Abstract
For the past 40 years, bacteriophage lambda has been crucial in revealing fundamental principles underlying control of transcription by elements positioned close to promoters. With the discovery that lambda CI repressors bound to distant sites can interact efficiently, lambda also provides a model for long range gene regulation, including the action of enhancer elements.
In eukaryotes, transcription is often controlled by enhancers, DNA sequences that can be located many kilobases away from the promoter. These long-range effects involve DNA looping and direct contact between proteins bound at the enhancer and the promoter. However, the complexity of eukaryotic transcriptional control has precluded a detailed molecular understanding of enhancer-promoter complexes, so that it is not known how their formation can be efficient and sp.ecific nor precisely how they regulate the initiation process.Citation1
In prokaryotes, most transcription regulators bind near the promoterCitation2,Citation3; repressors usually overlap with and compete with RNAP binding, activators usually bind adjacent to RNAP and contact it to stabilize various initiation intermediates.Citation2,Citation4 Activators often contact the C-terminal domain of one of the α subunits of RNAP (αCTD); the flexible linkers attaching the αCTDs to the rest of RNAP allow these activators, such as catabolite activator protein (CAP), to work from sites located a few tens of basepairs upstream of the promoter sequences.Citation2,Citation5 The αCTD can also bind to DNA sequences termed UP elements,Citation6 which can also stimulate transcription if located just upstream of the promoter.Citation7
Sigma-54-dependent promoters provide an important prokaryotic example of long-range activation. Here, activator proteins assemble at enhancer-like sequences that can be as far as 15 kb away and downstream or upstream of the promoter, and interact with the bound σ54-RNAP by DNA looping, utilizing ATP to remodel the polymerase and stimulate initiation.Citation8
Long-range interactions are also critical in regulation of transcription by the CI repressor of the E. coli bacteriophage λ. A CI tetramer bound to the λ OR ‘switch’ region and a tetramer bound at the distal OL site interact cooperatively to form a CI octamer and a 2.3 kb DNA loop.Citation9,Citation10 This improves CI repression of the lytic PR promoterCitation9,Citation11,Citation12 and is also necessary for effective CI repression of the PRM lysogenic promoter because it allows a CI dimer bound to a third operator at OL to assist CI binding to the very weak OR3 site overlapping PRM.Citation11-Citation17
CI activation of PRM from short range is well understood. The N-terminal domain (NTD) of CI bound to OR2 contacts the σ subunit of RNAP, stimulating the transition from the closed to the open complex.Citation18,Citation19 The αCTD is also involved in CI activation of PRM, contacting the DNA between OR1 and OR2.Citation20 However, the presence of OL can also increase CI activation of PRM both in vivoCitation21,Citation22 and in vitro.Citation15 Recently we showed that DNA looping, mediated by binding of the bacteriophage λ CI repressor to the distant OL site, brings the PRM promoter at OR near to an activator UP element sequence at OL, allowing it to be contacted by the α subunit of RNA polymerase (RNAP)Citation16 (). Molecular and statistical-mechanical modeling showed that by bridging OL and PRM, the CI octamer creates a protein-DNA interface for RNAP to simultaneously contact PRM DNA, CI and the UP element. This interaction was entirely reliant upon the sites being brought together by a DNA bound lambda CI octamer and was the first demonstration that an UP element, normally located within 100bp of the promoter, can operate at long distances. These results established the λ OL site as a multifaceted enhancer, able to stimulate both repression and activation from a distance, and provided an atomic-level model of an enhancer-promoter complex. Work described in a recent paperCitation17 supports these conclusions.
