Figures & data
Figure 1 Scheme of the biosynthetic pathway of eumelanins and pheomelanins. The activities of tyrosinase are indicated in the scheme. Moreover, the enzyme can oxidize DHICA to its o-quinone directly, or it can oxidize DHICA and DHI indirectly via the formation of o-dopaquinone. TRP2 (dopachrome tautomerase) or Cu2+ can participate in the evolution of dopachrome to DHICA. The oxidation of DHICA can be catalyzed by TRP1, (DHICA oxidase), tyrosinase or Cu2+. When glutathione or L-cysteine attack o-dopaquinone, glutathione-dopa or cysteinyl-dopa adducts are formed and these later evolve to pheomelanins Citation67.
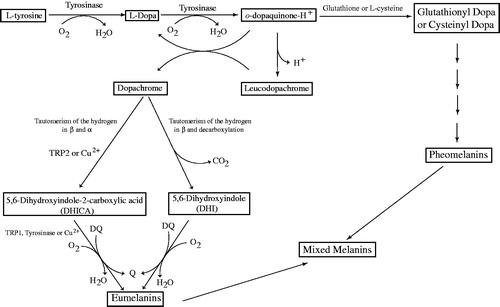
Figure 2 Monophenolase and diphenolase activities of Tyrosinase. EmM, met‐tyrosinase/monophenol complex; M, monophenol; D, o-diphenol; Em, met‐tyrosinase; EmD, met‐tyrosinase/o‐diphenol complex; Ed, deoxy‐tyrosinase; O2, molecular oxygen; Eox, oxy‐tyrosinase; EoxD, oxy‐tyrosinase/o‐diphenol complex; EoxM, oxy‐tyrosinase/monophenol complex; Q, o-quinone; Cr, Dopachrome.
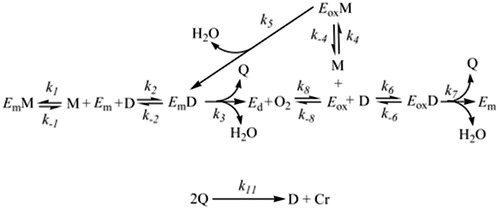
Figure 3 Detail of the structural mechanism proposed to explain the suicide inactivation of tyrosinase during its action on o‐diphenols. Em, met‐tyrosinase; Eox, oxy‐tyrosinase; EoxD, oxy‐tyrosinase/o‐diphenol complex; (Eox‐D)1, oxy‐tyrosinase/o‐diphenol complex axially bound to a Cu atom; (Eox‐D)2, oxy‐tyrosinase/o‐diphenol complex axially bound to the two Cu atoms; (Eox‐D)3, oxy‐tyrosinase/o‐diphenol complex axially bound to one Cu atom and the deprotonated hydroxyl group of C‐3; Ei, inactive form of tyrosinase. A general view of this scheme is shown in Ref Citation98.
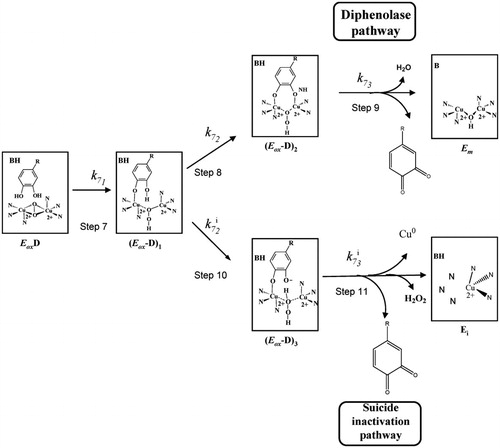
Figure 6 Inhibitory effects of the coumarins derivatives against mushroom tyrosinase activity: 3-aryl and 3-heteroarylcoumarins (1–10, 23–24), 3-hydroxycoumarin (11), 4-hydroxycoumarin (12), 6-hydroxycoumarin (13), 7-hydroxycoumarin (14), umbelliferone analogs (15–16), Esculetin (17) umbelliferone (18), 3-phenyl coumarins with bromo substituent (19), thiophosphonic acid diamides (20–22).
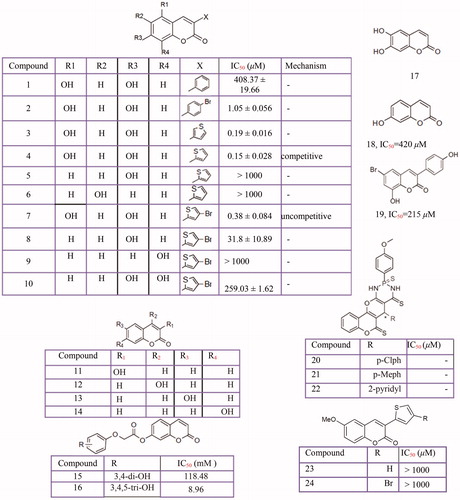
Figure 7 Tyrosinase Inhibition Activity of chalcone derivatives inhibitors: Oxindole-based chalcone (1–8), chalcones isolated from Morus australis (9–12) azachalcones (13–14), oxime based chalcone series (15,16) 2,3-dihydro-1H-inden-1-one chalcone-like derivatives (17,18), Dihydrochalcones from Flemingia philippinensis (19–21). chalcone (22).
