Abstract
Cancer is a devasting disease resulting in millions of deaths worldwide in both humans and companion animals, including dogs. Treatment of cancer is complex and challenging and therefore often multifaceted, as in the case of osteosarcoma (OS) and soft tissue sarcoma (STS). OS predominantly involves the appendicular skeleton and STS commonly develops in the extremities, resulting in treatment challenges due to the need to balance wide-margin resections to achieve local oncological control against the functional outcomes for the patient. To achieve wide tumor resection, invasive limb salvage surgery is often required, and the patient is at risk for numerous complications which can ultimately lead to impaired limb function and mobility. The advent of tumor ablation techniques offers the exciting potential of developing noninvasive or minimally invasive treatment options for extremity tumors. One promising innovative tumor ablation technique with strong potential to serve as a noninvasive limb salvage treatment for extremity tumor patients is histotripsy. Histotripsy is a novel, noninvasive, non-thermal, and non-ionizing focused ultrasound technique which uses controlled acoustic cavitation to mechanically disintegrate tissue with high precision. In this review, we present the ongoing development of histotripsy as a non-surgical alternative for extremity tumors and highlight the value of spontaneously occurring OS and STS in the pet dog as a comparative oncology research model to advance this field of histotripsy research.
Introduction
Cancer is a devasting disease resulting in millions of deaths annually worldwide [Citation1]. Overall patient prognosis and survival outcomes have significantly improved over the last several decades with advancements in cancer diagnostics and treatment options, including surgical techniques. Nevertheless, malignant primary extremity tumors such as osteosarcoma (OS), Ewing’s sarcoma, chondrosarcoma, and soft tissue sarcoma (STS) continue to pose treatment challenges due to the need to balance wide margin resection to achieve local oncologic control against functional outcomes for the patient. Limb salvage surgery techniques have continued to advance and provide viable surgical resection options for extremity tumor patients. However, they are invasive and remain associated with potential complications such as infection, implant construct complications, and can lead to decreased limb function and mobility [Citation2–6]. Metastatic extremity tumors commonly manifest in the bone, representing metastases from primary tumors such as breast and prostate cancers [Citation7], and pose treatment challenges. Current treatment of extremity bone metastases utilizes a palliative approach to provide symptomatic relief with combinatorial medical, surgical and radiation therapies [Citation7]. Resection of bone metastases is usually performed only in select patients, and the option of surgery is limited in cases of multifocal metastatic lesions [Citation7]. The advent of noninvasive or minimally invasive tumor ablation techniques offers the exciting potential of developing treatment options which avoid the morbidity of current therapies, and expands treatment possibilities for multifocal disease. One promising novel tumor ablation technique that has strong potential to serve as a noninvasive limb salvage treatment option for primary and metastatic extremity tumor patients is histotripsy.
This review aims to provide an overview of the ongoing development of histotripsy as a novel tumor ablation technique for extremity tumors, highlighting the strength of the comparative oncology research model of spontaneously occurring canine extremity tumors (OS and STS) to inform translation of histotripsy into human clinical treatment.
The canine as a comparative oncology model
Due to many shared characteristics between human and canine cancers, companion animals diagnosed with naturally-occurring cancer have presented a unique opportunity as a ‘parallel patient population’ to advance our understanding of cancer and evaluate the potential for clinical success of cancer therapies for humans [Citation8–13]. Conventional preclinical models such as rodents provide excellent means of addressing mechanistic research questions. However, in contrast to laboratory rodents, the pet dog has the advantage of being an outbred species like humans and shares environmental influences and stressors with humans. Additionally, just like humans, companion animals spontaneously develop cancers, which involve complex interactions between the immune system, and malignant and nonmalignant cells. The >10-fold increased incidence of some cancers such as OS in dogs in combination with a compressed course of disease, also gives the advantage of assessing canine clinical study outcomes in relatively short periods of time compared to human clinical trials. In this section, we highlight the strengths of canine OS and STS for comparative oncology research to develop histotripsy for extremity tumor ablation.
Osteosarcoma
OS is the most common primary bone malignancy in both pediatric and canine cancer patients, accounting for greater than 55% and 80–90% of primary bone tumor diagnoses, respectively [Citation14–16]. Human and canine OS share parallel biological behavior [Citation8,Citation17,Citation18], global gene expression signatures [Citation18,Citation19], and nearly identical histological characteristics [Citation8,Citation10,Citation17]. OS occurs primarily in the metaphysis of appendicular long bones in predominantly tall rapidly growing youth and large to giant breed dogs [Citation10,Citation14,Citation15,Citation20]. Current definitive standard-of-care treatment for human and canine OS includes surgical resection of the primary tumor via limb amputation or limb-salvage surgery and chemotherapy for management of metastatic disease. Both limb salvage and amputation surgeries are invasive procedures with potential for complications and functional impairment [Citation5,Citation21–25]. In addition to the challenges and morbidity of surgical primary tumor resection, metastatic disease remains the predominant cause of mortality in human and canine OS patients despite chemotherapeutics [Citation8,Citation10,Citation20]. The survival outcomes for both humans and dogs have not improved significantly for decades. In the absence of metastatic disease at the time of OS diagnosis, the 5-year survival for human OS patients is ∼70% and the median survival for dogs is 10-12-months [Citation10]. The similarities in tumor biology, disease phenotype, treatments and oncologic outcomes of human and canine OS allow dogs with OS to serve as a strong comparative oncology research model for human OS to bridge the translational gap from murine preclinical studies to human clinical trials.
Soft tissue sarcoma
Soft tissue sarcoma (STS) is a heterogenous group of tumors that arise from mesenchymal tissues, comprising more than 50 different histological subtypes that share common biological behavior and treatment challenges. They are characterized by locally expansile masses arising from soft tissues such as muscle and skin, 40-60% of which affect the extremities [Citation26–29]. Of the vast variety of STS subtypes in people, malignant fibrous histiocytoma, liposarcoma, synovial sarcoma, leiomyosarcoma, are most prevalent in adult patients, and rhabdomyosarcoma in pediatric patients [2730]. STS in humans is relatively rare, accounting for less than 1% of all cancer diagnoses, but a higher prevalence of STS has been reported in dogs [Citation12,Citation13]. Human and canine STS are histologically similar and have a common tumor grading system that guides treatment planning and prognosis prediction [Citation26,Citation31–34]. STS tumors can invade adjacent critical structures and local disease control is the major life-limiting aspect for human and canine patients with STS [Citation27,Citation29–31,Citation33–36]. Surgical removal of extremity STS is the treatment of choice in both species, ideally achieved via limb salvage procedures when feasible, but limb amputation may be required in some cases [Citation33,Citation37,Citation38].
Given the strong similarities between canine and human extremity OS and STS, the dog has served as a comparative oncology research model for investigating numerous thermal ablation modalities such as high intensity focused ultrasound (HIFU) and microwave ablation (MWA) for extremity tumor treatment [Citation39–41]. Furthermore, the pet dog with spontaneously occurring OS and STS has been utilized to pursue proof-of-concept investigations [Citation42–44] of histotripsy ablation as a limb sparing treatment alternative for extremity tumors.