Figure 1. The effect of CAP operator position at OL on activation of PRM. (A) A CI octamer-mediated complex which bridges OR and OL forms a 2.3kb DNA loop, and allows the α subunit of an RNAP (αCTD) bound at PRM to contact the UP element at OL. (B) A structural model of an RNAP-lambda CI-OR-OL complex, assembled as described in Cui et al.,Citation16 and positioned to mimic the view in (A). The model shows that when an octamer of CI (red) connects OR and OL, it is plausible that an RNAP αCTD subunit (green) can interact with the UP element sequence (blue) at OL. The DNA connecting OR and OL is represented by a red line. (C)The α subunit of RNAP is known to interact with DNA-bound CAP.Citation30 This cartoon shows how a CAP operator, artificially replacing the UP element, may be able to recruit an RNAP α subunit to OL, and thereby activate PRM. We predicted that this contact, and hence the degree of PRM activation, would be sensitive to CAP operator sp.acing. (D) A structural model of an RNAP-lambda CI-DNA-CAP-αCTD subunit complex was constructed by replacing the UP element DNA in part (B) with the CAP-DNA-αCTD structure (taken from PDB 1LB2). The CAP-DNA-αCTD complex has been positioned to reproduce the CAP-10 construct (below), where the edge of the CAP operator is positioned 10bp from the edge of the OL3 operator. The DNA connecting OR and OL is represented by a red line. Inherent flexibility of the OL DNA, the CI octamer complex and the αCTD linker peptide (shown as a green dashed line) means that the structure shown here is one of many possible conformations of the complex. (E) The UP element at OL was replaced with a consensus operator for the CAP protein. The arrangement of the PRM lacZ reporters used to assay the effect of CAP operator position relative to OL is shown. A CAP consensus operator was placed at various positions (distances in base pairs are indicated) on the OL3 side of the distal OL site, replacing the λ UP element. OR3− and OL3− mutations were used to eliminate CI repression of PRM. (F) Effect of CAP site sp.acing on CI activation of PRM. The 3.3 WLU λCI expression construct was integrated at φ 186 attBCitation16 and CAP was expressed from the wild type chromosomal gene. CI activation is defined as the fold increase of PRM activity at 3.3 WLU CI over basal (absence of CI). Error bars represent 95% confidence intervals (n ≥ 8). The mean activity of PRM in the absence of CI was 143 ± 5 units (n = 56).
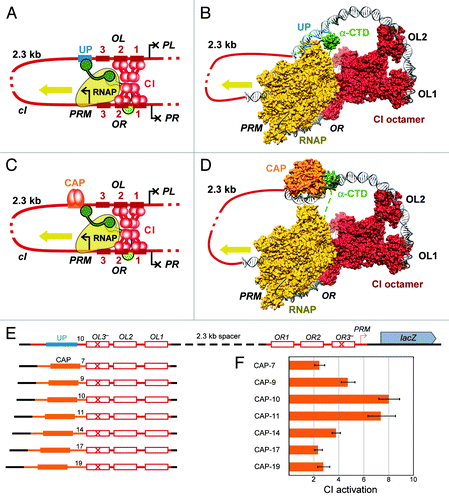
Statistical mechanical analysis of the operation of the lambda switch, based on both in vitro and in vivo dataCitation16,Citation23-Citation25 has permitted a quantitative description of the system in terms of binding free energies. A notable outcome of these analyses is that relatively modest changes in free energy have a significant impact on gene expression. Analysis of DNase I footprinting experiments were originally used to derive the alternate pairwise model of cooperativity between lambda CI dimers binding to the OR region.Citation23,Citation26 This has now become the textbook example of a cooperative protein-protein interaction,Citation27 and involves a free energy change of just -2.5 to -3 kcal/mol. Similarly, free energies equivalent to the formation of just a few additional hydrogen bonds between DNA bound transcription factors (such as CI in the context of PRM) and RNAP are sufficient to recruit RNAP to the promoter and markedly activate transcription.Citation4,Citation19
Extension of this thermodynamic model to include CI-mediated looping between OR and OL, based on in vivo PR and PRM transcriptional reporter data has shown the importance of two key looping parameters. ΔGoct, the free energy of forming a DNA bound CI octamer from two DNA bound CI tetramers (-0.5 kcal/mol) and ΔGtet, the free energy required to form a CI tetramer between OR3 and OL3 (-2.4 kcal/mol) are similarly small in magnitude.Citation25 Remarkably, a recent studyCitation28 using high resolution, single molecule imaging methods to directly visualize CI mediated DNA looping inside live E. coli cells derived very similar estimates for these parameters (+ 0.3 and -3.2 kcal/mol, for ΔGoct and ΔGtet, respectively). In vitro data on CI mediated DNA looping, obtained using a tethered particle motion approach, indicated a somewhat weaker value for ΔGoct, no doubt reflecting the nature of the naked, non-supercoiled DNA in vitro.Citation14
Our most recent extension of the thermodynamic modeling to include RNAP and its contact with the distal UP element suggested that the RNAP αCTD to UP element contact, across the 2.3kb OR-OL DNA loop, improved RNAP binding by approximately -2.7 kcal/mol.Citation16
These small net energy changes are a balance between favorable protein-protein and protein-DNA interactions, unfavorable DNA bending and distortion energies, and the entropic cost of tethering two distant DNA sites close to each other. While the entropic effect increases with the distance between the sites, the energy penalty for deforming the intervening DNA is reduced. In addition, structural modeling of the RNAP-CI-DNA complex suggests that the OL3-UP element region itself must undergo substantial deformation to successfully contact the RNAP αCTD ().Citation16 In the phage context, the cost of this short range DNA deformation may be paid in part by binding of integration host factor (IHF), a DNA bending protein that has a binding site immediately adjacent to the UP element sequence.Citation29 Indeed, recent work by Michalowski and LittleCitation17 shows that mutation of the IHF site at OL reduced UP element-mediated stimulation of PRM.
