Abstract
Temporary streams on the Canadian Prairies have a dry streambed with some regularity. Attributes that capture these zero flows could be used to better compare temporary streams with perennial streams from the same area, and contribute to a broader stream classification. This case study of observed variability in flow rates of prairie streams is a step towards the goal of establishing a stream classification system that would be widely useful for practitioners who need to address prediction in ungauged basins in this region. Such a classification system could support inferences that need to be made for streams that are either not currently gauged or for which the period of record is either too short or too discontinuous to allow an understanding of the potential streamflow responses. An approach to streamflow classification that relies solely on hydrometric record may be of particular value for temporary streams that are common in the southern Canadian Prairie Provinces. Similarity of hydrometric station streamflow response within groups is illustrated using both common techniques such cumulative annual and daily mass curve analysis, and a novel streamflow metric, the ratio of the magnitude of the third quartile monthly flow totals to the maximum of the monthly total flow for the period of record is also examined. The purpose of this case study is to demonstrate that by applying conventional analytical techniques in innovative ways the practicing hydrologist can better infer the behavior of streams in areas that are either ungauged, have been gauged only for a short period of time, or are gauged intermittently. While a complete classification system for these streams would include physical, biological, and chemical properties in addition to those that can be determined from hydrometric records, the grouping of hydrologically similar sites is a first step. Such analysis also provides insight into anticipated stream response that will allow for improving design and operation of hydrometric networks. The analysis presented is restricted to data from the Canadian Reference Hydrologic Basin Network (RHBN).
Les cours d'eau temporaires des Prairies canadiennes voient leur lit s'asscher avec une certaine rgularit. Des attributs qui caractrisent ces dbits nuls pourraient tre utiliss pour mieux comparer les cours d'eau temporaires aux cours d'eau prennes de la mme rgion et tendre ainsi le classement des cours d'eau une plus grande chelle. La prsente tude de cas de la variabilit observe des dbits des cours d'eau des Prairies constitue un pas vers la ralisation de l'objectif qui consiste tablir un systme de classement des cours d'eau qui serait largement utile aux praticiens ayant besoin de se tourner vers des prdictions lies aux bassins non jaugs dans cette rgion. Un tel systme de classement pourrait soutenir les infrences qui doivent tre formules lgard des cours d'eau qui l'heure actuelle ne sont pas jaugs ou pour lesquels la priode d'enregistrement est soit trop courte, soit trop discontinue pour permettre une comprhension des rponses de lventuel coulement fluvial. Une approche du classement des cours d'eau qui repose entirement sur les enregistrements hydromtriques pourrait s'avrer d'une valeur particulire pour les cours d'eau temporaires, qui sont courants dans le sud des provinces des Prairies. La similarit de la rponse des dbits des stations hydromtriques au sein des groupes est illustre au moyen la fois de techniques courantes comme l'analyse de la courbe des valeurs cumules annuelles et quotidiennes et une nouvelle mthode d'analyse mtrique du dbit; de plus, le rapport entre l'importance des dbits mensuels totaux du troisime quartile et le maximum du dbit total mensuel pour la priode d'enregistrement est examin. Cette tude de cas a pour but de dmontrer qu'en appliquant des mthodes d'analyse traditionnelles, qui reposent sur des moyens innovateurs, l'hydrologue peut tirer de meilleures conclusions du comportement des cours d'eau dans des secteurs qui ont t jaugs, qui n'ont t jaugs que pendant une courte priode ou encore qui sont jaugs de faon intermittente. Mme si un systme de classification complet pour ces cours d'eau engloberait les proprits physiques, biologiques et chimiques, en plus de celles pouvant tre dtermines partir des dossiers hydromtriques, le regroupement des sites semblables sur le plan hydrologique constitue une premire tape. Une telle analyse nous claire galement sur la rponse prvue du cours d'eau, d'o la possibilit d'amliorer la conception et l'exploitation des rseaux de stations hydromtriques. L'analyse prsente se limite aux donnes tires du Rseau hydromtrique de rfrence (RHR) du Canada.
Introduction
Hydrometric networks represent a small sample of streams on the landscape for various periods of time. Generally, the information from the individual gauge sites forms the historical record. Both the limited number of gauging locations in the network and the delay in access to the observations results in water managers, environmental regulators, and others making decisions based on past experience and by comparison to other measured results, particularly for ungauged sites. A stream classification system would support decision making by providing a common basis for comparison of expected stream response at ungauged locations or poorly gauged locations to sites which have previously experienced similar drivers to the streamflow (e.g., severe weather).
Hydrologic or catchment classification systems, such as proposed by Wagener et al. (Citation2007), are predicated upon a conceptual understanding of physical, chemical and biological processes, thus requiring assimilation of data from a variety of sources and an understanding of many separate processes. Alternatively, a classification system for streams that relies solely on demonstrable variation within an existing hydrometric record may ease the burden of data review yet still provide a sufficiently good understanding of streamflow characteristics to allow for prediction of stream response at ungauged locations.
An approach to classification that relies solely on the hydrometric record may be of particular value for temporary streams such as those found in the southern Canadian Prairie Provinces. Agricultural practices have disrupted much of the landscape and changed both the quantity and the timing of the natural drainage of water throughout the catchments through the practice of storage, irrigation and drainage (McGee et al., Citation2012). Since development of landscape has evolved at the same time as the hydrometric network was evolving it is difficult to definitively classify catchments based on physio-climate parameters.
The Canadian Prairies is the region in the interior continental plains of North America between the Rocky Mountains and Lake Winnipegosis. The most populated area of the three Prairie Provinces lies within the prairie ecozone. This region has extensive agricultural development and native vegetation is drought resistant. The prairie ecozone is one of the driest areas of the country with average annual precipitation rates (P) less than 500 mm/yr and average annual potential evapotranspiration rates (PET) from 600900 mm/yr (Burn et al., Citation2008).
