Abstract
Understanding the structure and function of ecosystems in Canada's national parks is essential in fulfilling the Parks Canada Agency's mandate to manage for ecological integrity. Wapusk National Park is representative of the Hudson Bay Lowlands and small lakes and ponds make up a considerable component of the landscape. The Hudson Bay Lowlands have experienced relatively recent isostatic rebound from submarine conditions, hence proximity to the coast was found to be a major factor in determining the concentration of dissolved salts in pond water. It was observed that the ionic composition of the water in ponds throughout much of the park is consistent, indicating that most of the ponds are an expression of surface water maintaining little connection to groundwater. The prevalence of permafrost throughout much of the park is likely the reason for this stability. Ponds within the park also show considerable variability in the amount of dissolved organic carbon (DOC) they contain. DOC shows a strong north-south and east-west trend. Ponds in the northeast of the park have lower DOC values while ponds in the southwest of the park have higher levels of DOC. Changes in DOC within the park appear to be driven by changes in the terrestrial vegetation surrounding the ponds.
Climatically mediated changes in northern tree line and permafrost are likely to cause the greatest alteration of aquatic habitats in Wapusk National Park. The paucity of background data makes it impossible to assess the amount of change that may have already occurred in the park. This research provides the first landscape-level study for the area and shows that there are distinct limnological patterns over this landscape that are likely to be sensitive to climate change and should be readily detectable with ongoing monitoring.
La comprhension de la structure et de la fonction des cosystmes en parcs nationaux du Canada est essentielle en accomplissant le mandat de l'agence du Canada de parcs pour contrler pour l'intgrit cologique. Le parc national de Wapusk est reprsentant des terres en contre-bas de compartiment de Hudson et les petits lacs et les tangs composent un composant considrable du paysage. Les terres en contre-bas de compartiment de Hudson ont prouv le rebond isostatique relativement rcent des conditions submersibles, par consquent la proximit la cte s'est avre un facteur important en dterminant la concentration des sels dissous dans l'eau dtang. On l'a observ que la composition ionique de l'eau dans les tangs dans tous une grande partie du parc est conforme, indiquant que la plupart des tangs sont une expression de l'eau de surface maintenant peu de raccordement aux eaux souterraines. La prdominance du perglisol dans tous une grande partie du parc est probable la raison de cette stabilit. Les tangs dans le parc montrent galement que variabilit considrable dans la quantit de carbone organique dissous (COD) qu'ils contiennent. Le COD montre une tendance au nord-sud et est-ouest forte. Les tangs dans le nord-est du parc ont des valeurs plus basses de COD tandis que des tangs dans le sud-ouest du parc font sembler des niveaux plus levs des changements de COD de COD dans le parc tre conduits par des changements de la vgtation terrestre entourant les tangs.
Les changements climatiquement ngocis de la ligne d'arbre et du perglisol nordiques sont susceptibles de causer le plus grand changement d'habitats aquatiques dans le parc national de Wapusk. Le manque des donnes de fond le rend impossible dvaluer la quantit de changement qui a pu stre dj produite en parc. Cette recherche fournit la premire tude de paysage-niveau pour le secteur et prouve qu'il y a des modles limnological distincts au-dessus de ce paysage qui sont susceptibles dtre sensibles au changement climatique et devraient tre aisment discernables avec la surveillance continue.
Introduction
The Parks Canada Agency's mandate to maintain and restore ecological integrity in Canada's national parks has led to the development of the Parks Canada ecological integrity monitoring program. In order to effectively monitor environmental change in a selected ecosystem, baseline information regarding environmental conditions for defined periods of time are necessary. This project was developed to inform managers and scientists about the water chemistry of a representative sample of ponds in Wapusk National Park.
Wapusk National Park of Canada is located in the Hudson Bay lowlands, an area bordering the southwestern corner of Hudson Bay in northern Manitoba (), which has the most southerly extent of permafrost and the coldest southward temperature excursions in northern Canada due to the refrigerating effect of Hudson Bay and predominant circulation patterns. These characteristics have the potential to make the area very susceptible to climatic warming, especially if accompanied by sea ice loss on Hudson Bay. Changes in ice cover on the bay and wetland permafrost are likely to not only respond to warming, but also strongly affect the rate and degree of warming (Gagnon and Gough, Citation2005).
Figure 1. Map of Wapusk National Park Showing Location of Study Sites and the nearby town of Churchill.