Extremity tumor ablation modalities
Histotripsy
Histotripsy is an emerging non-thermal, non-ionizing, and noninvasive focused ultrasound therapy currently under development for a variety of applications in human [Citation45,Citation46] and veterinary medicine [Citation46,Citation47]. The term ‘histotripsy’ was formulated from the two Greek terms ‘histo’ meaning “tissue”, and ‘tripsy’ meaning “to crush” (i.e., breakdown or destruct). The physical means by which histotripsy destroys tissue is through the use of short duration (microsecond) and very high amplitude (>10 – 25 MPa depending on pulsing scheme and cavitation medium) ultrasound pulses to generate acoustic cavitation from endogenous gas in tissues [Citation48]. Acoustic cavitation is the generation of microbubbles (termed a ‘cavitation cloud’) which rapidly expand and collapse imparting high strain on surrounding cells to effectively disintegrate targeted tissue over repeated pulses [Citation46,Citation49–52]. The threshold to initiate an acoustic cavitation cloud and the number of pulses required to achieve ablation have both been shown to vary depending on different tissue mechanical properties, thus conferring tissue selectivity to histotripsy ablation [Citation53,Citation54]. As a tumor ablation modality, histotripsy has been reported in a human clinical trial for ablating liver tumors [Citation55,Citation56] and in veterinary medicine for ablating primary bone sarcomas [Citation43,Citation44,Citation57] and STS [Citation42]. There are currently three primary types of histotripsy under development: shock-scattering histotripsy, intrinsic threshold histotripsy, and boiling histotripsy [Citation46,Citation48,Citation58], which have been recently reviewed elsewhere [Citation58]. For the development of histotripsy ablation for extremity tumors intrinsic threshold histotripsy (referred to here on in as single cycle histotripsy) was investigated [Citation42–44], and is the type of histotripsy on which this manuscript is focused. Histotripsy has numerous clinically attractive characteristics for extremity tumor ablation, including advantages over other ablation modalities described below (). The non-thermal nature of histotripsy contributes to its high precision, tissue selective properties, and ability to permit ablation of tumors near critical structures [Citation53,Citation54,Citation93]. The histotripsy cavitation cloud can be visualized in real-time using ultrasound imaging [Citation46,Citation94], enabling immediate treatment feedback. Additionally, the resolution of histotripsy tumor ablation zones with replacement of ablated tissue by normal tissue has been demonstrated in human liver tumor ablation clinical trials [Citation55], preclinical rodent liver tumor models [Citation95], porcine liver tumor model [Citation96] renal and prostate tissue [Citation97,Citation98]. Furthermore, histotripsy has the potential to induce local and systemic immunomodulation potentially resulting in an abscopal effect and anti-tumor immune response that could mitigate metastatic disease [Citation56,Citation99–102]. The mechanism and biological effects of histotripsy are visually depicted and summarized in .
Figure 1. A Pictorial diagram of the histotripsy mechanism and downstream biological effects. Histotripsy works by generating acoustic cavitation bubble cloud from endogenous gas in tissues via ultrasound pulses. The expansion of the microbubbles that the cavitation cloud is composed of exerts pressure on cells ultimately leading to cell lysis (mechanical stress and cell lysis panel). The lysis of targeted cells results in the release of immunogenic proteins stimulating an immune response. The stimulated immune response has the potential to shift an immunological quiescent tumor into an immunological responsive state to aid in tumor regression (inflammation & immunity panel). Over time the acellular homogenate created by histotripsy ablation is resorbed and the targeted tissue is repaired (ablated tissue resorption and repair panel). created with BioRender.com.
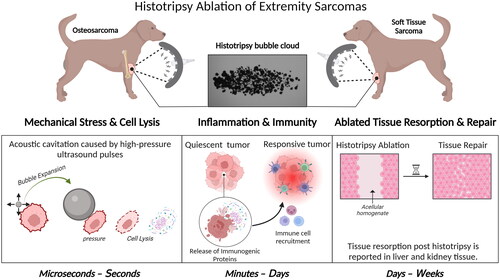
Table 1. A summary of relevant literature pertaining to thermal ablation modalities and radiation therapy for extremity tumors in human and veterinary medicine.
Thermal and Radiation-Based ablation modalities
Thermal ablation modalities have been investigated and utilized clinically as non-surgical alternatives for extremity tumors or in conjunction with surgery to provide definitive and palliative care ().
Although thermal and radiation-based ablation modalities have proven to be successful as ablative modalities for treating extremity tumors, they are also associated with limitations. Thermal ablation techniques incur risks such as thermal skin damage, ablation site infection, fracture, the heat sink effect, and tissue damage due to off-target ablation or thermal diffusion [Citation68,Citation75]. The heat sink effect is the reduction of ablation efficacy near large blood vessels as a result of the cooling effect from blood flow, potentially resulting in incomplete ablation of a targeted region. Furthermore, both the heat sink effect and off-targeting potential of thermal ablation techniques limit their use near critical anatomic structures such as vessels and nerves. Radiation-based tumor ablation is associated with potential short- and long-term adverse effects [Citation87,Citation88], with major long-term adverse effects being an especially important clinical consideration in limiting patient selection.
Since histotripsy is a non-thermal ablation modality, it is not subject to the heat sink effect, and the tissue selective properties of histotripsy increase ablation precision and reduce the risk of off-target ablation and consequent tissue damage [Citation46,Citation53,Citation103]. Histotripsy is also noninvasive and thus does not incur the risk of ablation site infection due to ablation probe insertions, unlike minimally invasive thermal ablation modalities such as laser ablation, RFA and MWA [Citation104]. Histotripsy, being a non-ionizing ablation modality, does not incur the risks of late side effects caused by ionizing radiation.
In these subsequent sections, we detail the development of histotripsy for extremity tumor ablation with a focus on using the canine model of spontaneous tumors to provide the foundation to successfully translate histotripsy for human extremity tumor ablation.
Developing histotripsy ablation for extremity tumors
Recent publications have detailed ex vivo and in vivo investigations for histotripsy as an extremity tumor ablation modality for OS [Citation43,Citation44,Citation57] and STS [Citation42] using the canine comparative oncology research model (). An ex vivo study using excised canine OS tumor samples to investigate histotripsy’s ablative efficacy reported that histotripsy could generate complete ablation of targeted OS while maintaining the histologic structural integrity of grossly normal bone and nerves [Citation57]. Subsequent treat-and-resect feasibility canine clinical trials for US and STS demonstrated that histotripsy could effectively ablated targeted regions within appendicular OS tumors and superficial canine extremity STS tumors () [Citation42]. To our knowledge, as of the preparation of this review, these are the only reports in the scientific literature on developing histotripsy for extremity tumor ablation. In the following sections, we discuss 1) Histotripsy equipment and dosing parameters, 2) Clinical and general safety considerations, 3) Current engineering limitations and potential solutions, 4) Imaging modalities for histotripsy ablation, all of which are pertinent to the advancement of histotripsy for canine extremity OS and STS ablations to inform human clinical translation.