The combined grasslands and aspen parkland landscapes of the plains are almost entirely within the Nelson River basin that flows into Hudson Bay. The recent geological history of the prairies is predominantly glacial. The combination of semiarid cold climate and extensive glacial deposits that blanket the region produce a distinct hydrologic and hydrogeologic regime (van der Kamp and Hayashi, Citation2009). Snow redistribution is an important hydrologic process on the prairies (e.g., Fang and Pomeroy, Citation2009). The resulting hummocky terrain with small lakes and sloughs can reduce contributing areas and streamflow volumes directed to large order rivers.
Stichling and Blackwell (Citation1957) demonstrate that the contributing area is variable over time because the amount of runoff needed to fill available storage depends upon antecedent runoff and climatic conditions; areas which contribute runoff one year may not contribute in a subsequent year. Hillslope runoff on the prairies is often characterized by drainage into depression storage rather than into the stream network (Shook and Pomeroy, Citation2011) and changes in the connectivity of the drainage strongly affect the hydrologic response.
Water on the prairies is extensively and increasingly managed (Burn et al., Citation2008; McGee et al., Citation2012). Flow duration curves illustrate the relative dryness of the prairies and frequent dry conditions result in intermittent flows (Burn et al., Citation2008). Ehsanzadeh et al. (Citation2012) show that flood frequency curves are affected by depression storage. Where depressions do not exist, the curves mirror the shape of the precipitation curve; the shape and slope of flood frequency curves are controlled by the nature of depression storage. Prairie streams also exhibit different relationships between measures of flow and drainage area than perennial streams (Burn et al., Citation2008; Buttle et al., Citation2012).
Prairie streams as a group are generally not well studied (Buttle et al., Citation2012); of those that are gauged, many are regulated, and most are only operated seasonally (March to October). This lack of long-term continuous observations is problematic in our understanding of the prairie hydrology. In many studies that consider the impacts of climate and land use, the records from seasonally operated hydrometric gauges that are subject to some degree of anthropogenic influence or regulation are not considered because they do not conform to the requirement of continuity (e.g., Whitfield and Cannon, Citation2000). It is unfortunate that Canada's national hydrometric program is unable to continuously monitor at least some sites on the prairies; Monk et al. (Citation2011) suggest that streams from this region constitute a major gap in theirs and other studies since prairie streams may be a distinct hydrologic regime. For the purpose of this discussion, seasonally operated sites are included as it was apparent from the analysis that winter flow volumes are at least an order of magnitude smaller (sometimes several orders of magnitude smaller) than the summer season flows for all sites in the study. Preliminary analysis indicated that even if the annual average total streamflow for sites located in the southern prairie grasslands was doubled in magnitude, the annual yield from grasslands sites would still be significantly lower than other regions; this would be sufficient for them to be considered a distinct response type. However, if all sites with seasonal record were excluded from the analysis, there would be no data for temporary streams in this part of Canada. Temporary streams are considered to be streams that experience a dry streambed with some degree of regularity including watercourses that may be called: episodic, ephemeral, or intermittent (Burn et al., Citation2008; Buttle et al., Citation2012). For comparison, the same suite of characteristics has been tabulated for streams where such dry bed conditions have not been observed.
A basic stream classification for the Canadian Prairies would include two broad categories:
1. | Native Prairie Streams: streams that respond to localized water sources, i.e., precipitation and snowmelt, proportionally throughout the basin, and that can be dry from time to time, and | ||||
2. | Exotic Prairie Streams: Streamflow is predominately conveyed from the headwaters and these streams do not experience dry bed conditions. They respond to localized water sources disproportionally throughout the basin. |
Native prairie streams are characterized by a temporary nature. Such streams are entirely dependent on runoff from grasslands sources and frequently are found to demonstrate zero flow conditions during the winter and periodically during the March to October season.
Examples of streams flowing through the Canadian Prairies with exotic sources of water include the Bow, Oldman, St. Mary, and South Saskatchewan Rivers. Flows in these streams are dominated by headwater conditions on the eastern slopes of the Rocky Mountains and they are little influenced by runoff from the immediate prairie landscape that they are passing through. Typically, these exotic prairie streams do not run dry. The distinct quality of continuous or persistent streamflow in exotic prairies streams rendered them as prime candidates for regulation. Consequently, most of the exotic streams on the Canadian Prairie Provinces have been subjected to significant water management works with the building of dams, reservoirs, diversions, canals, and levees to allow for storage and re-distribution of surface water (e.g., South Saskatchewan River). Thus, there are virtually no naturally flowing exotic streams in the Canadian Prairies from which to extract data that would allow one to establish measures of streamflow variability that lead to a useful classification scheme. However, within the Canadian Prairie Provinces there are areas where the headwaters of exotic prairie streams are unregulated and have long periods of continuous record that can be used for demonstrating distinct natural characteristics of streamflow from one stream type to another.
This study was undertaken with a view to establishing streamflow attributes that are characteristic of temporary streams in the Canadian Prairies; ultimately allowing one to make inferences as to the behavior of streams at locations that are not gauged from what has been observed at gauged sites. To achieve this, a suite of metrics has been assembled that demonstrates distinct groupings of streamflow response for the study area.
