Abstract
Potential shifts in extreme rainfall at the local level require revisions of existing water infrastructure management regulations as well as changes in design practices. This paper assesses the change in IDF curves for use by the City of London, Ontario under changing climatic conditions. A non-parametric K-Nearest Neighbour weather generator (WG) algorithm is used to synthetically create long time series of weather data. Nine daily maximum rainfall datasets (5, 10, 15, 30 minutes, 1, 2, 6, 12, and 24 hour) collected from the London Airport station for the period 19612002 are used as input into the WG. The WG uses sophisticated shuffling and perturbation mechanisms to generate synthetic rainfall records similar (but not identical) to the observed historic record. The shuffling mechanism generates synthetic rainfall values and the perturbation mechanism can generate extreme rainfall values outside the observed bounds to simulate plausible future rainfall amounts not yet observed. Two climate scenarios are selected for the analysis: climate change lower bound (CC_LB) and climate change upper bound (CC_UB). The CC_LB scenario uses observed rainfall record as WG input. The CC_UB scenario modifies the observed record using the results of a selected global climate model (change fields) and then uses the modified rainfall datasets as WG input. Outputs are used to generate IDF curves. Results of the study include numerical and graphical presentation of updated IDF curves for 2, 5, 10, 25, 50, and 100 years for the city of London, Ontario. The comparison between climate change scenario IDF curves and Environment Canada IDF curves indicates an increase in maximum rainfall intensities and magnitudes which has major implications on ways in which current (and future) municipal water management infrastructure is designed, operated, and maintained.
Les changements ventuels touchant les prcipitations extrmes au niveau local exigent des rvisions des rglements existants en matire de gestion de l'infrastructure de l'eau ainsi que des changements dans les pratiques de conception. La prsente communication value le changement dans les courbes IDF dont se sert la ville de London, en Ontario, dans des conditions climatiques changeantes. Un algorithme de gnrateur stochastique de conditions mtorologiques ou Weather Generator (WG) des k plus proches voisins, non paramtrique, est utilis afin de crer de manire synthtique des sries long terme de donnes mtorologiques. Neuf ensembles de donnes de prcipitations maximales quotidiennes (5, 10, 15, 30 minutes, 1, 2, 6, 12, et 24 heures), donnes recueillies la station de l'aroport de London pour la priode de 1961 2002, sont utiliss comme donnes d'entre pour le gnrateur. Le gnrateur fait appel des mcanismes perfectionns de rorganisation et de perturbation afin de gnrer des enregistrements synthtiques de prcipitations, semblables (mais non identiques) aux enregistrements historiques observs. Le mcanisme de rorganisation gnre des valeurs de prcipitations synthtiques et le mcanisme de perturbation peut gnrer des valeurs de prcipitations extrmes en dehors des limites observes afin de simuler des quantits de prcipitations futures plausibles non encore observes. Deux scnarios climatiques sont retenus pour l'analyse: la limite infrieure du changement climatique (LICC) et la limite suprieure du changement climatique (LSCC). Le scnario LICC fait appel des enregistrements observs en tant que donnes d'entre du gnrateur. Le scnario LSCC modifie l'enregistrement observ l'aide des rsultats d'un modle climatique mondial slectionn (les champs tant modifis) et utilise ensuite les ensembles de donnes de prcipitations modifis en tant que donnes d'entre du gnrateur. Les donnes de sortie servent gnrer les courbesIDF. Les rsultats de ltude comprennent la prsentation numrique et graphique des courbes IDF mises jour de 2, 5, 10, 25, 50, et 100 ans pour la ville de London, en Ontario. La comparaison entre les courbes IDF du scnario de changement climatique et les courbes IDF d'Environnement Canada fait tat d'une augmentation des intensits et de l'importance des prcipitations maximales, ce qui a des incidences majeures sur les diffrentes faons dont l'infrastructure actuelle (et future) de gestion des eaux municipales est conue, exploite et entretenue.
Introduction
Municipal water management infrastructure (e.g., sewers, storm water management ponds or detention basins, street curbs and gutters, catch basins, swales) designs are typically based on the use of local rainfall Intensity Duration Frequency (IDF) curves. The IDF curves are developed using historical rainfall time series data. Annual extreme rainfall is fit to a theoretical probability distribution from which rainfall intensities, corresponding to particular durations and frequencies are obtained. In this procedure, an assumption is made that historic extremes can be used to characterize extremes of the future (i.e., the historic record is assumed to be stationary). This assumption is not valid under changing climatic conditions that may bring shifts in the magnitude and frequency of extreme rainfall. With these changes, historic IDF curves cannot be used to accurately represent future climate conditions. Therefore, water management infrastructure designed to historic IDF standards may not be able to handle the additional load that may be the consequence of changing climate. In some cases, changing hydro-climatic conditions may also require upgrading, retrofitting, rebuilding, or the construction of additional water management infrastructure. Environment Canada (EC) provides IDF curves on their website that are released at intervals dependent on the availability of recent data and resources. These curves, however, reflect only historically observed rainfall; climate change is not explicitly incorporated into their IDF curves. A process is under way within EC to revise the IDF development methodology and consider potential impacts of climate change (Canadian Standards Association (CSA), Citation2010). As weather patterns shift, it is important to understand how local infrastructure may be affected, as extreme rainfall events have the potential to cause direct and indirect damages to communities. Therefore, design standards and codes should be developed using the most up to date knowledge and data. To reduce adverse effects of climate change and to prevent underperformance of critical infrastructure systems, it is necessary for stakeholders to understand potential climate change effects and develop appropriate adaptation strategies (Prodanovic and Simonovic, Citation2007). Changes must be made now to accommodate the future demands imposed by climate change.
