Abstract
A pot experiment was conducted to investigate differences in antioxidative defence and carbon–nitrogen metabolism between acid-tolerant (YJSM) and acid-sensitive (YHSM) rice cultivars under acid stress. Acid-tolerant and acid-sensitive rice were planted in both acidic soil (pH 4.21) and normal soil (pH 6.13). Forty-eight days after sowing, rice shoots and roots from four treatments were collected, and the other four replicates were harvested at seed maturity. The results revealed that the grain yield of acid-tolerant YJSM was significantly higher than that of acid-sensitive YHSM under acid stress. The activities of antioxidant enzymes (superoxide dismutase and catalase) and contents of non-enzymatic antioxidants (ascorbate and reduced glutathione) of acid-tolerant YJSM were both higher than those of acid-sensitive YHSM under acid stress. Moreover, the enzyme activities (nitrate reductase, glutamine synthetase, glutamate synthase and glutamate dehydrogenase) and product contents (soluble sugar and soluble protein) of carbon–nitrogen metabolism of acid-tolerant YJSM were higher than those of acid-sensitive YHSM under acid stress. The NO3–N and carbon (C) contents in leaves of acid-tolerant YJSM were both significantly higher than those of acid-sensitive YHSM under acid stress. This study suggests that the acid-tolerant rice cultivar has better antioxidative defence and carbon–nitrogen metabolism systems than the acid-sensitive rice cultivar and is more effective in resisting acid stress.
Abbreviations:
SOD | = | superoxide dismutase; |
POD | = | peroxidase; |
CAT | = | catalase; |
MDA | = | malondialdehyde; |
GSH | = | glutathione; |
ASA | = | ascorbate acid; |
NR | = | nitrate reductase; |
GS | = | glutamine |
1. INTRODUCTION
Soil acidification is a major problem for crop production in many countries, occupying a worldwide area of at least 17 million ha (Andriesse and Van Mensvoort Citation2005; Nordmyret al. Citation2008). Acid induces changes in the cellular biochemistry and physiology of crop and limits crop growth and production (Masuliliet al. Citation2010). Among several agricultural crops, rice is grown as a major crop under acidic soil conditions in China. Responses to acid stress of rice have been reported, and the results indicate that there are substantial differences in the yield and productivity of rice cultivars under acidic conditions (Yang et al. Citation2009). Many scientists have suggested that selection is convenient if the crop species possesses distinctive indicators of stress tolerance (Ashraf Citation2002; Munns Citation2002; Parvaiz and Satyawati Citation2008). Studies have been performed to determine rice response to acid stress by measuring plant yields under increasing acid stress and relating yield reduction directly to soil acidification. This method permits distinguishing of acid tolerant and acid sensitive plants and choosing of an appropriate planting pattern corresponding with the expected soil acidification. The method is practical, but it does not, however, explain the behaviour of plants under acid stress or why plants differ in acid tolerance.
Physiological parameters are able to supply more objective evidence in cultivar selection than agronomic criteria or visual assessment when screening for component traits of the physiological metabolic system (Ashraf and Harris Citation2004). The ability of plants to overcome the effect of acid stress and sustain its yield is related to the scavenging of acid-induced toxic oxygen species, such as hydroxyl, superoxides, peroxy radicals and so on (Lin et al. Citation2012; Fenget al. Citation2013). Antioxidative enzyme and non-enzymatic antioxidants are two major systems for plant removal of toxic oxygen species (Freeman et al. Citation2010). The extent of acid tolerance of plants is determined by the capacity of antioxidative enzymes and non-enzymatic antioxidants to suppress the toxic levels of superoxides, hydroxyl radicals and peroxy radicals at the cellular level. Despite a great deal of research into the acid tolerance of plants in relation to the free radical metabolism system, the differences in the antioxidant capabilities of acid-tolerant and acid-sensitive rice cultivars are still not well reported.
Acid soil contains a number of limiting factors of plant growth such as deficiency of essential minerals (phosphorus, calcium, magnesium) and toxicity of some metals (aluminium, manganese)(Robson and Foy Citation1990). In our experiment, aluminium toxicity is one of the major factors that limit plant growth. Previous study showed that the organic acid secretions are the major mechanisms of alleviating aluminium toxicity by plants (Ma Citation2000; Yang et al. Citation2011).When organic acids are released into the rhizosphere, they can effectively chelate the toxic Al3+ and prevent its entry into plant root (Li et al. Citation2000; Ma et al. Citation2001). Organic acids not only act as key components in mechanisms that plants use to cope with Al-toxicity at the rhizosphere but also as metabolites in carbon metabolism (López-Bucioet al. Citation2000). In fact, the secretion of organic acids is heavily dependent on the carbon and nitrogen metabolism system, because carbon and nitrogen metabolism system can supplement the organic product consumed in secreting of organic acids (Bénardet al. Citation2009; Borsaniet al. Citation2009). López-Bucioet al. (Citation2000) showed that an increase in root organic acid exudation has been correlated to nitrogen-fixing activity behaviour. Besides, plant stress theory suggests that stress-tolerant plants can not only supplement the carbon–nitrogen metabolism product consumed in resisting harmful changes but also can use the rest of the product to maintain normal growth and reproduction (Parida and Das Citation2005). Many studies have focused more on the secretion of organic acids of plants on strongly acid soils (Ma et al. Citation1997a; Zhenget al. Citation1998; Silva et al. Citation2001; Yu et al. Citation2012), but little attention has been paid to the role of carbon–nitrogen metabolism systems of plants in resisting aluminium toxicity.
Carbon and nitrogen metabolism are closely interlinked, and nitrogen is required to allow carbohydrates to be utilized for synthesis of metabolites (Fritz et al. Citation2006a; Nunes-Nesiet al. Citation2010). The control of carbon–nitrogen metabolism constitutes an extremely complex network, involving signals emanating from ammonium, nitrate and nitrogen-containing enzymes, such as nitrate reductase (NR), glutamine synthetase (GS), glutamate synthase (GOGTA) and glutamate dehydrogenase (GDH), as well as signals from metabolites such sugars, proteins and amino acids (Coruzzi and Zhou Citation2001; Fritz et al. Citation2006b; Miller et al. Citation2007). The energy and materials of carbon–nitrogen metabolism are the basic requirements for plant growth and resisting environmental stress (Scheibe Citation2004). The carbon–nitrogen metabolic and regulatory mechanisms utilized by plants to survive acid stress are still not well understood, especially the differences between an acid-tolerant and an acid-sensitive rice cultivars.
OryzasativaL. acid-tolerant cv. YJSM and acid-sensitive cv. YHSM were selected as the test materials from more than 30 rice cultivars. As it happens, the acid-sensitive rice YHSM is the offspring of acid-tolerant rice YJSM which have been mated with the high-yielding (normal soil) rice HFZ. Our aim was to analyse whether the acid treatment affects carbon–nitrogen metabolism (enzymes and products) and antioxidant (enzymes and non-enzymatic) systems, which play the key role in the supply of energy consumed in resisting stress and the oxidative stress defence, in acid-tolerant and acid-sensitive rice cultivars. Therefore, we hypothesized that acid-tolerant cultivar under acid stress would be less negatively affected at the antioxidant and carbon–nitrogen compared to acid-sensitive cultivar grown under the same acid condition.