Methodology
Data
The data used in this study were obtained from the archived information available from the HYDAT data set (Environment Canada, Citation2011) and supplemented by the Station Visit Summary Data maintained in the Water Survey of Canada district offices in Calgary, Regina, and Winnipeg. Each site considered in this study is a Reference Hydrometric Basin Network (RHBN) site (Brimley et al., Citation1999). RHBN sites are characterized by pristine or stable hydrological conditions, unaffected by developments within the basin, and which have good quality data for at least 20 years. However, these criteria were applied with a degree of discretion; for example, the criterion of having less than 10% of the surface area of the basin modified in some fashion would likely discount vast areas of agricultural land where the original forests or aspen groves have been cleared and/or the depressions filled and drainage improved. Other considerations such as: basins with structures controlling less than 5% of the area of a basin makes little sense in a prairie context where the streamflow is not well correlated with the drainage area. Nevertheless, these RHBN sites do represent the most stable record available, and thus, formed the basis of this study.
The importance of water management in the grasslands of the Canadian Prairies has influenced the stream gauging network. Consequently, the majority of stream gauging sites are on regulated streams and often do not reflect natural flow conditions; such gauging sites are generally not included in the RHBN and have not been used in this study. Because many native prairie streams cease to flow during the cold, dry winter conditions, the majority of the stream gauges are operated on a seasonal basis, generally from March to October.
The daily streamflow data for 54 RHBN (both continuous and seasonal), in the three Prairie Provinces (Alberta, Saskatchewan, and Manitoba) () were extracted from Environment Canada's HYDAT database. Of these, some have been discontinued, some are operated for a three month season, and some have many missing periods of record, leaving only 35 sites with sufficient data for analysis across the region. The focus of this study was to understand streams that are somewhat temporary in nature, and to do so by comparing and contrasting other sites in the study area. Therefore, the analysis was restricted to the 27 sites south of 54N latitude. In , the final groupings of stations developed here are shown, as it will be useful for the reader to see the spatial distribution of these locations.
Figure 1. Location of Reference Hydrometric Basin Network (RHBN) sites in the Canadian Prairie Provinces. Symbols indicate the grouping of similar characteristics identified in the study; squares indicate Group A streams, diamonds indicate Group B streams, and circles indicate Group C streams. Solid symbols indicate the RHBN sites examined south of 54N.
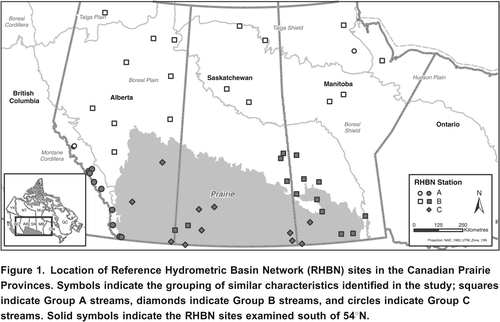
Analysis
The series of metrics described below were considered as a potential basis for classification. All metrics in the study were chosen with the requirement that each could be derived from the available streamflow records of daily mean discharges.
Average Annual Basin Yield
Average annual basin yield and mean annual runoff are terms that are used interchangeably. The streamflow records were transformed from mean daily flow rate (m3/s) to average annual basin yield (mm/yr) based on the gross drainage area reported in HYDAT. This allows runoff depth comparison independent of drainage area. In these calculations, if the winter flow was not available it was assumed to be negligible. All the seasonal sites in the study had extensive periods of zero flow during the summer months, so this assumption was considered to be reasonable. Because there are no native prairie RHBN sites with year round flow records to consider, seasonal sites had to be used to investigate temporary streams.
Double mass analysis, where the cumulative annual basin yield is plotted against the cumulative number of years in the hydrometric record, was used to generate the average annual basin yield for each location in the study. Applying linear regression to these data results in a line the slope of which is representative of the long-term average of flow to the hydrometric gauge. It is the slope of the double mass line that was used to determine the average annual basin yield shown later in and in and . This cumulative analysis, i.e., the double mass curve, often shortened to mass curve, also clearly demonstrates any inconsistencies in basin yield from year to year. For example, a completely dry year or an extraordinarily high flow year stand would out as an abrupt change in the trace of points plotted on the graph.
Table 1. Subset of RHBN Prairie Sites considered in this study. These sites are all located south of 54N.
Table 2. Summary of the most useful streamflow statistics for discriminating between RHBN groupings.
It is important to understand that the average flow to the gauge is distinct from the average condition in the catchment, as for prairie streams the flow may accumulate disproportionately throughout the catchment/drainage basin. If one is looking at data for an exotic stream, the average value may not be representative of any identifiable point in the drainage basin.
Apart from looking at the whole period of record, in the mass curve analysis the data were also split into two parts to demonstrate runoff variability between the first half and second half of the station records; rivers with consistent long-term annual runoff will present as straight lines of similar slope, while streams with highly variable runoff are expected to exhibit changes in slope.
Quartiles of Monthly Runoff Hydrographs
Temporary streams are highly variable; to reach an understanding of the monthly variability for all sites in the study, the minimum, maximum, and quartiles for each month were computed and plotted as a hydrograph. Preliminary examination of these plots suggested the proportion of the third quartile to the maximum was a characteristic worth exploring. This last quartile ratio (LQR), shown in Equation
Equation 1
, is the ratio of the difference between the maximum monthly flow (Q4) and 75% (Q3), to the difference between 75% (Q3) and the minimum (Q0).
Since there are many zeros in the data base for some months and some totally dry years, the calculation was only performed for months where Q3 flow was greater than zero, generally during the March to July period.
LQR is computed for different time periods so as to gain a feel for seasonality in the statistic. The first time period presented compares only the months where no sites in the study demonstrated zero flow for Q3, i.e., March through May. For these months both the average and the range in LQR are computed as was done in the case of basin yield. Comparing the ratio of the range to the average was useful for basin yield in comparing the hydrometric sites, but ratio of the mean LQR to range in LQR did not serve to distinguish any difference among gauge sites.