The average temperature of Canada's climate is warming at a greater rate than initial projections suggested (Intergovernmental Panel on Climate Change (IPCC), Citation2007; CSA, Citation2010). Consequently, Canada may observe increases in atmospheric water vapour content and intensification of the global hydrological cycle. IDF curves are important in the design of stormwater and wastewater conveyance and storage infrastructure (CSA, Citation2010).
A number of Canadian studies have looked at methodologies used to develop future IDF curves using statistical downscaling methods with GCM outputs. Coulibaly and Shi (Citation2005) developed IDF curves for two regions within Ontario by downscaling daily GCM outputs data. IDF curves were generated for current (19612000) and future (20102039, 20402069, and 20702100) climates. In general, daily and sub-daily rainfall intensities were found to increase in most cases, with the possibility of increases up to 35% by the decade 2080. Vasiljevic (Citation2007) assesses changes to precipitation intensities for thirteen stations in Ontario. Based on a single site temporal IDF analysis, the majority of findings indicate that heavy rainfall intensity is changing. With respect to changes in rainfall intensity over time, no uniform pattern is identified for the study area, even though more increasing than decreasing trends are detected in temporal IDF curves for both annual and seasonal analyses. Cheng et al. (Citation2007) makes future estimates of climatic conditions by statically downscaling global circulation model outputs from global to local scales for five different models. The study indicates that Ontario could more frequently experience heavy rainfall events by the middle to late part of this century as a result of changes in the global climate. The study also projects increases in the number of days with measureable rainfall and increases in seasonal rainfall totals. Despite uncertainties and limitations in statistical downscaling methods, most regional IDF case studies are pointing towards an increase in extreme rainfall events for future climates (CSA, Citation2010). Return periods of design storms of a particular duration may occur more frequently under future climate.
The objective of this paper is to (a) provide a methodology for updating the IDF curves to reflect the changing climatic conditions; and (b) to use the developed methodology and perform the modification of IDF curves for the London Airport station for use by the City of London. Synthesis of the research findings is presented in the form of IDF curves, for two future climate scenarios defining the upper and lower limit of potential climate change for the region.
Methodology
This paper presents a methodology and a case study that assists in the assessment of impacts of climate change, specifically the intensity, duration and frequency of extreme rainfall events, on municipal infrastructure through the development and use of IDF curves. The implementation of the methodology is based on the simulation of synthetic rainfall for possible future climate scenarios, which is then used to develop the IDF curves for use in local stormwater management practices. By better understanding the demand of the municipal infrastructure under future climate scenarios, the community will be better prepared to deal with extreme rainfall events. The schematic diagram of the methodological procedure developed in this study is shown in , and includes the following steps: (i) selection of global climate change scenarios; (ii) preparation of rainfall data; (iii) implementation of the K-Nearest Neighbour (K-NN) weather generator (WG) algorithm; (iv) development of IDF curves; and (v) recommendations for the City of London to improve stormwater management design and practices.
Figure 1. Flowchart of procedure for the CC_LB scenario (shuffling and perturbing an unmodified historical rainfall record) and CC_UB scenario (shuffling and perturbing a modified historical rainfall record using climate change factors from GCMs). In both cases, synthetic climate records are created using the Weather Generator which are then used to produce intensity-duration-frequency curves for the lower (CC_LB) and upper (CC_UB) bounds for a range of potential climate impacts.
