Abstract
Temporary streams lack streamflow at some time in the seasonal cycle, and include ephemeral, intermittent and episodic streams. They often serve as headwaters for the perennial stream network in a drainage basin, and given that headwater streams can comprise the majority of the drainage network, temporary streams are significant hydrologic features across the country. Nevertheless, they have received relatively little attention compared to perennial streams. In addition, much previous work on temporary streams has focussed on semi-arid and arid landscapes where annual evapotranspiration exceeds annual precipitation. While such climatic conditions do control the occurrence of temporary streams in some regions in Canada, temporary streams can also occur in sub-humid and humid climates. This paper examines the major controls on the occurrence and behaviour of temporary streams at the regional and reach scales in Canada; however, where necessary we also review literature from outside Canada on aspects of temporary streams relevant to the Canadian context. The paper assesses the temporal dynamics of temporary streams, along with key aspects of their geomorphology and ecology as well as current monitoring and modelling approaches. Temporary streams are very sensitive to anthropogenic and natural activities that can modify their hydrology and hydroecology, and they deserve greater attention from the Canadian hydrological community. Improved monitoring and process studies should be pursued in Canada.
Les cours d'eau temporaires manquent dcoulement fluvial certains moments au cours du cycle saisonnier et ils englobent les cours d'eau phmres, intermittents et pisodiques. Ils servent souvent de cours d'eau suprieurs pour le rseau de cours d'eau prennes dans un bassin hydrologique et, du fait que les cours d'eau suprieurs peuvent constituer la majeure partie du rseau hydrographique, les cours d'eau temporaires reprsentent des caractristiques hydrologiques d'importance la grandeur du pays. Nanmoins, une attention relativement minime leur a t accorde comparativement aux cours d'eau prennes. De plus, les travaux antrieurs sur les cours d'eau temporaires ont en grande partie mis l'accent sur les paysages arides et semi-arides o lvapotranspiration annuelle dpasse les prcipitations annuelles. Bien que de telles conditions climatiques exercent effectivement une influence sur la prsence des cours d'eau temporaires dans certaines rgions du Canada, les cours d'eau temporaires peuvent galement tre prsents dans des climats subhumides ou humides. La prsente communication examine les principaux facteurs qui ont une emprise sur la prsence et sur le comportement des cours d'eau temporaires lchelle rgionale et lchelle des passages au Canada; cependant, l o cela s'avre ncessaire, nous nous penchons galement sur de la documentation de l'extrieur du Canada portant sur des aspects des cours d'eau temporaires pertinents pour le contexte canadien. L'article value la dynamique temporelle des cours d'eau temporaires, ainsi que les aspects cls de leur gomorphologie et de leur cologie, de mme que les approches de surveillance et de modlisation actuelles. Les cours d'eau temporaires sont trs sensibles aux activits anthropiques et naturelles pouvant modifier leur hydrologie et leur hydrocologie et mritent une plus grande attention de la part de la communaut hydrologique canadienne. Une meilleure surveillance et de meilleures tudes de processus s'imposent au Canada.
Introduction
Much of our understanding of stream hydrology, in Canada and elsewhere, is based on examination of perennial streams (Williams, Citation1988) that flow constantly during a typical hydrologic year (Nadeau and Rains, Citation2007). Baseflow to these perennial streams is maintained by groundwater discharge to the streambed, as the groundwater elevation adjacent to the stream is typically higher than the elevation of the streambed itself (Levick et al., Citation2008). However, a large number of streams may cease to flow for variable time periods and can thus be defined as temporary streams, which include intermittent, ephemeral and episodic streams.
Intermittent streams are timed seasonally: they flow during times of the year in which rainfall and, in Canada in particular, snowmelt inputs exceed losses to groundwater and evapotranspiration. This seasonal tie means that they flow with a regular frequency, and their flow duration depends on the magnitude of the inputs. An intermittent stream may have alternating dry and flowing reaches during low flow periods (Leopold and Miller, Citation1956) and may cease to flow entirely during very dry periods. In some cases water will enter underlying groundwater systems (characterized as a losing or influent stream, Allaby and Allaby (Citation1999); ). Uys and O'Keeffe (Citation1997) distinguished between intermittent seasonal (surface flow disappears for a period of each year or for a sequence of years; flow commences only in the wet season and may be sustained or intermittent during this season) and intermittent aseasonal streams (exhibit intermittent, unpredictable and highly variable flow within and between years; flow follows no distinct pattern and drying may occur in any season; duration of flow, no flow and drying events are highly variable within and between years, depending on antecedent climatic conditions). Ephemeral streams flow only in direct response to rainfall or snowmelt. They do not receive appreciable water quantities from any other source, and their channels are above the water table at all times (; Nadeau and Rains, Citation2007). The term episodic has been used to encompass both intermittent and ephemeral streams, but has also been applied to highly flashy systems that flow only following extreme rainfalls that exceed a threshold rainfall depth. These streams may not flow during any sequence of years, or only once in several years (Uys and O'Keeffe, Citation1997).
Figure 1. A conceptual model of the continuum concept (after Uys and O'Keeffe, Citation1997). Three hydrological states are shown: perennial where water is always present; intermittent where flow disappears, but water remains; and ephemeral where water disappears. The right panels show channel flow (shaded areas) for these hydrological states in relation to the water table (dotted lines).
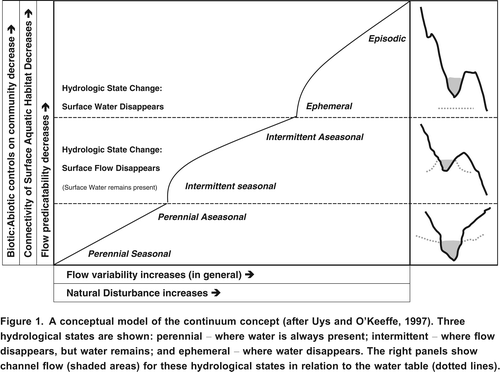
Regardless of their type, temporary streams are characterized by the repeated onset and cessation of flow, and by complex hydrological dynamics in the longitudinal dimension. illustrates the two types of hydrological state changes (Uys and O'Keeffe, Citation1997): surface flow disappears but some surface water is present (which separates perennial from intermittent streams), and disappearance of surface water from the channel for long periods (which separates intermittent from ephemeral streams). This classification is consistent with recent work (e.g., Svec et al., Citation2005) that applies flow duration analysis to distinguish between perennial, intermittent, and ephemeral streams. Perennial and temporary streams can also be placed on a continuum of: (1) flow variability, (2) natural disturbance, (3) the ratio of biotic:abiotic controls on the stream community, (4) connectivity of surface aquatic habitat, and (5) flow predictability (Uys and O'Keeffe, Citation1997; ). Increases in the first two attributes and decreases in the last three create a spectrum of stream types ranging from perennial to intermittent to ephemeral to episodic.
Temporary rivers comprise up to one third of the total number, length and discharge of the world's rivers (Poff, Citation1992; Tooth, Citation2000). However, they are rarely discussed in modern hydrologic textbooks. The index of Dingman (Citation1993) contains one mention each of ephemeral or intermittent streams, while the indexes for Hornberger et al. (Citation1998), Brutsaert (Citation2005) and Bedient et al. (Citation2008) make no mention of ephemeral or intermittent streams (although the latter has a single mention of influent streams). Results from the conterminous United States suggest that intermittent and ephemeral streams comprise ~59% of total stream length (Nadeau and Rains, Citation2007). Unfortunately, there are no comparable data on the fraction of drainage networks in Canada that consists of temporary streams. Our understanding of the hydrologic and biogeochemical behaviour of such streams is incomplete, as most Canadian hydrometric stations and water quality monitoring sites tend to be on perennial streams (Tzoraki et al., Citation2007), and most ecological studies are conducted either in wetter locations or on drier uplands (Levick et al., Citation2008).
Temporary streams are among the most common and hydrologically-dynamic freshwater ecosystems (Larned et al., Citation2010). Their predominant location in headwaters means that they supply water, sediment, nutrients, seeds, and organic matter for downstream systems, as well as habitat for many species (Gomi et al., Citation2002; Boulton et al., Citation2010). Temporary streams may drive much of the hydrological and ecological connectivity in a landscape (Levick et al., Citation2008), acting biogeochemically as landscape hotspots (high reaction rates relative to their surroundings) and experiencing hot moments (short time periods with disproportionately high reaction rates relative to longer periods with low reaction rates, sensu McClain et al., Citation2003) during periods of expansion and integration with the perennial drainage network. They are vital parts of the biological integrity of the entire drainage network, such that their degradation and loss of connection to larger streams has negative consequences not only for the inhabitants of temporary streams, but also for downstream diversity (Levick et al., Citation2008). There have been recent calls in the United States (US) for enhanced understanding of the hydrology, hydrochemistry and ecology of such headwater streams (e.g., Bishop et al., Citation2008), partially driven by a 2006 ruling by the US Supreme Court regarding the Clean Water Act. This stated that a temporary stream must have a significant nexus to navigable water in the US for the former to fall under the jurisdiction of the Clean Water Act, thus protecting it from development and alteration (Cataldo et al., Citation2010).
While there is no such catalyst to improve knowledge of temporary Canadian streams, there is clearly a need for an enhanced understanding of these systems. This paper provides an overview of the hydrologic, geomorphic, biogeochemical and ecological properties of temporary streams, along with current monitoring and modelling approaches for such streams and suggested areas for further research. While temporary streams exist around the world, the emphasis here is on temporary streams in Canada, as there are aspects of their hydrology that are relatively unique to the Canadian landscape. These include the role of snowmelt and permafrost in controlling stream sensitivity and response to water inputs and releases from storage. Given the extent of Canada that is mantled by continuous and discontinuous permafrost, it is particularly important to understand how climate change will affect the hydrology of both perennial and temporary streams in these regions. Research results from studies of temporary rivers from other parts of the world that are applicable to Canada will also be highlighted, particularly where no Canadian literature exists. A key objective of this paper is to build on our understanding of the hydrology, geomorphology and ecology of temporary streams to examine how these characteristics may change in response to various direct and indirect environmental stressors.
Controls on Temporary Stream Occurrence and Behaviour
The factors that determine whether a stream is perennial or temporary vary from those that operate at the continental or sub-continental scale to local considerations of geology and vegetation cover. Thus, it is possible to distinguish between controls on temporary stream occurrence and behaviour operating at the regional and reach scales.
Regional Scale Controls
Climate
Climatic conditions, such as the amount and timing of precipitation (P) and energy inputs in a region, are generally considered a first-order control on the occurrence of temporary streams. Several studies (e.g., Levick et al., Citation2008) have emphasized the linkage between the presence of temporary streams and arid and semi-arid regions (drylands) characterized by low and variable annual P that is exceeded by annual potential evapotranspiration (PET) (). Indeed, much of the research on temporary rivers has been conducted in such landscapes (e.g., US southwest, central Australia). Dry-subhumid areas extend northwards from the US into Alberta, Saskatchewan and western Manitoba, while semi-arid conditions extend into southern Alberta and Saskatchewan as well as parts of southern BC ( inset). Snowfall is a critical component of annual P in these areas. An issue of particular importance to water supply to streams in the southern Prairies is the redistribution of snow from upland areas into topographic depressions. Meltwater generated from this redistributed snow may lead to a rapid and pronounced spring streamflow response (Fang et al., Citation2010), while recharge from melting of thinner snowcover on upland areas may be insufficient to sustain flows throughout the summer. However, Canadian temporary streams can also occur in regions that fall outside these drylands, such as Arctic streams that cease to flow in winter (Young and Woo, Citation2000) and wetland lagg streams that only flow when excess water is available (Quinton and Roulet, Citation1998).
Figure 2. Global distribution of hyper-arid, arid and semi-arid regions (as defined by the United Nations Environment Programme's Global Humidity Index, UNEP/GRID, Citation1991). Inset map shows arid and semi-arid regions in western North America.
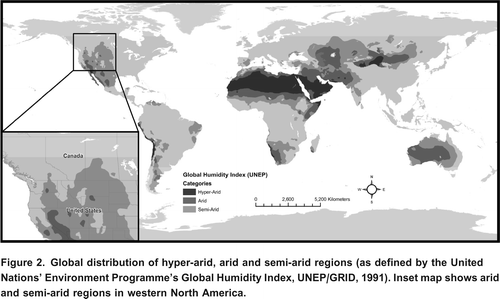
It may be instructive to view the balance between energy considerations (e.g., net radiation inputs to the ground surface, the liquid or solid state of water reaching the ground surface as dictated by air temperature) and water availability (P ET) as a determinant of whether streams are perennial or temporary. This requires consideration of seasonal variability in the P-ET relationship, as well as the form of P (rain or snow). On an annual basis, P > ET would suggest that there is sufficient water to supply baseflow to a perennial stream; however, P < ET conditions may exist on a seasonal basis, particularly in summer. The magnitude of this deficit may result in the stream drying up for a period of time, thus producing intermittency.
contrasts mean monthly P and potential and actual ET for Saskatoon, Saskatchewan and Peterborough, Ontario. Pronounced summer soil water deficits are not surprising given the former's location on the boundary of the semi-arid hydroclimatic region (). However, while annual P > ET at the latter location, temporary headwater streams are still common during periods of summer water deficit. Temporary rivers can also be found in humid cold parts of Canada, such as in central and northern Quebec. Here P > ET at the annual scale (Hydrological Atlas of Canada, Citation1978); nevertheless, cold winter temperatures mean that P accumulates as snowcover throughout the winter and thus does not sustain flow in smaller streams which may freeze into their beds. This exemplifies a situation where energy controls on the phase of both P and water in the stream channel override the importance of water availability in dictating whether a stream is perennial or temporary. Energy controls are also manifested in topographically-controlled variations in the surface energy balance, which governs both the spatial pattern of snowmelt and active layer development in permafrost landscapes (Quinton and Carey, Citation2008) and the volume of melt production from glaciated regions. These in turn can dictate the timing and magnitude of water delivery from slopes and/or glaciers to the stream channel, which in turn control whether the stream experiences zero flow periods at some time during the year.