2. MATERIALS AND METHODS
2.1 Materials and experimental treatments
A pot experiment was conducted in the greenhouse of the Institute of Agricultural Resources and Environment in Guangdong Academy of Agricultural Sciences in Guangzhou, China (23°12′ N, 113°35′ E), from 9 August to 19 November, 2013. The pot experimental soil was collected from Chonglou town, Taishan, China, typical acidic soil (Entisols). The chemical properties of the acidic soil were as follows: pH 4.21 (soil water ratio of 1:5), organic matter 21.7 mg kg−1, alkaline hydrolysis N 140.0 mg kg−1, Olsen-P 8.95 mg kg−1, available K 113.0 mg kg−1, exchangeable Al 7.4 cmol kg−1 and available Mn 21.2 mg kg−1. Rice seeds were obtained from the Rice Research Institute of Guangdong Academy of Agricultural Sciences.
Each pot was filled with 2 kg of soil and was fertilized with the following macroelements (g kg−1 soil): N 0.2, P2O5 0.15 and K2O 0.2, which were supplied in the form of CO(NH2)2, NH4H2PO4 and KCl, respectively. The microelements were supplied with 1 mL of Arnon’s microelement solution for each kilogram of soil. The pH 4.21 acidic soil in this experiment was taken out in the natural state from a low-yielding rice field to create an acid-stressed environment in this experiment. The addition of lime to theacidic soil increased its pH to the normal range, thereby creating a normal soil pH environment (pH 6.13) in the control treatment (Quanet al. Citation2004; Haling et al. Citation2010). The experiment was conducted using acid-tolerant rice YJSM and acid-sensitive rice YHSM grown in acidic soil and normal soil. Each treatment was replicated eight times, and the seedlings were thinned to two seedlings per pot 7 days after planting.
Forty-eight days after sowing, the rice shoots and roots from four treatments were collected and separated, immersed in N2 liquid immediately, and then stored at minus 80 degrees for further analysis. The other four replicates were harvested at seed maturity, and the grain yield was determined.
2.2 Analysis of antioxidant enzyme activities
Superoxide dismutase (SOD) activity was determined by measuring the inhibition of the photochemical reduction of nitro blue tetrazolium according to the method of Carteset al. (Citation2010). Fresh 0.5 g root samples were ground in 5 mL of 50 mM potassium phosphate (pH 7.8), and the homogenate was centrifuged at 10,000 × g for 15 min at 4°C. The 3 mL reaction mixture contained 13 mM methionine, 75 μM NBT, 0.1 mM EDTA, 2 μM riboflavin and 100 μL of enzyme extract. The reaction tubes were illuminated for 15 min, and the absorbance of the samples was determined at 560 nm. One unit of SOD activity was defined as the amount of enzyme corresponding to 50% inhibition of the NBT reduction. Non-illuminated and illuminated reactions without supernatant were used as controls.
Peroxidase (POD) activity was tested by monitoring the oxidation of tetraguaiacol at 470 nm for 240 s based on the method of Mora et al. (Citation2008). Fresh 0.5 g root samples were extracted with 5 mL of 50 mM potassium phosphate buffer (pH 5.5). The homogenate was centrifuged at 10,000 × g for 15 min at 4°C. The reaction mixture contained 1 mL of extraction buffer, 5 μL of 30% H2O2, 5 μL of guaiacol and 15 μL of supernatant. One unit of POD activity was defined as an absorbance change of 0.01 units/min.
Catalase (CAT) activity was estimated by monitoring the decrease in absorbance at 240 nm for 3 min (Zhang et al. Citation2012a). Briefly, approximately 0.5 g of rice root samples was ground in 5 mL of 50 mM potassium phosphate buffer (pH 7.0). The homogenate was centrifuged at 10,000 × g for 15 min at 4°C. The reaction mixture contained 50 mM potassium phosphate buffer, 10 mM H2O2 and 200 μL of enzyme extract. One unit of CAT activity was defined as the change in absorbance of 0.01 units per minute under assay conditions.
Malondialdehyde(MDA) content was assayed using the method of Han et al. (Citation2013). Briefly, 0.5 g of rice root samples was ground in 5 mL of 10% TCA, and the homogenate was centrifuged at 4000 rpm. The reaction mixture (2 mL supernatant and 2 mL 0.6% TBA) was measured at 532 nm and corrected for nonspecific turbidity by subtracting the absorbance at 600 nm and 450 nm.
2.3 Analysis of non-enzymatic antioxidants
Ascorbate acid (ASA) and glutathione (GSH) were both tested by the method of Bao (Citation2002). Fresh 1 g of root samples was extracted with 5 mL of 5% TCA, and the homogenate was centrifuged for 15 min at 10,000 × g. The reaction mixture contained 0.2 mL supernatant, 0.2 mL 150 mM NaH2PO4 (pH 7.4), 0.2 mL H2O2, 0.4 mL 10% TCA, 0.4 mL 44% H3PO4, 0.4 mL 4% 2,2-Dipyridyl and 0.2 mL 3% FeCl3. The absorbance was measured at 525 nm after 60 min of stabilization in a bath at 37°C, and the ASA content was calculated using a standard curve.
Then, 1 g of roots was homogenized with 5 mL of 5% TCA, and the homogenate was centrifuged for 15 min at 10,000 × g. The assay contained 0.25 mL of supernatant, 2.6 mL of 150 mM NaH2PO4 (pH 7.7) and 0.15 mL of DTNB. The absorbance was measured at 412 nm after 30 min of reaction in a bath at 30°C, and the GSH content was calculated based on a standard curve.
2.4 Analysis of carbon–nitrogen metabolism enzyme activities
For assessment of NR activity (Wang et al. Citation2008), 0.5 g of leaves was extracted with 4 mL of 0.025 M phosphate buffer (pH 8.7) containing 10 mM cysteine and 1.3 mM EDTA. The homogenate was centrifuged at 4000 rpm for 20 min at 4°C. The reaction mixture contained 0.4 mL of supernatant, 1 mL of 0.1 M KNO3 and 0.6 mL of 2.7 mM NADH. The concentration of nitrite produced by NR in the reaction mixture was determined by subtracting the value of the time 0 control. The activity of NR was expressed as μg of NO2− produced per hour and per gram of fresh weight.
The method of determining GDH activity was described by Zhang et al. (Citation2013). Approximately 1 g of rice leaves was ground in 3 mL of cold 0.2 M Tris-HCl buffer solution (pH 8.2). The homogenate was centrifuged at 12,000 × g for 20 min at 4°C. The reaction mixture contained 2.8 mL of reaction liquid (60 mM L-glutamate, 1.6 mM NAD+, 360 mM pH 8.2 Tris-HCl) and 0.2 mL of crude extraction solution. The OD value at 340 nm was recorded once per minute.
