Figures & data
Figure 1. Modular software architecture. Three distinct parts exist in the framework: a front-end integration environment (shown as a rectangle), an object repository (shown as a cylinder), and five modules (shown as ellipses).
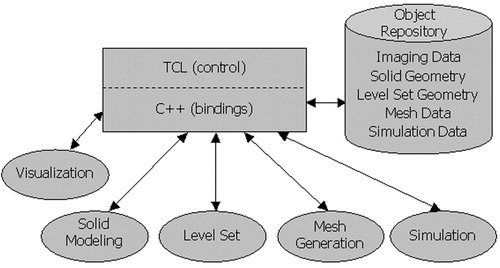
Figure 2. Overview of preoperative model construction. Initially, volumetric medical imaging data are acquired (a). Medial axis vessel paths are then extracted (b), followed by two-dimensional segmentation of the lumen boundary along each path (c). Solid models are then created for each vessel (d). Finally, the solid models representing the vessels are joined (Boolean addition) to create a solid model representing the flow domain (e). [Color version available online]
![Figure 2. Overview of preoperative model construction. Initially, volumetric medical imaging data are acquired (a). Medial axis vessel paths are then extracted (b), followed by two-dimensional segmentation of the lumen boundary along each path (c). Solid models are then created for each vessel (d). Finally, the solid models representing the vessels are joined (Boolean addition) to create a solid model representing the flow domain (e). [Color version available online]](/cms/asset/d6feb610-bd7f-4ea1-a02d-f525241f6148/icsu_a_123027_f0002_b.jpg)
Figure 3. The software system developed in this work was used by a vascular surgeon to create virtual surgical geometric models prior to the patient's actual surgery.
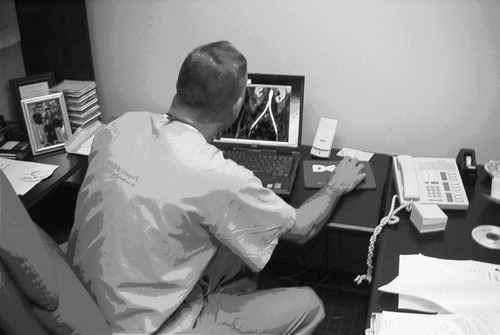
Figure 4. PCMRI slice plane acquisition locations for 67-year-old female. Seven planes of through-plane velocity information were acquired at the locations shown in the figure.
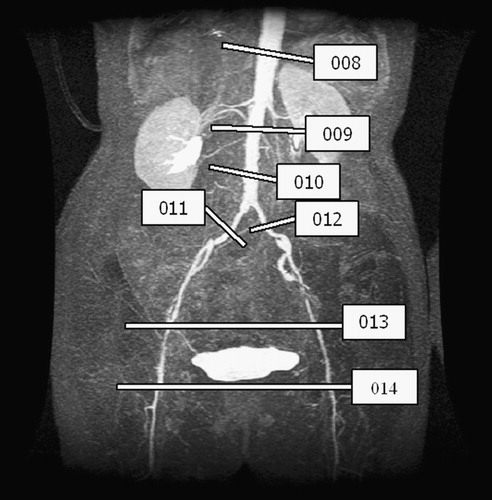
Figure 5. An example of the graphical user interface (GUI) of the system developed for surgical planning. The ‘Main Menu’ GUI guides a technician through the steps to go from medical imaging data to one- and three-dimensional hemodynamic simulation. The remaining windows in the figure are examples of controlling and running a one-dimensional analysis. [Color version available online]
![Figure 5. An example of the graphical user interface (GUI) of the system developed for surgical planning. The ‘Main Menu’ GUI guides a technician through the steps to go from medical imaging data to one- and three-dimensional hemodynamic simulation. The remaining windows in the figure are examples of controlling and running a one-dimensional analysis. [Color version available online]](/cms/asset/d42cc097-ee07-4886-9637-36c759e8b472/icsu_a_123027_f0005_b.jpg)
Figure 6. MIP of preoperative and postoperative MRA for a 67-year-old female. (a) The extent of the occlusive disease preoperatively (notice the tight stenosis in the patient's left external iliac) and (b) the bypass graft and the occlusion of the external iliac arteries postoperatively.