Hydrogeology
It is critical to consider hydrogeological conditions in a given landscape (Winter, Citation2007), especially the storage (e.g., porosity and thickness of deposit or formation) and transmission (e.g., hydraulic conductivity) properties of the overburden and bedrock. These conditions can enhance or override the influence of hydroclimatic conditions on the potential occurrence of temporary streams. Winter (Citation2007) noted that headwater streams originating in rocks or sediments with low permeability have small incipient discharge, a point of origin that moves up and down the channel seasonally, and that these streams commonly go dry at some time during the year. Conversely, streams with their sources in extensive aquifers generally have substantial discharge and a stable point of origin.
As noted above, although annual P > ET across much of Canada's Precambrian Shield, water deficits can develop during the summer. In areas with relatively impermeable bedrock mantled by thin, rapidly drained soils, hillslopes may not store sufficient water to sustain baseflow (Devito et al., Citation1996), resulting in an intermittent stream that is dry during the summer. Such a landscape would likely also contain numerous ephemeral streams that flow during or following episodic water inputs (rainfall, snowmelt) due to limited storage capacity in hillslope soils combined with rapid transmission of inputs to stream channels (e.g., Buttle et al., Citation2004). Alternatively, few temporary streams may occur when annual P > ET in a landscape with a different hydrogeology. For example, the Oak Ridges Moraine in southern Ontario consists of thick, permeable glacial and glaciofluvial deposits with immense water storage capacity. Overlying soils generally have high infiltration capacities, while the stratigraphy of the morainal deposits may not include a near-surface layer of reduced hydraulic conductivity that would encourage lateral subsurface stormflow to stream channels. Thus, the stream network is sustained by substantial baseflow contributions and remains relatively fixed in space (e.g., Waddington et al., Citation1993), with minor up-gradient extension of stream channel heads during or following storms or snowmelt since most inputs infiltrate and recharge deeper groundwater.
Drainage Network Topology
In the context of hydrology, topology concerns the relative role of components of a flow network in modulating water inputs from slopes as they move to the basin outlet, and thus is an index of the degree of hydrologic connectivity of the stream network (Buttle, Citation2006). In addition to the role of climate and hydrogeology, occurrence and behaviour of temporary streams is partly dictated by connectivity to upstream water sources, such as lakes and wetlands. When storage in these landscape elements falls below a specific threshold (such as the lake outlet level), then these features may no longer contribute surface flow to downvalley channels, resulting in the cessation of streamflow. Therefore, the storage-discharge relationships of these landscape elements can be a critical control on their ability to sustain downstream flow, and can dictate whether a given portion of the drainage network is perennial or temporary. Spence and Woo (Citation2006) illustrated this for the Canadian Shield near Yellowknife, NWT, where streamflow is frequently intermittent because runoff from the upper watershed must fill landscape storage requirements before flow is contributed further downstream. These insights are part of the development of the Element Threshold or storage excess conceptual model of basin hydrology (Spence and Woo, Citation2006; Sayama et al., Citationin review), and are discussed in greater detail in the context of the temporal dynamics of temporary streams.
Reach Scale Controls
Reach scale factors operate both at a finer spatial scale and within the context of regional scale controls described above, as they determine whether a particular section of a stream network exhibits temporary flow properties. As with the regional controls, the main determinant of the occurrence of a temporary stream reach is the balance between water inputs and outputs (). The stream will carry surface water if inputs (e.g., P, lateral inputs of surface and subsurface water, vertical inputs from groundwater, inputs from upstream) exceed outputs (e.g., evaporation (E) from the water surface, transpiration by vegetation in the stream and the adjacent riparian zone, infiltration of water through the streambed, discharge out of the reach). Thus, it is possible to find temporary stream reaches even in regions where P > ET on both annual and seasonal bases.
Figure 4. Water inputs and outputs for a stream reach: 1 inflow from upstream; 2 precipitation onto stream surface; 3 lateral surface inflow; 4 subsurface inflows; 5 evaporation from stream surface; 6 evapotranspiration from riparian vegetation; 7 overbank flow losses; 8 infiltration through streambed; 9 outflow from reach.
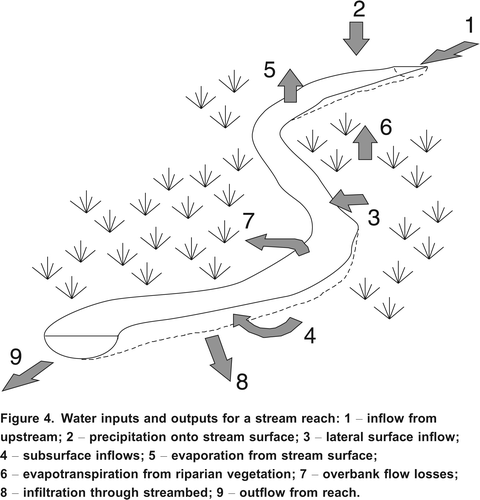
A stream reach is gaining if groundwater discharge to the channel exceeds infiltration and losing if the opposite condition exists. Local potentiometric gradients are directed towards the channel in the former case, and away from the channel in the latter (). The water table may drop below the streambed due to a combination of reduced water input from upslope areas and water loss by riparian zone ET and infiltration through the streambed and banks (Richardson and Moore, Citation2009). Winter (Citation2007) notes the role of ET by riparian vegetation in causing groundwater levels to drop and thus encouraging loss of stream water. Riparian cottonwoods (Populus sp.) in the southern Canadian Prairies, for instance, are dependent on shallow alluvial groundwater that is linked to stream water (Rood et al., Citation2003).
Intra-basin variations in hydrogeological conditions (storage and transmission properties) are also critical in controlling whether a given stream reach is perennial or temporary. As noted earlier, thin soils over bedrock at the regional scale may have insufficient storage to maintain lateral water inputs to a stream reach during summer dry periods. Such hydrogeological controls may also occur in localized areas of deeper overburden. For example, Todd et al. (Citation2006) found a significant relationship between flow duration from sub-basins of a drumlinized 78 km2 basin in southern Ontario and the fraction of the sub-basin area consisting of sand and gravel deposits. Sub-basins containing these deposits may be connected to relatively large local groundwater systems that can maintain streamflow for most, if not all, of the summer dry period. Another illustration of hydrogeological control on reach scale conditions is the role of bed material type and elevation. Incursions of landslides or other debris into the main channel of streams in BC's Okanagan region results in the disappearance of the stream under this material and its subsequent reappearance further downstream (E Huxter, pers. comm., Citation2011).
The presence of bedrock fractures may also result in major alterations in stream connectivity and associated sudden losses or gains in streamflow along the watercourse that are independent of overburden characteristics. The hydrogeology of karst landscapes is probably the most dramatic example of the influence of bedrock geology on stream behaviour; there can be a strong relationship in karst landscapes between the occurrence of losing streams and lineaments and fracture traces in limestone bedrock (e.g., George, Citation2007). Surface streams may lose some or all of their flow upon reaching point inputs known as swallow holes (also referred to as swallets or stream-sinks). Ford and Williams (Citation2007) emphasize the balance between the amount of streamflow supplied to the swallow hole and the capacity of the input passages in the karst to regulate recharge, such that inflow in excess of recharge may allow surface flow (streamflow) to continue downvalley of the swallow hole. For example, there is no regional groundwater body in the Nahanni Karst in the Northwest Territories, yet groundwater fluxes are crucial to maintaining flow in the South Nahanni River and its tributaries. Groundwater moves along independent or poorly connected conduits and aquifers that are recharged during spring melt and release water during large summer rainstorms (Brook and Ford, Citation1980).
Special consideration must be paid at the reach scale to transmission loss: the infiltration of water from the stream channel through the bed and banks. Transmission loss can produce significant downstream decreases in total flow volumes, flood peaks, and flood frequencies (Tooth, Citation2000). The rate of transmission loss from a river reach is a function of the characteristics of the channel alluvium (such as its sedimentology and antecedent wetness), channel geometry, wetted perimeter, flow characteristics (including volume and duration), and depth to groundwater (Cataldo et al., Citation2004; Levick et al., Citation2008).
Transmission losses from temporary streams can be considerable (; ). Estimates of such losses for temporary streams in Canada are rare, although Todd et al. (Citation2006) obtained values ranging from 0.62103 to 6.2103 m3km1 in a wetland-dominated basin with an intermittent drainage network in southern Ontario, which are similar in magnitude to those reported at sites in other countries (). Transmission losses from temporary streams may provide an important source of groundwater recharge in some landscapes. For example, Todd et al. (Citation2006) reported that groundwater recharge from wetlands and intermittent streams channels was ~22% of the spring-to-fall input of 1,020 mm to a 78 km2 basin in southern Ontario. Such infiltration losses may form a moist but unsaturated curtain directly below and adjacent to the channel that penetrates well below the rooting zone and facilitates regional ground-water recharge (Nadeau and Rains, Citation2007, p 126). A type of transmission loss distinct to lake-dominated watercourses, such as those on the Canadian Shield, is via lake E. The attenuating influence of lakes increases the opportunity for E losses, which can prevent small runoff events from maintaining a measurable flood wave through a basin (Spence, Citation2006).
Figure 5. Frequency of transmission losses from 101 storm events as a percentage of upstream flow for 31 reaches monitored on 19 streams in the western and mid-western US (Cataldo et al., Citation2004).
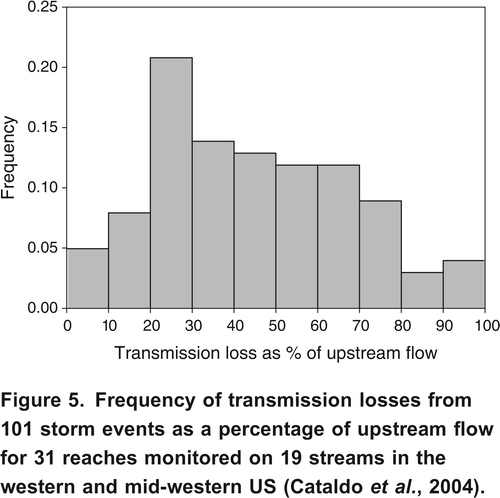
Table 1. Examples of transmission losses during floods in dryland rivers (after Knighton and Nanson, Citation1997; Tooth, Citation2000).
Climatic controls remain important at the reach scale, since the nature of water inputs to a basin can affect downstream reductions in flow. Storm characteristics (size, position of the storm track in relation to the drainage net) are significant for transmission losses, particularly in larger basins or those subject to discrete convective events (Tooth, Citation2000). Widespread precipitation or snowmelt may result in tributary contributions that can lead to downstream increases in flow even in the face of significant transmission losses. Conversely, spatially-localized precipitation combined with hydrograph attenuation and an absence of appreciable tributary inputs in the lower part of the basin can result in significant downstream decreases in total flow volume, flood peak, and flow frequencies (Levick et al., Citation2008).
While transmission losses remove water from a temporary stream reach, they may also play an important role in maintaining hydrologic connectivity at the larger basin scale. Winter (Citation2007) gave the example of streams emanating from mountains and flowing across alluvial fans in arid, semi-arid and sub-humid landscapes. These streams may disappear as they flow across the fan and their water becomes part of the groundwater flow system. Nevertheless, these infiltration losses provide hydrologic continuity to areas downgradient of the fan, where the water may eventually return to the surface and supply streamflow to reaches in lower parts of the basin.
Other Factors Particular to the Canadian Environment
Permafrost (ground with temperatures that remain below 0C for at least two consecutive years) underlies over half of Canada (Brown, Citation1970). It restricts hydrologic interaction between near-surface supra-permafrost water and sub-permafrost groundwater (Quinton and Carey, Citation2008), and its presence and extent control the rate at which precipitation and stormflows are transmitted to streams in much of northern Canada. Permafrost also plays a significant role in temporary stream formation given the seasonality of active layer thickness. Abundant snowmelt combined with low storage capacity associated with a shallow active layer can produce extensive temporary runoff. Deep, late-lying snow drifts are important to the maintenance of streams in the High Arctic, as this snow can provide water well into the summer when all other sources have been extinguished (Young et al., Citation1997). The volume of available storage increases as the active layer thickens through the summer, reducing the amount of water that can be released downslope and increasing intermittency (McCartney et al., Citation2006; Woo and Steer, Citation1982). Active layer thickness is itself a function both of air temperature and streamflow; sensible heat is transferred to the active layer through radiation absorption and advective heat from water flow (Guan et al., Citation2010). When air temperatures begin to fall below freezing later in the season, streamflow can be maintained as the stream water temporarily insulates the underlying substrate from seasonal refreezing.
Water inputs to temporary streams may also result from the seasonal production of glacier meltwater. In periglacial environments, temporary streamflow is driven by both glacier meltwater inputs and seasonal thawing of the ice-cemented permafrost to create an active layer. Seasonal flow in these systems is thus largely a function of suitable energy inputs to create glacier melt and runoff (e.g., Orwin et al., Citation2009). While Canadian researchers have studied the proportion of streamflow derived from glacier melt and the potential implications for stream temperature and ecology (Young and Ommanney, Citation1984; Munro, Citation2000, Citation2005), the contribution of glacier-fed systems to the temporary stream continuum has not been directly quantified.