Consistent with these thermodynamic arguments, we showed that the UP element-mediated improvement in PRM activation was lost when the OR-OL loop size was increased to 10kb and the entropic penalty is high. However, replacement of the UP element at OL with an operator for catabolite activator protein (CAP) showed significant activation of PRM, even at a distance of 10kb.Citation16 CAP binds and bends DNA strongly, and contacts the RNAP αCTD, which in turn makes minor groove contacts with the DNA adjacent to CAP.Citation30 Thus, compared with the simple αCTD to UP element contact, the additional contacts between CAP and the αCTD are able to overcome the increased entropy cost at the longer distance.
Unlike the relatively non-sp.ecific minor groove contacts between the αCTD and the AT-rich UP sequence, we predicted that steric constraints on the contact between the RNAP-αCTD and the CAP DNA complex should impose DNA helix face dependence of the CAP-mediated activation of PRM (). To test the effect of the CAP operator position on PRM activation, we used chromosomal PRM.lacZ fusions, placing a consensus CAP operator at various positions relative to OL3, where OL was 2.3 kb upstream of PRM (). When placed 10 or 11 bp from the edge of OL3, a CAP operator was able to substantially increase activation of PRM. However, when the CAP site was positioned closer (7 or 9bp) or further away (17 or 19bp) from OL3, the activation effect was severely reduced. Docking of a DNA bound CAP dimer structure onto our model of the RNAP-CI-OR-OL complex (), indicates that an RNAP αCTD to CAP contact is plausible when the CAP operator is located at a position corresponding to the CAP-10 construct. It also seems reasonable, on the basis of this model, that moving the CAP operator by half a helical turn in either direction may make the αCTD -DNA contact less favorable, as a result of either steric clashes with the body of the RNAP, or by moving CAP beyond the reach of the αCTD.
The distal OL operator of lambda thus has the defining properties of a complex enhancer, able to activate or repress the distant PRM promoter (). Activation by recruitment, that is, formation of the active transcription complex by simple binding interactions of relatively small net free energies, appears sufficient to explain the mechanism of activation in lambda.Citation16,Citation17 In the more general case, proteins recruited to enhancer-promoter complexes may also possess enzymatic activity capable of modifying other components (DNA or protein) of the complex, providing additional positive or negative feedback to reinforce the regulatory outcome.
Figure 2. Lambda OL as a multi-faceted enhancer. The types of long-range protein-protein and protein-DNA interactions that characterize an RNA polymerase-enhancer complex are depicted (A), and compared with the corresponding interactions that occur during transcription from the lambda PRM promoter (B).
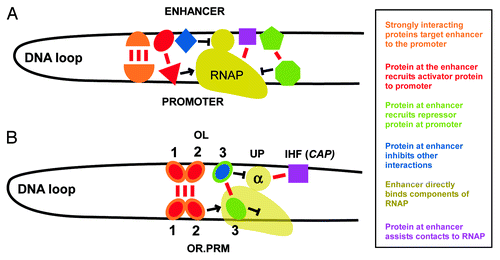
Abbreviations: | ||
RNAP | = | RNA polymerase |
αCTD | = | C-terminal domain of the alpha subunit of RNAP |
CAP | = | catabolite activator protein |
WLU | = | wild type lysogenic units |
IHF | = | integration host factor |
Acknowledgments
This work was funded by Human Frontiers Scientific Program Grant RGP0051 and Australian Research Grants DP110100824 and DP11010470. Lun Cui was supported by the China Scholarship Council.
Disclosure of Potential Conflicts of Interest
No potential conflicts of interest were disclosed.