One problem in understanding the LQR as a classification tool for temporary streams is that not all sites in the study area had flow during mid to late summer, hence the MarchJuly LQR is only calculated for months where Q3 is greater than zero. The minimum observable LQR may occur during the high flow season (e.g., May, June, July), because the Q3 is also very high. The maximum observable LQR typically occurs during low flow months. For streams in the southern Prairie Provinces that have low annual yields, the LQR in the shoulder seasons is generally found to be extremely high, which is indicative of extreme snowmelt or rainfall events during a normally low flow period. For example, if a stream typically demonstrates trickling amounts of flow, say 0.001 m3/s on average during September, and in one year responds to a rainfall event that generates an average monthly flow of 1 m3/s, which may not be an abnormal flow rate in June, but is rare in September, the LQR for September would compute to be 1000, a similar event for the same stream in June may only result an LQR in the range of 3 to 5. The occurrence of rare, high volume localized meteorologic events, such as extreme rainfall impacting the low flow season, suggests that for such times of year the LQR is event driven and, as such, may not be a good metric for comparison without the context of other LQR statistics. Native prairie streams may go decades without experiencing a high precipitation event. Still it is important to understand as a group what generally occurs and what can be expected to occur, hence a suite of LQR statistics may be necessary to gain confidence in assigning a grouping to any individual site. Four attributes of LQR were considered for each site in the study: 1) average March to May LQR, 2) March to May LQR range, 3) non-zero minimum average LQR, and 4) maximum monthly LQR.
Cumulative Daily Discharges
Similar to the annual mass curves of runoff, cumulative daily flow volumes for each year of record were calculated. Plots of cumulative daily flow (i.e., daily discharge in dam3) were used to determine the average start date of basin freshet, the average duration of the period of peak yields, and the start date of recession or return to base flow.
Population Proportion to Annual Total Flow
Other metrics derived from the data for the common 30 year period from 1980 to 2009 included: average number of days per year with flow 0.05% of annual yield, average number of days per year with flow > 1.0% of annual yield, and average number of days per year with flow > 2.0% of annual yield.
The selection of these individual metric thresholds is explained in terms of a theoretical stream, which, if demonstrated, equal flow for every day of the year would have a flow proportion of 1/365 or 0.27%; 0.05% is the equivalent of 1/2000 of the annual yield; a value that would be expected to always be exceeded in the theoretical stream. In natural streams, such a value is expected to be commonly exceeded in continuous streams, but rarely exceeded in temporary streams, and thus, would be a good descriptor to distinguish between these two types of streams. Of course, the theoretical stream does not at all resemble any stream in a natural setting, but the concept of population proportion of annual flow might still be descriptive. For the other thresholds, a 1% level would amount to flow that is exceeded 3.65 days per year in the theoretical stream; a 2% level would represent 1 week a year. A variety of ratios were considered before choosing these rather arbitrary ranges that are shown in . The proportion of the year with flows less than 0.05% of annual total was the first example where a distinction could be made. While other ratios were considered, only the proportion of the year with 0.05%, 1%, and 2% of the year are presented as potential distinguishing characteristics of temporary streams.
This population proportion was computed on a 365 day year even though many of the gauging sites are only operated during the 244 day season from March to October. During the analysis it was observed that for seasonal streams that had partial record during the period from late October to early March the data were always in the < 0.05% range. From that observation, the assumption was made that daily flows for seasonal streams is less than 0.05% of the annual total during the winter period; hence, there would be no difference in the result if a 365 day year was used for all sites.
Dry or Zero Flow Occurrences
The daily flow record from the HYDAT hydrometric database makes no distinction between dry channel or zero flow within a wet channel, although these are significantly different hydrologic events. Nevertheless, the frequency and duration of the occurrence of zero flow days was considered worthy of noting when compiling a list of easily discernable data from the database. Over the common period 1980 to 2009, the proportion of the record for each gauging site with completely dry years (i.e., zero flow) and the average number of zero flow days for the March to October season are expressed as percentages. Because many sites are not operated from November through February, a comparison of zero flow occurrences during the winter was not possible.
Results
A series of metrics were investigated in this study, some showed little promise as a defining characteristic of the amount of streamflow passing a gauging site, while others showed some value in terms of providing context or contrast along with other metrics, but that individually would not provide a basis for classification. All metrics considered were based on the analysis of the series of mean daily discharge or the average annual runoff, i.e., basin yield.
Average Annual Basin Yield
All RHBN streams south of 54N in the Prairie Provinces exhibit a relationship between the logarithm of mean annual discharge and logarithm of drainage basin area ( a). This separation also exists for the logarithm of mean annual runoff and the logarithm of drainage area ( b). For ease of discussion, the groupings of hydrometric stations displaying similar streamflow response are labeled as A, B, and C. Slopes of the lines in the log-log plot for Group A and Group B are more or less parallel and are greater than unity. The slope of the log-log plot for Group C streams is less than unity ( a). The difference in the log-log offset between stream types changes from 456 to 61 to 13 for Group A, B, and C, respectively.
Figure 2. Relationship between drainage basin area and a) mean annual discharge, and b) annual runoff for the three groups of RHBN stations in the Prairie Provinces south of 54N.
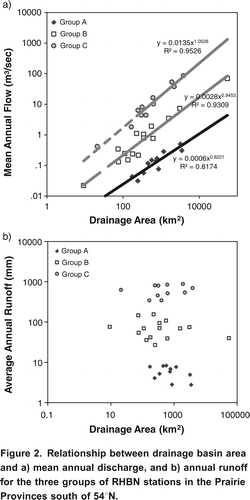
The derived metrics for the 27 RHBN sites are shown in . A clear progression of streams ranging in average annual runoff from 4 mm per year to as much as 913 mm per year is shown. The comparison between the observed ranges of annual yield to the average annual yield may be the most descriptive of this group of metrics. This ratio for sites in Group A (), have a ratio of 1.5 or less; Group B ranges from 1.5 to 2.6; and Group C sites range from 3.3 to 8.9.