Streamflow Dynamics of Temporary Streams
Conceptual Models of Streamflow Generation
As noted previously, gaining and losing streams and stream reaches are defined by potentiometric gradients, which may be highly variable both in time and space. Assuming no marked gradients in P and no major variations in the hydrogeologic conditions in the basin, the likelihood of a stream reach being a gaining one increases with distance down basin, since elevation head comprises most of the total potentiometric gradient. The classic conceptual model, by which an intermittent headwater stream becomes a gaining stream, is a rising water table below the channel fed by groundwater from adjacent hillslopes (Hewlett and Hibbert, Citation1967; Dunne, Citation1978). As the water table continues to rise in elevation, so too does the stream head, and the stream and contributing area expand upvalley (or contract downvalley during drying) in accordance with the Variable Source Area (VSA) concept.
Studies in Canadian landscapes (e.g., Spence and Woo, Citation2003; Buttle et al., Citation2004) have identified situations where landscape and snowmelt heterogeneity result in upslope P and flows rather than rising groundwater fluxes from below driving intermittent flow in a reach. These situations adhere more to the Element Threshold concept (Spence and Woo, Citation2006), which posits that runoff is generated from a landscape element only when its storage threshold is exceeded. The Element Threshold concept appears to apply particularly where basins are highly heterogeneous or the climate is relatively dry. There is a potential in a basin's headwaters for several water reservoirs to exist within adjacent hillslopes, each with a different storage-discharge relationship. The synchrony with which each of these reservoirs reacts during wet periods will influence how temporary streams respond to water inputs. In many Canadian landscapes, the location of wetlands (e.g., Branfireun and Roulet, Citation1998; Todd et al., Citation2006) and the state of ground frost (Carey and Woo, Citation2001) are important for controlling this synchrony. Transience of these storage-discharge processes depends greatly on the interplay of the magnitude and duration of rain or snowmelt inputs and ET in near-stream or riparian zones (Spence and Woo, Citation2006).
Differences between these two conceptual models of streamflow generation also have implications for the flow characteristics of temporary streams. Unlike perennial streams, which are fed by groundwater contributions to baseflow during dry periods, temporary streams do not have access to consistent storage from which water for streamflow can be obtained. Thus, the frequency and duration with which flow does occur depends on how easily storage can be replenished and maintained, and the mechanism by which storage is replenished influences the temporal dynamics of temporary streamflow.
When runoff is controlled by processes that can be conceptualized with the VSA model, groundwater contributions can sustain a minimum saturated area within parts of the basin even during drought. Thus, there is the potential for saturation overland flow from these areas even during summer rainfalls, leading to a continuous change in runoff ratios: from small (but non-zero) values when the VSA is at its minimum (during summer dry periods) to large values at times of peak basin wetness. An example of such a system would be the HP4-21 basin, a 4.1 ha headwater basin in south central Ontario mantled by till up to 15 m in depth. The basin maintains a small surface-saturated area upstream of the outlet, which expands during wetter conditions, such that the ratio of the depth of quickflow (QF; Hewlett and Hibbert, Citation1967) to P tends to increase with increasing rainfall (a).
Figure 6. Quickflow/precipitation (QF/P) ratios for the HP4-21 and PC1-08 basins, south central Ontario. Data are for rainfall events recorded from March 1, 1988 to May 31, 1992.
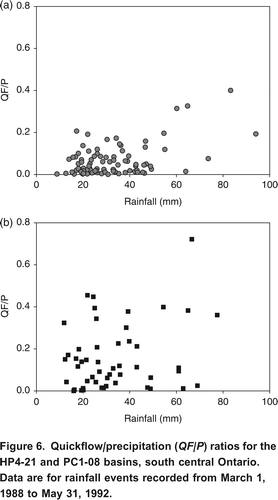
Conversely, water inputs can produce remarkably variable runoff ratios where streamflow generation is better described by the Element Threshold concept. Most runoff ratios are at or near zero for small storms; however, given the threshold-mediated nature of these basins, runoff ratios can be quite large when contributing areas expand quickly. This is illustrated by the PC1-08 basin, within 30 km of HP4-21 and of similar size (3.4 ha), but which consists of bedrock outcrops and thin till cover. QF:P ratios are much larger for modest rainfall events than is the case for HP4-21, and the general increase in this ratio with event size observed for HP4-21 is not seen (b).
Discussions of the relationship between competing conceptual models of streamflow generation in temporary streams and the resulting streamflow characteristics should be examined in the context of the role that input magnitude plays in the relationship between hydrologic processes and basin physiography. Graf (Citation1988), in his analysis of fluvial processes in arid landscapes, hypothesized that processes control forms in high magnitude events, while forms control processes in low magnitude events. Camarasa and Tilford (Citation2002) extended this concept in their discussion of modelling ephemeral streamflows in semi-arid eastern Spain, where dominant events are infrequent but of large magnitude. Surface-runoff predominates during floods, as the role of basin characteristics is reduced substantially relative to rainfall properties (amount, intensity, spatial distribution).
While this is a direct analogue for semi-arid temporary streams in Canada, such as in the badlands of southeast Alberta, it may also be applicable to other temporary stream systems in Canada that fall outside the semi-arid hydroclimatic region. Buttle and Eimers (Citation2009) observed that basin physiography did not explain inter-basin differences in QF response during spring snowmelt in intermittent streams in south central Ontario, and all basins generated similar QF depths. However, in other seasons, basin physiography did explain inter-basin differences in QF production. Inter-basin differences in the ability to store and transfer water inputs (Wagener et al., Citation2007) had a greater influence on stormflow generation than input properties, which were relatively uniform across the basins. Similarly, Todd et al. (Citation2006) noted that all monitored intermittent headwater streams in a basin in southern Ontario generated streamflow during and immediately after spring snowmelt. Nevertheless, only those basins with sand and gravel deposits that could serve as local groundwater systems were able to sustain streamflow throughout the summer and respond to smaller rainfall inputs.
Streamflow Metrics
Lag Times
It can be hypothesized that basins that generate runoff via the VSA model should have relatively short lag times between peak rainfall and peak runoff, particularly if they contain saturated areas in the lower basin that would generate rapid runoff via saturation overland flow. For example, Dunne (Citation1978) gave lag times for VSA hydrographs of 6 hours for basins less than ~100 km2 in area. This can be contrasted with temporary streams where storage of inputs associated either with basin size or heterogeneity in landscape elements delays the coalescence of the stream network, leading to lag times as long as three weeks in places where large storage deficits accrue in downslope elements (Spence and Rouse, Citation2002). Conversely, runoff to temporary streams in semi-arid areas such as the Alberta badlands is often generated by Horton overland flow (Bryan and Campbell, Citation1986), and lag times may be 1 hour for basins 10 km2 (Dunne, Citation1978).
Recession Coefficients
Differences in storage properties between basins drained by perennial versus temporary streams may also manifest themselves in recession coefficients, with larger values indicating a more protracted hydrograph recession. The short-lived streamflow response to P inputs for ephemeral and episodic streams, combined with transmission losses as water moves along the stream channel, might be expected to produce a relatively steep hydrograph recession limb and small recession constants compared to perennial basins where baseflow is sustained by groundwater inputs. Documented recession coefficients are rare for Canadian temporary stream basins that both exhibit traits of the Element Threshold concept and are larger than a few hectares (Allan and Roulet, Citation1994; Spence and Woo, Citation2003). In those studies that are available, recession coefficients are all on the order of 0.70.8 d1. Conversely, Dunne (Citation1978) gave recession constants for VSA basins ranging from 0.001 to 0.9 d1. Thus, the existing data do not support the assumption presented above, which may partly reflect both their scarcity and consequent inability to represent the full range of temporary stream system types explored here. Nevertheless, a more rigorous examination of the recession coefficients of perennial and temporary streams in different landscapes across Canada is warranted, particularly given the value of these coefficients in assessing basin storage characteristics and for the design of storage structures on watercourses.
Peak Flow Properties of Temporary Streams
Wolman and Gerson (Citation1978) argued for a distinct difference in the effectiveness of diverse rainfall events of similar frequency on hillslopes and on adjacent low order streams, both in humid and arid climates. In humid climates, the headwaters of larger perennial rivers are often ephemeral or intermittent streams; thus, the frequency of surface runoff is very low on hillslopes and in first order streams compared to higher order channels. Arid and semi-arid regions, however, experience a greater frequency of flow on hillslopes than in the adjacent streams, where much of the potential overland flow percolates into downslope colluvium or into in-channel sediments. Nevertheless, flow frequency may increase with distance downstream.
Tooth (Citation2000) noted that dryland rivers typically have a high ratio of large to small flows, which leads to highly skewed flood frequency distributions and steep regional and relative flood frequency curves which are typically established by a few very large floods. Furthermore, steep portions of a flood frequency curve can indicate the activation of temporary headwater streams (Ehsanzadeh et al., Citation2011). This is consistent with the progressively nonlinear runoff-rainfall relationship with increasing basin area often observed in temporary streams. The nonlinearity is partly a result of channel transmission losses and partial area storm coverage (Goodrich et al., Citation1997), but is also due to differences in the relationships between contributing area and the number of stream sources for perennial and temporary streams. Thus, Hansen's (Citation2001) study of well-defined drainage networks in the US Appalachian Mountains found that the relatively strong power function between basin size and number of stream sources became weaker as streams became more temporary.
Transmission losses may also account for differences in mean annual runoff volume versus drainage area relationships for perennial and ephemeral streams observed in the United States (Wolman and Gerson, Citation1978). While mean annual runoff volume increases with drainage area for both perennial and ephemeral streams, the rate of increase is much lower for the latter and the degree of variability around the best-fit relationship is much greater (a). Similar results were obtained for perennial and temporary streams that are part of the Canadian Reference Hydrometric Network (c). Re-expression of the Wolman and Gerson (Citation1978) data as mean annual runoff depth shows no obvious relationship between runoff depth and drainage area for perennial streams, but a significant decrease in mean annual runoff with basin size for ephemeral streams (b). This decrease is probably the result of transmission losses rather than partial area storm coverage, which would tend to average out across a drainage basin on an annual basis. Higher transmission losses in larger basins likely explain the smaller increase in runoff volume with drainage area for ephemeral streams shown in a, and are consistent with De Boer's (Citation1992) observation that gross drainage area is an unreliable surrogate measure of runoff volume for temporary streams in southern Alberta. Mean annual runoff from perennial streams in the Rocky Mountain or Parkland regions shows no trend with basin area (d), consistent with US perennial rivers. However, although mean annual runoff data from intermittent Canadian Prairie streams suggest a decrease with area, the trend is not significant at the p = 0.05 level. This may partly reflect the smaller number and range in basin sizes for temporary streams in the Canadian data, and a more complete analysis of relationships between runoff depth and basin area for temporary streams in various regions in Canada would be highly useful.
Figure 7. (a) Mean annual runoff volume vs. drainage area for streams in humid, semi-arid and arid regions of the United States, including perennial and ephemeral streams (data from Wolman and Gerson, Citation1978); (b) runoff from (a) re-expressed as equivalent depth vs. drainage area; (c) mean annual runoff vs. drainage area for perennial and intermittent streams gauged as part of the Canadian Reference Hydrometric Basin Network; (d) runoff from (c) re-expressed as equivalent depth vs. drainage area. Least-squares lines of best-fit significant at p = 0.05 are shown.
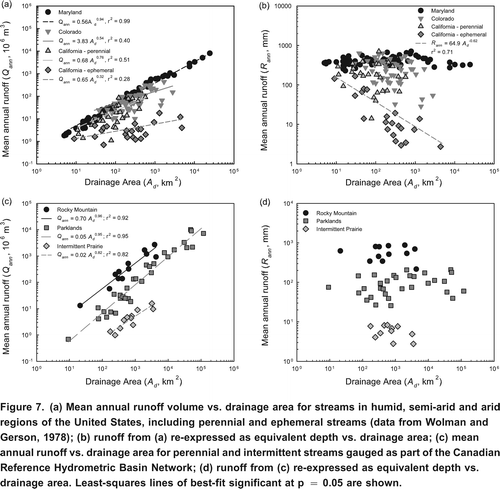
shows regional flood frequency curves for temporary rivers in humid and semi-arid parts of Canada. Floods have been standardized relative to the mean annual flood (Q 2.33 ), while return periods have been estimated using the Gumbel distribution. Humid temporary streams are represented by flows from small (< 2 km2) headwater basins in south central Ontario (monitored by the Dorset Environmental Research Centre (DESC)) that experience zero flow for an average of 30 days per year. For these streams the Q/Q 2.33 ratio never exceeds 2.5 for return periods in the order of 25 years, and values are similar to those presented for basins in the humid eastern US. This may be because, while many of the DESC streams frequently dry up during periods of summer soil moisture deficits, large peak flows are consistently produced during the spring snowmelt period (Buttle and Eimers, Citation2009). Instantaneous peak flows from temporary non-regulated rivers (> 100 km2) gauged by the Water Survey of Canada in southern Alberta and Saskatchewan are used to represent semi-arid basins. The Q/Q 2.33 ratios are much larger than 2.5 at return periods in the order of 25 years, and for some basins ratios for greater return periods are similar to those presented by Farquharson et al. (Citation1992) for arid basins around the globe. Many of the peak flows for the Canadian Prairie basins were producedduring the summer, presumably by convective storms. Results suggest that flood frequency relationships for temporary streams will differ between snowmelt- and rainfall-dominated peak flow regimes.
Figure 8. Peak flows (standardized by the mean annual flood, Q 2.33 ) vs. return period for basins in southern Ontario (DESC basins) and southern Alberta (AB) and Saskatchewan (SK). Regional flood frequency curves for humid basins in the eastern US (from Tooth, Citation2000) and arid basins in various countries (from Farquharson et al., Citation1992) are also shown.