Table 2. A summary of the histotripsy ablation studies for canine OS and STS.
Table 3. Reported and proposed methods for monitoring histotripsy ablation in real-time during extremity tumor ablation.
Histotripsy Equipment and dosing parameters for canine extremity sarcomas
Equipment
The prototype histotripsy system utilized in previously described treat-and-resect canine clinical trials [Citation42–44] is composed of the following: 1) a therapy transducer with a coaxially aligned US imaging probe for real-time imaging during treatment; 2) a robotic positioning arm to move the transducer, 3) a coupling bowl filled with degassed water as a transmission medium for the US; 4) driving electronics, including the amplifier and power supply; and 5) a computer equipped with treatment planning software to define and execute the histotripsy ablation (). The transducer (), coupled to the target tissue with degassed water, is integrated onto the robotic positioner and controlled via treatment planning software, allowing the user to position the cavitation bubble cloud within the target region of the tumor on US imaging and generate a volumetric tumor ablation plan. The tumor ablation plan, consisting of overlapping focal cavitation zones as points in a defined volume, is then automatically delivered through computer programing and can be monitored in real-time via US imaging.
Figure 2. Experimental histotripsy set-up. A) a robotic micro-positioner is connected to an articulating arm supporting the therapy and imaging transducer assembly. The transducer assembly was submerged in a water coupling bowl coupled to the patient’s tumor, and treatment was monitored in real-time using ultrasound imaging. B) The 500 kHZ histotripsy transducer with coaxially aligned ultrasound imaging probe utilized for OS and STS extremity tumor ablation in canine patients.
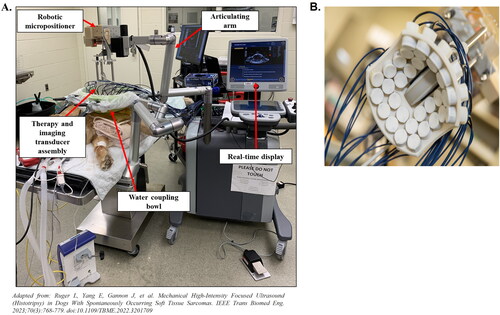
The transducer is essential for the successful delivery of histotripsy to targeted regions, and numerous factors are considered for the transducer selection process for extremity tumor ablation. These factors include the transducer frequency, quantity and spatial arrangement of therapy elements, focal depth (distance from the transducer to the geometric focus), and maximum pressure output. Generally, histotripsy ablation is delivered using a transducer with a center frequency of 500 kHz − 1 MHz and pulsing schemes with a low duty cycle (≤1%) [Citation46,Citation105]. For single cycle histotripsy a key factor in generating precise acoustic cavitation is that transducers must be designed with a low f-number and a high focal gain ratio (≥30) [Citation46]. Additionally, the size, shape, and density of the cavitation bubble cloud is dependent on the number and spatial arrangement of the therapy elements within the transducer, the geometry of the transducer scaffolding, and the f-number. A low frequency transducer tends to generate a bubble cloud composed of large individual bubbles, and a high frequency transducer tends to generate a dense cloud composed of small bubbles [Citation106]. For single cycle pulsing, the f-number of the histotripsy transducer also influences the bubble cloud characteristics, with higher f-number (less focused) transducers having a reduced bubble cloud density and a larger deviation in cloud size [Citation103]. The frequency and f-number of the transducer also affect the efficiency of the histotripsy treatment, with higher doses required to fully ablate the target tissue at higher frequencies [Citation106] and higher f-numbers [Citation103], and longer treatment times for equivalent volume ablations observed for smaller clouds. All of these considerations are especially relevant for extremity tumors, where a balance between treatment efficiency and precision is needed to treat large sized tumors without damaging the skin or other adjacent healthy tissues.
Dosing parameters
The histotripsy dose is another important factor to consider and optimize for extremity tumor ablation. Due to the tissue selective properties of histotripsy, a wide spectrum of histotripsy doses has been utilized for different tissue types to achieve tissue ablation [Citation42–44,Citation55,Citation107,Citation108]. Both OS and STS can be treated at a pulse repetition frequency (PRF) of 500 Hz, but previously reported results have shown that achieving tumor ablation in structurally dense OS requires the delivery of a dose of 1000 PPP [Citation44] (), whereas ablation was achieved in half as many pulses at a dose of 500 PPP in STS [Citation42] ().
Figure 3. Representative images demonstrating histotripsy ablation in OS. (A) gross pathology and (B) low- (2×) and high-magnification (20×) microscopic histology images comparing treated and untreated OS tumor regions. In all patients shown, histotripsy-treated regions of the tumor (thick black boxes in (a)) were characterized by hemorrhage, tissue softening, and/or necrosis. Sections from untreated areas of OS tumors showed dense proliferation of neoplastic osteoblasts variably surrounding the osteoid matrix (black arrowheads). in contrast, sections from treated tumors exhibited hemorrhage and necrosis, abundant cell death (empty arrowheads identify dead or dying cells), complete tissue obliteration with replacement basophilic slipping (white star), and/or matrix degeneration (not shown). (B) were taken from boxed regions in columns 1 and 3, respectively.
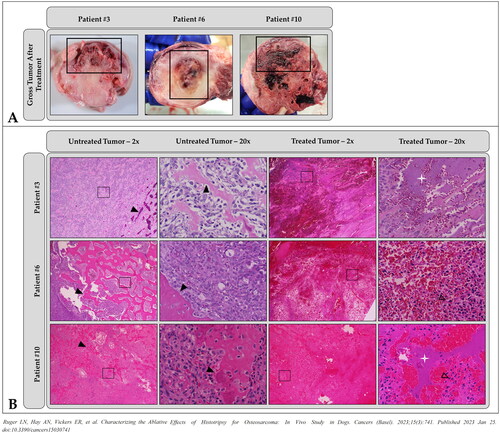
Figure 4. Representative images demonstrating histotripsy ablation of STS. (a) Gross visualization of lesion characterized by extensive tissue necrosis and hemorrhage (arrow). (b-f) H&E stained sections compared (d) untreated (magnification 40x) and (e) treated (magnification 40x) tumor tissues and (b,c,f) interface regions (c – magnification 2x; f – magnification 40x). clearly delineated boundaries between treated (+) and untreated (*) tumor tissue were observed.
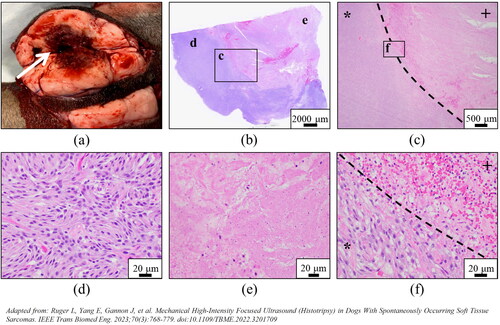
Clinical considerations
When considering the histotripsy dose for extremity tumor clinical trial patients, whether human or canine, there are numerous clinical considerations that must be acknowledged.