In presenting results, one member of each group was selected for comparative purposes; these examples are: 05NF002 Antler River near Melita, MB; 05DE007 Rose Creek at Alder Flats, AB; and 05BB001 Bow River at Banff, AB. Antler and Rose Creeks were chosen randomly, and the Bow River at Banff was selected as a well known example of an upstream site on an exotic prairie stream. In to , the sites progress from the continental divide, with the Bow River site ( a) being the closest to the divide and Antler River ( c) being the furthest from the divide.
Figure 3. Cumulative annual mass curve for a) Bow River at Banff 05BB001, b) Rose Creek near Alder Flats 05DE007, and c) Antler River near Melita 05NF002.
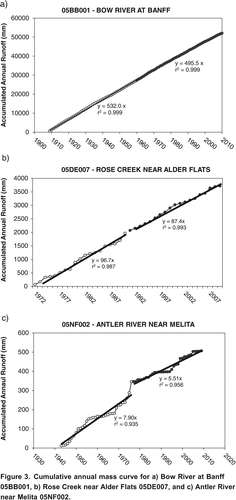
Figure 4. Cumulative distribution of daily mean discharge (gray) for a) Bow River at Banff 05BB001, b) Rose Creek near Alder Flats 05DE007, and c) Antler River near Melita 05NF002.
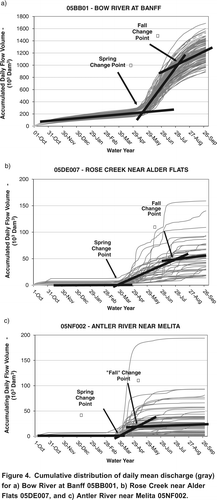
Figure 5. Distribution of monthly quartiles for a) Bow River at Banff 05BB001, b) Rose Creek near Alder Flats 05DE007, and c) Antler River near Melita 05NF002.
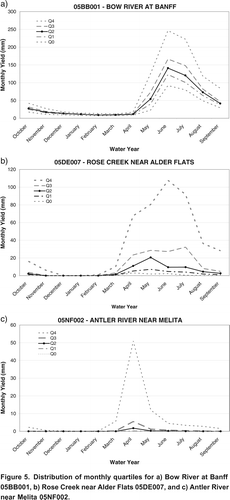
Cumulative mass curves of total annual yield for the three examples above are shown in . A number of patterns emerge which are characteristic of all sites in each group. Antler Creek ( c) demonstrates periods of zero flow as shown by subsequent years which have the same value as the preceding year. Of the eleven sites in Group C, only three did not have completely dry years. Neither Group A nor Group B sites have any completely dry years.
Additionally, two things are shown in the examples that are characteristic of the data collected for other hydrometric sites in the same groupings: first, the magnitude of the average runoff (as shown by the slope of the line) decreases dramatically from Group A to Group B to Group C. Also, the year-to-year variability increases in the same way between the three groups. Typically, hydrometric sites in Group A show little year-to-year variability as demonstrated by the nearly straight mass curve line. On the other hand, Group C sites show large year-to-year variability, and Group B sites are in between.
Duration of Period of Peak Yield
The determination of the duration of the period of peak yield was derived from the charts depicting accumulated daily flow volumes, examples of which are provided in . As shown, there is comparatively little increase in accumulation volume in the winter and late fall compared to the large increases in the spring and early summer months. Spring and fall change points were established by computing intersecting slopes of the average accumulating volume.
Although this parameter has some merit, as streams with the least yield tend to also have significantly shorter durations, it is likely fair to say that this parameter is not, by itself, able to show distinct differences in class of stream; however, in the context of other metrics this parameter may help to sway the balance in choice of stream class.
The streams compared in are representative of their respective grouping in terms of the duration of peak yield. Typically, Group A streams (e.g., a; Bow at Banff), have at least two months of high flows persisting in some cases up to three months (60 to 90 days). What differentiates Group A from Group B is not so much the duration of the average peak yield period, but the consistent annual pattern. The large year-to-year variability shown for Group B streams (e.g., b; Rose Creek near Alder Flats) is never seen in Group A streams. For this metric, Group C streams show similar year-to-year variability as Group B streams, but the duration is significantly less, ranging from 30 to 60 days. The results for all sites in this study area are summarized in .
Population Proportion to Annual Total Flow
These metrics () demonstrate that the 0.05% threshold is descriptive only at the extremes of average annual yield. At the 1% threshold no pattern is evident across the range of gauging sites. When the proportion of population of daily flow values in excess of 2% of the annual flow threshold is used, the results are similar to other metrics such as the ratio of the range in annual yield to the average annual yield and last quartile ratios.
Dry or Zero Flow Occurrences
Where the average number of zero flow days per season was one day or more, dry or zero flow occurrences () shows no correlation to the proportion of the record with completely dry years; this was unexpected. Examples such as Sage Creek at Q Ranch show zero flow on average for 75% of the March to October season, yet the 30 year period from 1980 to 2009 only experienced one year where no flow occurred for an entire year. This site is contrasted by Lyons Creek at International Boundary, which on average has 89% zero flow days per season, yet had 12 totally dry years during that time. From this review, it appears that the number of dry days per year is not a predictor of the occurrence of totally dry years.