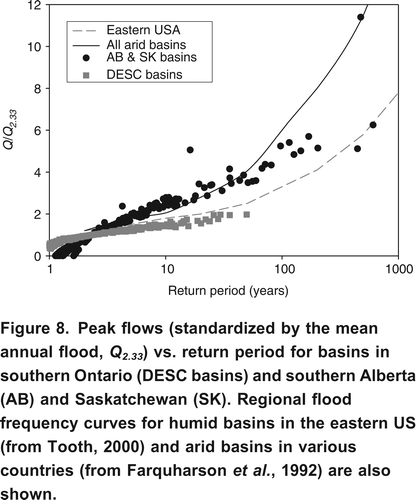
Geomorphology of Temporary Streams
The episodic nature of streamflow in temporary streams has important implications for geomorphic properties and processes. A key distinction between temporary and perennial streams is that process and form are not always closely linked in the former as they are assumed to be in the latter (Sandercock and Hooke, Citation2010), since the fluvial process-form system in temporary streams is often markedly discontinuous in both time and space (Tooth, Citation2000). The skewed flood frequency curves for temporary rivers noted earlier suggests that geomorphologically-effective floods are likely to be of relatively large magnitude, and repair of flood damage by lower flows in the periods between large floods is relatively limited (Tooth, Citation2000). The example of Graburn Creek in the Cypress Hills of southeast Alberta suggests the effects of large floods may persist for long periods of time. Thus, channel forms may represent the effects of the last major flood rather than an equilibrium between form and current processes, as is generally assumed to be the case in perennial streams (McPherson and Rannie, Citation1969; Sauchyn, Citation1993). This disequilibrium between process and form can manifest itself in the channel form of temporary streams, the associated hydraulics and bedforms, and aspects of sediment transport. It also may invalidate the paleohydrologic reconstruction of flow conditions in temporary streams using hydro-morphological relationships; for example, those between mean annual discharge and channel bankfull channel width (e.g., Sidorchuk et al., Citation2001) that were derived for perennial streams.
Channel Form
There is disagreement regarding differences in channel hydraulic geometry between perennial and temporary streams. Leopold and Miller (Citation1956) noted that ephemeral stream channels in New Mexico demonstrate the same basic hydraulic geometry relationships between width, depth and velocity and discharge and stream order as perennial streams. However, these relationships were originally derived for basins in which discharge generally increases with basin area, and thus may not apply for temporary rivers that experience significant transmission losses (Tooth, Citation2000). They may also be inapplicable to channels in permafrost environments, where channel form may be driven in part by variations in active layer thickness. In mountainous sub-arctic and arctic regions, stream cross-sections may be more box-shaped as a function of the stream freezing to the bed and creating a convex ice surface that directs water to the channel banks, causing thermoerosion and block collapse (Walker, Citation1975; Priesnitz, Citation1990).
Dunkerley and Brown (Citation1999) argued that, as with perennial flow channels, bankfull flow in temporary streams has the greatest capacity to transform the channel geometry given the reduced significance of transmission losses relative to flows below and above bankfull stage. Transmission losses also appear to influence a stream's longitudinal profile, which tends to be concave upward in perennial drainage systems (Gregory and Walling, Citation1973). Modelling studies suggest that the longitudinal profiles of ephemeral streams will be concave downward when there is discharge loss through the channel, and concave upward when the channel is gaining water in that reach from tributary contributions (e.g., Renard and Laursen, Citation1975). Field observations in the Cypress Hills confirm that seepage erosion during saturation overland flow creates upward concavities in the longitudinal profile of headwater channels (Spence and Sauchyn, Citation1999).
Hydraulics and Bedforms
Tooth (Citation2000) noted that the flow hydraulics and roughness coefficients in some dryland channels are strongly influenced by vegetation growing on the normally dry channel beds, which exploits moisture held in subsurface sediments. This vegetation can significantly reduce flow velocities and stream power, thus promoting sedimentation (Sandercock and Hooke, Citation2010). Roughness provided by vegetation on and adjacent to the stream may increase with stream stage in channels where there is a transition from grasses to trees with distance up the channel bank (Tooth, Citation2000), which may be particularly important for headwater channels in forest landscapes in Canada. The extreme and rapid variations in flood regime in temporary streams noted earlier mean that they rarely reach process-form equilibrium (Levick et al., Citation2008), i.e., flow conditions may change too rapidly for bedforms to develop a form that matches that flow. Thus, sedimentary structures can give a misleading picture of the prevailing flow conditions in temporary streams (North, Citation2005).
Sediment Transport
Sediment delivery from hillslopes to streams in sub-humid and humid forest landscapes is likely similar whether the receiving stream is temporary or perennial. However, in semi-arid landscapes there may be a pronounced increase in sediment transport in temporary streams due to reduced or completely absent vegetation cover on basin slopes. Bryan and Campbell (Citation1986) examined a semi-arid ephemeral tributary of the Red Deer River in Dinosaur Provincial Park, southeastern Alberta. Unlike in forest landscapes, Horton overland flow was the major runoff-generating process, supplemented by both shallow surface and deeper pipe flow. Suspended sediment concentrations in the ephemeral stream were typically lower on the hydrograph's falling limb due to between-storm preparation of material exposed on barren slopes and in channels, as well as sediment flushing on the hydrograph's rising limb followed by progressive armouring of hillslope and channel surfaces. Bryan and Campbell (Citation1986) also noted occasional increases in sediment concentrations during multi-peaked hydrographs, which appeared to reflect erosion of swelling clay surfaces as they approach saturation plus increasing contributions of sediment-rich subsurface flow.
Similar hysteretic relationships between discharge and suspended sediment occur in Canadian arctic streams. The role of the volume of spring meltwater in sediment erosion, transport and yield is well established (Forbes and Lamoureux, Citation2005), and three general phases of sediment transport in temporary arctic streams have been identified. The initial stages of the freshet are characterized by water flowing on or through snow and ice choked channels, negating the possibility of sediment transport. As runoff temperatures and volumes rise, thermal and kinetic erosion can begin to entrain sediment. Sediment transport is maximized when the sum of kinetic and thermal energy is at its greatest and there is access to the streambed. This occurs either during peak runoff (Cockburn and Lamoureux, Citation2008) or immediately afterwards (Priesnitz, Citation1990).
There may also be pronounced differences between perennial and temporary streams in terms of sediment transport within the stream channel. Leopold and Miller (Citation1956) noted that, unlike in perennial streams, sediment concentration increased with distance downstream in ephemeral streams in New Mexico. This was due either to a gradual and progressive entrainment of sediment from the streambed or to transmission losses; these increases in suspended sediment concentrations with flow distance in temporary streams may have important implications for water quality and aquatic ecology. Reid and Laronne (Citation1995) examined bedload sediment flux between three types of streams, and found that the flux in ephemeral streams was a function of stream power and reached higher levels than in perennial streams of similar power. They suggested that these differences are a product of the lack of armouring in ephemeral streams due to long no-flow periods and the absence of vegetation, and that flux rates are not supply limited. This agrees with Tooth's (Citation2000) contention that temporary river channels are largely transport- as opposed to source-limited, with the caveat of the aforementioned role of in-channel snow and ice in restricting sediment supply to arctic temporary streams.
Transmission losses and decreasing discharge with distance downstream noted earlier in some temporary streams promote stepwise movement, deposition and storage of sediment (Levick et al., Citation2008). This leads to a pulsating style of sediment transport, with sediment moving in spasmodic steps as a series of waves over various time periods ranging from hours to centuries (Tooth, Citation2000). Thus, temporary streams are highly effective in removing and storing suspended sediment and other particulate matter (e.g., plant fragments) from the water column, which can be subsequently released to downstream ecosystems during large storm events (Dieterich and Anderson, Citation1998).
Ecology of Temporary Streams
There is a paucity of studies in Canada on the hydroecology of temporary streams; nevertheless, results from other locations with similar biogeoclimatic conditions to those in parts of Canada may assist in understanding temporary stream ecology in this country. For example, in semi-arid regions such as Montana, where ephemeral and intermittent streams outnumber perennial streams, temporary streams are often critical refugia (i.e., groundwater-fed pools provide oases of moisture and specific stream temperature), breeding areas or food sources for wildlife, and harbour plant species that could not survive in surrounding uplands (Vance, Citation2009). Dodds (Citation1997) noted that small streams (which would include temporary streams) link terrestrial habitats across the landscape with downstream rivers, lakes, estuaries and oceans. Thus, they serve as temporal ecotones between terrestrial and aquatic ecosystems and contribute substantially to the overall biodiversity of these ecosystems (Boulton et al., Citation2010). Temporary streams may be connected to downstream waters only during episodic and short-lived flow pulses (Nadeau and Rains, Citation2007); nevertheless, they may act as important sources of water, sediment, nutrients, seeds, and organic matter for downstream systems as well as habitat for many species (Gomi et al., Citation2002). Temporary streams may also provide episodic connection and flow between the main stream channels and perched lakes, ponds and wetlands during high stage events in deltaic environments, such as the Peace-Athabasca Delta in northern Alberta (Peters et al., Citation2006).
In-stream processes exert a significant control on both the hydrology and hydrogeochemistry of streams. A key demonstration of this in temporary streams is the decrease in flow rates in a downstream direction due to transmission losses. This can promote in-channel silt and clay deposition that may benefit biotic communities, since finer sediments are often associated with higher levels of dissolved organic carbon (DOC), nitrogen (N) and phosphorus (P), and higher soil water contents (Levick et al., Citation2008). This creates and maintains microhabitat for a variety of organisms (Jacobson et al., Citation2000), and leads to more rapid nutrient uptake and removal in temporary headwater streams relative to larger downstream channels (Peterson et al., Citation2001). Thus, Fisher and Grimm (Citation1985) found that ephemeral streams in Arizona retained between 50 and 90% of the N and P supplied during moderate storm events.
Small alterations of the flow regime in temporary streams may lead to major changes in the extent of drying and rewetting, which in turn has major consequences for the biota and ecosystem processes in temporary stream systems. Doering et al. (Citation2007) provided important quantitative information about drying and rewetting cycling along a large gravel-bed river over a multi-year period. They noted that small alterations of the flow regime can lead to major changes in the duration, frequency, timing and extent of drying and rewetting, with probable severe consequences for terrestrial and aquatic communities and ecosystem processes. Biotic communities have evolved specific adaptive strategies to cope with alternating loticlenticdry conditions, such that increases in the length or severity of the dry season can pose lethal environmental stresses for key groups of biota both within the stream (Tzoraki et al., Citation2007) and in supported riparian habitats.
Soil moisture also plays a key ecological role in temporary streams. Bloss and Brotherson (Citation1979) demonstrate the relationship between ecological diversity and a soil moisture index in areas occupied by ephemeral streams. Temporary streams in Mediterranean regions are characterized by a stream hydrology with a marked seasonal pattern and high inter-annual variability (Bernal et al., Citation2004), which is mirrored in the patterns of other processes such as nitrogen fixation and leaching of solutes. This biological mediation between hydrology and nutrient availability in the floodplain soils of temporary stream systems was noted by Harms and Grimm (Citation2010), who highlighted the response of microbial processes in these soils to precipitation and discharge. Fritz and Dodds (Citation2005) developed a harshness index (based on variables describing the hydrologic regime: flow variability and drying, as well as distance to perennial surface water) to explain macroinvertebrate assemblage characteristics at intermittent and perennial sites in Kansas. They found that annual and historical hydrologic parameters appeared to account for assemblage characteristics to a greater extent than distance to the nearest permanent habitat.
Considering the importance of potential alterations to flow regimes associated with land-use change, water abstraction, and construction of reservoirs for irrigation and hydroelectric power generation, a key challenge for temporary streams is the development of ecological instream flow needs (IFN) recommendations to maintain the natural river ecosystem. IFN research and application in Canada and internationally has historically focused on the specific protection goal of maintaining minimum instream flows to sustain fish species of interest in perennial rivers (Arthington et al., Citation2006; Peters et al., Citation2011). However, it has recently become clear that all components of the river ecosystem need to be protected, many of which are strongly influenced by temporal variation in streamflow (Poff et al., Citation1997). In particular, flow intermittence is an extreme form of flow variation and one that has pervasive ecological effects (Larned et al., Citation2010).
An important distinction between temporary and perennial streams is that maintenance of both static water pools and hyporheic zone flow may be of ecological importance in the former, by supporting the survival of aquatic communities until the end of the dry season. However, this hydroecological characteristic is difficult to define in relation to IFN, partially due to our limited knowledge of the relationship between the two (Hughes, Citation2005). Thus, ecological flow determination for temporary rivers requires a holistic approach to ecosystem protection and possibly the development of new indices and metrics beyond those used in natural versus modified channel flow regime methods.
Monitoring and Data Analysis of Flows in Temporary Streams
Presence and Type of Temporary Streams
It is often important to identify the occurrence and type of temporary stream in a given landscape, since water quality protection measures adopted by management agencies are often related to stream type (Hansen, Citation2001; Imperial Oil Resources Ventures, Citation2004). As Svec et al. (Citation2005) noted: Generally, as flow duration increases the potential for non-point source pollution increases, and BMPs (Best Management Practices) intensify to protect water quality. Incorrectly determining stream class can lead to either increased non-point source pollution from increased activity where flow duration would dictate less disturbance, or costly deferral of revenue from the too stringent application of the BMPs (p. 171). Flow duration is often used as the primary descriptor of different stream types (); however, flow duration is seldom measured or verified (Hansen, Citation2001).