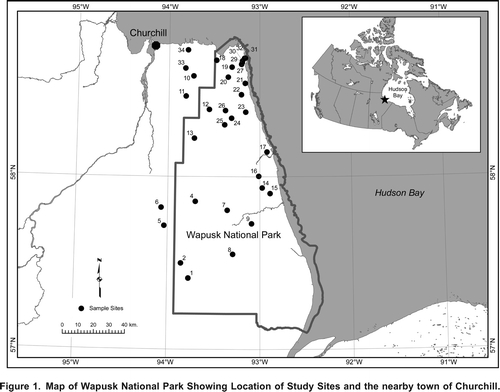
Wapusk National Park exhibits a strong ecotone with forested environments transitioning to tundra from south to north and west to east (Riley, Citation1982). Lakes and ponds in areas bordering northern tree line have shown significant change in the characteristics of their lake-water and species composition as a result of shifting tree line in their catchment due to climate change (Ruhland et al., Citation2003). Research from the Canadian High Arctic (e.g., Douglas et al., Citation1994) and tree line (Ruhland et al., Citation2003) ecosystems confirms that some northern ponds and lakes have undergone significant change related to rising temperatures, while other northern lakes have not been strongly affected by warming (Laing et al., Citation2002). The dramatic changes, landscapes, and unique ecotones within Wapusk National Park highlight its potential value for aquatic monitoring due to the sensitivity of the area.
Increases in frequency and intensity of fire are expected to occur in the Hudson Bay Lowlands as a consequence of climate change (Brook, Citation2006). As far as we are aware, no data exist for the impacts of fire on the freshwater resources of the park. In a similar environment, McEachern et al. (Citation2000) have shown that forest fires in catchments with permafrost-peatland dominated soils can lead to persistent (i.e., >38 years) phosphorus enrichment. However, Vitt and Bayley (Citation1984) did not detect long-term (8 years) impacts of fire on water chemistry in a Sphagnum fallax black spruce (Picea mariana) mire. A review by MacLean et al. (Citation1983) concluded that fire rarely burns the entire organic layer in circumpolar areas and that changes in soil water chemistry do not result in nutrient flux from peat-derived soils.
Wapusk National Park is a remote and logistically challenging landscape where very few limnological studies have been undertaken. Freshwater aquatic habitat forms a major component of Wapusk National Park (approximately 40% of the surface area) (Bello and Smith, Citation1990), yet even the general limnological conditions and landscape patterns are not well known. The objectives of this paper are to provide baseline information on aquatic conditions in the park, analyse landscape-level patterns seen in the limnic environment, and describe the factors likely driving these patterns. The data included in this paper provide a snapshot of current aquatic conditions in the park that may be used for future reference. Environmental stressors likely to change limnological conditions and potential trajectories are discussed.
Study Area
The Hudson Bay lowlands are the second largest continuous peatland in the world and span the boundary between the high subarctic and low arctic ecoclimatic regions. The region is characterized by very little topographic relief (less than 1 mkm1) and continues to experience isostatic rebound. The emergent sea bottom has substrates mainly of marine silts/clays (Martini, Citation1989), marine washed tills and subglacial deposits (Lafleur and Rouse, Citation1995). The largely impermeable nature of these deposits combined with the slight topographic relief of the area creates ideal conditions for the development of extensive wetlands (Riley, Citation1982). Bello and Smith (Citation1990) estimated that lakes and ponds comprise over 40% of the surface area. Peat topsoil dominates the low-lying areas and ranges from 100 to 500 mm thick (Duguay and Lafleur, Citation2003).
The mean annual temperature at Churchill is 7.2 C, the mean July temperature is 12.5 C and the mean January temperature is 27.5 C (Mortsch, Citation1994). Annual precipitation is low (411 mm), of which 235 mm falls as rain during MaySeptember and the remaining 43% falls as snow between October and May. Most of the study area is underlain by permafrost with active layer depths ranging from 75 to 180 cm (Bello and Smith, Citation1990). The extent of permafrost underneath lakes and ponds is not well known, for example, Bello and Smith (Citation1990) recorded a 1.5 m deep pond freezing to the bottom in 1986, but not in 1984.
Ponds are found in a variety of ecosystems throughout the region. Three major ecological zones, which run roughly parallel to the coast, can be identified as: tundra, forest-tundra and open forest. Landsat based vegetation mapping for the park identified 15 unique vegetation classes with dryas heath upland, lichen spruce bog, sedge bullrush poor fen and regenerating burns constituting the most common classes (Brook and Kenkel, Citation2002).