Last Quartile Ratio
From the examples of monthly runoff quartiles (), the differences in peak flows throughout the period of record are shown. The quantiles for the Bow River at Banff ( a) demonstrate the strong seasonal pattern of a nival watershed and the general consistency of monthly flows, where all quantiles demonstrate the same seasonal pattern. Rose Creek ( b) also shows a strong seasonal pattern; however, this is generally reflected in only the higher quantiles. For the Antler River ( c), the seasonal difference is only observed in the maximum values, reflecting the large variability of these systems. The last quartile ratio (LQR) is a metric that demonstrates this characteristic. The ratio of the difference between the maximum mean monthly discharge and the third quartile to the difference between the third quartile and the minimum of the population of mean monthly discharge values gives a metric relating the difference between the normal range of flow and what might be considered more severe or rare variations.
contains four different perspectives on this metric. The table compares the March to May average LQR, the range in LQR for the March to May period, the minimum non-zero LQR and the maximum LQR, both for the March to May period. Regardless of how the LQR is determined, there appears to be a distinct correlation with average annual runoff. At the high yield end of the spectrum, the ratio is generally less than a factor of 2; streams at the lower end of annual runoff (less than 15 mm/year) range from 10 to 500 times the amount for streams with average annual yield values exceeding 500 mm/year.
Discussion
Streams on the Canadian Prairies respond to localized surface water inputs (i.e., precipitation and snowmelt) and average yields can be considered to be proportional throughout the basin at a decadal scale. However, the proportionality for Group C sites, which are all situated in the grasslands, was not consistent from one year to the next. Significant periods of time occurred when these streams were dry and the duration of dry periods was unrelated to the areal extent of the drainage basin. These findings indicate that the hydrologic practitioner should be cautious when considering potential yields based on drainage area alone for grasslands streams unless one is making general statements for time period in excess of 10 years. Because of this large variability, practitioners should consider the attributes of a large number of sites rather than relying only on nearby stations. Furthermore, closely proximal gauging sites may not be comparable and care should be taken when using one to fill or extend data for another. The hydrologist should consider native and exotic sources for the flow in prairie streams, and that proximity alone may be an inappropriate parameter when attempting to in-fill missing records, extend a data set, or estimate flows at ungauged locations.
Hydrometric sites that make up Group B have an average annual runoff in the range of 15 to 300 mm/year; and while periods of zero flow were observed, these streams all had flow at some time during the year. Group C streams had an average annual runoff of less than 15 mm/year, and these streams may have entire years where no flow is observed.
All RHBN streams south of 54N on in the Prairie Provinces exhibit a relationship between the logarithm of mean annual discharge and the logarithm of drainage basin area (
a). This relationship has been described previously (Dawdy, Citation1961; Rodriguez-Iturbe et al., Citation1992; de Vries et al., Citation1994; Emerson et al., Citation2005). Classically, this is shown as the relationship in Equation
Equation 2
:
Re-writing this in terms of the logarithms:
where Q is mean annual discharge (dam3), A is basin area (km2), and the parameters k and c are, respectively, the intercept (or offset) and the slope of the logarithmic functions. For streams that contribute flow proportionally to their drainage area, the parameter c will be equal to unity and k would be the mean annual runoff (mm) averaged for the group by the linear regression that produced the best fit line. Galster et al. (Citation2006) suggest that k is often not illustrative of watershed processes. However, for the streams in the Prairie Provinces, the parameter k is an important indicator of stream group and varies in a tangible way. This is demonstrated in
a, where the parameter c is indicative of the slope of the line, and the parameter k is the intercept of mean annual discharge if gross drainage area is equal to unity.
a demonstrates that the parameter k varies greatly by stream type and is in the order of magnitude of the mean annual runoff for the individual groups. This is to be expected, as division of the intercept in dam3 by 1 km2 would result in units of millimeters. Following from Equation
Equation 3
, if the gross drainage area is equal to 1 km2, Q would be equal to k. Whitfield and Spence (Citation2011) suggest that differences in the power law relationship between mean annual discharge and basin area is highly influenced by hydrologic process; here, it could be interpreted that this could also be used to differentiate the streamflow regime.
This separation by stream grouping also exists for annual runoff ( b). Whitfield and Spence (Citation2011) suggest that hydrologic process is responsible for the displacement by magnitude in these stream types. The results in and suggest that there are groupings of characteristics rather than a continuum of responses in the hydrometric network. It is important from the perspective of predicting flows in ungauged basins to consider where the stream in question may fit within a distinct group of gauged sites, as opposed to a large continuum of all sites. Some additional research is needed to demonstrate if there is a continuum of the relationship between runoff and drainage area or if there are three distinct groupings. In other words, are there other types of streams that lie between these groups and are not observed, or are there really three distinct groupings?
The examples provided in , , and are typical of the 27 Prairie Province RHBN sites south of 54N considered here. These figures support the proposition that the Prairie RHBN sites can be categorized into three separate stream types. No efforts were made to select by category or classification of streams when the RHBN data set was established. As Brimley et al. (Citation1999) explain, no process was identified to segregate the RHBN network by stream characteristic, rather the selection criteria were based on subjective measures of alteration of landscape or hydrology that may impact basin yield (quantity, duration, seasonality, etc.), and data characteristics such as record length.
In , the annual runoff (annual volume in dam3/gross drainage area in km2) was accumulated over the period of record. For Group A (streams such as the Bow River at Banff), mass curves are typically straight lines with small deviations from the mean line from year-to-year. These streams are the most robust of all streams in the Canadian Prairie Provinces in terms of both the magnitude and the certainty that there will be flow. As demonstrated by the slope of these mass curves, the range in magnitude of average annual yield for Group A streams is generally an order of magnitude higher than Group B; Group B streams being again an order of magnitude higher than Group C. The mass analysis diagrams for Group A streams demonstrate relatively consistent flow for each year in the period of record, with only small variance from the average line being apparent. The departure of individual annual totals from this mean line increases in both magnitude and frequency of occurrence, progressing from Group A, to B, to C streams.