Table 2. Examples of the use of flow duration to define stream class (after Svec et al., 2005). As is common in hydrology, the place of study strongly influences the definition.
In the absence of this information, one could try and predict the presence and type of stream from maps; however, the blue line drainage network on topographic maps has long been known to under predict and misclassify the presence of temporary streams (Hansen, Citation2001). Hansen (Citation2001) found that the blue line network represented ~75% of field-assessed perennial stream network at 1:24 000 scale, and only 50% at 1:100 000 scale; no temporary streams were mapped at either scale. The presence of temporary streams can be estimated from contour crenulations; however, the method can be highly subjective (Marks, Citation1983) and it is often difficult to map headwater channels in areas of high drainage density from air photo analysis, particularly in forested landscapes (Hansen, Citation2001). Hansen (Citation2001) described criteria that could be employed in a field approach to estimating stream type (). He recognized that adjustments in stream type criteria may be needed for terrain and climactic conditions, especially when conditions: (1) are relatively flat, (2) lack soil, (3) are dominated by rock, boulders, or bedrock materials, (4) have little interaction with surface features, (5) have extremely erosive soils that respond to any concentrated flow, (6) develop in semi-arid and arid lands with little or no plant cover, (7) are dominated by frozen terrain, or (8) have recent extreme flow events. At least one of these eight traits can be used to describe much of the Canadian land mass.
Table 3. Field criteria for determining stream type (Hansen, Citation2001). These criteria were originally developed for southern Appalachia, USA.
Monitoring Flow Conditions in Temporary Streams
Temporary rivers do not lend themselves well to classical hydrometric techniques used to monitor flows in perennial streams. Spatial and temporal patterns of runoff are rarely monitored for temporary streams, and flashy streamflows coupled with mobile sediments create impediments to measuring and monitoring water flux (Constanz et al., Citation2001; Hooke, Citation2007). A further problem is posed by the potentially large fraction of total flow that moves through rather than over the streambed. Although daily mean discharges are commonly provided for stream gauges on perennial streams in Canada and elsewhere, these data are not particularly relevant in streams where most days have zero flow or where streamflow may rise and fall rapidly in response to intense but short-lived rainfall inputs (Camarasa and Tilford, Citation2002). Other measures of streamflow that may be more relevant include the temporal and spatial occurrence of days with flow, instantaneous peak flows, channel losses, and duration of flow periods.
Monitoring of temporary streams requires alternative approaches to those applicable to perennial streams. Differential stream gauging along a reach can be used to estimate transmission losses (Cataldo et al., Citation2004), although the expense of installing and maintaining a series of gauging stations has encouraged more economical approaches to studying the hydrology of temporary streams. For example, crest stage recorders can be used to determine maximum water depths experienced in the channel between site visits (Hooke, Citation2007; Sandercock and Hooke, Citation2010); however, they do not indicate the timing of the peak stage and do not record multiple flows (Hooke, Citation2007).
Continuous monitoring of streambed temperature is a robust and inexpensive means of detecting streamflow frequency and duration in temporary streams, but requires correction for changing atmospheric conditions (Constanz et al., Citation2001). Ronan et al. (Citation1998) used temperature sensors to investigate flow below an ephemeral stream and estimate recharge rates. Constanz et al. (Citation2002) also employed temperature profiles to provide continuous estimates of ephemeral streamflow, where the episodic nature of the stream results in shifting channel morphology and degradation of the rating curves of hydrometric stations. Using heat as a tracer of surface water and groundwater exchanges and streambed percolation rates, they compared temperature-based results with surface water measurements and found that percolation losses were about 3050% of the channel loss.
Such temperature profiles provide a continuous record of conditions within the channel; nevertheless, they represent point estimates and do not provide information regarding flow volume, while the precision of the recharge estimates derived from temperature profiles in the streambed is only to an order of magnitude. However, limitations of point-scale monitoring of stream temperatures can be overcome by recent advances in the use of fibre optic temperature sensing (Selker et al., Citation2006; Vogt et al., Citation2010), which enable continuous monitoring of vertical and long profile stream temperatures in space and time. Resistivity sensors can also be used to detect water presence in the stream channel; Bhamjee and Lindsay (Citation2010) presented an improved sensor that can withstand some of the environmental challenges posed by temporary streams, such as high sediment transport and deposition. The devices were only able to detect wet and dry, rather than flow and non-flow, states; nevertheless, the method appears to reduce noise in the data as well as the subjectivity associated with interpreting events.
Monitoring Sediment Transport in Temporary Streams
Standard approaches, such as bedload traps and automatic water samplers, can be used to determine bedload transport and suspended sediment concentrations in temporary streamflow. However, the periodic absence of flow in temporary streams means that such channels lend themselves well to the use of repeated detailed ground surveys of channel reaches, which allow estimates of erosion/deposition and sediment budgets to be obtained (Martin and Church, Citation1995; Hooke, Citation2007).
Analysis of Data from Temporary Streams
The scarcity of events and general paucity of data for temporary streams are problematic. Kisiel et al. (Citation1971) characterized ephemeral flow in arid lands as alternating random periods of wetness and dryness traits that likely apply to most temporary streams. Thus, the study of temporary streamflows involves examination of several random variables, including: (1) flow duration; (2) timing within the wet season; (3) antecedent dry period; (4) magnitude (flow volumes); and (5) time of travel of flood waves within the stream network. In addition, transmission losses combined with the localized nature of convective storms (Tooth, Citation2000; Hooke, Citation2007) that are often responsible for generating flows in temporary stream channels (particularly during the summer in Canada), or the limited duration of the snowmelt period which supplies water to temporary streams, mean that these streamflow characteristics vary in space within a given basin. Flow duration curves (FDCs) provide a useful means of characterizing the frequency distribution of a stream's flow regime (Crocker et al., Citation2003); however, they can only be obtained at gauging stations and are complicated by periods of no flow, such as occur in temporary streams. In response, Crocker et al. (Citation2003) used the theory of total probability to obtain complete FDCs for temporary streams. This approach combines a model for predicting the percentage of time with zero flow with a predicted FDC for the non-zero flow period.
Modelling of Flows in Temporary Streams
There is little existing literature regarding modelling of temporary streamflows in Canada. Such modelling has application in the prediction of streamflow response to given water inputs, in the restoration of partial or seasonal flow records, and in assessing the potential impacts on temporary streams of water management decisions as well as changes in climate and land use. Modelling is an essential approach to addressing temporary streams in Canada where monitoring data and studies are generally scant. In this section, the distinction is made between statistical, stochastic, deterministic and combined models for predicting temporary streamflow and related flow properties.
Statistical Models
A general example of this type of model is the regression of streamflow characteristics against such factors as P input and drainage basin properties. Regression equations for temporary streams involve highly non-linear relationships between rainfall and runoff, and there are relatively few available data for deriving and testing these relationships relative to perennial streams. Cataldo et al. (Citation2004) reviewed simple regression equations for predicting transmission losses (or outflow volume) as a function of such covariates as upstream flow volume, channel antecedent condition, channel slope, channel bed material, flow duration and active channel width. They noted that while such models are straightforward to implement, they lack direct connection to the specific physical processes governing transmission losses, making them difficult to apply at sites other than the one(s) for which they were developed.
Stochastic Models
These models lend themselves well to the stochastic nature of rainfall that may generate streamflow and the resulting streamflow response; there are many examples in the literature of their use in simulating temporary streamflow. Lee (Citation1975) synthesised monthly ephemeral streamflow in arid lands using a Weibull-based approach to describe intermittent and ephemeral streamflow events in terms of streamflow duration, inter-event time and total streamflow volume. Srikanthan and McMahon (Citation1980) tested six procedures for generating monthly flows in ephemeral streams. The major problem they encountered was modelling of zero flows; this could be addressed by the method of fragments, although other procedures also yielded acceptable results. Chebaane et al. (Citation1995) presented a stochastic model capable of handling the high percentage of zero flows in intermittent streams, reproducing the percentage of zero flows in each month, the monthly mean and variance, and the month to month correlation of the intermittent flows.
Aksoy and Bayazit (Citation2000a) provided a stochastic model for generating synthetic daily flows for intermittent streams. It considers four determinations: (1) days on which flow occurs; (2) days on which a flow increment occurs (estimated using a three-state Markov Chain); (3) magnitude of the increment by assuming a gamma distribution; and (4) the magnitude of the flow decrement when flow is reduced, using a two parameter recession coefficient. Model parameters were estimated from observed daily streamflow records and the model preserved short-term characteristics (hydrograph shape and peak) in addition to the long-term attributes (mean, variance, lag-one and higher autocorrelation functions, and zero flow percentage). They then used the model to generate daily streamflows for intermittent streams (Aksoy and Bayazit, Citation2000b). Model parameters were determined monthly and the model preserved both short and long term characteristics of a 35 year streamflow record.
Wang and Robertson (Citation2011) developed a Bayesian joint probability modelling approach for forecasting flows in temporary streams by treating zero flow occurrences as censored data. Claps et al. (Citation1993) provided a multivariate approach autoregressive moving average (ARMA) model and a multi-station conceptual ARMA using spatial correlation that is suitable for discontinuous time series such as temporary streamflows. Cigizoglu et al. (Citation2002) characterized streamflow series in arid and semi-arid regions as having flow episodes separated by period of zero discharge. They used two point process models, one to model the excess clustering of flow events and the second to model flow properties within clusters of events.
Deterministic Models
Deterministic models are those that incorporate algorithms representing the key physical processes that control streamflow generation and movement in the drainage basin. These algorithms generally contain parameters that must either be measured directly or estimated via calibration against the observed hydrologic behaviour of the system being modelled. Ye et al. (Citation1997) used three such models (GSFB with eight parameters, IHACRES with six parameters, and LASCAM with 22 parameters) to model runoff in three catchments where runoff is 212% of rainfall. Because of the role of antecedent conditions, they felt that this provided a stern test of the deterministic models, which explained more than 90% of the variance in observed streamflow in independent periods at a monthly time scale. More complex models performed better in these dry catchments at a daily time scale. Ye et al. (Citation1997) identified a clear need to account for deep groundwater and antecedent moisture conditions when simulating flow in these temporary streams.
An alternative approach was that of Camarasa and Tilford (Citation2002), who simulated streamflow in ephemeral streams using a transfer function. The approach worked best in basins where Horton overland flow was the dominant runoff process, such that rainfall characteristics had a greater influence on the streamflow hydrograph than basin physiography. There are several Canadian physically-based deterministic models (see Davison and van der Kamp (Citation2008) for a brief review), including the Cold Regions Hydrological Model (Pomeroy et al., Citation2007) and Watflood (Kouwen et al., Citation1993), which incorporate the hydrological processes discussed earlier that could lead to intermittency. However, few examples of applications of these models to simulate temporary flow exist. Some hydrologists debate whether these semi-distributed models in their present form are capable of properly simulating low flow and intermittent flow behaviour (Smerdon et al., Citation2005; Davison and van der Kamp, Citation2008), but progress is being made with successful applications in the Canadian Rockies (DeBeer and Pomeroy, Citation2010).
Combined Models
These are a combination of different model types outlined previously, such as a linkage of deterministic and stochastic models. For example, Shamir et al. (Citation2007) modelled ephemeral streamflow by combining stochastic simulation of the hourly P point process and a deterministic model that transforms P into flow. The model was constructed for four seasons and for different types of interannual variability, and reproduced the variability in observed streamflow at daily, seasonal and annual time scales. Cataldo et al. (Citation2004) emphasized the value of the combined use of differential equations and regression to predict transmission losses in temporary stream reaches. This allows the modeller to consider physical processes related to transmission losses and to use regression or optimization to develop site-specific equations. The advantage of this approach over simplified differential equations is that regression provides estimates of model parameters for site-specific predictions. Cataldo et al. (Citation2004) argued that this modelling approach has the greatest promise in predicting transmission losses in temporary streams.
The Role of Anthropogenic Activities and Climate Change in Modifying Temporary Stream Hydrology
Anthropogenic Impacts
Bull (Citation1997) noted that ephemeral streams may be more sensitive than perennial streams to anthropogenic or climate disturbance. These disturbances can arise from a variety of human activities, one of the most dramatic being urbanization. This land use change includes urban, suburban and exurban development, which have the potential to change significantly the hydrologic characteristics of a drainage basin via covering uplands with impervious surfaces, and removing, channelizing or armouring small or headwater streams (Levick et al., Citation2008). Expansion of artificial surfaces and rapid conveyance of stormflow from urban areas via artificial drainageways that function as temporary streams may also reduce groundwater recharge to such an extent that previously perennial streams may become temporary. Conversely, unintended water delivery into urban drainage systems, such as via groundwater inflows and leakage from water supply networks (Welty et al., Citation2007), can transform temporary urban streams into perennial systems. These changes can impair or destroy natural flow patterns and sediment transport functions, while concurrently promoting downstream flooding and alterations to downstream water chemistry, with ecological as well as water-supply implications (Levick et al., Citation2008).
Agricultural activities also alter the hydrology and hydroecology of temporary streams (EPA South Australia, Citation2010) through such effects as:
• | Increased salinity caused by clearing of native vegetation which raises the groundwater reservoir; | ||||
• | Reduced flows from groundwater pumping or stream diversions for irrigation; | ||||
• | Increased nutrients and turbidity from the use of fertilizers that run off into streams across the land surface or through the soil, causing excessive algae growth; | ||||
• | Fish, aquatic invertebrate and bird kills from pesticides that run off into the streams or leach into the groundwater. |
In addition to these impacts, livestock often depend on temporary streams for their water supply, particularly in semi-arid areas. Improper management of livestock in the riparian zones and channels of temporary streams may lead to soil compaction, trampling of stream banks, vegetation removal and contamination of stream water by wastes (Levick et al., Citation2008).