GS activity was assayed by the method of Tang et al. (Citation2013). A fresh 1 g sample of frozen rice leaves was homogenized in 3 mL of cold 0.05 M Tris-HCl buffer solution (pH 8.0) containing 2 mM MgSO4, 0.4 M sucrose, and 0.2 mM DTT. The homogenate was centrifuged at 12,000 × g for 20 min at 4°C. The reaction mixture contained 1.6 mL of 0.1 M pH 7.4 Tris-HCl buffer solution (80 mM MgSO4, 20 mM glutamate, 20 mMcysteic acid, 2 mM EGTA and 80 mM hydroxylamine hydrochloride), 0.7 mL of crude enzyme extraction solution and 0.7 mL of ATP. The mixture was incubated for 30 min at 37°C, and then 1 mL of reagent was added (0.2 M TCA, 0.37 M FeCl3, 0.6 M HCl). After centrifugation at 5000 g for 10 min, the supernatant was measured at 540 nm.
GOGAT activity was determined by the method of Lin and Kao (Citation1996). A fresh 1 g sample of frozen rice leaves was homogenized in 4 mL of cold 0.05 M Tris-HCl buffer solution (pH 7.6) containing 1.0 mM DTT and 1.0 mM EDTA-Na2. The homogenate was centrifuged at 12,000 × g for 20 min at 4°C. The reaction mixture contained 0.4 mL of 20 mM L-glutamine, 0.5 mL of 20 mM α-ketoglutaric acid, 0.1 mL of 10 mMKCl, 0.2 mL of 3 mM NADH, 0.3 mL of crude enzyme extract and 1.5 mL of 25 mMTris-HCl (pH 7.6). The absorbance was measured at 340 nm once per minute.
2.5 Analysis of carbon–nitrogen metabolism products
Soluble sugar and soluble protein concentrations were determined by anthronecolourimetry and Coomassie brilliant blue methods, respectively (Gao Citation2005). The free amino acids were measured by the ninhydrin method (Li Citation2000).
2.6 Analysis of NO3–N and carbon contents
The nitrate content was determined using the method of Li (Citation2000), and the total C content in leaves was determined using a carbon and nitrogen elemental analyser (Analytikjena Multi N/C 2100, HT 1300).
2.7 Statistical analysis
The data were statistically analysed with SPSS 12.0 software, and the mean values of each treatment were subjected to multiple comparisons using the LSD-test at the p < 0.05 level.
3. RESULTS
3.1 Yield and plant height of the two cultivars
As shown in , though acid stress significantly (p < 0.05) decreased the yields of the two cultivars of rice, YJSM (acid-tolerant) had a dramatically (p < 0.05) greater grain yield than YHSM (acid-sensitive) under acid stress. However, there was no significant difference of grain yield under normal soil conditions between the two cultivars, which indicated YHSM still had the ability to obtain high grain yield under normal soil conditions. This suggests that YJSM had a better acid tolerance than YHSM. In addition, the results also revealed that acid caused a significant decrease in plant height (), but there was no significant difference between the two cultivars.
Figure 1 Grain yield and height of the two rice cultivars under different growing soil conditions. Acidic soil and normal soil indicate that the samples that were collected from rice grown in acidic soil and normal soil, respectively. The bars indicate the standard error of the mean. The mean values for each treatment with different lowercase letters indicate significant differences by the LSD-test (p < 0.05 n = 4).
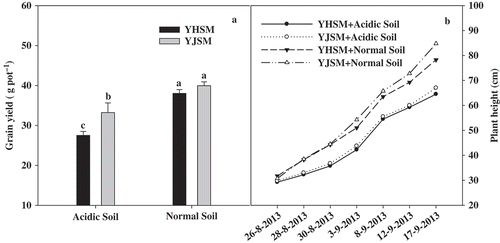
3.2 Antioxidant enzyme activities of the two cultivars
The MDA contents of acid-sensitive YHSM were higher than acid-tolerant YJSM in the roots under acid stress (), and there were no significant differences of MDA content in YJSM between the acidic and normal conditions, which indicate that YJSM had better capacity to prevent membrane peroxidation. The activities of three antioxidative enzymes, SOD, POD and CAT, are presented in . Comparing the activities of antioxidative enzymes in the roots of two cultivars under acid stress, the SOD activity of YJSM were 69.3% higher than that of YHSM. No significant differences were observed in root POD activities between the two cultivars under acidic or normal conditions. In regards to the antioxidant enzymes activities induced by stress, the activities of SOD, POD and CAT of the two cultivars under the acidic condition were higher than those under the normal condition.
Figure 2 The MDA content in the roots of two rice cultivars under different growing soil conditions. The acidic soil and normal soil indicate that the samples were collected from rice grown in acidic soil and normal soil, respectively. The bars indicate the standard error of the mean. The mean values for each treatment with different lowercase letters indicate significant differences by the LSD-test (p < 0.05 n = 4).
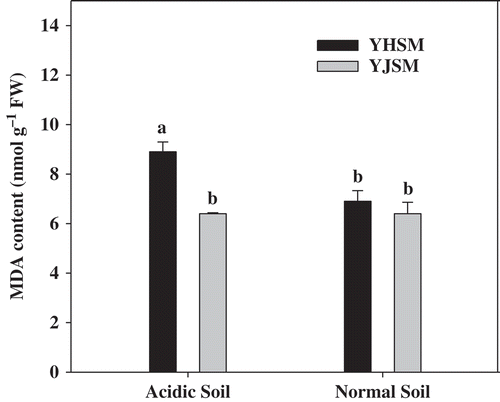
Figure 3 SOD, POD and CAT activities in the roots of the two rice cultivars under different growing soil condition. Acidic soil and normal soil indicate that the samples were collected from rice grown in acidic soil and normal soil, respectively. The bars indicate the standard error of the mean. Mean values for each treatment with different lowercase letters indicate significant differences by the LSD-test (p < 0.05 n = 4)
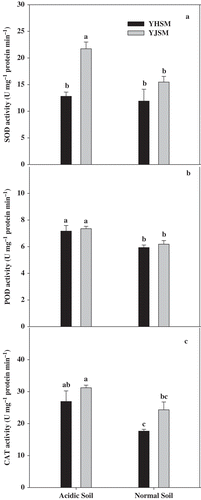
3.3 Non-enzymatic antioxidant content of the two cultivars
Comparing the non-enzymatic antioxidants contents in roots of the two cultivars under acid stress, the ASA () and GSH () contents of acid-tolerant YJSM were 83.6% and 29.0% higher than those of acid-sensitive YHSM, respectively. The same trends for the ASA and GSH contents also were observed in the roots of two cultivars under the normal condition. The ASA and GSH contents of YJSM were 56.0% and 60.3% higher than those of YHSM, respectively. These results indicate that YJSM has a better non-enzymatic antioxidant defence system than YHSM under stress and even in normal unstressed conditions.