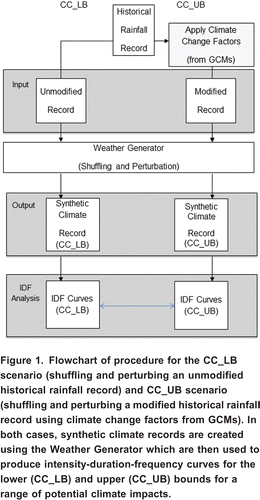
The present study considers a general methodology for constructing IDF curves considering climate change. Global Circulation Models (GCMs) are used to capture a range of potential change of climatic conditions in the future. The methodology incorporates the use of two climate change scenarios to represent the lower and upper bounds of future possible climate impacts in IDF analysis; the details of these scenarios are discussed further in this paper. These two climate scenarios form a range of potential future climate scenarios which illustrates the limitations and magnitude of uncertainty associated with the selection of, and subscription to, a single climate scenario. A stochastic Weather Generator (WG) was used to downscale GCM data for the region of interest. Historic and modified rainfall datasets were used as input into the WG model to synthesize future rainfall using a series of complex mathematical reshuffling and perturbation mechanisms based on the work of Sharif and Burn (Citation2007) and Prodanovic and Simonovic (Citation2007).
Global Circulation Models (GCMs)
Assessment of climate change impacts on a local scale incorporates projection of climate variables on a global scale and then downscaling of global scale climatic variables to the local scale. Global scale climate variables are commonly projected by Global Circulation Models (GCMs), which provide a numerical representation of climate systems based on the physical, chemical and biological properties of their components and feedback interactions (IPCC, Citation2001). These models are currently the most reliable tools available for obtaining the physics and chemistry of the atmosphere and oceans and for deriving projections of meteorological variables (temperature, precipitation, wind speed, solar radiation, humidity, pressure, etc.). They are based on various assumptions about the effects of the concentration of greenhouse gases in the atmosphere coupled with projections of CO2 emission rates (Nakicenovic et al., Citation2000).
It has been established that the accuracy of GCMs decreases at finer spatial and temporal scales; a typical resolution of GCMs ranges from 250 km to 600 km, but the need for IDF analyses conversely increases at finer scales. The representation of regional rainfall is distorted due to this coarse resolution and thus it cannot capture the subgrid-scale processes required for the formation of site-specific conditions. While some models are parameterized, details of the land-water distribution or topography in others are not represented. Studies have found that the models fail to predict the high variability in daily rainfall and do not accurately simulate present-day monthly rainfall amounts.
A number of techniques have been developed to enhance the information from GCMs in order to bridge the gap between the climate model outputs from global to local scale. To study the impacts of climate change at a local level, downscaling techniques are often implemented to integrate GCM outputs with local historical data (Cunderlik and Simonovic, Citation2007; Prodanovic and Simonovic, Citation2007). The method used in this study involved comprehensive comparative analyses of the GCM simulation results for various models and various emission scenarios. Through the comparative analyses of change in basic GCM output variables, the choice was made of the range of potential climate change impacts at the desired location. This range was captured through the selection of two climate scenarios to represent the upper and the lower bound of potential climate change impacts.
For the work in this paper, GCM output data for model CCSRINES was obtained from the Canadian Climate Impacts Group at University of Victoria, Canada (http://www.cics.uvic.ca). Time series data was selected for the grid cell containing the south-western Ontario region for the time slice of 20402069, thus representing average climatic conditions for the year 2050. Historic global circulation data, also from the University of Victoria, consisted of data for period of 19611990 and represent the baseline global data. Results of GCMs simulations were converted into climate change fields for precipitation on a monthly temporal scale. These change fields were computed using the global circulation data as the percent difference from the baseline case of monthly precipitation averaged for all years of output. The global climate information was integrated with the local data and then downscaled to the local scale by the use of a weather generator.
Climate Change Scenarios
Climate change scenarios are not intended to predict or forecast future climate, but are used to explore the possibilities of climate change impacts if development was to continue in a particular direction (e.g., population growth, increased greenhouse gas (GHG) emissions, increased development). Many potential scenarios are possible for the description of future conditions (Nakicenovic et al., Citation2000). Therefore, the final results of the climate impact analyses are directly dependent on the scenario selected for GCM simulations. In this study, an original and simple method was implemented to deal with the uncertainty resulting from the choice of emissions scenario. Two climate change scenarios were selected to define the range of potential impacts of climate change and are described as follows:
1. | Climate change lower bound (CC_LB) scenario. The CC_LB scenario describes potential changes in the climate in an environment where emissions of GHG have ceased. However, changes in climate will continue to be observed due to the longevity of GHGs existence in the atmosphere, even if mitigation measures are immediately introduced. The CC_LB scenario emphasizes current conditions and uses local observed rainfall datasets as input into the weather generator model. The WG shuffles and perturbs the data to generate a new rainfall record similar to the historical dataset. The WG perturbation mechanism provides the possibility for data to be pushed beyond the historically observed record to potentially produce extreme rainfall values not observed in the historic record. | ||||
2. | Climate change upper bound (CC_UB) scenario. The CC_UB scenario describes a potential future climate based on warmer and wetter conditions, characterized by increased magnitude of extreme rainfall events. The CC_UB scenario emphasizes future wet conditions and uses modified rainfall datasets as input into the weather generator model. CC_UB scenario was constructed by modifying the historically observed rainfall dataset with climate change fields produced from GCMs (). In this case, GCM CCSRNIES and B21 scenario was considered to represent the most critical future climate. The B21 emissions scenario is based on IPCC (Citation2001) story line B2; characterized by moderate increases population, economic development, and technological advancement. It represents the possibilities of what might happen if future development follows a certain course of action and its criterion for selection was based on the ability of the scenario to produce a range of future possible climates. |
Table 1. Change fields for CCSRNIES B21 scenario, used to modify historical rainfall records for the climate change upper bound (CC_UB) scenario (after Prodanovic and Simonovic, Citation2008).