Methods
Ponds were sampled between July 21 and July 28, 2004. Most ponds were accessed by helicopter. Sampling was conducted using an inflatable belly boat with chest waders and diving fins. Ponds were sampled from their deepest location. For the smaller ponds, this was generally near the centre, while ponds larger than 50 ha were sampled approximately 100 m from shore near the deepest spot that had been observed from the helicopter. Secchi depths were measured using a standard 22 cm secchi disk, it was lowered to its extinction point and the average of appearance and disappearance was recorded. Pond depth was recorded after further lowering the secchi disk to the bottom.
Water samples were taken from 0.5m depth and treated in the field according to sampling procedures outlined in an Analytical Methods Manual (Environment Canada, Citation1979). Samples for trace metals analysis, total dissolved phosphorus (TDP), total nitrogen (TN), dissolved inorganic carbon (DIC) and dissolved organic carbon (DOC) were filtered through 0.45 m cellulose acetate membrane filters. 100 ml samples for trace metals were preserved with 1 ml concentrated HNO3. TDP and unfiltered total phosphorus (TP) samples were stored in 125ml glass bottles, pre-rinsed with filtered water and preserved with one millilitre of 30% H2SO4. Major ions (Na, K, Cl, SO4, Fe), reactive dissolved silica, alkalinity and bicarbonate were determined from samples stored in 500ml HDPE bottles. Chlorophyll samples treated with 1 mL of saturated MgCO3 solution were filtered through 0.45 m membrane filters, folded once, wrapped in a labelled paper filter, placed in a petri dish, wrapped in aluminum foil and frozen until analysis. Water samples were kept cold and in the dark until analysis by PSC analytical labs (Burnaby, BC, Canada). Samples were shipped for analysis the day following sampling and were analysed within 48 to 96 hours after sampling. Conductivity, Dissolved Oxygen, pH and temperature profiles were measured at 0.5 m intervals with a YSI 556 handheld meter. Geographic coordinates of the ponds were recorded using a Garmin etrex Vista handheld GPS unit (datum is WGS84).
Environmental variables used to examine inter-pond variability in water chemistry included geographic location, pond depth, the ratio of catchment to pond surface area and the composition of surrounding terrestrial vegetation. Terrestrial Vegetation composition surrounding the ponds was determined from Brook's (Citation2005) Landsat based vegetation classification. For each pond, a polygon was overlain which surrounded the pond and included the terrestrial area adjacent to the pond. The size and shape of this polygon was determined by the shape of the pond and the proximity of adjacent ponds. Polygons were shaped to include an area with a radius three times that of the pond, with the restriction that this boundary could not extend further than half the distance to an adjacent pond, which was the limiting factor for most ponds in this densely aquatic landscape. The range of the ratio of catchment surface area to pond surface area was between 1.9 and 14 for the dataset. Pond catchments included the vegetation types, Sedge Rich Fen, Willow Birch Shrub, Sedge Larch Fen, Sphagnum Spruce Bog, Graminoid Willow Salt Marsh, Lichen Spruce Bog, Sedge Bulrush Poor Fen, Lichen Meltpond Bog, Lichen Peat Plateau, Dryas Heath, Regenerating Burn, Recent Burn, Unvegetated Ridge and Unvegetated Shore (as defined in Brook and Kenkel, Citation2002).
Data were screened for normality (Jongman et al., Citation1995) using histograms and the Shapiro-Wilk W test as implemented in NCSS 2001 software (Number Cruncher Statistical Systems, Kaysville Utah, USA). Variables that showed a skewed distribution were square-root, log or log(n +1) transformed. For use in ordination, geographic coordinates were expanded using a cubic polynomial equation in order to account for the possibility of nonlinear patterns over space (Legendre, Citation1990). Principal Components Analysis (PCA) was used to investigate the dominant patterns in water chemistry. Redundancy Analysis (RDA), a technique in which the ordination axes are constrained to be a linear combination of environmental descriptors (geographic location, catchment vegetation, etc.), was used to determine the amount of variance in the water chemistry that could be described by the environmental descriptors. We used forward stepwise selection of environmental variables to determine the significance of each variable to the RDA. Forward selection determines the successive contribution of each environmental descriptor to explaining the total variation in water chemistry data and adds only those variables that contribute significantly. Ordination analyses were conducted using the computer program Canoco v. 4.0 (Centre for Biometry, CPRO-DLO, Wageningen, The Netherlands).