Figure 4 ASA and GSH contents in the roots of the two rice cultivars under different growing soil conditions. Acidic soil and normal soil indicate that the samples were collected from rice grown in acidic soil and normal soil, respectively. The bars indicate the standard error of the mean. Mean values for each treatment with different lowercase letters indicate significant differences by the LSD-test (p < 0.05 n = 4).
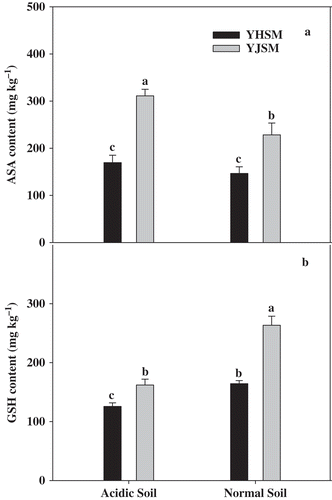
3.4 Carbon–nitrogen metabolism enzyme activities of the two cultivars
The NR activities of the two varieties were both significantly decreased under acid stress (), but the NR activity of the acid-tolerant YJSM was 76.7% higher than that of acid-sensitive YHSM in leaves under stress. The GS activity of YJSM was 31.3% higher than that of YHSM under stress (), but no significant difference was observed between the two cultivars under normal conditions. Comparing the GOGAT (b) and GDH () activities in the leaves of the two cultivars under acid stress, there were no significant differences between the two cultivars.
Figure 5 NR activities in the leaves of two rice cultivars under different growing soil conditions. Acidic soil and normal soil indicate that the samples were collected from rice grown in acidic soil and normal soil, respectively. The bars indicate the standard error of the mean. The mean values for each treatment with different lowercase letters indicate significant differences by the LSD-test (p < 0.05 n = 4).

Figure 6 GS, GOGAT and GDH activities in the leaves of the two rice cultivars under different growing soil conditions. Acidic soil and normal soil indicate that the samples were collected from rice grown in acidic soil and normal soil, respectively. The bars indicate the standard error of the mean. Mean values for each treatment with different lowercase letters indicate significant differences by the LSD-test (p < 0.05 n = 4).
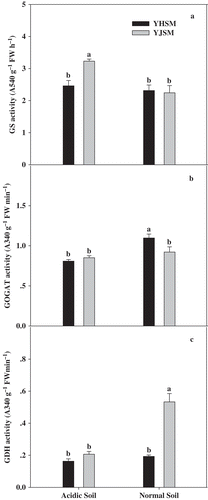
3.5 Carbon–nitrogen metabolism product contents of the two cultivars
Comparing carbon–nitrogen metabolism products contents in the leaves of two cultivars under acid stress, the soluble protein () and soluble sugar () contents of acid-tolerant YJSM were 17.1% and 25.6% higher than those of acid-sensitive YHSM, respectively. The opposite trend for free amino acid contents occurred in the leaves of two cultivars, and the free amino acid content of YHSM was 27.0% higher than that of YJSM (). These results indicate that acid-tolerant YJSM could transform more free amino acids into more important carbon–nitrogen metabolism products, such as soluble protein and soluble sugar.
Figure 7 Soluble protein, soluble sugar and free amino acid contents in the leaves of the two rice cultivars under different growing soil conditions. Acidic soil and normal soil indicate that the samples were collected from rice grown in acidic soil and normal soil, respectively. The bars indicate the standard error of the mean. Mean values for each treatment with different lowercase letters indicate significant differences by the LSD-test (p < 0.05 n = 4).
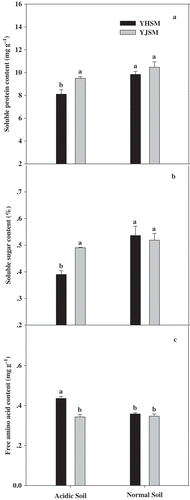
3.6 NO3–N and total C contents of the two cultivars
The NO3–N content in the leaves of acid-tolerant YJSM was significantly higher than that of acid-sensitive YHSM under acid stress (), but YHSM had a greater NO3–N content than acid-tolerant YJSM in the normal condition. Similarly, the total C content in the leaves of YJSM was significantly higher than that of YHSM under acid stress, whereas no significant difference was observed between the two cultivars under normal conditions ().
Figure 8 TheNO3–N and carbon (C) contents in the leaves of the two rice cultivars under different growing soil conditions. Acidic soil and normal soil indicate that the samples were collected from rice grown in acidic soil and normal soil, respectively. The bars indicate the standard error of the mean. Mean values for each treatment with different lowercase letters indicate significant differences by the LSD-test (p < 0.05 n = 4).
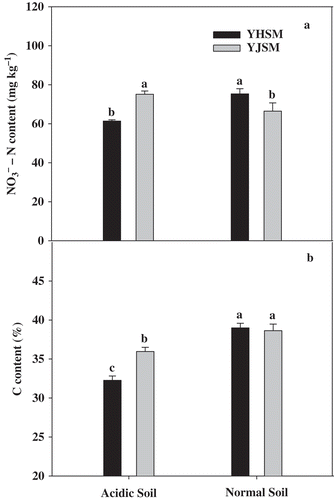
4. DISCUSSION
4.1 The acid-tolerant cultivar had a significantly higher grain yield than the acid-sensitive cultivar under acid stress
Aluminium toxicity is widely considered to be one of the major factor limiting crop growth and yield in strongly acid soils (pH < 5) (Ma et al. Citation1997b; Chen et al. Citation2013). Decline of grain yield of rice species in this experiment can also be attributed to aluminium toxicity in combination with low pH. Aluminium toxicity adversely affects plant growth and metabolism, and an increase in grain yield is widely used as an important indicator for aluminium tolerance in crops (Ma et al. Citation2001; Silva et al. Citation2010). Enhancing stress resistance in plants will directly improve their grain yield for grain crops (Cheong and Yun Citation2007). Therefore, one approach to increase grain productivity on acid soil is to develop crops that are more tolerant of aluminium stress. In our study, YJSM had a significantly greater grain yield than YHSM under acid stress, and there was no significant difference between the two cultivars in the normal condition (). This fact denoted that two cultivars had noticeable differences in their ability to tolerate acid, and the acid tolerance of YJSM was higher than that of YHSM.