Together, the CC_LB and CC_UB scenarios define the lower and upper bounds, respectively, of a range of potential future climates. It is important to note that the CC_LB and CC_UB scenarios are both equally likely to occur as future climates (Eum and Simonovic, Citation2009). This is also true for all of the climate scenarios between these two bounds.
Climate change fields are used for the CC_UB scenario to modify the original historical dataset based on GCM outputs for future potential climate conditions. The change fields for the CC_UB scenario are computed using the global circulation data as the percent difference from the baseline case of monthly precipitation averaged for all years of output (). The CC_UB scenario is formulated by multiplying the historic London Airport rainfall data with the monthly percentage change values. For example, if the change field for the month of January is +17.67%, then all January values in the historic record are multiplied by 1.1767; similarly, if the change field is 3.10% for December, all historic data is multiplied by 0.969. The modified records are then used as input into a stochastic WG model (Sharif and Burn, Citation2007; Prodanovic and Simonovic, Citation2007) that uses a mathematical algorithm to produce a sequence of synthetic meteorological data which would be expected under the different climate change conditions.
Weather Generator Model
Stochastic weather generators simulate weather data to assist in the formulation of water resource management policies. The basic assumption for producing synthetic sequences is that the past would be representative of the future. They are essentially complex random number generators, which can be used to produce a synthetic series of data. This allows the researcher to account for natural variability when predicting the effects of climate change. The modified K-NN weather generator (Sharif and Burn, Citation2007) was used in this study. In this version of the K-NN algorithm the historic resampled data was perturbed, and therefore generates data outside of the historically observed range. The use of perturbation mechanisms assumes that historic data (typically shorter record) does not capture extreme characteristics likely to be observed in longer records. The K-NN algorithm starts with randomly selecting the current day from observed precipitation dataset (of various durations) from the station of interest (London International Airport). A two week moving window was employed and days with similar characteristics to the current day were extracted from the record; these represent the set of potential neighbours. Distance between mean precipitation value for the current day and the potential set of neighbours was computed via the Mahalanobis distance metric, and was sorted from smallest to largest. Out of the sorted potential neighbour set, only the first K values are selected for further analysis, meaning that generated weather variable will be close (but not identical) to the current value. Using a resampling procedure, one among the potential neighbours was selected to represent the precipitation for the next day. For each variable, in this case precipitation values of various durations for multiple climate scenarios, a non-parametric distribution was fit to K nearest neighbours and an estimate was made of conditional standard deviation, , and bandwidth, . The conditional standard deviation is estimated from the K neighbours, while is calculated based on the work of Sharma et al. (Citation1997).
In this study, the use of historic precipitation for a single station, London International Airport, is used to represent the weather for the entire City of London region. This is a limitation to the approach, as there is high spatial variability of precipitation and use of historical data from a single station does not capture this variability. However, use of a single station was mandated by the City of London and was therefore adopted in this study.
The weather generator model, originally developed by Sharif and Burn (Citation2007), was modified in this work. The research performed in this study adopted a modified weather generation methodology to take into account available data of shorter durations. Nine daily maximum rainfall values for durations of 5, 10, 15, 30 minutes and 1, 2, 6, 12, and 24 hours were used as input into the weather generator. It is important to mention that the weather generator model needs complete datasets with as few missing values as possible to work effectively. Many of sub-daily elements contain a large percentage of missing data (in case of London approximately 17% of data is missing for durations shorter than 24 hours). The weather generator was therefore modified to incorporate use of sub-daily datasets containing missing values; the missing sub-daily values do not play a role in the selection of the nearest neighbours.
The global models are only able to produce monthly outputs with a high degree of accuracy. This is insufficient for the use at the local level where often there is interest in the frequency of occurrence of short-duration high-intensity events. Temporal downscaling of monthly global output must therefore be employed and shorter duration events be estimated. The use of monthly climate change ratio with these sub-daily datasets is therefore a limitation to the study caused by the fact that GCM outputs do not provide sufficient temporal resolution of daily or sub-daily data.
Weather generator simulations were performed using three different datasets: original, new and modified, described below. Each input dataset was used with two climate change scenarios in weather generator simulations. The CC_LB scenario simulation uses the three sets of observed data (without multiplying them by change fields), and shuffles and perturbs them using the algorithm presented above. The CC_UB scenario simulation on the other hand, modifies the historic data by applying change fields first, followed by shuffling and perturbation. The simulations for each climate scenario (CC_LB; CC_UB) were performed for 126 years (42 years of historic record simulated three times over), producing nine daily maximum rainfall elements for each of the three types of datasets.