Tumor tissue heterogeneity
Extremity tumors can manifest as phenotypically diverse tumors. Spontaneously occurring appendicular primary OS have heterogeneous gross tissue compositions, which creates challenges for consistent and uniform histotripsy ablation. Successful histotripsy ablation of bone sarcomas that were predominantly composed of lytic bone, proliferative bone, intact bony matrix, soft tissue, or a mixture of these tissue types have all been reported () [Citation43,Citation44]. However, even though histotripsy ablation has the advantage over current thermal ablation techniques, with the exception of HIFU, of providing real-time ultrasound monitoring of ablation, the presence of bone in OS tumors presents a challenge. Unsurprisingly, the variety of tissues that compose OS tumors influences the ability of ultrasound to clearly delineate tumor boundaries and visualize the cavitation bubble cloud for real-time treatment monitoring. OS tumors composed of lytic bone and/or contained a soft tissue component allowed for optimal delineation of the tumor and visualization of the cavitation bubble cloud for real-time ultrasound treatment monitoring. For tumors predominantly composed of proliferative bone and/or bony matrix, the tumor was challenging to delineate, and ablation progress could not be monitored in real-time with ultrasound and instead was monitored by passive cavitation detection (PCD) (see Imaging Modalities section) [Citation43,Citation44].
Figure 5. Representative photographs of grossly visible OS tumors and US bubble cloud photos during treatment. (a–c) Patient images showing different gross appearances between the treated primary bone tumors correlating to different radiographic tumor characteristics representing (a) a grossly visible extensive soft tissue component (arrow), (b) a primarily lytic tumor with a small amount of soft tissue component, and (c) a primarily proliferative bone tumor with mostly intact cortical bone. (d–f) B-mode ultrasound images during treatment. Cavitation bubble clouds (arrows) were visible when histotripsy was applied to the soft tissue tumor component and lytic tumors (d,e), but not when applied to patients with proliferative bone tumors and mostly intact cortical bone (f).
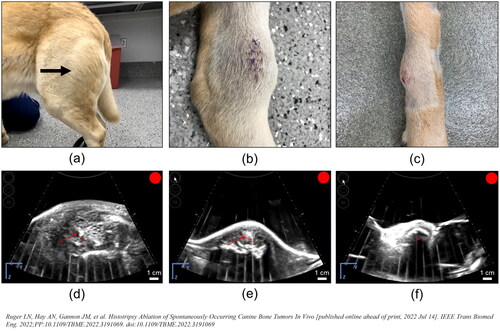
In contrast, even though there are several histologic subtypes of STS, these tumors are grossly composed of soft tissue. The primarily soft tissue composition and superficial nature of extremity STS offers the benefit of being palpable and easy to identify and target on US imaging, with no overlying structures blocking the acoustic window and/or causing excessive attenuation of the energy delivered to the ablation focus [Citation42].
Tumor location
Tumor location is an important clinical consideration for histotripsy treatment planning. Superficial tumors are more likely to be affected by pre-focal cavitation on the skin during histotripsy ablation, where residual hairs can trap air and seed cavitation on the skin. Extremity STS and OS, especially in the distal extremities, are closely associated with the overlying skin, and thus are at an increased risk of experiencing pre-focal cavitation trauma to the skin. This issue can typically be managed by ensuring thorough hair removal over the targeted area [Citation42–44]. Tumors where the overlying skin is ulcerated and/or lacks a continuous flat or arced surface, such as large extremity STS tumors, present an additional challenge with risk of injury from pre-focal cavitation, or potential thermal effects at aggressive histotripsy sequences similar to what has been reported for extremity HIFU ablations [Citation109]. Therefore, device improvements need to be made to decrease the risk of injury from pre-focal cavitation, especially for tumors located close to the overlying skin. Additionally, due to the set-up required for histotripsy treatment, the tumor must be adequately accessible for the water bolus and therapy transducer to be placed (). Based on the experiences reported in the aforementioned treat-and-resect studies, OS and STS tumors of the antebrachium and crus were the least challenging to position for transducer accessibility and medial humeral and femoral tumors were the most challenging to position. Improvements in the positioning dexterity of the histotripsy transducer and water bolus are needed to increase accessibility to medially located tumors of the humerus and femur.
Tumor size
Tumor size is another important clinical consideration for histotripsy treatment planning, as the current rate of histotripsy ablation used for the aforementioned OS and STS canine clinical studies would lead to ablation durations that are not clinically recommended due to the length of time patients would need to be maintained under general anesthesia. The large sizes that STS and OS tumors can sometimes manifest translate into the need for improvements and technological advancements in histotripsy delivery systems for rapid large tumor volume ablations of extremity sarcomas. It has previously been reported that treating a spherical tumor volume of a diameter of 3 cm in an STS tumor took approximately 1 h [Citation42] and treatment of 2 cm diameter spherical volume of an OS tumor took approximately 30 min with the reported device, but a treatment of a 3 cm diameter sphere is expected to take over 2 h [Citation43,Citation44]. Previous histotripsy investigations report STS and OS tumor diameters as large as 8–12 cm, suggesting a full tumor ablation would take >3 h with the reported histotripsy system and parameters [Citation42–44]. Ablation durations of 30-–60 min for 3–6 cm3 canine soft tissue sarcomas using US-guided thermal HIFU [Citation39], and 30–86 min for 0.46–19.3 cm3 human osteoid osteomas using MR-guided thermal HIFU [Citation110] have been reported. Strategies to increase the speed of histotripsy ablation to reduce treatment duration need to be evaluated while being mindful of the potentially increasing risk of histotripsy-induced thermal damage with more aggressive ablation protocols such as those that utilize high PRFs [Citation111].
Tumor shape
The shape of tumor being targeted also needs to be considered for treatment planning because typically the histotripsy treatment software dictates elliptical or spherical ablation volumes. The elliptical or spherical-shaped treatment pattern is advantageous for numerous tumor types such as liver tumors, but when targeting irregularly shaped tumors using a spherical or elliptical ablation volume, there is an increased risk of off-target damage to healthy tissue or incomplete tumor ablation. Thermal HIFU delivery systems, which have been developed over a longer period of time compared to histotripsy systems, have the ability to sculpt unique ablation volumes based on real-time US imaging [Citation39]. With time, improvements in histotripsy planning software to allow for more complex treatment volumes will be addressed with the continued advancement of histotripsy ablation for extremity tumors as these tumors are commonly irregularly shaped, especially for OS.