Results
The chemical, physical and catchment vegetation characteristics of the 32 ponds are shown in and . Secchi disk measurements were visible to the bottom of all but two ponds and were thus excluded from analyses. The dissolved metals: aluminium, antimony, arsenic, beryllium, bismuth, cadmium, chromium, cobalt, copper, lead, molybdenum, nickel, selenium, silver, tellurium, thallium, tin, titanium, vanadium, zinc and zirconium were below detection limits in all ponds. Measures of inorganic carbon including DIC, hardness, alkalinity and bicarbonate were all highly correlated (r > 0.93) and thus only DIC was used in analyses. Similarly, TDS and specific conductance were highly correlated (r = 0.99) and therefore only specific conductance was used in the analyses. TDP was not used in analyses because it was below detection limits in 22 of the ponds and well related to TP in the remaining ponds (r2 = 0.88, n = 10).
Table 1. Depth, temperature and water chemistry data for the 32 ponds.
Table 2. Terrestrial Vegetation composition surrounding ponds, after Brook's (2002) Landsat based vegetation map.
None of the ponds were strongly thermally stratified. Although some ponds had warmer surface waters (top 0.5 m) relative to bottom waters (maximum Ttopbottom = 2.8 C), neither specific conductance, DO or pH showed any change with depth. Thus, only surface measurements were used in analyses. Regardless of surface area, all of the ponds were shallow (max depth < 2 m).
Specific conductance of our samples ranged from 45 to 2451 Scm1. The ionic composition of most ponds was similar to that of seawater; although the concentration was often much lower (). Molar ratios of the dominant ions vs. chloride, the most conservative ion from seawater, show a similar pattern across the ponds. Despite changes in concentration, pond-water ionic composition retains the ratio found in seawater, with the exception of Ca and Alkalinity which show considerable enrichment in the ponds compared to seawater ().
Figure 2. The molar ratio of Na+1 and Cl1 found in the study ponds showing all ponds (A) and only the more dilute ponds (B). The line depicts the ratio that would be expected for seawater.
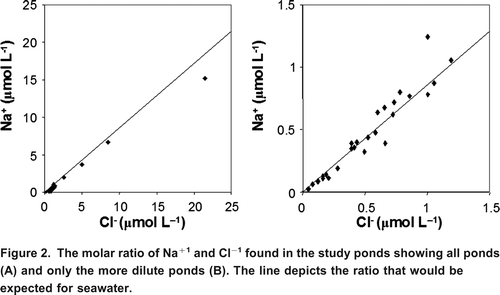
Table 3. Ratio of dominant ions to Cl.
Results from the PCA analysis show that the greatest variation in the ponds water chemistry is related to specific conductance and the dominant ions (). Axis one describes 53% of the variation in the water chemistry data and has the eigenvectors for Na, Cl, specific conductance and other common ions aligned with the axis. The second greatest direction of variance (Axis 2) only describes 14% of the variance seen in the data and is most strongly related to DOC and Fe. The unconstrained (PCA) and constrained (RDA) ordination techniques both showed similar patterns in the data ( and ). Forward selection of environmental variables in the RDA revealed that latitude, longitude, depth and the catchment vegetation variable Graminoid Willow Salt Marsh (GWSM) were the only four variables that contributed significantly to describing the variation in the water chemistry data. These descriptors captured most of the variation observed in the PCA. RDA Axis 1 described 45% of the variation in the water chemistry data (compared to 53% in the PCA) and Axis 2 described 9% of the variation (compared to 14% in the PCA).
Figure 3. Principal Components Analysis (PCA) showing the dominant variation in aquatic parameters amongst the ponds. The single greatest difference observed between the ponds is related to changes in salinity and the concentration of the dominant ions and is shown by the length and alignment of these vectors along Axis 1. The second greatest direction of variation in the PCA is related to changes in DOC (Axis 2).