4.2 The acid-tolerant cultivar had a superior antioxidative defence system than the acid-sensitive cultivar under acid stress
Previous studies opined that membrane injury by various environmental stresses including aluminium toxicity is related to an enhanced production of highly toxic oxygen-free radicals (Cakmak and Horst Citation2006; Meriga et al. Citation2010). It is widely recognized that the extent of oxidative stress is determined by the capacity of the plant antioxidative system to suppress the toxic levels of superoxides, hydroxyl radicals and peroxy radicals at the cellular level (Cui et al. Citation2010; Mroczek-Zdyrska and Wójcik Citation2012). Antioxidative enzymes such as SOD, POD and CAT are crucial for alleviating oxidative stress and enhancing stress tolerance in plants (Radwan Citation2012). The fluctuations in the level of lipid peroxidation because of the effect of the antioxidative system were accompanied by significant changes in the contents of MDA in rice cultured with acidic soil. When comparing the two rice cultivars, the MDA content in the roots of YHSM was significantly higher than that of YJSM under acid stress (). This result corresponds with the changes in the activities of SOD, and significant increase in the activity of antioxidative enzyme was observed in YJSM under acid stress, which is for the observed acid tolerance (). The benefits of the ability to increase the activities of antioxidative enzymes on plant growth have been often related to improvements in plant stress tolerance (Mora et al. Citation2008; Jiang and Yang Citation2009).
In addition, ASA and GSH, the non-enzymatic antioxidants, also play a key role in oxidative stress defence. ASA plays a crucial role in the protection of plants against the membrane lipid peroxidation resulting from aerobic metabolism and photosynthesis (Ahmad et al. Citation2010; Freeman et al. Citation2010). In association with other components of the non-enzymatic antioxidant system, GSH is also an important antioxidant, serving different functions, including H2O2 elimination and cell injury repair (Noctoret al. Citation2012). The protective role of non-enzymatic antioxidants against acid-induced oxidative stress was apparent when the results of ASA and GSH contents of two cultivars were compared. The ASA and GSH contents of YJSM were 83.6% and 29.0% higher than those of YHSM under acid stress, respectively (). These facts give reason to suppose that, in our study, YJSM has a stronger non-enzymatic antioxidant system. The increased antioxidative enzymes activities and non-enzymatic antioxidants contents in the acidic treatments may protect YJSM against oxidative damage under acid stress.
4.3 The acid-tolerant cultivar had superior carbon–nitrogen metabolism than the acid-sensitive cultivar under acid stress
The high H+ concentration in soils can result in proton toxicity that, in turn, can be exacerbated by the increase availability of Al3+. In consideration of previous evidence it showed that resistance to aluminium in plants is attributed to the release of organic acids by the root apex (Butareet al. Citation2012). The categories and relative contents of organic acid in plants are related to the activities of carbon–nitrogen metabolism (Carvalhaiset al. Citation2011). In addition, previous studies (Lópezet al. Citation2008; McDowell Citation2011) showed that carbon–nitrogen metabolism is closely involved in the physiological response to various stresses such as providing organic products consumed in resisting stress.Therefore, plants’ ability to resist stress is determined by multiple biochemical functions and maintaining high carbon–nitrogen metabolism activity is one of the most crucial features (Koyro Citation2006; Cag et al. Citation2009). The ability to coordinate carbon–nitrogen metabolism enables plants to regulate development and metabolic responses to different environmental stress. It is well known that there is a close relationship between carbohydrate accumulation and carbon–nitrogen metabolism ability. Increasing carbon–nitrogen activity can supplement the energy consumed in resisting harmful changes, and it is important that plants also have the rest of carbon–nitrogen metabolism products to maintain normal growth and reproduction (Parida and Das Citation2005; Rabinowitzet al. Citation2012). To accelerate carbon–nitrogen metabolism, plants need to improve the activities of enzymes, such as NR, GS, GOGAT and GDH. In this experiment, the NR activity in leaves of YJSM was apparently higher than that of YHSM under acid stress. This fact provides a basis to suppose that YJSM could assimilate more nitrates and tends to have stronger nitrogen metabolism ability than YHSM. In addition, the NO3–N content in the leaves of YJSM was 22.4% higher than that of YHSM under acid stress. It is noteworthy that YJSM had a higher NR activity, but it still accumulated more nitrates than YJSM under acid stress. Several reports provided evidence that facilitating acquisition of nutrients is one of the common features when plants suffer stress (Guoet al. Citation2003; Lechowskiet al. Citation2006; Attiaet al. Citation2008), and acid-tolerant rice tend to accumulate more nitrates to avoid nitrogen deficiency under stress conditions. NH4+-N metabolism responses are closely related with the activity of GS, GOGAT and GDH in plant tissues. GS/GOGAT and GDH enzymes are the primary routes of ammonium assimilation in plants (Lu Citation2002). In the process of ammonium assimilation, the activities of those enzymes are important parameters for ensuring adequate access to organic nitrogen compounds. The GS activity of YJSM was 31.3% higher than that of YHSM under acid stress. Therefore, we can speculate that YJSM had superior carbon–nitrogen metabolism enzyme activities under stress.
In addition, low molecular mass compounds, such as soluble proteins and soluble sugars, are important products of carbon–nitrogen metabolism. Plant growth responses are closely related to the concentrations of soluble proteins and soluble sugars in plant tissues (Parida and Das Citation2005; Couéeet al. Citation2006). Most of the soluble proteins are involved in enzymes of various metabolic processes, and an increase in soluble protein content is used as an important indicator of activity of overall metabolism in plants (Wang et al. Citation2009; Lainoet al. Citation2010). Several reports have provided evidence that soluble sugars are not the only components for structures and resources for energy but are also regulators in plants, and crosstalk between sugar and hormone signalling has been revealed (Sheen et al. Citation1999; Wingleret al. Citation2006; Eveland and Jackson Citation2012). Our results revealed that the soluble protein and soluble sugar contents of YJSM were 17.1% and 25.6% higher than those of YHSM under acid stress, respectively, which indicates that YJSM had superior carbon–nitrogen metabolism. Many plants accumulate free amino acids during environmental stress, such as proline, glutamate, aspartate and alanine (Kocsyet al. Citation2011; Yan et al. Citation2012). In our experiment, free amino acids markedly accumulated in YHSM exposed to acid stress, whereas YJSM accumulated fewer amino acids under the same condition. These results indicate that YHSM suffered greater acid stress than YJSM, and YHSM was not able to transform more free amino acids into more important carbon–nitrogen metabolism products. Free amino acids are fundamental ingredients in the process of protein synthesis and its use as an intermediate product of plant carbon–nitrogen metabolism (Zhang et al. Citation2012b). Although free amino acids play an important role in many physiological processes as well as in resisting stress, over accumulation of free amino acids is associated with a low efficiency of carbon–nitrogen metabolism (Hasegawa et al. Citation2000; Hamilton and Heckathorn Citation2001; Di Martino et al. Citation2003; Chen et al. Citation2014). With the contents of important carbon–nitrogen metabolites increased, the total C content of YJSM was significantly higher than that of YHSM under stress, which indicates that YJSM had stronger carbon assimilation ability than YHSM under acid stress. Therefore, acid-tolerant YJSM could produce more carbohydrates to resist stress. Combined with the aforementioned results, these findings allow us to deduce that acid-tolerant YJSM had superior carbon–nitrogen metabolism capacity on acid soil, and comprehensive investigation of the relationship between acid stress and carbon–nitrogen metabolism may provide some new clues to determine differential tolerance of rice cultivars to aluminium toxicity.