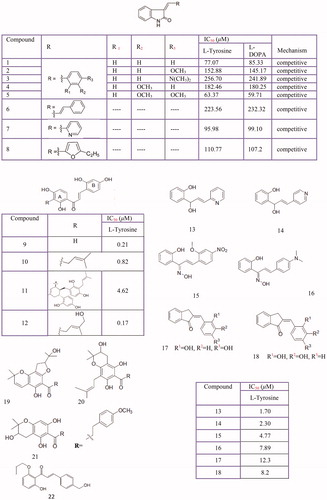
Figure 10 Inhibitory effects of some piperidine derivatives on mushroom tyrosinase activity. 4–(4-fluorobenzyl) piperidine derivatives (1–5) indole derivatives (6–13) amine (14) and N-ethyl (15).
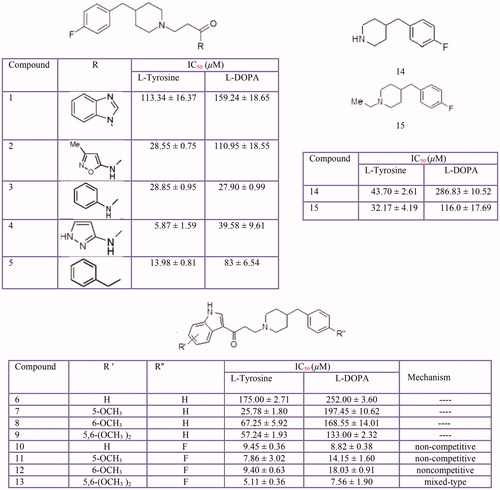
Figure 11 Inhibitory effects of some thiosemicarbazone derivatives on the tyrosinase monophenolase activity.
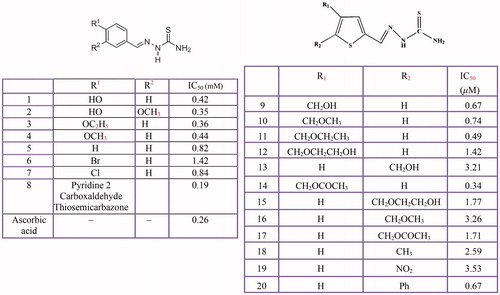
Figure 12 Thiourea derivatives (1–14), methimazole (15), carbimazole (16), thiouracil (17), methylthiouracil (18), propylthiouracil (19), 6–(3-chlorophenylurenyl) saccharin (20), 6–(3-iodophenylthiourenyl) saccharin (21), 4,5,6,7-tetrahydro- 2-[[(phenylamino)thioxomethyl]amino]-benzo[b]thiophene-3-carboxylic acid derivatives (22–25), 2–(1,3,4-thiadiazol-2-yl) thio acetic acid derivatives (26–29).
![Figure 12 Thiourea derivatives (1–14), methimazole (15), carbimazole (16), thiouracil (17), methylthiouracil (18), propylthiouracil (19), 6–(3-chlorophenylurenyl) saccharin (20), 6–(3-iodophenylthiourenyl) saccharin (21), 4,5,6,7-tetrahydro- 2-[[(phenylamino)thioxomethyl]amino]-benzo[b]thiophene-3-carboxylic acid derivatives (22–25), 2–(1,3,4-thiadiazol-2-yl) thio acetic acid derivatives (26–29).](/cms/asset/09c42d96-1d69-43f6-b5e2-460bcff8b112/ienz_a_1545767_f0012_c.jpg)
Figure 13 Thiadiazole derivatives: 1,3,4-thiadiazole derivatives (1–17) and thiazolidinones derivative (18–29).
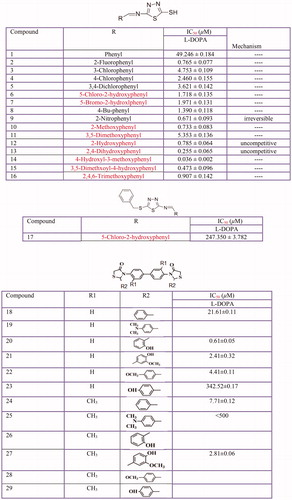
Figure 14 Some kojic acid analogs: hydroxybenzaldehydebased kojic acid analogs (5-substituted-3-[5-hydroxy-4-pyrone-2-ylmethylmercapto]-4-arylmethylamino-1,2,4-triazole (1–10) and 5-substituted-3-[5-hydroxy-4-pyrone-2-yl-methylmercapto]-4-arylmethyleneamino-1,2,4-triazole (11–14).
![Figure 14 Some kojic acid analogs: hydroxybenzaldehydebased kojic acid analogs (5-substituted-3-[5-hydroxy-4-pyrone-2-ylmethylmercapto]-4-arylmethylamino-1,2,4-triazole (1–10) and 5-substituted-3-[5-hydroxy-4-pyrone-2-yl-methylmercapto]-4-arylmethyleneamino-1,2,4-triazole (11–14).](/cms/asset/8420cdaf-df6f-4902-9ee1-38444229ad82/ienz_a_1545767_f0014_c.jpg)