General safety considerations
The associated safety considerations with histotripsy are limited due to its noninvasive modality, tissue-selective properties, and its non-thermal characteristics. However, there are still safety considerations that should be acknowledged and addressed during the continued development of histotripsy ablation. Since the delivery of histotripsy treatment requires the patient to be anesthetized, the duration of the anesthetic event should be limited to a time that does not pose substantially increased health risk to the patient. Previous reports of delivering a histotripsy dose of 500–1000 PPP at a PRF of 500 Hz suggest that this dose does not result in increased patient pain post-treatment or thermal damage, but these risks should be considered when exploring new doses and PRFs above 1000 PPP and 500 Hz, respectively. Additionally, pre-focal cavitation needs to be monitored closely to prevent associated skin damage, especially in superficial tumors. Lastly, since the long-term risks associated with histotripsy ablation remain to be determined pathologic fracture risk for OS patients should be considered during patient selection and treatment planning.
Current engineering limitations and potential solutions
To improve histotripsy ablation efficiency, several factors of the histotripsy system and ablation approach should be considered, including the method by which the cavitation bubble cloud is repositioned throughout the planned ablation volume, the histotripsy delivery rate and pulsing scheme, and imaging modalities besides US for treatment planning and monitoring.
Mechanical and electronic steering of the cavitation bubble cloud
The required repositioning of the cavitation bubble cloud throughout the defined ablation volume can require thousands of miniscule manipulations to deliver a specified histotripsy dose, depending on the size of the cavitation bubble cloud and tumor. Since the cavitation bubble cloud is only millimeters in size, rapid repositioning of the cavitation bubble cloud throughout the ablation period is required to ablate a clinically relevant tumor volume. Current systems utilize robotic positioners to mechanically steer the ablation focus (and bubble cloud) throughout the target volume, which allows for ablation of unique volumes in a highly precise manner. With a robotic positioning system, the efficiency of cavitation cloud repositioning is determined by the robotic positioner’s maximal motor speed, which in turn determines the overall treatment time. Robotic positioning results in precise tumor ablation, but for large tumor volumes, the time it takes to reposition the focus to each point in the ablation volume adds up and increases the overall treatment duration. A possible option for reducing treatment time (and therefore reducing anesthesia duration and related complications) without sacrificing ablation precision is to replace mechanical steering with electronic steering or combine the two techniques. Electronic steering has previously been reported to significantly reduce ablation times compared to mechanical steering [Citation112–114]. The mechanism of electronic steering is nearly instantaneous and does not require actual physical movement of the transducer, but rather activation and deactivation of transducer elements to change the bubble cloud position [Citation112,Citation113,Citation115]. While bubble cloud repositioning with electronic steering is rapid, it requires large histotripsy transducers with hundreds of elements. Furthermore, electronic steering has limited repositioning capabilities as positions are limited to those which can be configured by the activation of the number of elements required to generate sufficient pressure to generate a bubble cloud [Citation112]. Combining electronic and mechanical steering may present an optimal approach to reduce histotripsy treatment times [Citation113]. Future studies are needed to develop this approach and assess the risk of the development of thermal effects related to electronic steering.
Altering histotripsy pulsing schemes
Another potential solution to reduce treatment times is to increase the PRF at which histotripsy is applied. Investigating the use of PRFs >1000 Hz to ablate large tumors and/or stiff tissues may enable more efficient ablation of extremity tumors, but increasing the PRF also carries the risk of inducing thermal effects [Citation116]. The potential thermal effects from histotripsy at high PRFs must be considered and managed when developing aggressive pulsing schemes for efficient volumetric ablation of large and/or stiff tumors.
Another option to increase histotripsy efficiency in large tumors is the use of gradient doses tailored to the composition of each targeted tumor [Citation117]. As previously mentioned, appendicular OS tumors are often heterogeneous, and soft regions of the tumors require a lower treatment dosage than stiffer regions. By implementing real-time feedback of tissue breakdown throughout treatment, the cavitation bubble cloud could be repositioned from point to point within the tumor more efficiently throughout treatment. Additionally, gradient dose ablation strategies could be implemented based on pretreatment magnetic resonance imaging (MRI) planning [Citation117,Citation118]. Overall, more complex and aggressive pulsing and gradient dosing schemes to enhance histotripsy ablation efficiency need to be investigated to overcome the challenges posed by the heterogeneity of extremity tumors such as OS.
Imaging modalities for histotripsy ablation
CT or MRI imaging are effective modalities for characterizing soft tissue and bone tumors to create an overall histotripsy treatment plan for canine extremity OS and STS prior to the ablation session [Citation42–44]. During the histotripsy ablation session, the use of US is a common imaging modality to monitor histotripsy ablation as it provides real-time feedback and it offers increased accessibility due to its portable size compared to other imaging modalities such as CT and MRI. In the context of extremity tumors, US imaging is particularly useful for STS as the soft tissue composition of those tumors provides an ideal acoustic window for not only real-time visualization of the tumor but also the histotripsy bubble cloud. In tumors composed of soft tissues, the bubble cloud is clearly visualized with US imaging as an oscillating hyper-echoic region. While the utility of US imaging for treatment planning and monitoring for soft tissue tumors such as STS is effective, tumors composed of bone such as OS may require different real-time imaging modalities. Bone can reflect and absorb US energy creating imaging artifacts and blocking the visualization of structures deep to the bone [Citation119]. This results in poor visibility of the histotripsy bubble cloud when ablating bone tumors primarily encased within intact cortical bone. However, bone tumors with expansile soft tissue components and/or lytic bone offer notably clearer visualization of the bubble cloud on US imaging ().
Alternatives and advancements for monitoring of histotripsy ablation bone tumors
Since US imaging provides adequate visualization of the cavitation cloud in STSs, this section is focused on alternatives for real-time image monitoring of histotripsy ablation for bone tumors. In the reported primary bone tumor canine study, single-element PCD was used to indirectly monitor the cavitation bubble cloud when it was not visible on US [Citation44]. Single-element PCD describes a process where one of the transducer’s elements is connected to an oscilloscope via a high-voltage probe and used to detect the presence of cavitation in the focal region [Citation93,Citation120]. Single-element PCD does not provide any definitive spatial information regarding whether the bubble cloud is being generated at the targeted location within the tumor.
One advanced PCD technique that could improve indirect visualization of histotripsy cavitation and the resulting ablated tissue is an implementation of a full-transducer transmit-and-receive PCD system [Citation48]. Transmit-and-receive histotripsy systems are equipped with transducers that have elements that can both send histotripsy pulses and detect cavitation signals. These systems have previously been employed for histotripsy ablation in the brain and can detect cavitation signals and monitor tissue liquefication via cavitation collapse time [Citation108,Citation121,Citation122]. Improved PCD methods could improve 3D treatment visualization and spatially locate bubble clouds based on cavitation signals to assist in monitoring for pre-focal cavitation and off-target damage for tumors that are difficult to image with the US. The use of multi-element PCD for 3D passive cavitation mapping, particularly for tumors that are difficult to visualize with US imaging, provides an alternative for monitoring cavitation but does not allow for visualization of the tumor; therefore, modalities such as CT or MRI should also be utilized for tumor visualization ().