5. CONCLUSIONS
In our experiment, acid-tolerant YJSM had a significantly greater grain yield than acid-sensitive YHSM under acid stress, and there was no significant different between the two cultivars under the normal condition. The activity of SODand contents of GSH and ASA of acid-tolerant YJSM were higher than those of acid-sensitive YHSM under acid stress. Furthermore, the acid-tolerant YJSM displayed carbon–nitrogen metabolism than the acid-sensitive YHSM under acid stress. Therefore, we can speculate that the acid-tolerant rice cultivar has better antioxidant defence and carbon–nitrogen metabolism systems than the acid-sensitive rice cultivar and is more effective in resisting acid stress.
ACKNOWLEDGMENTS
This project was supported by the Special fund for Agro-scientific Research in the Public Interest (201003016) and the Guangdong Scientific Program (2012A020100004).
REFERENCES
- Ahmad P, Jaleel CA, Salem MA, Nabi G, Sharma S 2010: Roles of enzymatic and nonenzymatic antioxidants in plants during abiotic stress. Crit. Rev. Biotechnol., 30, 161–175. doi:10.3109/07388550903524243
- Andriesse W, Van Mensvoort MEF 2005: Acid sulfate soils: distribution and extent. In Encyclopaedia of Soil Science, Ed.Lal, R., pp. 14–19. Marcel Dekker, Inc, New York.
- Ashraf M 2002: Salt tolerance of cotton: some new advances. Crit. Rev. Plant Sci., 21, 1–30. doi:10.1016/S0735-2689(02)80036-3
- Ashraf M, Harris PJC 2004: Potential biochemical indicators of salinity tolerance in plants. Plant Sci., 166, 3–16. doi:10.1016/j.plantsci.2003.10.024
- Attia H, Karray N, Rabhi M, Lachaǎl M 2008: Salt-imposed restrictions on the uptake of macroelements by roots of Arabidopsis thaliana. Acta Physiologiae Plantarum, 30, 723–727. doi:10.1007/s11738-008-0172-4
- Bao SD 2002: Soil and Agricultural Chemistry Analysis, 3rd ed. China Agricultural Press, Beijing.
- Bénard C, Gautier H, Bourgaud F, Grasselly D, Navez B, Caris-Veyrat C, Weiss M, Génard M 2009: Effects of low nitrogen supply on tomato (solanumlycopersicum) fruit yield and quality with special emphasis on sugars, acids, ascorbate, carotenoids, and phenolic compounds. J. Agric. Food Chem., 57, 4112–4123. doi:10.1021/jf8036374
- Borsani J, Budde CO, Porrini L, Lauxmann MA, Lombardo VA, Murray R, Andreo CS, Drincovich MF, Lara MV 2009: Carbon metabolism of peach fruit after harvest: changes in enzymes in involved in organic acid and sugar level modifications. J. Exp. Bot., 60, 1823–1837. doi:10.1093/jxb/erp055
- Butare L, Rao I, Lepoivre P, Cajiao C, Polania J, Cuasquer J, Beebe S 2012: Phenotypic evaluation of interspecific recombinant inbred lines (RILs) of Phaseolus species for aluminium-toxic acid soil. Euphytica, 186, 715–730. doi:10.1007/s10681-011-0564-1
- Cag S, Cevahir-öz G, Sarsag M, Gören-Saglam N 2009: Effect of salicylic acid on pigment, protein content and peroxidase activity in excised sunflower cotyledons. Pakistan J. Bot., 41, 2297–2303.
- Cakmak I, Horst WJ 2006: Effect of aluminium on lipid peroxidation, superoxide dismutase, catalase, and peroxidase activities in root tips of soybean (Glycine max). PhysiologiaPlantarum, 83, 463–468. doi:10.1111/j.1399-3054.1991.tb00121.x
- Cartes P, Jara AA, Pinilla L, Rosas A, Mora ML 2010: Selenium improves the antioxidant ability against aluminium-induced oxidative stress in ryegrass roots. Ann. Appl. Biol., 156, 297–303. doi:10.1111/j.1744-7348.2010.00387.x
- Carvalhais LC, Dennis PG, Fedoseyenko D, Hajirezae MR, Borriss R, von Wiren N 2011: Root exudation of sugars, amino acids, and organic acids by maize as affected by nitrogen, phosphorus, potassium, and iron deficiency. J. Plant Nutr. Soil Sci., 174, 3–11. doi:10.1002/jpln.201000085
- Chen C, Li Q, Zhou Q, Sun L, Zheng M, Gao Y 2014: Accumulation of free amino acids in marine diatom resting cells during rejuvenation. J. Sea Res., 85, 483–490. doi:10.1016/j.seares.2013.08.003
- Chen J, Wang WH, Wu FH, You CY, Liu TW, Dong XJ, He JX, Zheng HL 2013: Hydrogen sulphide alleviates aluminium toxicity in barley seedlings. Plant Soil, 362, 301–318. doi:10.1007/s11104-012-1275-7
- Cheong MS, Yun DJ 2007: Salt-stress signaling. J. Plant Biol., 50, 148–155. doi:10.1007/BF03030623
- Coruzzi GM, Zhou L 2001: Carbon and nitrogen sensing and signaling in plants: emerging ‘matrix effects’. CurrOpin Plant Biol., 4, 247–253. doi:10.1016/S1369-5266(00)00168-0
- Couée I, Sulmon C, Gouesbet G, Amrani AE 2006: Involvement of soluble sugars in reactive oxygen species balance and responses to oxidative stress in plants. J. Exp. Bot., 57, 449–459. doi:10.1093/jxb/erj027
- Cui J, Zhang R, Wu WL, Zhu HM, Yang H 2010: Salicylic acid reduces napropamide toxicity by preventing its accumulation in Rapeseed (Brassica napus L.). Arch. Environ. Contam.Toxicol.,59, 100–108. doi:10.1007/s00244-009-9426-4
- Di Martino C, Delfine S, Pizzuto R, Loreto F, Fuggi A 2003: Free amino acids and glycine betaine in leaf osmoregulation of spinach responding to increasing salt stress. New Phytologist, 158, 455–463. doi:10.1046/j.1469-8137.2003.00770.x
- Eveland AL, Jackson DP 2012: Sugars, signalling, and plant development. J. Exp. Bot., 63, 3367–3377. doi:10.1093/jxb/err379
- Feng RW, Wei CY, Tu SX 2013: The roles of selenium in protecting plants against abiotic stresses. Environ. Exp. Bot., 87, 58–68. doi:10.1016/j.envexpbot.2012.09.002
- Freeman JL, Tamaoki M, Stushnoff C et al. 2010: Molecular mechanisms of selenium tolerance and hyperaccumulation inStanleyaPinnata. Plant Physiol., 153, 1630–1652. doi:10.1104/pp.110.156570
- Fritz C, Mueller C, Matt P, Feil R, Stitt M 2006b: Impact of the C–N status on the amino acid profile in tobacco source leaves. Plant Cell. Environ., 29, 2055–2076. doi:10.1111/j.1365-3040.2006.01580.x
- Fritz C, Palacios-Rojas N, Feil R, Stitt M 2006a: Regulation of secondary metabolism by the carbon-nitrogen status in tobacco: nitrate inhibits large sectors of phenylpropanoid metabolism. Plant J., 46, 533–548. doi:10.1111/tpj.2006.46.issue-4
- Gao JF 2005: Experimental Guidance for Plant Physiology, pp. 74–76. Higher Education Press, Beijing, China.