Human activities, such as mining and water supply management, lead to the abstraction of water from or release to temporary streams. Water abstraction may turn a perennial stream into an intermittent or ephemeral stream, while the opposite may occur following water diversion and introduction into a stream channel, or upstream impoundment of streamflow and subsequent release. As with perennial streams, dams and retention or detention basins impact temporary stream hydrology by disrupting natural surface flow and sediment transport, interfering with natural geomorphic processes, altering water temperatures, and severing hydrologic and ecologic connectivity in the landscape (Levick et al., Citation2008).
Temporary streams may also serve as conduits for effluent from storm drains in urban and suburban areas as well as from waste water treatment plants (Levick et al., Citation2008). The addition of waste water may shift a dry channel with occasional floods to a stream with a continuous flow pattern similar to that of humid areas (Hassan and Egozi, Citation2001). For example, treated sewage effluent from Regina, Saskatchewan comprises almost 100% of streamflow in Wascana Creek, a snowmelt-dominated temporary stream system. The stream's aquatic ecology may be severely impacted by the nutrients, pharmaceuticals and personal care products carried by this effluent (Waiser et al., Citation2011a; Citation2011b).
Less obvious is the role of groundwater abstraction, which may cause streamflow to decline and thus transform a perennial stream to an intermittent or even ephemeral stream (Winter, Citation2007; Constantz and Essaid, Citation2007). Sophocleous (Citation2000) noted the effect of groundwater withdrawal from the Ogallala aquifer in the US mid-west on changes in the extent of flowing stream reaches in western Kansas. Groundwater abstraction in the Santa Cruz River of southern Arizona led to perennially flowing stream reaches becoming intermittent or ephemeral (Glennon and Maddock, Citation1994). The reduction in water table levels that accompanies groundwater abstraction can kill riparian vegetation whose roots no longer reach the groundwater reservoir (Rood and Mahoney, Citation2000), leading to a loss of bank stability and increased bank erosion (Levick et al., Citation2008).
Climate Change
The hydrology of temporary rivers may be substantially altered as a consequence of climate change and the associated modification of P and air temperature; temporary rivers are expected to increase in importance under a warmer climate. Schindler (Citation2001) reviewed the potential impacts of climate change on North American freshwater systems and suggested that first-order streams may become ephemeral or may remain dry for longer periods due to increased evapotranspiration. More recently, Brooks (Citation2009) concluded that in addition to reduced hydroperiods and more flashy flow patterns, static pools would be increasingly isolated for longer periods and reduced in volume in forested watersheds of the northeastern USA. These alterations appear likely in various regions across Canada.
Jones and Rinehart (Citation2010) suggested that climatic warming in permafrost areas may reduce permafrost extent and increase basin storage; thus, summer P may be retained and not contribute to streamflow, leading to ephemeral flow conditions. However, streamflow from permafrost-dominated basins is highly responsive to P inputs (Newbury, Citation1974), and it can be hypothesized that some basins currently exhibiting zero flows due to rapid drainage of slopes with thin active layers may experience sustained summer flows as a result of the ability of the deeper active layer to store and release water to the stream.
The key climate shifts that will affect temporary streams in the Cordillera will likely relate to changes in snow accumulation and snowmelt rates. Hauer et al. (Citation1997) argued that climate change in the Rocky Mountains may result in streams becoming intermittent. Changes in winter snow accumulation or spring snowmelt rates would directly affect the hydrological regime of mountain streams, whether they are snowmelt fed (e.g., permanent streams becoming ephemeral with a decrease in snowpack) or glacial fed (e.g., rapid glacier ablation and disappearance). Changes in temperature are likely to affect temporary stream ecosystems in montane landscapes through a broad influence upon P phase (i.e., snow vs. rain), snowmelt rate, biogeochemical flux rates and the rate of the metabolic functions of biota.
Covich et al. (Citation1997) reported that the low topographic complexity but high hydrological diversity in the Great Plains of the US (and by extension the southern Prairie Provinces) mean that the area is very responsive to climate variability and change. There are many temporary streams in this landscape, and reductions in P amounts and increases in ET may shift perennial streams to intermittent streams and intermittent streams to ephemeral streams. Anticipated changes in the frequency of extreme P events (Kharin and Zwiers, Citation2005) may increase the number of annual streamflow responses from episodic streams such that they begin to behave as ephemeral ones.
Conclusions
Temporary streams are key elements of what Bishop et al. (Citation2008) termed Aqua Incognita the unknown headwaters. However, simply because these streams are often not on the map does not mean that they should not be on our radar. Much of our understanding of the hydrology, geomorphology and ecology of temporary streams comes from work done in semi-arid and arid landscapes in other countries. These study results may apply to temporary streams in semi-arid parts of southern Alberta, Saskatchewan and British Columbia; nevertheless, this must be confirmed through further research. The streams in these regions face similar pressures (e.g., groundwater abstraction, climate change) to those occurring in arid and semi-arid landscapes such as the US southwest, and approaches to monitoring and ameliorating the consequences of these impacts that have been used in other countries may also be applicable to temporary streams in semi-arid areas of Canada. Nevertheless, there are aspects of temporary stream hydrology that are relatively unique to Canada, such as the role of permafrost in controlling the responsiveness of streams to water inputs and to water release from storage. Given the extent of Canada mantled by continuous and discontinuous permafrost, it is particularly important to understand how climate change will affect the hydrology of both perennial and temporary streams in these regions.
In addition to improving our understanding of temporary stream hydrology in Canada's distinct landscapes, there is a continuing need for:
• | Confirming whether process literature from other areas is applicable in Canada. This review has relied extensively on literature from other places as Canadian sources are lacking. Since temporary rivers are highly sensitive to land use and climate, we should determine the validity of applying the results of such studies to Canadian temporary streams. | ||||
• | Adapting temporary stream classification criteria from other countries to the Canadian context. | ||||
• | Enhancing our ability to predict the location and type of temporary streams. | ||||
• | Improving the monitoring of flow in such transient flow systems. | ||||
• | Exploring promising advances in techniques for detecting the timing and location of temporary streamflow, such as automatic monitoring of stream temperature. | ||||
• | Improving our ability to model temporary streamflow, particularly where controls involve snowmelt and/or permafrost. This should include both deterministic and statistical models. | ||||
• | Improving our ability to measure and model processes such as transmission losses in temporary streams, specifically by better understanding the controls on these losses in different landscapes. This will require more explicit consideration of the controls on the occurrence of temporary streams and of the manner of streamflow generation in the particular basin of interest, whether it is the Variable Source Area (VSA) or the Element Threshold concept. | ||||
• | Framing studies of temporary stream behaviour in the context of these (and other) conceptual models. This may assist in explaining differences in the flow properties of temporary streams in different landscapes, as well as the associated geomorphic and ecologic characteristics of such stream systems. |
Temporary streams are critical but highly vulnerable hydrological and hydroecological systems that are often the first parts of the drainage network to respond to many natural and anthropogenic impacts. They are vital but relatively poorly understood components of the biological integrity of whole drainage basins, such that their degradation and loss of connection to larger streams can have profound localized as well as downstream consequences. They deserve greater attention from the Canadian hydrological community than they have received to date.
Acknowledgements
JMB acknowledges support from the Natural Sciences and Engineering Research Council of Canada. Thanks to Greg MacCulloch (Water Survey of Canada) for providing streamflow data used to construct , and to two anonymous reviewers for their constructive comments on an earlier version of this paper.
References
- Allan , C. and Roulet , N. 1994 . Runoff generation in zero order Precambrian Shield catchments: The stormflow response of a heterogeneous landscape . Hydrological Processes , 8 : 369 – 388 .
- Aksoy , H. and Bayazit , M. 2000a . A model for daily flows of intermittent streams . Hydrological Processes , 14 : 1725 – 1744 .
- Aksoy , H. and Bayazit , M. 2000b . A daily intermittent streamflow simulator . Turkish Journal of Engineering , 24 : 265 – 276 .
- Allaby, A., and M. Allaby. 1999. A dictionary of earth sciences, 2nd Edition. Oxford, UK: Oxford University Press, 619 pp.
- Arthington , A. H. , Bunn , S. E. , Poff , N. L. and Naiman , R. J. 2006 . The challenge of providing environmental flow rules to sustain river ecosystems . Ecological Applications , 16 : 1311 – 1318 .
- Bedient, P. B., W. C. Huber, and B. E. Vieux. 2008. Hydrology and floodplain analysis, 4th Edition. Upper Saddle River, NJ: Prentice Hall, 795 pp.
- Bernal , S. , Butterini , A. , Riera , J. L. , Vazquez , E. and Sabater , F. 2004 . Calibration of the INCA model in a Mediterranean forested catchment: The effect of hydrological inter-annual variability in an intermittent stream . Hydrology and Earth System Sciences , 8 : 729 – 741 .
- Bhamjee , R. and Lindsay , J. B. 2010 . Ephemeral stream sensor design using state loggers . Hydrology and Earth Systems Science Discussions , 7 : 6381 – 6405 .
- Bishop , K. , Buffam , I. , Erlandsson , M. , Flster , J. , Laudon , H. , Seibert , J. and Temnerud , J. 2008 . Aqua Incognita: The unknown headwaters . Hydrological Processes , 22 : 1239 – 1242 .
- Bloss , D. A. and Brotherson , J. D. 1979 . Vegetation response to a moisture gradient on an ephemeral stream in central Arizona . Great Basin Naturalist , 39 : 161 – 176 .
- Boulton , A. J. , Thibault Datry , T. , Kasahara , T. , Mutz , M. and Stanford , J. A. 2010 . Ecology and management of the hyporheic zone: Streamgroundwater interactions of running waters and their floodplains . Journal of the North American Benthological Society , 29 : 26 – 40 .
- Branfireun , B. and Roulet , N. 1998 . The baseflow and storm flow hydrology of a Precambrian Shield headwater peatland . Hydrological Processes , 12 : 57 – 72 .
- Brook , G. A. and Ford , D. C. 1980 . Hydrology of the Nahanni karst, northern Canada, and the importance of extreme summer storms . Journal of Hydrology , 46 : 103 – 121 .
- Brooks , R. T. 2009 . Potential impacts of global climate change on the hydrology and ecology of ephemeral freshwater systems of the forests of the northeastern United States . Climatic Change , 95 : 469 – 483 .
- Brown, R. J. E. 1970. Permafrost in Canada its influence on northern development. Toronto, ON: University of Toronto Press, 234 pp.
- Brutsaert, W. 2005. Hydrology: An introduction. Cambridge, UK: Cambridge University Press, 605 pp.
- Bryan , R. B. and Campbell , I. A. 1986 . Runoff and sediment discharge in a semiarid ephemeral drainage basin . Zeitschrift fr Geomorphologie Supplementbnde , 58 : 121 – 143 .
- Bull , W. B. 1997 . Discontinuous ephemeral streams . Geomorphology , 19 : 227 – 276 .
- Buttle , J. M. 2006 . Mapping first-order controls on streamflow from drainage basins: The T3 template . Hydrological Processes , 20 : 3415 – 3422 .
- Buttle , J. M. and Eimers , M. C. 2009 . Scaling and physiographic controls on streamflow behaviour on the Precambrian Shield, south-central Ontario . Journal of Hydrology , 374 : 360 – 372 .
- Buttle , J. M. , Dillon , P. J. and Eerkes , G. R. 2004 . Hydrologic coupling of slopes, riparian zones and streams: An example from the Canadian Shield . Journal of Hydrology , 287 : 161 – 177 .
- Camarasa , A. M. and Tilford , K. A. 2002 . Rainfall-runoff modelling of ephemeral streams in the Valencia region (Eastern Spain) . Hydrological Processes , 16 : 3329 – 3344 .
- Carey , S. K. and Woo , M.-K. 2001 . Slope runoff processes and flow generation in a subarctic, subalpine catchment . Journal of Hydrology , 253 : 110 – 129 .
- Cataldo, J., C. Behr, F. Montalto, and R. J. Pierce. 2004. A summary of published reports of transmission losses in ephemeral streams in the U.S. A Report to the National Center for Housing and the Environment. Poolesville, MD: Wetland Science Applications Inc., 42 pp.
- Cataldo , J. , Behr , C. , Montalto , F. and Pierce , R. J. 2010 . Prediction of transmission losses in ephemeral streams, western U.S.A . The Open Hydrology Journal , 4 : 19 – 34 .
- Chebaane , M. , Salas , J. D. and Boes , D. C. 1995 . Product periodic autoregressive processes for modeling intermittent monthly streamflows . Water Resources Research , 31 : 1513 – 1518 .
- Cigizoglu , H. K. , Adamson , P. T. and Metcalfe , A. V. 2002 . Bivariate stochastic modelling of ephemeral streamflow . Hydrological Processes , 16 : 1451 – 1465 .
- Claps , P. , Rossi , F. and Vitale , C. 1993 . Conceptual-stochastic modeling of seasonal runoff using autoregressive moving average models and different scales of aggregation . Water Resources Research , 29 : 2545 – 2559 .
- Cockburn , J. M. H. and Lamoureux , S. F. 2008 . Hydroclimate controls over seasonal sediment yield in two adjacent High Arctic watersheds . Hydrological Processes , 22 : 2013 – 2027 .
- Constantz , J. and Essaid , H. 2007 . Influence of groundwater pumping on streamflow restoration following upstream dam removal . Hydrological Processes , 21 : 2823 – 2834 .
- Constanz , J. , Stonestrom , D. , Stewart , A. E. , Niswonger , R. and Smith , T. R. 2001 . Analysis of streambed temperatures in ephemeral channels to determine streamflow frequency and duration . Water Resources Research , 37 : 317 – 328 .