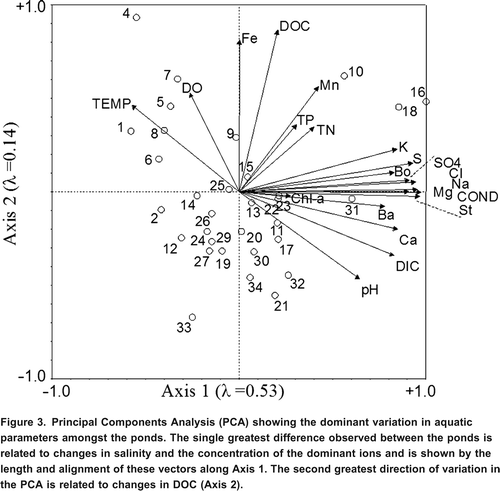
Figure 4. Redundancy Analysis (RDA) shows a similar pattern to PCA, indicating that the environmental descriptors latitude, longitude, depth and graminoid salt marsh (shown with dashed vector lines) describe the variation in the water chemistry data well. The relationship between the environmental descriptors and water chemistry data can be interpreted by the alignment of the vectors. The diagram shows that the major variation in the pond ionic concentration is found to increase eastward and northward in the park, while DOC (the second greatest source of variation among the ponds) is found to decrease with increasing latitude and also slightly decrease from west to east in the park. The only significant vegetation descriptor, Graminoid Willow Salt Marsh (GWSM), captures variation independent of latitude and longitude and is associated with higher DIC and specific conductance.
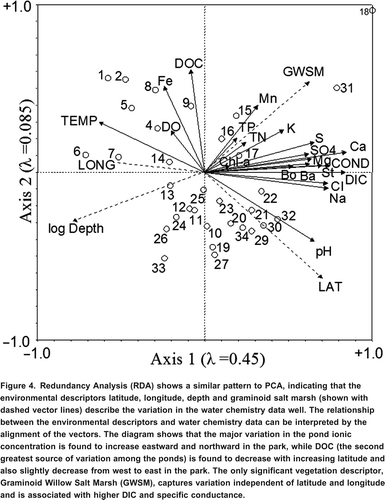
In general, the parameters that varied the greatest between the ponds were temperature, DOC, pH and a group of compounds related to ionic concentration. DOC and surface temperature had elevated levels in more southerly ponds. Conductivity was highest in the ponds closest to the coast (increasing latitude and decreasing longitude) (). Depth, latitude, longitude and GWSM vegetation were the only environmental descriptors that showed a significant relationship with water chemistry.
Discussion
In general, the ponds studied were dilute, oligotrophic, with circumneutral to slightly alkaline pH measurements. Riley (Citation1982) documented a trend of increasing pH with latitude for wetlands in the Hudson Bay lowlands south of our study area. Consistent with this, the mean pH of our more northerly sites was even higher than that measured by Riley (Citation1982). DOC was relatively high in these ponds (range 4.816 mg/L) although not as high as has been observed in other peat-dominated areas from this latitude (mean = 16 mgL1 McEachern et al., Citation2000). The higher DOC values observed by McEachern et al. (Citation2000) may be due to the higher drainage area to lake area ratio of their lakes and a higher proportion of forested catchment. Similar to Eilers et al. (Citation1992) we found that none of the ponds in our study area were acidic, despite relatively high concentrations of DOC, much of which is likely from acidophilic bryophytes such as Sphagnum. Organic acid production in the catchments is apparently insufficient to overcome alkalinity production from watershed weathering and in situ alkalinity generation within the ponds.
Although weak thermal stratification was observed in those ponds sampled on warm still days, energy from strong coastal winds seemed sufficient to overcome stratification, which was not observed in ponds sampled on windy days. Given the similarity of ponds sampled on different days, it is likely that all of the ponds are polymictic throughout the ice-free period. On windy days considerable amounts of suspended sediment were observed in the water column of the ponds and thus the entire water column is likely in almost continuous contact with the sediment during the ice-free period. TN:TP ratios were generally high (range by mass = 11128, mean = 62, range by molar ratio = 25283, mean =133) in these ponds and indicates they are likely phosphorus rather than nitrogen limited (Downing and McCauley, Citation1992). The high TN:TP ratio's observed from our shallow ponds are consistent with research from Alexander et al. (Citation1989) which demonstrated that water in shallow ponds from Alaska is essentially always in contact with sediment, which serves as a nitrogen source and thus the ponds tend to be phosphorus limited.