- Guo TR, Zhang GP, Lu WY, Wu HP, Chen JX, Wu FB, Zhou MX 2003: Effect of Al on dry matter accumulation and Al and nutrition contents of barleys differing in Al tolerance. Plant Nutr.Fertil. Sci., 9, 324–330.
- Haling RE, Simpson RJ, Delhaize E, Hocking PJ, Richardson AE 2010: Effect of lime on root growth, morphology and the rhizosheath of cereal seedlings growing in an acid soil. Plant and Soil, 327, 199–212. doi:10.1007/s11104-009-0047-5
- Hamilton EW, Heckathorn SA 2001: Mitochondrial adaptations to NaCl. Complex I is protected by anti-oxidants and small heat shock proteins, whereas complex II is protected by proline and betaine. Plant Physiol., 126, 1266–1274. doi:10.1104/pp.126.3.1266
- Han D, Li XH, Xiong SL, Tu SX, Chen ZG, Li JP, Xie ZJ 2013: Selenium uptake, speciation and stressed response of Nicotianatabacum L. Environ. Exp. Bot., 95, 6–14. doi:10.1016/j.envexpbot.2013.07.001
- Hasegawa PM, Bressan RA, Zhu JK, Bohnert HJ 2000: Plant cellular and molecular responses to high salinity. Annu. Rev. Plant Physiol. Plant Mol. Biol., 55, 373–399.
- Jiang L, Yang H 2009: Prometryne-induced oxidative stress and impact on antioxidant enzymes in wheat. Ecotoxicol. Environ. Saf., 72, 1687–1693. doi:10.1016/j.ecoenv.2009.04.025
- Kocsy G, Simon-Sarkadi L, Kovács Z, Boldizsár A, Sovány C, Kirsch K, Galiba G 2011: Regulation of free amino acid and polyamine levels during cold acclimation in wheat. Acta Biol. Szegediensis, 55, 91–93.
- Koyro HW 2006: Effect of salinity on growth, photosynthesis, water relations and solute composition of the potential cash crop hahophytePlantagocoronopus (L.). Environ. Environ. Bot., 56, 136–146. doi:10.1016/j.envexpbot.2005.02.001
- Laino P, Shelton D, Finnie C, De Leonardis AM, Mastrangelo AM, Svensson B, Lafiandra D, Masci S 2010: Comparative proteome analysis of metabolic proteins from seeds of durum wheat (cv. Svevo) subjected to heat stress. Proteomics, 10, 2359–2368. doi:10.1002/pmic.v10:12
- Lechowski Z, Mej E, Bialczyk J 2006: Accumulation of biomass and some macroelements in tomato plants grown in media with (+)-usnic acid. Environ. Exp. Bot., 56, 239–244. doi:10.1016/j.envexpbot.2005.03.001
- Li HS 2000: Principles and Techniques of Plant Physiological Biochemical Experiment. Higher Education Press, Beijing.
- Li XF, Ma JF, Matsumoto H 2000: Pattern of aluminium-induced secretion of organic acids differs between rye and wheat. Plant Physiol., 123, 1537–1544. doi:10.1104/pp.123.4.1537
- Lin CC, Kao CH 1996: Disturbed ammonium assimilation is associated with growth inhibition of roots in rice seedlings caused by NaCl. Plant Growth Regulation, 18, 233–238. doi:10.1007/BF00024387
- Lin L, Zhou W, Dai H, Cao F, Zhang G, Wu F 2012: Selenium reduces cadmium uptake and mitigates cadmium toxicity in rice. J. Hazard. Mater., 235-236, 343–351. doi:10.1016/j.jhazmat.2012.08.012
- López M, Herrera-Cervera JA, Iribarne C, Tejera NA, Lluch C 2008: Growth and nitrogen fixation in Lotus japonicus and Medicagotruncatula under NaCl stress: Nodule carbon metabolism. J. Plant Physiol., 165, 641–650. doi:10.1016/j.jplph.2007.05.009
- López-Bucio J, Nieto-Jacobo MF, Ramírez-Rodríguez V, Herrera-Estrella L 2000: Organic acid metabolism in plants: from adaptive physiology to transgenic varieties for cultivation in extreme soils. Plant Sci., 160, 1–13. doi:10.1016/S0168-9452(00)00347-2
- Lu JL 2002: Plant Nutrition, 2nd ed. China Agricultural Press, Beijing.
- Ma JF 2000: Role of organic acids in detoxification of aluminium in higher plants. Plant Cell. Physiol., 41, 383–390. doi:10.1093/pcp/41.4.383
- Ma JF, Ryan PR, Delhaize E 2001: Aluminium tolerance in plants and the complexing role of organic acids. TRENDS Plant Sci., 6, 273–278. doi:10.1016/S1360-1385(01)01961-6
- Ma JF, Zheng SJ, Matsumoto H 1997a: Specific secretion of citric acid induced by Al stress in Cassia toraL., Plant Cell. Physiol., 38, 1019–1025. doi:10.1093/oxfordjournals.pcp.a029266
- Ma JF, Zheng SJ, Matsumoto H, Hiradate S 1997b: Detoxifying aluminium with buckwheat. Nature, 390, 569–570. doi:10.1038/37518
- Masulili A, Utomo WH, Syechfani MS 2010: Rice husk biochar for rice based cropping system in acid soil 1. The characteristics of rice husk biochar and its influence on the properties of acid sulfate soils and rice growth in West Kalimantan, Indonesia. J. Agric. Sci., 2, 39–47.
- McDowell NG 2011: Mechanisms linking drought, hydraulics, carbon metabolism, and vegetation mortality. Am. Soc. Plant Biol., 155, 1051–1059.
- Meriga B, Ramgopal IHAM, Ediga A, Kavikishor PB 2010: Differential tolerance to aluminium toxicity in rice cultivars: Involvement of antioxidative enzymes and possible role of aluminium resistant locus. Acad. J. Plant Sci., 3, 53–63.