This increasing magnitude of departures from the average condition is a characteristic difference in these stream types and represents the presence of discreet and successive extreme annual totals. In c, Antler River near Melita, dry periods show up as near flat lines for the periods 1956 to 1968, 1988 to 1994 and 2005 to 2009. Similarly, this plot shows extreme high flow volume years as vertical jumps in the line, for example, during the periods 1973 to 1976 and 1994 to 2005. Such periods of discontinuity are not present in Group A streams; they are obvious yet rare in long term records in Group B streams and they occur commonly in Group C streams.
In , additional characteristics of the three streams groupings are demonstrated. In the Group A stream example, Bow River at Banff ( a) there are no zero flow conditions and no flat spots on the curve, demonstrating flow throughout the year. Both Group B and Group C streams have periods of zero flow resulting in periods of flat lines in the annual cumulative flow series ( b and c). Also distinctive in are the spring and fall change points. For Group A streams, the change points rarely vary by more than a few weeks; for Types B and C the change points can vary by months; distinct between Group B and Group C is the time between change points for any single year, which is generally much shorter for Group C due to the significantly smaller volumes of water and less certain sourcing that Group C streams exhibit. This is likely due to the large variability in annual runoff generation as demonstrated by the range in annual runoff.
also shows that the range in annual yield for Group A streams is about equal to the average annual total. This statistic is reproduced for all streams in the study in . For Group A streams the average annual yields are approximately equal to the ranges, for Group B streams the ranges in yield are about twice the average, and for Group C streams the observed ranges are five to ten times the average.
In , two specific characteristics are apparent. First, the relative departure between Q4 and Q3 as compared to Q3Q0 increases as stream type changes from Groups A to B to C. For Group A streams, the Q0 through Q4 for the period from May to September in arithmetic scale can easily be identified. In the Bow River at Banff ( a), the departure between the maximum monthly values (Q4) and the 3rd quartile (Q3) is about equal to the remaining range. This ratio doubles for the Group B stream example, Rose Creek at Alder Flats ( b), i.e., the ratio of the difference between Q4 and Q3 is twice the difference between Q3 and Q0. For the Group C stream example, Antler River near Melita ( c), the Q4 to Q3 difference is about 10 times the Q3 to Q0 range.
In general, the results of this examination of RHBN sites suggest that Group C, which are predominately grasslands streams, as a rule have little to no flow except for a brief window of time in most years (). The flow for these streams varies between years with respect to both its timing and magnitude, and in some years no flow is observed. The pattern of flow also varies widely between streams within any one year, indicating the importance of localized sources of water. Streamflow sourced from snowmelt is not consistently evident in the record. This could be due to the quantity of accumulation or perhaps the possibility that melt water in the stream had occurred and passed the gauge prior to the start of seasonal gauge operation (generally the 1st of March each year). For the example of Antler Creek near Melita, the difference between the minimum total annual runoff (0 mm) and the maximum total annual runoff (58 mm) compared to the difference between the average (7 mm) and the maximum is small. Group B streams have flow in most years and annual runoffs are variable (); every year there is a likelihood that some period of zero flow will occur. Flows accumulate over a wide period of time each year, beginning with the start of snowmelt, and summer precipitation extends the period when flow occurs. In the example for Rose Creek at Alder Flats, the range between maximum and minimum annual runoff is 260 mm, while the difference between the average and the maximum is only 161 mm. A distinction between Group B and Group C streams is that when looking at the collective group members, the difference between the maximum annual yield and the average annual yield is, in general, a factor between 2 and 3 for Group B streams; this metric can range up to a factor of 10 for Group C streams ().
Group A streams reviewed in this study are all Rocky Mountain streams and are distinctly different than the Group B and Group C prairie streams; Group A streams in this study do not have zero flow days in the record; seasonal snowmelt freshet occurs over an extended period, and some have late summer glacial contributions. While the range between maximum and minimum total annual runoff is generally about a factor of 2 for Group A streams, the more consistent flow demonstrated for streams in this group place the annual average runoff in about the middle of the range, which is not the case for individual streams within Groups B or C. The maximum annual runoff for individual Group A streams compared to the average annual runoff for individual group members is generally a factor ranging from 0.5 to 1.5.
This approach demonstrates that streamflow characteristics are determinable from the hydrometric record. The basin response can be identified from the streamflow record itself with less reliance on physiographic characteristics. While, physiographic characteristics should not be discounted, the hydrometric record has a demonstrated value in our understanding of the long-term variation in stream response that should also be included in a stream classification. Metrics such as those generated here could be produced as part of the national database for reference and may benefit many decision makers who rely on streamflow record for its many uses, e.g., flow forecasting, stream crossing, water licensing, flow apportionment, habitat studies, resource development, etc. The approach presented has merit for other geographic areas and future work should include a review of monitored sites across Canada with a view to creating a national stream classification.
Native prairie streams have highly variable characteristics, most related to the high frequency of low or zero flow in the stream. Users who are addressing prediction in ungauged basins should respect the collective behaviour of these streams and not rely on nearby watersheds alone to make their predictions.
Conclusions
Understanding the history of hydrologic response of streams in the gauged network is fundamental to making valid estimates of runoff where gauged data are not available. This study demonstrated that it is possible to establish some defining characteristics of natural streams in the Prairie Provinces. The results presented here suggest that the hydrometric record for native prairie streams represent two distinct groups that have different hydrometric properties; clearly these are different from the sources of the mountain streams that flow into the Prairies from the west. Metrics presented that support three distinct stream types amongst the RHBN sites in the south of the Prairie Provinces include (): the last quartile ratio of monthly peak flow, the ratio of the range in annual yield to the average annual basin yield, the average number of days per year with flow greater than 2% of the annual total, the average number of zero flow days, and the average annual yield.