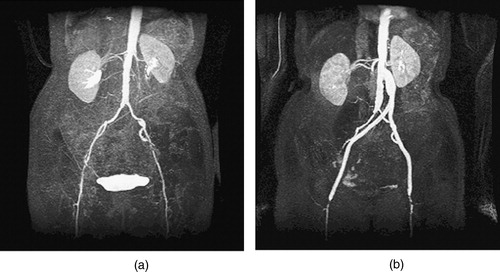
Figure 7. Two surgical alternatives for the 67-year-old female. (a) The preoperative model constructed prior to surgery. (b) A close-up of a high-grade stenosis in the left femoral artery. (c) The idealized endovascular repair (angioplasty and stenting), and (d) an aorto-femoral direct reconstruction using a Dacron bifurcating graft. [Color version available online]
![Figure 7. Two surgical alternatives for the 67-year-old female. (a) The preoperative model constructed prior to surgery. (b) A close-up of a high-grade stenosis in the left femoral artery. (c) The idealized endovascular repair (angioplasty and stenting), and (d) an aorto-femoral direct reconstruction using a Dacron bifurcating graft. [Color version available online]](/cms/asset/57a3413e-d2e3-4748-8169-b82373fa8fb5/icsu_a_123027_f0007_b.jpg)
Figure 8. Anatomic variability caused by vascular disease. The preoperative solid model is shown (in red) with three views created from the MRA data using thresholding. (a) The celiac trunk is not patent because of advanced vascular disease. (b) A dissection near the branch of the left internal iliac artery. (c) A tight stenosis that causes the vessel to appear disjoint because of the limitations in the image resolution and the threshold value selected. [Color version available online]
![Figure 8. Anatomic variability caused by vascular disease. The preoperative solid model is shown (in red) with three views created from the MRA data using thresholding. (a) The celiac trunk is not patent because of advanced vascular disease. (b) A dissection near the branch of the left internal iliac artery. (c) A tight stenosis that causes the vessel to appear disjoint because of the limitations in the image resolution and the threshold value selected. [Color version available online]](/cms/asset/4933b635-a290-4a6f-8a36-7ab964ba7243/icsu_a_123027_f0008_b.jpg)
Figure 9. Two different time points of the supra-celiac aorta acquired using PCMRI. During peak systole (a), the supra-celiac aorta is approximately 20 pixels across and the lumen boundary is clearly visible in the zoomed view of the magnitude of the gradient plot (b). However, during parts of diastole, the lumen boundary is not as clearly defined, as seen in (c) and the corresponding zoomed magnitude of the gradient plot (d).
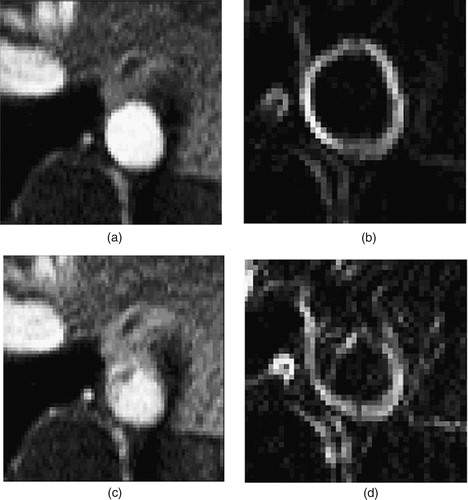
Table I. Mean volumetric flow rate, vessel cross-sectional area, and diameter from PCMRI data.
Table II. Mean volumetric flow rates from postoperative PCMRI data.
Table III. Iterations to calculate resistance values for three-dimensional simulation to match target mean volumetric flow distribution.
Figure 10. Rest and exercise supra-celiac flow waveforms for the 67-year-old female. The rest flow waveform was experimentally acquired using PCMRI. The exercise flow waveform was generated by shortening the diastolic portion of the rest curve by one-third and scaling the mean volumetric flow rate by a factor of two.
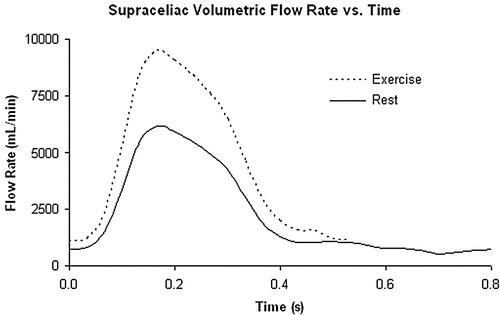
Figure 11. Exterior surface mesh for the model of the 67-year-old female patient. The mesh consists of 1.8 million tetrahedral elements.
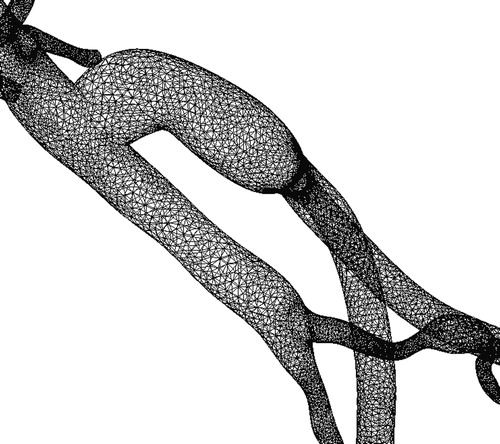
Figure 12. Mean wall shear stress for preoperative model. (a) shows the mean wall shear stress for the entire preoperative model. (b) shows a zoomed anterior view of the infra-renal aorta, while (c) shows a posterior view of the infra-renal aorta. Notice the region of low mean wall shear stress located just proximal to the aortic bifurcation, an area predisposed to atherosclerosis.