The specific conductance of our samples spanned a much larger gradient than previously observed in the Saqvaqjuac research lakes located near Hudson Bay to the Northwest of our sites. Specific conductance of our samples ranged from 45 to 2451 Scm1, whereas the lakes observed in Saqvaqjuac spanned 40100 Scm1. These differences are likely due to some of our sites being more proximate to Hudson Bay. The ionic composition of most ponds was similar to that of seawater, although the salinity was often much more dilute (). Many of the ponds have only recently been elevated from the sea and some likely still receive significant amounts of sea spray, the molar ratio of Na:Cl in the ponds (0.62 x 1.29, mean = 0.92) spanned that of seawater (0.86). In general, both Na+ and Cl, had elevated levels in ponds more proximate to Hudson Bay (). The stability of ionic composition amongst all the ponds regardless of concentration is consistent with a dominance of surface rather than groundwater influences on most ponds.
Pond water chemistry was not strongly related to catchment vegetation as identified by Landsat classification (Brook and Kenkel, Citation2002). The only vegetation characteristic that showed a significant relationship to the water chemistry data was Graminoid Willow Salt Marsh. GWSM habitat is only found immediately adjacent to the coast, and only Pond 18 had GWSM as a significant component (17%) of the catchments vegetation. Ponds 16 and 18 had much higher salinity than the rest of the ponds and ordinate together in the PCA. However, Pond 18 differs from Pond 16 in that it also has much higher alkalinity. Thus, for this data set, Pond 18 has the unusual combination of high alkalinity and high salinity, and it is likely this combination of features that GWSM is able to uniquely describe. Our dataset does not have sufficient redundancy to say whether this is a general characteristic of ponds with significant amounts of GWSM in the catchment.
In other northern areas, a strong relationship has been observed between DOC and the extent of forest development in the catchment (e.g., Pienitz et al., 1997; Ruhland et al., Citation2003). Surprisingly, Landsat-based measures of arboreal vegetation such as Willow Birch Shrub Fen, Sedge Larch Fen, Sphagnum Spruce Bog and Lichen Spruce Bog were not significantly related to pond water chemistry. However, latitude and longitude were significantly related to the variation in the water chemistry data. Wapusk National Park does have increasing forest development as you move south and west in the park. Correspondingly, the RDA analysis shows increasing DOC with decreasing latitude and increasing longitude. It is unclear why latitude and longitude would be better metrics for tree cover than the Landsat derived vegetation measures.
The shallow depth (Zmax < 2 m) of all the sampled waterbodies makes it likely that they all freeze completely in winter and are thus ponds. Anecdotal evidence from sediment coring that was conducted at the same time as water sampling supports this hypothesis. In shallow ponds where contact with the corer could be maintained it was obvious that core length was limited due to striking a permafrost layer. In deeper ponds it was difficult to ascertain with confidence if permafrost was present, but the short length of the retrieved cores indicates that permafrost may exist in ponds as deep as 2.5 m. Bello and Smith (Citation1990) reported the potential for permafrost (pond completely frozen to bottom) for a lake 60 km south of our study sites.
Changes in climate and ultraviolet radiation levels in the Arctic may have far-reaching impacts on northern aquatic ecosystems in ways that are difficult to project (Wrona et al., Citation2006). Climatically mitigated changes in evapotranspiration, rainfall, permafrost, fire frequency and tree line all have the potential to alter aquatic conditions within Wapusk National Park. Without site-specific climatic models it is difficult to ascertain what the rate, magnitude or combined effect of these changes may be. Our data show that the dominant limnological patterns in the park are a strong gradient in ionic concentration driven by proximity to the coast and isostatic rebound; conservation of ionic ratios likely due to permafrost isolating the ponds from groundwater; and a latitudinal and longitudinal gradient in DOC related to forest cover. The dominant limnological patterns we have described point to areas where changes in the park are likely to be detected by future monitoring.
As demonstrated by Welch and Legault (Citation1986), the delivery of solutes to waterbodies in the permafrost setting bordering Hudson Bay is strongly affected by the timing and magnitude of runoff. Climate change is likely to cause increased solute delivery to these waterbodies. In particular, increases in summer rainfall once the active layer has become well-developed results in high solute output from the watershed (Welch and Legault, Citation1986). Currently much of the melt occurs before the ponds are ice free so much of the solute is lost in runoff. However, ponds may retain most of the nutrients delivered to them if they become ice free at the time of melt. Permafrost isolates ponds from interactions with groundwater so future climate induced disruption to permafrost may alter the stable ionic composition that ponds currently exhibit.
The northeast to southwest trend in DOC levels is the second greatest variation seen in the water chemistry in this study. Any environmental change that alters northern tree line may alter the distribution of DOC and associated diversity of aquatic environments found in the park.