Generally speaking, natural flow sites on the Canadian Prairies are difficult to monitor and are under-represented on the landscape. The streamflow observed at these sites may be very sensitive to climate and weather, and may be valuable indicators of climate variability. This study showed that including non-continuous (seasonal) data has value and that native prairie streams demonstrate dramatically different responses compared to other stream types; however, many investigators have discounted the use of these data because they does not meet their study requirements for continuous data. Clearly, these examples demonstrate the need to monitor at least a few prairie RHBN sites on a continuous basis so that other investigators are comfortable to include this large and important area of Canada's landscape in future analysis.
Acknowledgements
We would like to express our appreciation to the participants and colleagues at the Zeroflow workshop for their interest and encouragement in the issue of monitoring streams that periodically or frequently have no discharge. We greatly appreciate the comments and suggestions of two anonymous reviewers and the Associate Editor on a previous version of this manuscript.
References
- Brimley, B., J.-F. Cantin, D. Harvey, M. Kowalchuk, P. Marsh, T. M. B. J. Ouarda, B. Phinney, P. Pilon, M. Renouf, B. Tassone, R. Wedel, and T. Yuzyk. 1999. Establishment of the reference hydrometric basin network (RHBN) for Canada. Ottawa: Environment Canada, 41 pp.
- Burn , D. H. , Buttle , J. M. , Cassie , D. , MacCulloch , G. , Spence , C. and Stahl , K. 2008 . The processes, patterns and impacts of low flows across Canada . Canadian Water Resources Journal , 33 : 107 – 124 .
- Buttle , J. M. , Boon , S. , Peters , D. L. , Spence , C. , van Meerveld , H. J. and Whitfield , P. H. 2012 . An overview of temporary stream hydrology in Canada . Canadian Water Resources Journal , 37 ( 4 ) : 279 – 310 .
- Dawdy, D. R. 1961. Variation in flood ratios with size of drainage area. U.S. Geological Survey Professional Paper 424-C, C36. Washington: U.S. Geological Survey.
- de Vries , H. , Becker , T. and Eckhardt , B. 1994 . Power law distribution of discharge in ideal networks . Water Resources Research , 30 ( 12 ) : 3541 – 3543 .
- Ehsanzadeh , E. , Spence , C. , van der Kamp , G. and McConkey , B. 2012 . On the behaviour of dynamic contributing areas and flood frequency curves in North American Prairie watersheds . Journal of Hydrology , 414415 : 364 – 373 .
- Emerson, D. G., A. V. Vecchia, and A. L. Dahl. 2005. Evaluation of drainage-area ratio method used to estimate streamflow for the Red River of the North Basin, North Dakota and Minnesota. U.S. Geological Survey Scientific Investigations Report 20055017. Washington: U.S. Geological Survey, 13 pp.
- Environment Canada. 2011. HYDAT Surface water and sediment data. Ottawa: Environment Canada, Water Survey of Canada. http://www.wsc.ec.gc.ca/applications/H2O/index-eng.cfm (accessed September 2011).http://www.wsc.ec.gc.ca/applications/H2O/index-eng.cfm
- Fang , X. and Pomeroy , J. W. 2009 . Modelling blowing snow redistribution to prairie wetlands . Hydrological Processes , 23 : 2557 – 2569 .
- Galster , J. C. , Pazzaglia , F. J. , Hargreaves , B. R. , Morris , D. P. , Peters , S. C. and Weisman , R. N. 2006 . Effects of urbanization on watershed hydrology: The scaling of discharge with drainage area . Geology , 34 : 713 – 716 .
- McGee , D. , Boon , S. and van Meerveld , H. J. 2012 . Impacts of rural water diversions on Prairie streamflow . Canadian Water Resources Journal , 37 ( 4 ) : 415 – 424 .
- Monk , W. A. , Peters , D. L. , Curry , R. A. and Baird , D. J. 2011 . Quantifying trends in indicator hydroecological variables for regime-based groups of Canadian rivers . Hydrological Processes , 25 : 3086 – 3100 .
- Rodrguez-Iturbe , I. , Ijjsz-Vsquez , E. J. , Bras , R. L. and Tarboton , D. G. 1992 . Power law distributions of discharge mass and energy in river basins . Water Resources Research , 28 ( 4 ) : 1089 – 1093 .
- Shook , K. R. and Pomeroy , J. W. 2011 . Memory effects of depressional storage in northern Prairie hydrology . Hydrological Processes , 25 : 3890 – 3898 .
- Stichling , W. and Blackwell , S. R. 1957 . Drainage area as a hydrologic factor on the glaciated Canadian Prairies . IUGG Proceedings , 111 : 365 – 376 .
- van der Kamp , G. and Hayashi , M. 2009 . Groundwater-wetland ecosystem interaction in the semiarid glaciated plains of North America . Hydrogeology Journal , 17 : 203 – 214 .
- Wagener , T. , Sivapalan , M. , Troch , P. and Woods , R. 2007 . Catchment classification and hydrologic similarity . Geography Compass , 1 : 901 – 931 .
- Whitfield , P. H. and Cannon , A. 2000 . Recent variations in climate and hydrology in Canada . Canadian Water Resources Journal , 25 : 19 – 65 .
- Whitfield , P. H. and Spence , C. 2011 . Estimates of Canadian Pacific Coast runoff from observed streamflow data . Journal of Hydrology , 410 : 141 – 149 .