198; PMID: 8804314
- Garvie CW, Hagman J, Wolberger C. Structural studies of Ets-1/Pax5 complex formation on DNA. Mol Cell 2001; 8:1267 - 76; http://dx.doi.org/10.1016/S1097-2765(01)00410-5; PMID: 11779502
- Pufall MA, Graves BJ. Ets-1 flips for new partner Pax-5. Structure 2002; 10:11 - 4; http://dx.doi.org/10.1016/S0969-2126(01)00701-8; PMID: 11796106
- Khanna A, Okkeri J, Bilgen T, Tiirikka T, Vihinen M, Visakorpi T, et al. ETS1 mediates MEK1/2-dependent overexpression of cancerous inhibitor of protein phosphatase 2A (CIP2A) in human cancer cells. PLoS One 2011; 6:e17979; http://dx.doi.org/10.1371/journal.pone.0017979; PMID: 21445343
- Selga E, Noé V, Ciudad CJ. Transcriptional regulation of aldo-keto reductase 1C1 in HT29 human colon cancer cells resistant to methotrexate: role in the cell cycle and apoptosis. Biochem Pharmacol 2008; 75:414 - 26; http://dx.doi.org/10.1016/j.bcp.2007.08.034; PMID: 17945194
- Pallai R, Simpkins H, Chen J, Parekh HK. The CCAAT box binding transcription factor, nuclear factor-Y (NF-Y) regulates transcription of human aldo-keto reductase 1C1 (AKR1C1) gene. Gene 2010; 459:11 - 23; http://dx.doi.org/10.1016/j.gene.2010.03.006; PMID: 20338228
- Shore P, Sharrocks AD. The ETS-domain transcription factors Elk-1 and SAP-1 exhibit differential DNA binding specificities. Nucleic Acids Res 1995; 23:4698 - 706; http://dx.doi.org/10.1093/nar/23.22.4698; PMID: 8524663
- Oikawa T, Yamada T. Molecular biology of the Ets family of transcription factors. Gene 2003; 303:11 - 34; http://dx.doi.org/10.1016/S0378-1119(02)01156-3; PMID: 12559563
- Dittmer J. The biology of the Ets1 proto-oncogene. Mol Cancer 2003; 2:29; http://dx.doi.org/10.1186/1476-4598-2-29; PMID: 12971829
- Kodandapani R, Pio F, Ni CZ, Piccialli G, Klemsz M, McKercher S, et al. A new pattern for helix-turn-helix recognition revealed by the PU.1 ETS-domain-DNA complex. Nature 1996; 380:456 - 60; http://dx.doi.org/10.1038/380456a0; PMID: 8602247
- Treisman R. The serum response element. Trends Biochem Sci 1992; 17:423 - 6; http://dx.doi.org/10.1016/0968-0004(92)90013-Y; PMID: 1455511
- Shore P, Bisset L, Lakey J, Waltho JP, Virden R, Sharrocks AD. Characterization of the Elk-1 ETS DNA-binding domain. J Biol Chem 1995; 270:5805 - 11; http://dx.doi.org/10.1074/jbc.270.11.5805; PMID: 7890710
- Sharrocks AD. Complexities in ETS-domain transcription factor function and regulation: lessons from the TCF (ternary complex factor) subfamily. The Colworth Medal Lecture. Biochem Soc Trans 2002; 30:1 - 9; http://dx.doi.org/10.1042/BST0300001; PMID: 12023815
- Shaw PE, Saxton J. Ternary complex factors: prime nuclear targets for mitogen-activated protein kinases. Int J Biochem Cell Biol 2003; 35:1210 - 26; http://dx.doi.org/10.1016/S1357-2725(03)00031-1; PMID: 12757758
- Boros J, Donaldson IJ, O’Donnell A, Odrowaz ZA, Zeef L, Lupien M, et al. Elucidation of the ELK1 target gene network reveals a role in the coordinate regulation of core components of the gene regulation machinery. Genome Res 2009; 19:1963 - 73; http://dx.doi.org/10.1101/gr.093047.109; PMID: 19687146
- Boros J, O’Donnell A, Donaldson IJ, Kasza A, Zeef L, Sharrocks AD. Overlapping promoter targeting by Elk-1 and other divergent ETS-domain transcription factor family members. Nucleic Acids Res 2009; 37:7368 - 80; http://dx.doi.org/10.1093/nar/gkp804; PMID: 19789270
- Hollenhorst PC, Shah AA, Hopkins C, Graves BJ. Genome-wide analyses reveal properties of redundant and specific promoter occupancy within the ETS gene family. Genes Dev 2007; 21:1882 - 94; http://dx.doi.org/10.1101/gad.1561707; PMID: 17652178
- Shukla AA, Jain M, Chauhan SS. Ets-1/Elk-1 is a critical mediator of dipeptidyl-peptidase III transcription in human glioblastoma cells. FEBS J 2010; 277:1861 - 75; http://dx.doi.org/10.1111/j.1742-4658.2010.07603.x; PMID: 20236318
- Choi YA, Park JS, Park MY, Oh KS, Lee MS, Lim JS, et al. Increase in CIP2A expression is associated with doxorubicin resistance. FEBS Lett 2011; 585:755 - 60; http://dx.doi.org/10.1016/j.febslet.2011.01.018; PMID: 21241697
- Chen KF, Liu CY, Lin YC, Yu HC, Liu TH, Hou DR, et al. CIP2A mediates effects of bortezomib on phospho-Akt and apoptosis in hepatocellular carcinoma cells. Oncogene 2010; 29:6257 - 66; http://dx.doi.org/10.1038/onc.2010.357; PMID: 20729919
- Wilson LA, Yamamoto H, Singh G. Role of the transcription factor Ets-1 in cisplatin resistance. Mol Cancer Ther 2004; 3:823 - 32; PMID: 15252143
- Khanna A, Mahalingam K, Chakrabarti D, Periyasamy G. Ets-1 expression and gemcitabine chemoresistance in pancreatic cancer cells. Cell Mol Biol Lett 2011; 16:101 - 13; http://dx.doi.org/10.2478/s11658-010-0043-z; PMID: 21225469
- Messeguer X, Escudero R, Farré D, Núñez O, Martínez J, Albà MM. PROMO: detection of known transcription regulatory elements using species-tailored searches. Bioinformatics 2002; 18:333 - 4; http://dx.doi.org/10.1093/bioinformatics/18.2.333; PMID: 11847087
- Farré D, Roset R, Huerta M, Adsuara JE, Roselló L, Albà MM, et al. Identification of patterns in biological sequences at the ALGGEN server: PROMO and MALGEN. Nucleic Acids Res 2003; 31:3651 - 3; http://dx.doi.org/10.1093/nar/gkg605; PMID: 12824386
- Kurachi S, Huo JS, Ameri A, Zhang K, Yoshizawa AC, Kurachi K. An age-related homeostasis mechanism is essential for spontaneous amelioration of hemophilia B Leyden. Proc Natl Acad Sci U S A 2009; 106:7921 - 6; http://dx.doi.org/10.1073/pnas.0902191106; PMID: 19416882
- Yang LX, Razzaghi H, Hokanson JE, Kamboh MI. Identification and characterization of a novel 5 bp deletion in a putative insulin response element in the lipoprotein lipase gene. Biochim Biophys Acta 2009; 1791:1057 - 65; http://dx.doi.org/10.1016/j.bbalip.2009.06.003; PMID: 19563912