Changes in water budgets may also strongly affect the ponds of the Hudson Bay lowlands. Predictions of climate warming impacts include reduce overland flow during the key melt period due to a deeper active layer and a longer unfrozen period increasing infiltration and active layer water storage (Rouse et al., Citation1997). At the same time, warming will likely cause drying of the catchment surrounding ponds. Evaporation from ponds in the area can be strongly affected by drying of terrestrial vegetation and the surrounding air (Bello and Smith, Citation1990), which could lead to water deficits or complete loss of ephemeral ponds (Smol and Douglas, Citation2007).
Forest and tundra fires occur frequently over large areas within the Hudson Bay Lowlands and are predicted to increase in frequency and extent in response to climate change (Brook, Citation2006). Changes in nutrient levels associated with fire have been documented in other high latitude areas (e.g., McEachern et al., Citation2000). However, the two ponds (1 and 6) in our study that had been affected by fire do not show elevated nutrient levels compared to other ponds in the study. Similar to the conclusions of Vitt and Bayley (Citation1984) and MacLean et al. (Citation1983), ponds within the park do not appear to be particularly sensitive to fire and this is one of the climatically mediated variables that may have less of an effect on limnological conditions within the park.
The ponds of Wapusk National Park show considerable variability over the relatively short distances encompassed by the park. The diversity observed in pond water chemistry is undoubtedly due to the position of the park, which spans permafrost to boreal environments and retains the fingerprint of past isostatic rebound. Although climate change scenarios for the region remain uncertain in the short term, long term warming of the area will likely result in migration of both permafrost and tree line, and are likely to strongly impact the character of the ponds in the park. Currently the park shows a diverse range of limnic conditions primarily related to the distance of ponds from the coast, the proximity to tree line, and the stabilizing effect of permafrost on pond-water ionic composition. Monitoring the ponds within the park should focus on parameters related to these variables.
Acknowledgements
We would like to thank Bob Reside (Chief Park Warden Wapusk), Greg Lundie, and Melissa Gibbons for logistical support and assistance in the field.
References
- Alexander , V. , Whalen , S. C. and Klingensmith , K. M. 1989 . Nitrogen cycling in Arctic lakes and ponds . Hydrobiologia , 172 : 165 – 172 .
- Bello , R. L. and Smith , J. D. 1990 . The effect of weather variability on the energy balance of a lake in the Hudson Bay Lowlands, Canada . Arctic and Alpine Research , 22 : 98 – 107 .
- Brook, R. K. 2005. Mapping fires in the Greater Wapusk ecosystem: Historical fire mapping and updating the 1996 vegetation map. Unpublished report prepared for Wapusk National Park of Canada, 83 pp.
- Brook , R. K. and Kenkel , N. C. 2002 . A multivariate approach to vegetation mapping of Manitoba's Hudson Bay Lowlands . International Journal of Remote Sensing , 23 : 4761 – 4776 .
- Brook, R. K. 2006. Forest and tundra fires in the Hudson Bay Lowlands of Manitoba. In Climate change: Linking traditional and scientific knowledge, ed. R. Riewe and J. Oakes, 365378. Winnipeg, Manitoba, Canada: Aboriginal Issues Press.
- Douglas , M. S. V. , Smol , J. P. and Blake , W. 1994 . Marked post-18th century environmental change in High-Arctic ecosystems . Science , 266 : 416 – 419 .
- Downing , J. A. and McCauley , E. 1992 . The nitrogen:phosporus relationship in lakes . Limnology and Oceanography , 37 : 936 – 945 .
- Duguay , C. R. and Lafleur , P. M. 2003 . Determining depth and ice thickness of shallow sub-Arctic lakes using space-borne optical and SAR data . International Journal of Remote Sensing , 24 : 475 – 489 .
- Eilers , J. M. , Brakke , D. F. and Henriksen , A. 1992 . The inapplicability of the Gibbs model of world water chemistry for dilute lakes . Limnology and Oceanography , 37 ( 6 ) : 1335 – 1337 .
- Environment Canada. 1979. Analytical methods manual. Inland Waters Directorate, Water Quality Branch, Ottawa, Ont., 620 pp.
- Gagnon , A. S. and Gough , W. A. 2005 . Climate change scenarios for the Hudson Bay region: An intermodel comparison . Climatic Change , 69 : 269 – 297 .