- Miller AJ, Fan X, Shen Q, Smith SJ 2007: Amino acids and nitrate as signals for the regulation of nitrogen acquisition. J. Exp. Bot., 59, 111–119. doi:10.1093/jxb/erm208
- Mora ML, Pinilla L, Rosas A, Cartes P 2008: Selenium uptake and its influence on the antioxidative system of white clover as affected by lime and phosphorus fertilization. Plant Soil, 303, 139–149. doi:10.1007/s11104-007-9494-z
- Mroczek-Zdyrska M, Wójcik M 2012: The influence of selenium on root growth and oxidative stress induced by lead in viciafaba L. minor plants. Biol. Trace Elem. Res., 147, 320–328. doi:10.1007/s12011-011-9292-6
- Munns R 2002: Comparative physiology of salt and water stress. Plant Cell Environ, 25, 239–250. doi:10.1046/j.0016-8025.2001.00808.x
- Noctor G, Mhamdi A, Chaouch S, Han YI, Neukermans J, Marquez-Garcia B, Queval G, Foyer CH 2012: Glutathione in plants: an intergrated overview. Plant Cell Environ., 35, 454–484. doi:10.1111/j.1365-3040.2011.02400.x
- Nordmyr L, Österholm P, Åström M 2008: Estuarine behaviour of metal loads leached from coastal lowland acid sulphate soil. Marine Environ. Res., 66, 378–393. doi:10.1016/j.marenvres.2008.06.001
- Nunes-Nesi A, Fernie AR, Stitt M 2010: Metabolic and signaling aspects underpinning the regulation of plant carbon nitrogen interactions. Mol. Plant, 3, 973–996. doi:10.1093/mp/ssq049
- Parida AK, Das AB 2005: Salt tolerance and salinity effects on plants: a review [J]. Ecotoxicol. Environ, Saf.,60, 324–349. doi:10.1016/j.ecoenv.2004.06.010
- Parvaiz A, Satyawati S 2008: Salt stress and phyto-biochemical responses of plants – a review. Plant Soil Environ., 54, 89–99.
- Quan SH, Wang YG, Luo SQ, Tang FL 2004: Effects of the pH value of bed soil on seedling quality in rice. Hybrid Rice, 19, 45–46.
- Rabinowitz JD, Rabitz HA, Xu Y 2012: Integration of carbon, nitrogen, and oxygen metabolism in escherichia coli–final report (No. DOE/SC0002077). Princeton University, Princeton, NJ.
- Radwan DEM 2012: Salicylic acid induced alleviation of oxidative stress caused by clethodim in maize (Zea mays L.) leaves. Pestic.Biochem. Physiol., 102, 182–188. doi:10.1016/j.pestbp.2012.01.002
- Robson ADE, Foy CD 1990: Soil acidity and plant growth. Soil Sci., 150, 903.
- Scheibe R 2004: Malate valves to balance cellular energy supply: redox regulation: from molecular responses to environmental adaptation. PhysiologiaPlantarum, 20, 21–26. doi:10.1111/j.0031-9317.2004.0222.x
- Sheen J, Zhou L, Jang JC 1999: Sugars as signaling molecules. Curr. Opin. Plant Biol., 2, 410–418. doi:10.1016/S1369-5266(99)00014-X
- Silva IR, Smyth TJ, Raper CD, Carter TE, Rufty TW 2001: Differential aluminum tolerance in soybean: an evaluation of the role of organic acids. PhysiologiaPlantarum, 112, 200–210. doi:10.1034/j.1399-3054.2001.1120208.x
- Silva S, Pinto-Carnide O, Martins-Lopes P, Matos M, Guedes-Pinto H, Santos C 2010: Differential aluminium changes on nutrient accumulation and root differentiation in an Al sensitive vs. tolerant wheat. Environ. Exp. Bot., 68, 91–98. doi:10.1016/j.envexpbot.2009.10.005
- Tang YF, Sun XC, Hu CX, Tan QL, Zhao XH 2013: Genotypic differences in nitrate uptake, translocation and assimilation of two Chinese cabbage cultivars [Brassica campestris L. ssp. Chinensis (L.)]. Plant Physiol. Biochem., 70, 14–20. doi:10.1016/j.plaphy.2013.04.027
- Wang L, Zhou Q, Sun DY 2008: Effect of cadmium toxicity on nitrogen metabolism in leaves of Solanumnigrum L. as a newly found cadmium hyperaccumulator. J. Hazardous Mater., 154, 818–825. doi:10.1016/j.jhazmat.2007.10.097
- Wang X, Guo PY, Yuan XY, Yao MS 2009: The effect of plant growth regulators on soluble protein content of opium poppy. Mod. Agric. Sci., 16, 12–13.
- Wingler A, Purdy S, MacLean JA, Pourtau N 2006: The role of sugars in integrating environment signals during the regulation of leaf senescence. J. Exp. Bot., 57, 391–399. doi:10.1093/jxb/eri279
- Yan H, Peng XB, Xue JJ 2012: Effects of NaCl stress on leaf photosynthesis characteristics and free amino metabolism of heyedysarumscoparium. J. Appl. Ecol., 23, 1790–1796.
- Yang JF, He LY, Liu YF, Wu ZH, Guo ZH 2009: Identification on ecological adaptation of different P efficiency rice in soils under different acidic soils. J. Huazhong Agric. Univ., 28, 31–34.
- Yang LT, Jiang HX, Tang N, Chen LS 2011: Mechanisms of aluminium-tolerance in two species of citrus: Secretion of organic acid anions and immobilization of aluminium by phosphorus in roots. Plant Sci., 180, 521–530. doi:10.1016/j.plantsci.2010.11.011
- Yu L, Yan J, Guo S, Zhu W 2012: Aluminum-induced secretion of organic acid by cowpea (Vignaunguiculata L.). ScientiaHorticulturae, 135, 52–58. doi:10.1016/j.scienta.2011.12.006
- Zhang JL, Gao F, Lin YJ, Wang YY, Yang CT, Zhang F, Li YH, Li XD 2013: Differences in seed kernel quality and related enzyme activities of different quality type peanut cultivars. Chin. J. Appl. Ecol., 24, 481–487.
- Zhang M, Hu CX, Zhao XH, Tan QL, Sun XC, Cao AY, Cui M, Zhang Y 2012a: Molybdenum improves antioxidant and osmotic-adjustment ability against salt stress in Chinese cabbage (Brassica campestris L. ssp. Pekinensis). Plant Soil, 355, 375–383. doi:10.1007/s11104-011-1109-z
- Zhang PP, Fu JM, Hu LX 2012b: Effects of alkali stress on growth, free amino acids and carbohydrates metabolism in Kentucky bluegrass (Poapratenis). Ecotoxicology, 21, 1911–1918. doi:10.1007/s10646-012-0924-1
- Zheng SJ, Ma JF, Matsumoto H 1998: High aluminum resistance in buckwheat l. Al-induced specific secretion of oxalic acid from root tips. Plant Physiol., 117, 745–751. doi:10.1104/pp.117.3.745