Clinically, MRI has been shown to delineate bone tumors in more detail than CT [Citation123,Citation124] and may represent a better imaging approach for histotripsy treatment planning and monitoring. MRI has been previously investigated to assess damage following histotripsy ablation of soft tissues, with clear differences seen between treated and untreated tissues [Citation55,Citation95,Citation102,Citation111,Citation125]. MRI has also been preliminarily investigated for real-time monitoring of histotripsy-induced cavitation bubble clouds but requires MR-compatible histotripsy systems [Citation117,Citation118,Citation126]. The current limited availability of MR-compatible histotripsy delivery systems causes challenges to access such technology. Encouragingly, thermal ablation zones induced by high intensity focused ultrasound (HIFU) have been visualized on MRI [Citation59]. Therefore, MRI has promise as a real-time imaging modality to monitor histotripsy ablation of extremity tumors in the future. MRI also has the potential to be used for treatment planning and for evaluation of post-histotripsy ablation efficacy, ablation lesion resorption over time, and tumor progression status.
Immunomodulation and histotripsy ablation
In addition to histotripsy’s potential as a non-surgical limb salvage technique for extremity tumors, another clinically beneficial feature of histotripsy ablation is its reported ability to induce immunomodulation within the targeted tumor microenvironment (TME) and subsequently stimulate an anti-tumor immune response [Citation99–102,Citation127–130]. The stimulation of a local and systemic anti-tumor immune response can result in immune targeting of non-ablated tumor cells within the TME to induce cell death and to promote anti-tumor immune memory that mitigates metastatic development. Often, an immunosuppressive TME allows for a permissive environment that promotes the development of metastatic disease. Thus, reprogramming the TME to stimulate an anti-tumor immune response that reduces metastatic development has a high potential to improve the survival prognosis for highly metastatic tumors such as OS. Even though STS are not as aggressively metastatic as OS, they can still benefit from the potential immunomodulation of the TME after histotripsy ablation. STSs are generally considered immunologically unresponsive in people and dogs, but subsets of immunologically responsive STSs are reported to be associated with significantly improved survival times [Citation131,Citation132]. Histotripsy’s immunomodulatory potential is expounded on below.
The non-thermal homogenization of histotripsy targeted tumor tissue causes the release of non-denatured immunogenic cellular proteins such as tumor-associated antigens and damage-associated molecular patterns (DAMPs), all of which serve to activate the immune system to recognize and eliminate tumor cells [Citation45,Citation100–102,Citation129,Citation133,Citation134]. As a non-thermal ablation modality, histotripsy has a hypothetical advantage over thermal ablation, which leads to heat-denaturation of released cellular proteins, potentially decreasing the immunogenicity and the stimulation of anti-tumor immune response by these proteins [Citation135]. A previous in-vitro investigation has reported that exposure of M2 macrophages to cancer cells ablated with boiling histotripsy resulted in the reprogramming of M2 macrophages into M1 macrophages [Citation128]. Additionally, previous rodent model investigations have reported an increase in cytotoxic T-lymphocyte (CTL) infiltration both locally at the primary tumor and in untreated contralateral tumors within 3 days post histotripsy treatment [Citation101]. In longer term studies, the abscopal immune response postulated to be associated with histotripsy was reported to decrease pulmonary metastases within 20 days post-histotripsy treatment in a preclinical melanoma model [Citation101]. In preclinical models of hepatocellular carcinoma and melanoma, tumor regression is reported after ablation of 50–70% of tumor volume [Citation102], and reduction of contralateral tumor volume was observed [Citation100], further supporting the potential for immune activation after histotripsy tumor ablation. In addition to the observation of the abscopal effect of histotripsy in the preclinical setting, this phenomenon was also observed in the first human liver clinical trial in which untreated tumors, distant from the histotripsy-treated tumor, decreased in size [Citation55,Citation136]. Canine studies have reported immune activation 24 h post histotripsy ablation in a canine OS pilot study [Citation43] and in STS [Citation42]. These data support histotripsy’s exciting potential to stimulate anti-tumor immune activity and the possibility of subsequent synergism between histotripsy and adjuvant therapies such as chemotherapy and immunotherapy.
Conclusions
There is a profound need for novel tumor treatment options for extremity tumors that yield improved prognosis and quality of life outcomes for both human and veterinary cancer patients. Initial studies reporting on the safety and feasibility of histotripsy ablation for extremity tumors utilizing canine patients with spontaneously occurring OS and STS indicate that histotripsy has promising potential to fulfill this need as a noninvasive treatment that could help patients avoid surgical limb salvage or amputation. The biological similarities between canine and human cancers allow the dog to serve as an informative comparative oncology model to aid in the advancement of clinical translation of histotripsy for extremity tumor ablation for human patients. These initial studies in OS and STS can also aid in translating histotripsy for ablation of other extremity tumor types. Further studies are required for improved device development, imaging, and increased efficiency of delivery of histotripsy ablation for extremity tumors. The results from the veterinary clinical trials demonstrating proof-of-concept for feasibility of targeting and ablating extremity tumors with histotripsy lay the groundwork for future studies to evaluate progression-free survival, overall survival, the long-term impact of treatment near critical anatomical structures such as nerves, and overall tumor regression, and tissue regeneration post histotripsy ablation. The current histotripsy literature supports the potential of histotripsy to generate an anti-tumor immune response which is essential for mitigating both metastatic disease and control of residual gross and microscopic primary disease. However, the ability of histotripsy to control metastatic and residual primary tumor in extremity tumors is undetermined at this time. Hence, future studies continuing to evaluate the histotripsy associated immune response will be crucial for understanding the full ability of histotripsy to mitigate both metastatic disease and local disease recurrence. The continued utilization of canine models of spontaneous extremity tumors will provide the critical data needed for rapid and successful translation of histotripsy into the human clinical space for treating extremity tumors.
Authors’ contributions
A.N.H. and L.R.- Contributed equally to the composition manuscript and are the primary contributors.
A.H.- Contributed to the composition of this manuscript.
E.R.V- Contributed to the composition of this manuscript.
S.K.- Contributed to the composition of this manuscript and is the principal investigator on the canine histotripsy STS study reported in this review.
E.V.- Oversaw the engineering work involved in the canine histotripsy STS and OS studies reported in this review, contributed to manuscript composition and revision.
J.T.- Contributed to the composition and revision of this manuscript, is corresponding author, and is the principal investigator of the canine histotripsy OS studies reported in this review.
Disclosure statement
Eli Vlaisavljevich has an ongoing research partnership and financial relationship with HistoSonics, Inc. Lauren Ruger has an ongoing consulting relationship with Theraclion. No other authors have a conflict of interest to report.
Data availability statement
Not applicable.
Additional information
Funding
References
- Sung H, Ferlay J, Siegel RL, et al. Global Cancer Statistics 2020: GLOBOCAN estimates of incidence and mortality worldwide for 36 cancers in 185 countries. CA Cancer J Clin. 2021;71(3):209–249. doi: 10.3322/caac.21660.