References
- Bulger M, Groudine M. Functional and mechanistic diversity of distal transcription enhancers. Cell 2011; 144:327 - 39; http://dx.doi.org/10.1016/j.cell.2011.01.024; PMID: 21295696
- Browning DF, Busby SJ. The regulation of bacterial transcription initiation. Nat Rev Microbiol 2004; 2:57 - 65; http://dx.doi.org/10.1038/nrmicro787; PMID: 15035009
- Gama-Castro S, Salgado H, Peralta-Gil M, Santos-Zavaleta A, Muñiz-Rascado L, Solano-Lira H, Jimenez-Jacinto V, Weiss V, García-Sotelo JS, López-Fuentes A, et al. RegulonDB version 7.0: transcriptional regulation of Escherichia coli K-12 integrated within genetic sensory response units (Gensor Units). Nucleic Acids Res 2011; 39:Database issue D98 - 105; http://dx.doi.org/10.1093/nar/gkq1110; PMID: 21051347
- Ptashne M, Gann A. Genes & Signals. Cold Spring Harbor, New York: Cold Spring Harbor Laboratory Press, 2002.
- Ushida C, Aiba H. Helical phase dependent action of CRP: effect of the distance between the CRP site and the -35 region on promoter activity. Nucleic Acids Res 1990; 18:6325 - 30; http://dx.doi.org/10.1093/nar/18.21.6325; PMID: 2173826
- Estrem ST, Ross W, Gaal T, Chen ZW, Niu W, Ebright RH, Gourse RL. Bacterial promoter architecture: subsite structure of UP elements and interactions with the carboxy-terminal domain of the RNA polymerase alpha subunit. Genes Dev 1999; 13:2134 - 47; http://dx.doi.org/10.1101/gad.13.16.2134; PMID: 10465790
- Meng W, Belyaeva T, Savery NJ, Busby SJ, Ross WE, Gaal T, Gourse RL, Thomas MS. UP element-dependent transcription at the Escherichia coli rrnB P1 promoter: positional requirements and role of the RNA polymerase alpha subunit linker. Nucleic Acids Res 2001; 29:4166 - 78; http://dx.doi.org/10.1093/nar/29.20.4166; PMID: 11600705
- Rappas M, Bose D, Zhang X. Bacterial enhancer-binding proteins: unlocking sigma54-dependent gene transcription. Curr Opin Struct Biol 2007; 17:110 - 6; http://dx.doi.org/10.1016/j.sbi.2006.11.002; PMID: 17157497
- Révet B, von Wilcken-Bergmann B, Bessert H, Barker A, Müller-Hill B. Four dimers of lambda repressor bound to two suitably spaced pairs of lambda operators form octamers and DNA loops over large distances. Curr Biol 1999; 9:151 - 4; http://dx.doi.org/10.1016/S0960-9822(99)80069-4; PMID: 10021390
- Bell CE, Lewis M. Crystal structure of the lambda repressor C-terminal domain octamer. J Mol Biol 2001; 314:1127 - 36; http://dx.doi.org/10.1006/jmbi.2000.5196; PMID: 11743728
- Dodd IB, Shearwin KE, Perkins AJ, Burr T, Hochschild A, Egan JB. Cooperativity in long-range gene regulation by the lambda CI repressor. Genes Dev 2004; 18:344 - 54; http://dx.doi.org/10.1101/gad.1167904; PMID: 14871931
- Svenningsen SL, Costantino N, Court DL, Adhya S. On the role of Cro in lambda prophage induction. Proc Natl Acad Sci U S A 2005; 102:4465 - 9; http://dx.doi.org/10.1073/pnas.0409839102; PMID: 15728734
- Dodd IB, Perkins AJ, Tsemitsidis D, Egan JB. Octamerization of lambda CI repressor is needed for effective repression of P(RM) and efficient switching from lysogeny. Genes Dev 2001; 15:3013 - 22; http://dx.doi.org/10.1101/gad.937301; PMID: 11711436
- Zurla C, Manzo C, Dunlap D, Lewis DE, Adhya S, Finzi L. Direct demonstration and quantification of long-range DNA looping by the lambda bacteriophage repressor. Nucleic Acids Res 2009; 37:2789 - 95; http://dx.doi.org/10.1093/nar/gkp134; PMID: 19276206