- Constanz , J. , Stewart , A. E. , Niswonger , R. and Sarma , L. 2002 . Analysis of temperature profiles for investigating stream losses beneath ephemeral channels . Water Resources Research , 38 : 52 – 65 .
- Covich , A. P. , Fritz , S. C. , Lamb , P. J. , Marzolf , R. D. , Matthews , W. J. , Poiani , K. A. , Prepas , E. E. , Richman , M. B. and Winter , T. C. 1997 . Potential effects of climate change on aquatic ecosystems of the Great Plains of North America . Hydrological Processes , 11 : 993 – 1021 .
- Crocker , K. M. , Young , A. R. , Zaidman , M. D. and Rees , H. G. 2003 . Flow duration curve estimation in ephemeral catchments in Portugal . Hydrological Sciences Journal , 48 : 427 – 439 .
- Davison , B. and van der Kamp , G. 2008 . Low-flow in deterministic modelling: A brief review . Canadian Water Resources Journal , 33 : 191 – 194 .
- DeBeer , C. M. and Pomeroy , J. W. 2010 . Simulation of the snowmelt runoff contributing area in a small alpine basin . Hydrology and Earth Systems Science , 14 : 1205 – 1219 .
- De Boer , D. H. 1992 . Constraints on spatial transference of rainfall-runoff relationships in semiarid basins drained by ephemeral streams . Hydrological Sciences Journal , 37 : 491 – 504 .
- Devito , K. J. , Hill , A. R. and Roulet , N. T. 1996 . Groundwater surface water interactions in headwater forested wetlands of the Canadian Shield . Journal of Hydrology , 181 : 127 – 141 .
- Dieterich , M. and Anderson , N. H. 1998 . Dynamics of abiotic parameters, solute removal and sediment retention in summer-dry headwater streams of western Oregon . Hydrobiologia , 379 : 1 – 15 .
- Dingman, S. L. 1993. Physical hydrology. Englewood Cliffs, NJ: Prentice Hall, 575 pp.
- Dodds , W. K. 1997 . Distribution of runoff and rivers related to vegetative characteristics, latitude, and slope: A global perspective . Journal of the North American Benthological Society , 16 : 162 – 168 .
- Doering , M. , Uehlinger , U. , Rotach , A. , Schlaepfer , D. R. and Tockner , K. 2007 . Ecosystem expansion and contraction dynamics along a large Alpine alluvial corridor (Tagliamento River, Northeast Italy) . Earth Surface Processes and Landforms , 32 : 1693 – 1704 .
- Dunkerley , D. and Brown , K. 1999 . Flow behavior, suspended sediment transport and transmission losses in a small (sub-bank-full) flow event in an Australian desert stream . Hydrological Processes , 13 : 1577 – 1588 .
- Dunne, T. 1978. Field studies of hillslope flow processes. In Hillslope hydrology, ed. M. J. Kirkby, 227293. Chichester, UK: Wiley.
- EPA South Australia. 2010. Water quality major threats & issues. http://www.epa.sa.gov.au/environmental_info/water_quality/major_threats_and_issues (accessed February 9, 2011).http://www.epa.sa.gov.au/environmental_info/water_quality/major_threats_and_issues
- Ehsanzadeh , E. , Spence , C. and van der Kamp , G. 2011 . On the behaviour of dynamic contributing areas and flood frequency curves in North American Prairie watersheds . Journal of Hydrology , 414415 : 364 – 373 .
- Fang , X. , Pomeroy , J. W. , Westbrook , C. J. , Guo , X. , Minke , A. G. and Brown , T. 2010 . Prediction of snowmelt derived streamflow in a wetland dominated prairie basin . Hydrology and Earth Systems Science , 14 : 991 – 1006 .
- Farquharson , F. A. K. , Meigh , J. R. and Sutcliffe , J. V. 1992 . Regional flood frequency analysis in arid and semi-arid areas . Journal of Hydrology , 138 : 487 – 501 .
- Fisher , S. G. and Grimm , N. B. 1985 . Hydrologic and material budgets for a small Sonoran Desert watershed during three consecutive cloudburst floods . Journal of Arid Environments , 9 : 105 – 118 .
- Forbes , A. C. and Lamoureux , S. F. 2005 . Climatic controls on streamflow and suspended sediment transport in three large middle arctic catchments, Boothia Peninsula, Nunavut, Canada . Arctic, Antarctic and Alpine Research , 37 : 305 – 315 .
- Ford, D., and P. Williams. 2007. Karst hydrogeology and geomorphology. Chichester, UK: Wiley, 562 pp.
- Fritz , K. M. and Dodds , W. K. 2005 . Harshness: Characterisation of intermittent stream habitat over space and time . Marine and Freshwater Research , 56 : 13 – 23 .
- George , S. E. 2007 . Structural controls on epikarst and surface water drainages in the Ozarks . 2007 National Cave and Karst Management Symposium , : 168 – 174 .
- Glennon , R. J. and Maddock III , T. 1994 . In search of subflow: Arizona's futile effort to separate groundwater from surface water . Arizona Law Review , 36 : 567 – 610 .
- Gomi , T. , Sidle , R. C. and Richardson , J. S. 2002 . Understanding processes and downstream linkages of headwater systems . Bioscience , 52 : 905 – 916 .
- Goodrich , D. C. , Lane , L. J. , Shillito , R. M. , Miller , S. N. , Syed , K. H. and Woolhiser , D. A. 1997 . Linearity of basin response as a function of scale in a semiarid watershed . Water Resources Research , 33 : 2951 – 2965 .
- Graf, W. L. 1988. Fluvial Processes in Dryland Rivers. Berlin: Springer-Verlag, 346 pp.
- Gregory, K. J., and D. E. Walling. 1973. Drainage Basin Form and Process. London, UK: Arnold, 458 pp.
- Guan , X. J. , Spence , C. and Westbrook , C. J. 2010 . Shallow soil moisture ground thaw interactions and controls: 2. Influences of water and energy fluxes . Hydrology and Earth System Sciences , 14 : 1387 – 1400 .
- Hansen , W. F. 2001 . Identifying stream types and management implications . Forest Ecology and Management , 143 : 39 – 46 .
- Harms , T. K. and Grimm , N. B. 2010 . Influence of the hydrologic regime on resource availability in a semi-arid stream-riparian corridor . Ecohydrology , 3 : 349 – 359 .
- Hassan , M. A. and Egozi , R. 2001 . Impact of wastewater discharge on the channel morphology of ephemeral streams . Earth Surface Processes and Landforms , 26 : 1285 – 1302 .
- Hauer , F. R. , Baron , L. S. , Campbell , D. H. , Fausch , K. D. , Hostetler , S. W. , Leavesley , G. H. , Leavitt , P. R. , Mcknight , D. M. and Stanford , J. A. 1997 . Assessment of climate change and freshwater ecosystems of the Rocky Mountains, USA and Canada . Hydrological Processes , 11 : 903 – 924 .
- Hedman, E. R., and W. R. Osterkamp. 1982. Streamflow characteristics related to channel geometry of stream in western United States. U.S. Geological Survey Water Supply Paper 2193.
- Hewlett, J. D. 1982. Principles of Forest Hydrology. Athens, GA: University of Georgia Press, 183 pp.
- Hewlett, J. D., and A. R. Hibbert. 1967. Factors affecting the response of small watersheds to precipitation in humid areas. In Forest Hydrology, ed. W. E. Sopper, and H. W. Lull, 275290. New York: Pergamon.
- Hooke , J. M. 2007 . Monitoring morphological and vegetation changes and flow events in dryland river channels . Environmental Monitoring and Assessment , 127 : 445 – 457 .
- Hornberger, G. M., J. P. Raffensperger, P. L. Wiberg, and K. N. Eshleman. 1998. Elements of Physical Hydrology. Baltimore, MD: Johns Hopkins University Press, 302 pp.
- Hughes , D. A. 2005 . Hydrological issues associated with the determination of environmental water requirements of ephemeral rivers . River Research Applications , 21 : 899 – 908 .
- Huxter, E. 2011. Personal communication. Department of Geography, Simon Fraser University.
- Hydrological Atlas of Canada. 1978. Department of Fisheries and Environment, Ottawa Ontario, Canada.
- Imperial Oil Resources Ventures. 2004. Mackenzie Gas Project Environmental Impact Statement V.2 Project Description. Submitted to National Energy Board and Joint Review Panel. APRCC.PR.2004.07. 330 pp.
- Jacobson , P. J. , Jacobson , K. M. , Angermeier , P. L. and Cherry , D. S. 2000 . Hydrologic influences on soil properties along ephemeral rivers in the Namib Desert . Journal of Arid Environments , 45 : 21 – 34 .
- Jones , J. B. and Rinehart , A. J. 2010 . The long-term response of stream flow to climatic warming in headwater streams of interior Alaska . Canadian Journal of Forest Research , 40 : 1210 – 1218 .
- Kharin , V. V. and Zwiers , F. W. 2005 . Estimating extremes in transient climate change simulations . Journal of Climate , 18 : 1156 – 1173 .
- Kisiel , C. C. , Duckstein , L. and Fogel , M. M. 1971 . Analysis of ephemeral flow in aridlands . Journal of the Hydraulics Division ASCE , 97 : 1699 – 1717 .
- Knighton , A. D. and Nanson , G. C. 1994 . Flow transmission along an arid zone anastomosing river, Cooper Creek, Australia . Hydrological Processes , 8 : 137 – 154 .
- Knighton, A. D., and G. C. Nanson. 1997. Distinctiveness, diversity and uniqueness in arid zone river systems. In Arid zone geomorphology: Processes, form and change in drylands, 2nd Edition, ed. D. S. G. Thomas, 185203. Chichester, UK: Wiley.
- Kouwen , N. , Soulis , E. D. , Pietroniro , A. , Donald , J. and Harrington , R. A. 1993 . Grouped response units for distributed hydrologic modelling . Journal of Water Resources Planning and Management, ASCE , 119 : 289 – 305 .
- Larned , S. T. , Datry , T. , Arscott , D. B. and Tockner , K. 2010 . Emerging concepts in temporary-river ecology . Freshwater Biology , 55 : 717 – 738 .
- Lee, S. 1975. Stochastic generation of synthetic streamflow sequences in ephemeral streams. In Publication no 117 de l'Association Internationale des Sciences Hydrologiques Symposium de Tokyo, December, 1975, pp. 691701.
- Leopold, L. B., and J. P. Miller. 1956. Ephemeral streams hydraulic factors and their relation to the drainage net. Geological Survey Professional Paper 282-A. Washington, DC: U.S. Government Printing Office, 37 pp.
- Levick, L., J. Fonseca, D. Goodrich, M. Hernandez, D. Semmens, J. Stromberg, R. Leidy, M. Scianni, D. P. Guertin, M. Tluczek, and W. Kepner. 2008. The ecological and hydrological significance of ephemeral and intermittent streams in the arid and semi-arid American southwest. Report by U.S. Environmental Protection Agency and USDA/ARS Southwest Watershed Research Center, EPA/600/R-08/134, ARS/233046. Washington, DC: U.S. Environmental Protection Agency, 116 pp.
- McCartney , S. E. , Carey , S. K. and Pomeroy , J. W. 2006 . Intra-basin variability of snowmelt water balance calculations in a subarctic catchment . Hydrological Processes , 20 : 1001 – 1016 .
- Martin , Y. and Church , M. 1995 . Bed-material transport estimated from channel surveys, Vedder River, British Columbia . Earth Surface Processes and Landforms , 20 : 347 – 361 .
- Marks , D. M. 1983 . Relations between field-surveyed channel networks and map-based geomorphometric measures, Inez, Kentucky . Annals of the Association of American Geographers , 73 : 358 – 372 .
- McClain , M. E. , Boyer , E. W. , Dent , C. L. , Gergel , S. E. , Grimm , N. B. , Groffman , P. M. , Hart , S. C. , Harvey , J. W. , Johnston , C. A. , Mayorga , E. , McDowell , W. H. and Pinay , G. 2003 . Biogeochemical hot spots and hot moments at the interface of terrestrial and aquatic ecosystems . Ecosystems , 6 : 301 – 312 .
- McPherson , H. J. and Rannie , W. F. 1969 . Geomorphic effects of the May 1967 flood in Graburn watershed, Cypress Hills, Alberta, Canada . Journal of Hydrology , 9 : 307 – 321 .
- Munro , D. S. 2000 . Progress in glacier hydrology: A Canadian perspective . Hydrological Processes , 14 : 1627 – 1640 .
- Munro , D. S. 2005 . A revised Canadian perspective: Progress in glacier hydrology . Hydrological Processes , 19 : 231 – 245 .
- Nadeau , T. L. and Rains , M. C. 2007 . Hydrological connectivity between headwater streams and downstream waters: How science can inform policy . Journal of the American Water Resources Association , 43 : 118 – 133 .
- Newbury, R. W. 1974. River hydrology in permafrost areas. In Permafrost Hydrology, Proceedings of Workshop Seminar 1974. Calgary, Alberta, February 2628, 1974, pp. 3137. Ottawa: Canadian National Committee for the International Hydrological Decade.
- North, C. 2005. Drylands rivers research. University of Aberdeen, Scotland. http://www.abdn.ac.uk/~gmi196/DrylandRivers/ (accessed Jan 20, 2011).http://www.abdn.ac.uk/~gmi196/DrylandRivers/
- Orwin, J. F., N. J. Cullen, and S. J. Fitzsimons. 2009. The fluvial sediment transfer response to energy balance tipping of melt generation, Joyce Glacier, McMurdo Dry Valleys, Antarctica: Preliminary results. In Proceedings of the Fourth Workshop of the International Association of Geomorphologists / Association Internationale des Gomorphologues Working Group on Sediment Budgets in Cold Environments (IAG/AIG SEDIBUD). Kingston, Ontario, October 1316, 2009, p. 31.