- AlDossary NM, Ostler C, Donovan-Hall M, et al. Non-oncological outcomes following limb salvage surgery in patients with knee sarcoma: a scoping review. Disabil Rehabil. 2022;44(16):4531–4545. doi: 10.1080/09638288.2021.1900409.
- Abu El Afieh J, Gray M, Seah M, et al. Endoprosthetic reconstruction in ewing’s sarcoma patients: a systematic review of postoperative complications and functional outcomes. J Clin Med. 2022;11(15):4612. doi: 10.3390/jcm11154612.
- Smolle MA, Andreou D, Tunn PU, et al. Advances in tumour endoprostheses: a systematic review. EFORT Open Rev. 2019;4(7):445–459. doi: 10.1302/2058-5241.4.180081.
- Grinberg SZ, Posta A, Weber KL, et al. Limb salvage and reconstruction options in osteosarcoma. Adv Exp Med Biol. 2020;1257:13–29. doi: 10.1007/978-3-030-43032-0_2.
- Kaneuchi Y, Yoshida S, Fujiwara T, et al. Limb salvage surgery has a higher complication rate than amputation but is still beneficial for patients younger than 10 years old with osteosarcoma of an extremity. J Pediatr Surg. 2022;57(11):702–709. doi: 10.1016/j.jpedsurg.2022.04.001.
- Tsukamoto S, Kido A, Tanaka Y, et al. Current overview of treatment for metastatic bone disease. Curr Oncol. 2021;28(5):3347–3372. doi: 10.3390/curroncol28050290.
- Mason NJ. Comparative immunology and immunotherapy of canine osteosarcoma. Adv Exp Med Biol. 2020;1258:199–221. doi: 10.1007/978-3-030-43085-6_14.
- Dow S. A role for dogs in advancing cancer immunotherapy research. Front Immunol. 2019;10:2935. doi: 10.3389/fimmu.2019.02935.
- Fenger JM, London CA, Kisseberth WC. Canine osteosarcoma: a naturally occurring disease to inform pediatric oncology. Ilar J. 2014;55(1):69–85. doi: 10.1093/ilar/ilu009.
- Paoloni M, Khanna C. Translation of new cancer treatments from pet dogs to humans. Nat Rev Cancer. 2008;8(2):147–156. doi: 10.1038/nrc2273.
- Rowell JL, McCarthy DO, Alvarez CE. Dog models of naturally occurring cancer. Trends Mol Med. 2011;17(7):380–388. doi: 10.1016/j.molmed.2011.02.004.
- Gustafson DL, Duval DL, Regan DP, et al. Canine sarcomas as a surrogate for the human disease. Pharmacol Ther. 2018;188:80–96. doi: 10.1016/j.pharmthera.2018.01.012.
- Dernell W, Ehrhart N, Straw R, et al. Withrow & MacEwen’s small animal clinical oncology. St Louis, Mo: Saunders Elsevier. 2007:540–567.
- Arnesen K, Gamlem H, Glattre E, et al. Registration of canine cancer. Tidsskr nor Laegeforen. 1995;115(6):714–717.
- Ghert M, Mwita W, Mandari FN. Primary bone tumors in children and adolescents treated at a referral center in Northern tanzania. J Am Acad Orthop Surg Glob Res Rev. 2019;3(3):e045. doi: 10.5435/JAAOSGlobal-D-17-00045.
- Schiffman JD, Breen M. Comparative oncology: what dogs and other species can teach us about humans with cancer. Philos Trans R Soc Lond B Biol Sci. 2015;370(1673):20140231. doi: 10.1098/rstb.2014.0231.
- Simpson S, Dunning MD, de Brot S, et al. Comparative review of human and canine osteosarcoma: morphology, epidemiology, prognosis, treatment and genetics. Acta Vet Scand. 2017;59(1):71. doi: 10.1186/s13028-017-0341-9.
- Paoloni M, Davis S, Lana S, et al. Canine tumor cross-species genomics uncovers targets linked to osteosarcoma progression. BMC Genomics. 2009;10(1):625. doi: 10.1186/1471-2164-10-625.
- Beird HC, Bielack SS, Flanagan AM, et al. Osteosarcoma. Nat Rev Dis Primers. 2022;8(1):77. doi: 10.1038/s41572-022-00409-y.
- Yang Y, Han L, He Z, et al. Advances in limb salvage treatment of osteosarcoma. J Bone Oncol. 2018;10:36–40. doi: 10.1016/j.jbo.2017.11.005.
- LaRue SM, Withrow SJ, Powers BE, et al. Limb-sparing treatment for osteosarcoma in dogs. J Am Vet Med Assoc. 1989;195(12):1734–1744.
- Liptak JM, Dernell WS, Ehrhart N, et al. Cortical allograft and endoprosthesis for limb-sparing surgery in dogs with distal radial osteosarcoma: a prospective clinical comparison of two different limb-sparing techniques. Vet Surg. 2006;35(6):518–533. doi: 10.1111/j.1532-950X.2006.00185.x.
- Mitchell KE, Boston SE, Kung M, et al. Outcomes of limb-sparing surgery using two generations of metal endoprosthesis in 45 dogs with distal radial osteosarcoma. A veterinary society of surgical oncology retrospective study. Vet Surg. 2016;45(1):36–43. doi: 10.1111/vsu.12423.
- Séguin B, O'Donnell MD, Walsh PJ, et al. Long-term outcome of dogs treated with ulnar rollover transposition for limb-sparing of distal radial osteosarcoma: 27 limbs in 26 dogs. Vet Surg. 2017;46(7):1017–1024. doi: 10.1111/vsu.12698.
- Oberoi S, Choy E, Chen YL, et al. Trimodality treatment of extremity soft tissue sarcoma: where do we go now? Curr Treat Options Oncol. 2023;24(4):300–326. doi: 10.1007/s11864-023-01059-2.
- Brooks AD, Heslin MJ, Leung DH, et al. Superficial extremity soft tissue sarcoma: an analysis of prognostic factors. Ann Surg Oncol. 1998;5(1):41–47. doi: 10.1007/BF02303763.
- Sakata K, Johnson FE, Beitler AL, et al. Extremity soft tissue sarcoma patient follow-up: tumor grade and size affect surveillance strategies after potentially curative surgery. Int J Oncol. 2003;22(6):1335–1343. doi: 10.3892/ijo.22.6.1335.
- Bonvalot S, Levy A, Terrier P, et al. Primary extremity soft tissue sarcomas: does local control impact survival? Ann Surg Oncol. 2017;24(1):194–201. doi: 10.1245/s10434-016-5462-2.
- Ferrari A, Miceli R, Meazza C, et al. Soft tissue sarcomas of childhood and adolescence: the prognostic role of tumor size in relation to patient body size. J Clin Oncol. 2009;27(3):371–376. doi: 10.1200/JCO.2007.15.4542.
- Cates JMM. The AJCC 8th edition staging system for soft tissue sarcoma of the extremities or trunk: a cohort study of the SEER database. J Natl Compr Canc Netw. 2018;16(2):144–152. doi: 10.6004/jnccn.2017.7042.