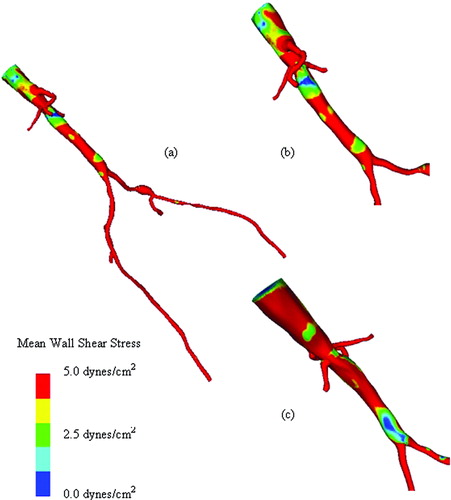
Figure 13. Mean wall shear stress for AFB model. Regions of low mean wall shear stress (shown in blue) are theorized to be sites of high risk for the development of atherosclerosis or neointimal hyperplasia.
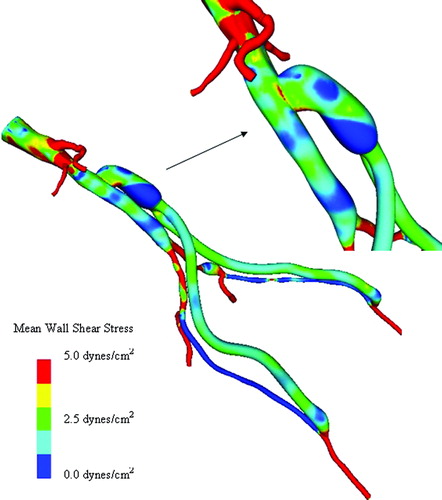
Figure 14. Scalar clearance time for AFB model. (a) The scalar field is initially set to 1.0 (shown in red) with a zero scalar Dirichlet boundary condition at the inlet. The scalar is then advected and diffused out of the flow domain over several cardiac cycles (where time is increasing from (a) until the final time point shown in (d)). The vessel walls have a scalar flux of zero.
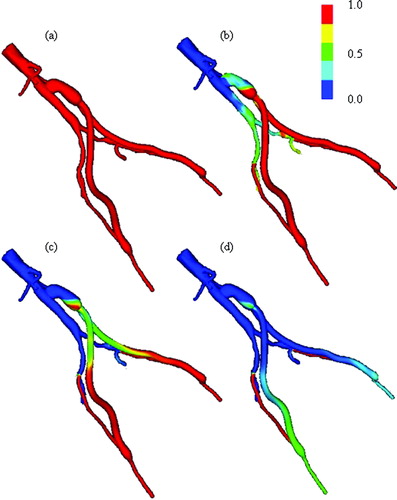
Table IV. Comparison of mean volumetric flow rates from three-dimensional simulations of rest and exercise.
Table V. Zero frequency mode of impedance boundary conditions at outlets for rest and exercise.
Table VI. Mean volumetric flow results from one-dimensional simulations.
Table VII. Pressure results from one-dimensional simulations.
Table VIII. Mean volumetric flow-rate differences between three-dimensional and one-dimensional simulations.
Figure 15. MIP of preoperative and postoperative MRA for a 55-year-old male. (a) shows the extent of the occlusive disease preoperatively (the tight stenosis in the left and right external iliac arteries), while (b) shows the bypass graft. Postoperatively, the distal native aorta and the external iliac arteries are occluded. The postoperative data were acquired 9 days after the surgery.
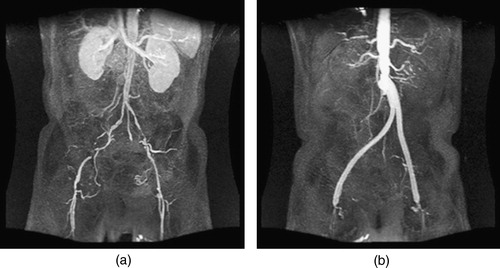
Figure 16. Views of the proximal anastomosis from the postoperative MRA dataset for the 55-year-old male. An isosurface of the postoperative MRA data set in the neighborhood of the proximal anastomosis is shown from two different angles. The distal native aorta occluded after surgery. [Color version available online]
![Figure 16. Views of the proximal anastomosis from the postoperative MRA dataset for the 55-year-old male. An isosurface of the postoperative MRA data set in the neighborhood of the proximal anastomosis is shown from two different angles. The distal native aorta occluded after surgery. [Color version available online]](/cms/asset/0a669cd0-a392-48ef-b57b-ceb4ceb6e0d9/icsu_a_123027_f0016_b.jpg)
Figure 17. Two bypass plans for 55-year-old male patient. (a) shows the preoperative model, (b) shows plan 1, and (c) shows plan 2. In (d), both plans are shown together to highlight differences between the two plans. [Color version available online]
![Figure 17. Two bypass plans for 55-year-old male patient. (a) shows the preoperative model, (b) shows plan 1, and (c) shows plan 2. In (d), both plans are shown together to highlight differences between the two plans. [Color version available online]](/cms/asset/24892999-b584-441c-80e6-9c88cf3ca556/icsu_a_123027_f0017_b.jpg)