IDF Curves Analysis
IDF analysis was used to capture the main characteristics of point rainfall for shorter durations. Such analysis provides an effective tool for statistically summarizing regional rainfall information, and is often used in municipal storm water management and other engineering design applications. The IDF analysis starts by gathering time series records of different durations. After time series data was gathered, annual extremes were extracted from the record for each duration (5, 10, 15, 30 minutes; 1, 2, 6, 12, 24 hours). The annual extreme data was then fit to a probability distribution in order to estimate rainfall quantities. The most widely applied probability distribution used in analysis of extreme rainfall statistics is the Gumbel (Extreme Value Type I) distribution. The Gumbel distribution is fit to the annual maximum series (of every rainfall duration) and is used by EC to calculate return periods for IDF curves (Environment Canada, Citation2008; CSA Citation2010) and was therefore adopted in this study. The Gumbel probability distribution has the following form (Watt et al., Citation1989):
Currently, EC IDF curves do not explicitly consider effects of a changing climate (EC, Citation2008). However, there is scientific evidence that the climate is changing. Statistical methodologies support an increase in extreme rainfall in the future in many areas of Canada (CSA, Citation2010). Even small changes in climate normals may have large impacts on infrastructure, health and biological systems (Solaiman and Simonovic, Citation2011). Short duration (sub-daily) rainfall events caused by thunderstorms can result in the most extreme rainfall intensities and infrastructure developed using historical IDF curves, especially those dependent on short duration designs, may be at increased risk of damage (CSA, Citation2010). Trends in precipitation vary widely across Canada so it is necessary to consider rainfall analysis at the local level using statistical downscaling methods. The following is the application of the methodology to the City of London, Ontario to produce regional IDF curves considering climate change.
Case Study Results
Study Area
The City of London is located in the Upper Thames River basin, south-western Ontario, Canada, between the Great Lakes Huron and Erie. The climate of south-western Ontario is in part moderated by the surrounding Great Lakes, which produce a milder climate compared to other areas of the country. Annual precipitation ranges from 750 mm to 1,000 mm, of which approximately 30% falls as snow. Severe thunderstorms are common in June and July across the region, although they may occur anytime from March to November (due to collision of colder Arctic air and warm, moist Gulf air). The methodology in this paper was applied to a single location within the basin, London Airport (Meteorological Services of Canada (MSC) ID: 6144475) in London, Ontario, Canada.
Rainfall Data
The weather generator (WG) used in this study was provided with nine daily maximum rainfall depth elements (5, 10, 15, 30 minute; 1, 2, 6, 12, 24 hours) from a single weather station (London Airport; MSC ID: 6144475) as input into the model. Rainfall data were from EC for the nine required elements at the London station for the period of 19612002. Information for the London Airport weather station is listed in , and the location is shown with respect to the Great Lakes in .
Figure 2. Meteorological station used in the study (London Airport, Meteorological Services Canada ID: 6144475) in the Upper Thames River Basin of southwestern Ontario, Canada.
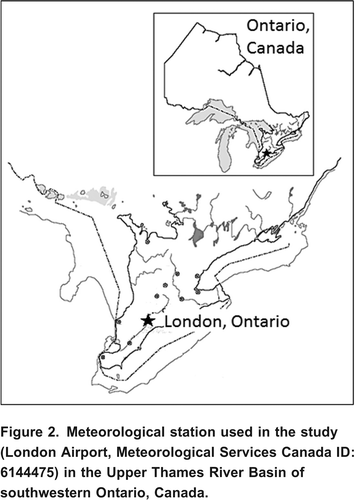
Table 2. Meteorological Service of Canada; London Airport rain gauge data.
Historical datasets for the City of London are available for the period 1961 2002 from EC; datasets prior to 1961 are not available for the City of London. The data elements used in the current study (and the resolution of this data) are listed in . These elements have a digital record dating back to 1961 and a paper record exists for some of the elements back to 1943. However, the paper records were not available for this study and therefore not included. Three datasets are used in this study (Simonovic and Peck, Citation2009), and used in the development of IDF curves: (i) Original dataset consisting of the nine daily rainfall maximums (5, 10, 15, 30 minute; 1, 2, 6, 12, 24 hours) provided by EC. The data elements are collectively referred to as DLY03. However, in the way these datasets were constructed, major rainfall events over 1 hour may not be well captured; this was especially the case for 12 and 24 hour events. These datasets extract maximum daily values for the nine elements from raw tipping bucket rain gauge data. These data are based on a calendar day and therefore split up larger events that cross days, which may be significant in the analysis of extreme rainfall events; (ii) New dataset consists of hourly data provided from EC for the period 19612002. This hourly raw data element is referred to as HLY03. New daily maximum datasets are created for the 2, 6, 12, and 24 hour elements using a moving window with the HLY03 dataset to capture events which cross day boundaries (i.e. past 11:59 pm). Rainfall events crossing the calendar day boundary were assigned to the day with the greater portion of the rainfall event volume. An algorithm is developed for the implementation of this procedure; and (iii) Modified dataset, which combines data from both datasets mentioned above. To overcome missing data in the records, 2, 6, 12, and 24 hour duration daily maximums are compiled by taking the maximum of either: (a) DLY03 for-the-day values for these durations, or (b) the maximum of the moving 2, 6, 12, and 24hr windows calculated from the HLY03 hourly rainfall observations. Study results presented in this paper include only the modified dataset.