- Lewis D, Le P, Zurla C, Finzi L, Adhya S. Multilevel autoregulation of λ repressor protein CI by DNA looping in vitro. Proc Natl Acad Sci U S A 2011; 108:14807 - 12; http://dx.doi.org/10.1073/pnas.1111221108; PMID: 21873207
- Cui L, Murchland I, Shearwin KE, Dodd IB. Enhancer-like long-range transcriptional activation by λ CI-mediated DNA looping. Proc Natl Acad Sci U S A 2013; 110:2922 - 7; http://dx.doi.org/10.1073/pnas.1221322110; PMID: 23382214
- Michalowski CB, Little JW. Role of cis-Acting Sites in Stimulation of the Phage λ PRM Promoter by CI-Mediated Looping. J Bacteriol 2013; 195:3401 - 11; http://dx.doi.org/10.1128/JB.02148-12; PMID: 23708136
- Li M, McClure WR, Susskind MM. Changing the mechanism of transcriptional activation by phage lambda repressor. Proc Natl Acad Sci U S A 1997; 94:3691 - 6; http://dx.doi.org/10.1073/pnas.94.8.3691; PMID: 9108039
- Jain D, Nickels BE, Sun L, Hochschild A, Darst SA. Structure of a ternary transcription activation complex. Mol Cell 2004; 13:45 - 53; http://dx.doi.org/10.1016/S1097-2765(03)00483-0; PMID: 14731393
- Kedzierska B, Szambowska A, Herman-Antosiewicz A, Lee DJ, Busby SJ, Wegrzyn G, Thomas MS. The C-terminal domain of the Escherichia coli RNA polymerase alpha subunit plays a role in the CI-dependent activation of the bacteriophage lambda pM promoter. Nucleic Acids Res 2007; 35:2311 - 20; http://dx.doi.org/10.1093/nar/gkm123; PMID: 17389649
- Anderson LM, Yang H. DNA looping can enhance lysogenic CI transcription in phage lambda. Proc Natl Acad Sci U S A 2008; 105:5827 - 32; http://dx.doi.org/10.1073/pnas.0705570105; PMID: 18391225
- Little JW, Michalowski CB. Stability and instability in the lysogenic state of phage lambda. J Bacteriol 2010; 192:6064 - 76; http://dx.doi.org/10.1128/JB.00726-10; PMID: 20870769
- Ackers GK, Johnson AD, Shea MA. Quantitative model for gene regulation by lambda phage repressor. Proc Natl Acad Sci U S A 1982; 79:1129 - 33; http://dx.doi.org/10.1073/pnas.79.4.1129; PMID: 6461856
- Shea MA, Ackers GK. The OR control system of bacteriophage lambda. A physical-chemical model for gene regulation. J Mol Biol 1985; 181:211 - 30; http://dx.doi.org/10.1016/0022-2836(85)90086-5; PMID: 3157005
- Dodd IB, Shearwin KE, Perkins AJ, Burr T, Hochschild A, Egan JB. Cooperativity in long-range gene regulation by the lambda CI repressor. Genes Dev 2004; 18:344 - 54; http://dx.doi.org/10.1101/gad.1167904; PMID: 14871931
- Johnson AD, Meyer BJ, Ptashne M. Interactions between DNA-bound repressors govern regulation by the lambda phage repressor. Proc Natl Acad Sci U S A 1979; 76:5061 - 5; http://dx.doi.org/10.1073/pnas.76.10.5061; PMID: 159452
- Ptashne M. A Genetic Switch. Phage Lambda revisted. New York: Cold Spring Harbor Laboratory Press, 2004.
- Hensel Z, Weng X, Lagda AC, Xiao J. Transcription-Factor-Mediated DNA Looping Probed by High-Resolution, Single-Molecule Imaging in Live E. coli Cells. PLoS Biol 2013; 11:e1001591; http://dx.doi.org/10.1371/journal.pbio.1001591; PMID: 23853547
- Giladi H, Gottesman M, Oppenheim AB. Integration host factor stimulates the phage lambda pL promoter. J Mol Biol 1990; 213:109 - 21; http://dx.doi.org/10.1016/S0022-2836(05)80124-X; PMID: 2140136
- Lawson CL, Swigon D, Murakami KS, Darst SA, Berman HM, Ebright RH. Catabolite activator protein: DNA binding and transcription activation. Curr Opin Struct Biol 2004; 14:10 - 20; http://dx.doi.org/10.1016/j.sbi.2004.01.012; PMID: 15102444