- Jongman, R. H. G., C. J. F. Ter Braak, and O. F. R. Tongeren. 1995. Data analysis in community and landscape ecology. Cambridge: Cambridge University Press, pp. 1623.
- Lafleur , P. M. and Rouse , W. R. 1995 . Energy partitioning at treeline forest and tundra site and its sensitivity to climate change . Atmosphere-Ocean , 33 : 121 – 133 .
- Laing , T. E. , Pienitz , R. and Payette , S. 2002 . Evaluation of limnological responses to recent environmental change and caribou activity in the Riviere George region, Northern Quebec, Canada. Arctic . Antarctic and Alpine Research , 34 : 454 – 464 .
- Legendre, P. 1990. Quantitative methods and biogeographical analysis. In Evolutionary biogeography of the marine algae of the North Atlantic. NATO ASI series, Volume G 22. ed. D. J. Garbary and R. R. South, 1523. Berlin, Germany: Springer-Verlag.
- MacLean, D. A., S. J. Woodley, M. G. Weber, and R. W. Wein. 1983. Fire and nutrient cycling. In The role of fire in northern circumpolar ecosystems, ed. R. W. Wien and D. A. MacLean, 111132. New York: John Wiley & Sons.
- Martini , I. P. 1989 . The Hudson Bay lowland: Major geological features and assets . Geologie en Mijnbouw , 68 : 25 – 34 .
- McEachern , P. , Prepas , E. E. , Gibson , J. J. and Dinsmore , W. P. 2000 . Forest fire induced impacts on phosphorus, nitrogen, and chlorophyll a concentrations in boreal subarctic lakes of northern Alberta . Canadian Journal of Fisheries and Aquatic Sciences , 57 : 73 – 81 .
- Mortsch , L. D. 1994 . Assessment of the temperature and precipitation of 1990 during the Northern Wetlands Study . Journal of Geophysical Research , 99 : 1429 – 1438 .
- Pienitz , R. , Smol , J. P. and Lean , D. R. S. 1997a . Physical and chemical limnology of 24 lakes located between Yellowknife and Contwoyto Lake, Northwest Territories (Canada) . Canadian Journal of Fisheries and Aquatic Sciences , 54 : 347 – 358 .
- Pienitz , R. , Smol , J. P. and Lean , D. R. S. 1997b . Physical and chemical limnology of 59 lakes located between the Southern Yukon and the Tuktoyaktuk Peninsula, Northwest Territories (Canada) . Canadian Journal of Fisheries and Aquatic Sciences , 54 : 330 – 346 .
- Riley , J. L. 1982 . Hudson Bay Lowland floristic inventory, wetlands catalogue and conservation strategy . Naturaliste Canadien , 109 : 543 – 555 .
- Rouse , W. R. , Douglas , M. , Hecky , R. , Hershey , A. , Kling , G. , Lesack , L. , Marsh , P. , McDonald , M. , Nicholson , B. , Roulet , N. and Smol , J. 1997 . Effects of climate change on the freshwaters of arctic and subarctic North America . Hydrological Processes , 11 : 873 – 902 .
- Ruhland , K. , Priesnitz , A. and Smol , J. P. 2003 . Paleolimnological evidence from diatoms for recent environmental changes in 50 lakes across Canadian arctic treeline . Arctic, Antarctic, and Alpine Research , 35 : 110 – 123 .
- Smol , J. P. and Douglas , M. S. V. 2007 . Crossing the final ecological threshold in high Arctic ponds . Proceedings of the National Academy of Sciences , 104 : 12395 – 12397 .
- Vitt , D. H. and Bayley , S. E. 1984 . The vegetation and water chemistry of four oligotrophic basin mires in northwestern Ontario . Canadian Journal of Botany , 62 : 1485 – 1500 .
- Welch , H. E. and Legault , J. A. 1986 . Precipitation chemistry and chemical limnology of fertilized and natural lakes at Saqvaqjuac, N.W.T . Canadian Journal of Fisheries and Aquatic Sciences , 43 : 1104 – 1134 .
- Wrona , F. J. , Prowse , T. D. , Reist , J. D. , Hobbie , J. E. , Lvesque , L. M. J. and Vincent , W. F. 2006 . Climate impacts on arctic freshwater ecosystems and fisheries: Background, rationale and approach of the Arctic Climate Impact Assessment (ACIA) . Ambio , 35 : 326 – 329 .