Table 3. Nine Environment Canada rainfall elements (datasets) used as input into the weather generator model (after Simonovic and Peck, Citation2009).
The historical (for CC_LB scenario) and modified (for CC_UB scenario) rainfall datasets were input into a K-NN WG model to generate long records of plausible rainfall data based on locally observed rainfall patterns. Annual extremes data was extracted from these records for each duration and fit to a Gumbel Extreme Value I probability distribution function. IDF curves were produced for all three datasets: original, new and modified for the City of London, Ontario (Simonovic and Peck, Citation2009). These curves were compared with IDF curves produced by EC. Graphs were constructed for comparison of EC IDF curves with IDF curves for both CC_LB and CC_UB scenarios. As an illustrative example, the complete set of IDF curves for the modified dataset is provided in and and and . The EC IDF curves () are provided for comparison.
Figure 3. Graph of intensity-duration-frequency curves for the climate change lower bound (CC_LB) scenario. These lines are created by shuffling and perturbing historical observed rainfall records for the London Airport Station. Rainfall magnitude and intensity for a range of durations and return periods (labelled in the upper right hand corner) are provided.
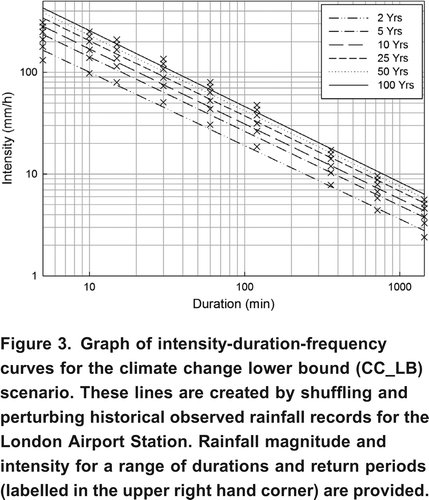
Figure 4. Graph of intensity-duration-frequency curves for the climate change upper bound (CC_UB) scenario. These lines are created by shuffling and perturbing modified historical rainfall records for the London Airport Station. Rainfall magnitude and intensity for a range of durations and return periods (labelled in the upper right hand corner) are provided.
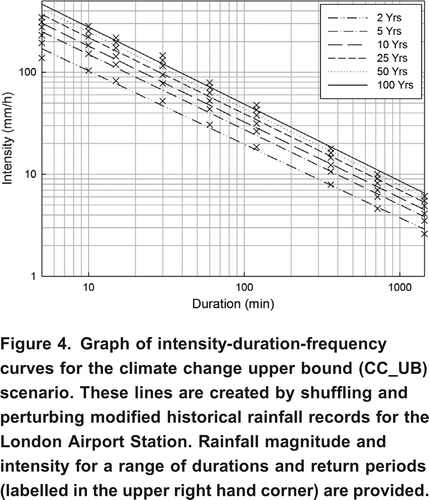
Figure 5. Graph of intensity-duration-frequency curves adapted from Environment Canada for the London Airport station. Rainfall magnitude and intensity for a range of durations and return periods (labelled in the upper right hand corner) are provided.
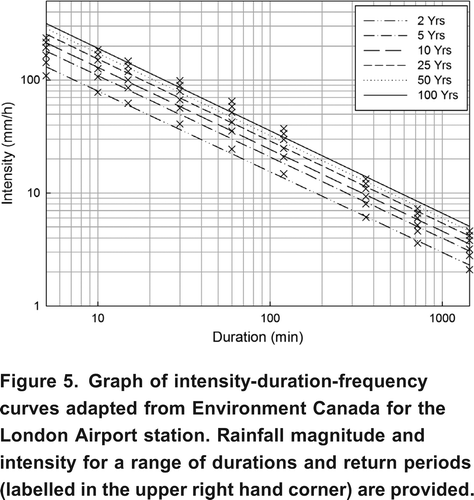
Table 4. Depth (mm) for CC_LB scenario for various return periods.
Table 5. Depth (mm) for CC_UB scenario for various return periods.