- Peters , D. L. , Prowse , T. D. , Marsh , P. , Lafleur , P. M. and Buttle , J. M. 2006 . Persistence of water within perched basins of the Peace-Athabasca Delta, northern Canada . Wetlands Ecology and Management , 14 : 221 – 243 .
- Peters , D. L. , Baird , D. J. , Monk , W. A. and Armanini , D. G. 2011 . Establishing standards and assessment criteria for ecological instream flow needs in agricultural regions of Canada . Journal of Environmental Quality ,
- Peterson , B. J. , Wollheim , W. M. , Mulholland , P. J. , Webster , J. R. , Meyer , J. L. , Tank , J. L. , Marti , E. , Bowden , W. B. , Valett , H. M. , Hershey , A. E. , McDowell , W. H. , Dodds , W. K. , Hamilton , S. K. , Gregory , S. and Morrall , D. D. 2001 . Control of nitrogen export from watersheds by headwater streams . Science , 292 : 86 – 90 .
- Poff , N. L. 1992 . Why disturbances can be predictable: A perspective on the definition of disturbance in streams . Journal of the North American Benthological Society , 11 : 86 – 92 .
- Poff , N. L. , Allan , J. D. , Bain , M. B. , Karr , J. R. , Prestegaard , K. L. , Richter , B. D. , Sparks , R. E. and Stromberg , J. C. 1997 . The natural flow regime: A paradigm for river conservation and restoration . Bioscience , 47 : 769 – 784 .
- Pomeroy , J. W. , Gray , D. M. , Brown , T. , Hedstrom , N. R. , Quinton , W. L. , Granger , R. J. and Carey , S. K. 2007 . The cold regions hydrological model: A platform for basing process representation and model structure on physical evidence . Hydrological Processes , 21 : 2650 – 2667 .
- Priesnitz , K. 1990 . Geomorphic activity of rivers during snow melt and break-up, Richardson Moutains, Yukon and Northwest Territories, Canada . Permafrost and Periglacial Processes , 1 : 295 – 299 .
- Quinton , W. L. and Carey , S. K. 2008 . Towards an energy-based runoff generation theory for tundra landscapes . Hydrological Processes , 22 : 4649 – 4653 .
- Quinton , W. L. and Roulet , N. T. 1998 . Spring and summer runoff hydrology of a subarctic pattern wetland . Arctic and Alpine Research , 30 : 285 – 294 .
- Reid , I. and Laronne , J. B. 1995 . Bed load sediment transport in an ephemeral stream and a comparison with seasonal and perennial counterparts . Water Resources Research , 31 : 773 – 781 .
- Renard , K. G. and Keppel , R. V. 1966 . Hydrographs of ephemeral streams in the Southwest . Journal of the Hydraulics Division ASCE , 92 ( HY2 ) : 33 – 52 .
- Renard , K. G. and Laursen , E. M. 1975 . Dynamic behavior model of ephemeral stream . Journal of the Hydraulics Division ASCE , 101 ( HY5 ) : 511 – 528 .
- Richardson, J. S., and R. D. Moore. 2009. Stream and riparian ecology. In Compendium of forest hydrology and geomorphology in British Columbia, ed. R. G. Pike, T. E. Redding, R. D. Moore, R. D. Winkler, and K. D. Bladon, 441460. BC Land Management Handbook. BC Ministry of Forests and Range Research Branch, Victoria, BC and FORREX Forum for Research and Extension in Natural Resources, Kamloops, BC.
- Ronan , A. D. , Prudic , D. E. , Thodal , C. E. and Constanz , J. 1998 . Field study and simulation of diurnal temperature effects on infiltration and variably saturated flow beneath an ephemeral stream . Water Resources Research , 34 : 2137 – 2153 .
- Rood , S. B. , Braatne , J. H. and Hughes , F. M. R. 2003 . Ecophysiology of riparian cottonwoods: Stream flow dependency, water relations and restoration . Tree Physiology , 23 : 1113 – 1124 .
- Rood , S. B. and Mahoney , J. M. 2000 . Revised instream flow regulation enables cottonwood recruitment along the St. Mary River, Alberta, Canada . Rivers , 7 : 109 – 125 .
- Sandercock , P. J. and Hooke , J. M. 2010 . Assessment of vegetation effects on hydraulics and of feedbacks on plant survival and zonation in ephemeral channels . Hydrological Processes , 24 : 695 – 713 .
- Sauchyn, D. J. 1993. Quaternary and late Tertiary landscape evolution in the western Cypress Hills. In Quaternary and late Tertuary landscapes of southwestern Saskatchewan and adjacent areas, ed. D. J. Sauchyn, 4658. Regina, SA: Canadian Plains Research Centre.
- Sayama, T., J. J. McDonnell, A. Dhakal, and K. Sullivan. In review. Storage excess: A new conceptual framework for subsurface water collection, storage and discharge at the watershed scale. Hydrological Processes.
- Schindler , D. W. 2001 . The cumulative effects of climate warming and other human stresses on Canadian freshwaters in the new millennium . Canadian Journal of Fisheries and Aquatic Sciences , 58 : 18 – 29 .
- Selker , J. , van de Giesen , N. , Westhoff , M. , Luxemburg , W. and Parlange , M. B. 2006 . Fiber optics opens window on stream dynamics . Geophysical Research Letters , 33 : L24401
- Shamir , E. , Meko , D. M. , Graham , N. E. and Georgakakos , K. P. 2007 . Hydrologic model framework for water resources planning in the Santa Cruz River, southern Arizona . Journal of the American Water Resources Association , 43 : 1155 – 1170 .
- Sharma , K. D. , Murthy , J. S. R. and Dhir , R. P. 1994 . Streamflow routing in the Indian arid zone . Hydrological Processes , 8 : 27 – 43 .
- Sidorchuk , A. , Borisova , O. and Panin , A. 2001 . Fluvial response to the Late Valdai/Holocene environmental change on the East European Plain . Global and Planetary Change , 28 : 303 – 318 .
- Smerdon , B. D. , Mendoza , C. A. and Devito , K. J. 2005 . Can lakes and ponds be represented in a hydrological model without excessive numerical intervention? . Elements , 23 : 22 – 26 .
- Sophocleous , M. 2000 . From safe yield to sustainable development of water resources the Kansas experience . Journal of Hydrology , 235 : 27 – 43 .
- Spence , C. 2006 . Hydrological processes and streamflow in a lake dominated water course . Hydrological Processes , 20 : 3665 – 3681 .
- Spence , C. and Rouse , W. R. 2002 . The energy budget of subarctic Canadian Shield terrain and its impact on hillslope hydrology . Journal of Hydrometeorology , 3 : 208 – 218 .
- Spence, C., and D. J. Sauchyn. 1999. Groundwater influence on valley head geomorphology, upper Battle Creek basin, Alberta and Saskatchewan. In Holocene climate and environmental change in the Palliser Triangle: A geoscience context for evaluating the impacts of climate change on the southern Canadian Prairies, ed. D. Lemmen, R. Vance. Geological Society of America Bulletin 534: 249256.
- Spence , C. and Woo , M.-K. 2003 . Hydrology of subarctic Canadian Shield: Soil-filled valleys . Journal of Hydrology , 279 : 151 – 166 .
- Spence , C. and Woo , M.-K. 2006 . Hydrology of subarctic Canadian Shield: Heterogeneous headwater basins . Journal of Hydrology , 317 : 138 – 154 .
- Srikanthan , R. and McMahon , T. A. 1980 . Stochastic generation of monthly flows for ephemeral streams . Journal of Hydrology , 47 : 19 – 40 .
- Svec , J. R. , Kolka , R. K. and Stringer , J. W. 2005 . Defining perennial, intermittent, and ephemeral channels in Eastern Kentucky: Application to forestry best management practices . Forest Ecology and Management , 214 : 170 – 182 .
- Texas Forest Service. 2010. Texas forestry best management practices. http://texasforestservice.tamu.edu/uploadedFiles/Sustainable/bmp/Publications/BMP%20Manual_Aug2010%20%20web.pdf (accessed February 9, 2011).http://texasforestservice.tamu.edu/uploadedFiles/Sustainable/bmp/Publications/BMP%20Manual_Aug2010%20%20web.pdf
- Todd , A. K. , Buttle , J. M. and Taylor , C. H. 2006 . Hydrologic dynamics and linkages in a wetland-dominated basin . Journal of Hydrology , 319 : 15 – 35 .
- Tooth , S. 2000 . Process, form and change in dryland rivers: A review of recent research . Earth-Science Reviews , 51 : 67 – 107 .
- Tzoraki , O. , Nikolaidis , N. P. , Amaxidis , Y. and Skoulikidis , K. T. 2007 . In-stream biogeochemical processes of a temporary river . Environmental Science and Technology , 41 : 1225 – 1231 .
- United Nations Environment Program/Global Resource Information Database (UNEP/GRID). 1991. Global digital datasets for land degradation studies: A GIS approach. Prepared by U. Diechmann and L. Eklundh. GRID case study series No. 4. Nairobi, Kenya: UNEP/GEMS and GRID, 103 pp.
- Uys , M. C. and O'Keeffe , J. H. 1997 . Simple words and fuzzy zones: Early directions for temporary river research in South Africa . Environmental Management , 21 : 517 – 531 .
- Vance, L. K. 2009. Geographically isolated wetlands and intermittent/ephemeral streams in Montana: Extent, distribution, and function. Report to the Montana Department of Environmental Quality and the U.S. Environmental Protection Agency. Helena, Montana: Montana Natural Heritage Program, 33 pp.
- Vogt , T. , Schneider , P. , Hahn-Woernle , L. and Cirpka , O. A. 2010 . Estimation of seepage rates in a losing stream by means of fiber-optic high-resolution vertical temperature profiling . Journal of Hydrology , 380 : 154 – 164 .
- Waddington , J. M. , Roulet , N. T. and Hill , A. R. 1993 . Runoff mechanisms in a forested groundwater discharge wetland . Journal of Hydrology , 147 : 37 – 60 .
- Wagener , T. , Sivapalan , M. , Troch , P. and Woods , R. 2007 . Catchment classification and hydrologic similarity . Geography Compass , 1/4 : 901 – 931 .
- Waiser, M. J., V. Tumber, and J. Holm. 2011a. Effluent-dominated streams. Part 1: Presence and effects of excess nitrogen and phosphorus in Wascana Creek, Saskatchewan, Canada. Environmental Toxicology and Chemistry 30: 496507.
- Waiser, M. J., D. Humphries, V. Tumber, and J. Holm. 2011b. Effluent-dominated streams. Part 2: Presence and possible effects of pharmaceuticals and personal care products in Wascana Creek, Saskatchewan, Canada. Environmental Toxicology and Chemistry 30: 508519.
- Walker , H. J. 1975 . Intermittent arctic streams and their influence on landforms . Catena , 2 : 181 – 192 .
- Walters , M. O. 1990 . Transmission losses in arid region . Journal of Hydraulic Engineering ASCE , 116 : 129 – 138 .
- Wang, Q. J., and D. E. Robertson. 2011. Multisite probabilistic forecasting of seasonal flows for streams with zero value occurrences. Water Resources Research 47: W02546, doi:1029/2010WR009333.
- Welty, C., A. J. Miller, K. T. Belt, J. A. Smith, L. E. Band, P. M. Groffman, T. M. Scanlon, J. Warner, R. I. Ryan, R. I. Shedlock, and M. P. McGuire. 2007. Design of an environmental field observatory for quantifying the urban water budget. In Cities of the future: Towards integrated sustainable water and landscape management, ed. V. Novotny, and P. Brown, 7491. London, UK: IWA Publishing.
- Williams , W. D. 1988 . Limnological imbalances: An antipodean viewpoint . Freshwater Biology , 20 : 407 – 420 .
- Winter , T. C. 2007 . The role of ground water in generating streamflow in headwater areas and in maintaining base flow . Journal of the American Water Resources Association , 43 : 15 – 25 .
- Wolman , M. G. and Gerson , R. 1978 . Relative scales of time and effectiveness of climate in watershed geomorphology . Earth Surface Processes , 3 : 189 – 208 .
- Woo , M.-K. and Steer , P. 1982 . Occurrence of surface flow on arctic slopes, southwestern Cornwallis Island . Canadian Journal of Earth Sciences , 19 : 2368 – 2377 .
- Ye , W. , Bates , B. C. , Viney , N. R. , Sivapalan , M. and Jakeman , A. J. 1997 . Performance of conceptual rainfall-runoff models in low-yielding ephemeral catchments . Water Resources Research , 33 : 153 – 166 .
- Young , G. J. and Ommanney , C. S. L. 1984 . Canadian glacier hydrology and mass balance studies; a history of accomplishments and recommendations for future work . Geografiska Annaler , 66A : 169 – 182 .
- Young, K. L., and M.-K. Woo. 2000. Hydrological environment of the Hot Weather Creek basin, Ellesmere Island, Nunavut. In Environmental response to climate change in the Canadian High Arctic, ed. M. Garneau and B.T. Alt. Geological Survey of Canada Bulletin 529: 347374.
- Young , K. L. , Woo , M.-K. and Edlund , S. A. 1997 . Influence of local topography, soils, and vegetation on microclimate and hydrology at a high arctic site, Ellesmere Island, Canada . Arctic and Alpine Research , 29 : 270 – 284 .