Review of the results indicates that rainfall magnitude will increase under climate change for all durations and return periods. In summary:
1. | the rainfall magnitude will be different in the future; | ||||
2. | the CC_UB scenario reveals significant increase in rainfall intensity for a range of durations and return periods for the City of London; and | ||||
3. | the increase in rainfall intensity and magnitude may have major implications on ways in which current (and future) municipal water management infrastructure is designed, operated, and maintained. |
The comparisons of updated IDF curves for climate change ( and ) with the IDF curves for London posted by EC () reveal that the CC_LB scenario maximum values are up to 35% higher than EC maximum values, while the CC_UB scenario produces maximum values up to 42% higher than EC, and up to 12% higher than the CC_LB simulation scenario for the 100 year short-duration rainfall events. shows differences between the 100 year LB, UB and EC IDF curves. Similar to findings of Prodanovic and Simonovic (2007, 2008), the rainfall intensity corresponding to the 100 year rainfall event in current climate is expected to occur more frequently in the future. An event of similar magnitude may now be expected to occur closer to once in every 30 years.
Study Recommendations
The work presented in this paper was commissioned and supported by the City of London as one of two activities associated with the development of climate change adaptation strategy for the City. Based on current data availability, the IDF curves developed in this study represent the best available knowledge at this time. The following are the main recommendations based on study results:
1. | Update. IDF curves contain data up to and including year 2002 and should be regularly updated as new data becomes available to reflect changes in the climate. | ||||
2. | Evaluate. The city of London should evaluate potential change of IDF curves in the range of about 20%, based on the comparisons between updated IDF historic climate change scenario and EC IDF curves. Detailed economic analysis should accompany this recommendation to justify the investment that this change would require. | ||||
3. | Revise. Comparisons between updated IDF curves with the modified dataset indicate small differences between upper and lower bound climate change scenarios (on average about 4.5%). Therefore the recommendation is to proceed with revisions to standards based on the lower bound climate change scenario. |
Conclusions
The methodology presented in this paper for constructing IDF curves considering climate change may be applied to other municipalities with appropriate GCM and emissions scenarios change fields for local conditions. The WG used in this study requires historical precipitation datasets with as few missing values as possible. The use of a single station with short or incomplete historical precipitation records may therefore limit the accuracy and applicability of the proposed methodology in some areas.
Currently, the City of London uses two different sets of IDF curves as standards for water management infrastructure design, operation and maintenance. Conveyance systems are designed based on curves provided by MacLaren (Citation1962), while most other storm water management facilities are designed using criteria provided by the City of London Sewer Design Standards (City of London, Citation2003). The IDF curves in use today for design of conveyance systems have been adopted from a study conducted in 1962, and were based on data from the 1950's for the Toronto area.
Results from this analysis suggest that these current IDF curves are not sufficient to represent future rainfall patterns that may occur with a changing climate. This study quantifies these changes. Their impact on design, operation and maintenance of municipal water management infrastructure (e.g., roads, bridges, culverts, drains, sewer, and conveyance systems) were assessed by Peck et al. (Citation2011). The results suggest revision of current IDF curves, as standards for water management infrastructure, to take into account potential impacts of climate change. New IDF curves represent the best available knowledge at this time but may be revised in the future to continue to best represent observed changes.
In the interest of effective stormwater management, the City of London has acknowledged limitations in the current IDF curves. After the completion of the presented study, the London City Council at their meeting of July 25, 2011 decided: to increase the current IDF curves by 21% in order to address the climate change (City of London, Citation2011).
Acknowledgements
Financial support for the study provided by the City of London is greatly acknowledged. The authors would like to thank Mr. Adam Van Ymeren for programming support and Mr. Robert Morris and Mrs. Joan Klaassen of Environment Canada for providing assistance with the data.
References
- Canadian Standards Association (CSA). 2010. PLUS 4013 Technical guide: Development, interpretation and use of rainfall intensity-duration-frequency (IDF) information: Guideline for Canadian water resources practitioners. Mississauga, Ontario: Canadian Standards Association, 185 pp.
- Cheng, C. S., G. Li, Q. Li, H. Auld, and D. MacIver. 2007. Climate Change and Extreme Rainfall-related Flooding and Surface Runoff Risks in Ontario Plain Language Summary Report, Climate Change Impacts and Adaptations Program Report no. A901. Toronto, Ontario: Environment Canada, 18 pp.
- City of London. 2003. City of London Sewer Design Standards. London, Ontario: Environmental and Engineering Services Department, 281 pp.
- City of London. 2011. Minutes of the Municipal Council Meeting, July 25, 2011. pp. 33, London, Ontario: http://council.london.ca/meetings/Council%20Minutes/2011-07-25%20Minutes/Council%20Minutes.pdf (accessed October 2011).http://council.london.ca/meetings/Council%20Minutes/2011-07-25%20Minutes/Council%20Minutes.pdf
- Coulibaly, P., and X. Shi. 2005. Identification of the effect of climate change on future design standards of drainage infrastructure in Ontario. McMaster University, Hamilton, Ontario: Department of Civil Engineering, 88 pp.
- Cunderlik , J. and Simonovic , S. P. 2007 . Inverse Flood Risk Modeling Under Changing Climatic Conditions . Hydrological Processes Journal , 21 ( 5 ) : 563 – 577 .
- Environment Canada. 2008. Weather and Meteorology, Notes on Environment Canada Rainfall Intensity-Duration-Frequency Tables and Graphs. Ottawa: http://www.ec.gc.ca (accessed September 2008).http://www.ec.gc.ca
- Eum, H., and S. P. Simonovic. 2009. City of London: Vulnerability of Infrastructure to Climate Change; Background Report 1- Climate and Hydrologic Modeling. Water Resources Research Report no. 68. University of Western Ontario, London, Ontario: Facility for Intelligent Decision Support, Department of Civil and Environmental Engineering, 103 pp.
- Intergovernmental Panel on Climate Change (IPCC). 2001. Climate Change 2001: Synthesis Report. A Contribution of Working Groups I, II, and III to the Third Assessment Report of the Intergovernmental Panel on Climate Change, eds. R. T. Watson and the Core Writing Team. Cambridge, United Kingdom: Cambridge University Press, 398 pp.
- Intergovernmental Panel on Climate Change (IPCC). 2007. Contribution of Working Groups I, II and III to the Fourth Assessment Report of the Intergovernmental Panel on Climate Change. eds. R. K. Pachauri and A. Reisinger. Geneva, Switzerland, 104 pp.
- MacLaren, J. F. 1962. Technical Discussion on Sewage and Drainage Works for Areas Annexed in 1961 by the City of London. Toronto, Ontario: MacLaren Associates, 23 pp.
- Ministry of Transportation Ontario (MTO). 1997. Ministry of Transportation of Ontario Drainage Management Manual. Ottawa, Ontario: Transportation Engineering Branch, Ministry of Transportation of Ontario, 1247 pp.
- Nakicenovic, N., J. Alcamo, G. Davis, B. de Vries, J. Fenhann, S. Gaffin, K. Gregory, and A. Gruebler. 2000. Special Report on Emissions Scenarios. Working Group III of the IPCC, Cambridge, UK: Cambridge University Press, 595 pp.
- Peck, A., E. Bowering, and S. P. Simonovic. 2011. City of London: Vulnerability of Infrastructure to Climate Change, Water Resources Research Report no. 074. University of Western Ontario, London, Ontario: Facility for Intelligent Decision Support, Department of Civil and Environmental Engineering, 66 pp.
- Prodanovic, P., and S. P. Simonovic. 2007. Development of Rainfall Intensity-Duration-Frequency Curves for the City of London under the Changing Climate, Water Resources Research Report No. 058. University of Western Ontario, London, Ontario: Facility for Intelligent Decision Support, Department of Civil and Environmental Engineering, 51 pp.
- Prodanovic, P., and S. P. Simonovic. 2008. Intensity Duration Frequency Analysis under Changing Climatic Conditions. In Proceedings of the 4th International Symposium on Flood Defence: Managing Flood Risk, Reliability and Vulnerability. Toronto, Ontario, Canada, May 68, 2008, 8 pp.
- Sharif , M. and Burn , D. H. 2007 . Improved K-Nearest Neighbor weather generating model . ASCE Journal of Hydrologic Engineering , 12 ( 1 ) : 42 – 51 .
- Sharma , A. , Tarboton , D. and Lall , U. 1997 . Streamflow simulation: A nonparametric approach . Water Resources Research , 33 ( 2 ) : 291 – 308 .
- Simonovic, S. P., and A. Peck. 2009. Updated Rainfall Intensity-Duration-Frequency Curves for the City of London Under a Changing Climate, Water Resources Research Report No. 65. University of Western Ontario, London, Ontario: Facility for Intelligent Decision Support, Department of Civil and Environmental Engineering, 64 pp.
- Solaiman, T., and S. P. Simonovic. 2011. Development of Probability Based Intensity-Duration-Frequency Curves under Climate Change, Water Resources Research Report No. 72. University of Western Ontario, London, Ontario: Facility for Intelligent Decision Support, Department of Civil and Environmental Engineering, 89 pp.
- Vasiljevic, B. 2007. Assessment of changes in precipitation intensities in Ontario; Master's Thesis. Guelph, Ontario: School of Engineering, University of Guelph, 122 pp.
- Watt, W., K. Lathem, C. Neill, T. Richards, and J. Rousselle. 1989. Hydrology of Floods in Canada: A guide to Planning and Design. Ottawa, Ontario: National Research of Canada, 245 pp.