- Trojani M, Contesso G, Coindre JM, et al. Soft-tissue sarcomas of adults; study of pathological prognostic variables and definition of a histopathological grading system. Int J Cancer. 1984;33(1):37–42. doi: 10.1002/ijc.2910330108.
- Dennis MM, McSporran KD, Bacon NJ, et al. Prognostic factors for cutaneous and subcutaneous soft tissue sarcomas in dogs. Vet Pathol. 2011;48(1):73–84. doi: 10.1177/0300985810388820.
- Bray JP, Polton GA, McSporran KD, et al. Canine soft tissue sarcoma managed in first opinion practice: outcome in 350 cases. Vet Surg. 2014;43(7):774–782. doi: 10.1111/j.1532-950X.2014.12185.x.
- Grimer RJ. Size matters for sarcomas!. Ann R Coll Surg Engl. 2006;88(6):519–524. doi: 10.1308/003588406X130651.
- Ramanathan RC, A'Hern R, Fisher C, et al. Modified staging system for extremity soft tissue sarcomas. Ann Surg Oncol. 1999;6(1):57–69. doi: 10.1007/s10434-999-0057-9.
- Lewis JJ, Leung D, Espat J, et al. Effect of reresection in extremity soft tissue sarcoma. Ann Surg. 2000;231(5):655–663. doi: 10.1097/00000658-200005000-00005.
- Stefanello D, Avallone G, Ferrari R, et al. Canine cutaneous perivascular wall tumors at first presentation: clinical behavior and prognostic factors in 55 cases. J Vet Intern Med. 2011;25(6):1398–1405. doi: 10.1111/j.1939-1676.2011.00822.x.
- Carroll J, Coutermarsh-Ott S, Klahn SL, et al. High intensity focused ultrasound for the treatment of solid tumors: a pilot study in canine cancer patients. Int J Hyperthermia. 2022;39(1):855–864. doi: 10.1080/02656736.2022.2097323.
- Kalamaras AB, Wavreille V, Jones SC, et al. Impact of microwave ablation treatment on the biomechanical properties of the distal radius in the dog: a cadaveric study. Vet Surg. 2020;49(7):1388–1395. doi: 10.1111/vsu.13481.
- Salyer SA, Wavreille VA, Fenger JM, et al. Evaluation of microwave ablation for local treatment of dogs with distal radial osteosarcoma: a pilot study. Vet Surg. 2020;49(7):1396–1405. doi: 10.1111/vsu.13491.
- Ruger L, Yang E, Gannon J, et al. Mechanical high-intensity focused ultrasound (histotripsy) in dogs with spontaneously occurring soft tissue sarcomas. IEEE Trans Biomed Eng. 2023;70(3):768–779. Pp. doi: 10.1109/TBME.2022.3201709.
- Ruger LN, Hay AN, Gannon JM, et al. Histotripsy ablation of spontaneously occurring canine bone tumors. IEEE Trans Biomed Eng. 2023;70(1):331–342. doi: 10.1109/TBME.2022.3191069.
- Ruger LN, Hay AN, Vickers ER, et al. Characterizing the ablative effects of histotripsy for osteosarcoma: in vivo study in dogs. Cancers (Basel). 2023;15(3):15. doi: 10.3390/cancers15030741.
- Khokhlova VA, Fowlkes JB, Roberts WW, et al. Histotripsy methods in mechanical disintegration of tissue: towards clinical applications. Int J Hyperthermia. 2015;31(2):145–162. doi: 10.3109/02656736.2015.1007538.
- Xu Z, Hall TL, Vlaisavljevich E, et al. Histotripsy: the first noninvasive, non-ionizing, non-thermal ablation technique based on ultrasound. Int J Hyperthermia. 2021;38(1):561–575. doi: 10.1080/02656736.2021.1905189.
- Latifi M, Hay A, Carroll J, et al. Focused ultrasound tumour ablation in small animal oncology. Vet Comp Oncol. 2021;19(3):411–419. doi: 10.1111/vco.12742.
- Bader KB, Vlaisavljevich E, Maxwell AD. For whom the bubble grows: physical principles of bubble nucleation and dynamics in histotripsy ultrasound therapy. Ultrasound Med Biol. 2019;45(5):1056–1080. doi: 10.1016/j.ultrasmedbio.2018.10.035.
- Vlaisavljevich E, Maxwell A, Warnez M, et al. Histotripsy-induced cavitation cloud initiation thresholds in tissues of different mechanical properties. IEEE Trans Ultrason Ferroelectr Freq Control. 2014;61(2):341–352. doi: 10.1109/TUFFC.2014.6722618.
- Crum LA, Roy RA, Dinno MA, et al. Acoustic cavitation produced by microsecond pulses of ultrasound: a discussion of some selected results. J Acoust Soc Am. 1992;91(2):1113–1119. doi: 10.1121/1.402638.
- Movahed P, Kreider W, Maxwell AD, et al. Cavitation-induced damage of soft materials by focused ultrasound bursts: a fracture-based bubble dynamics model. J Acoust Soc Am. 2016;140(2):1374–1386. doi: 10.1121/1.4961364.
- Parsons JE, Cain CA, Abrams GD, et al. Pulsed cavitational ultrasound therapy for controlled tissue homogenization. Ultrasound Med Biol. 2006;32(1):115–129. doi: 10.1016/j.ultrasmedbio.2005.09.005.
- Vlaisavljevich E, Kim Y, Owens G, et al. Effects of tissue mechanical properties on susceptibility to histotripsy-induced tissue damage. Phys Med Biol. 2014;59(2):253–270. doi: 10.1088/0031-9155/59/2/253.
- Vlaisavljevich E, Lin KW, Warnez MT, et al. Effects of tissue stiffness, ultrasound frequency, and pressure on histotripsy-induced cavitation bubble behavior. Phys Med Biol. 2015;60(6):2271–2292. doi: 10.1088/0031-9155/60/6/2271.
- Vidal-Jove J, Serres X, Vlaisavljevich E, et al. First-in-man histotripsy of hepatic tumors: the THERESA trial, a feasibility study. Int J Hyperthermia. 2022;39(1):1115–1123. doi: 10.1080/02656736.2022.2112309.
- Vidal-Jove J, Serres-Creixams X, Ziemlewicz TJ, et al. Liver histotripsy mediated abscopal effect-case report. IEEE Trans Ultrason Ferroelectr Freq Control. 2021;68(9):3001–3005. doi: 10.1109/TUFFC.2021.3100267.
- Arnold L, Hendricks-Wenger A, Coutermarsh-Ott S, et al. Histotripsy ablation of bone tumors: feasibility study in excised canine osteosarcoma tumors. Ultrasound Med Biol. 2022;48(7):1356. doi: 10.1016/j.ultrasmedbio.2022.03.012.
- Williams RP, Simon JC, Khokhlova VA, et al. The histotripsy spectrum: differences and similarities in techniques and instrumentation. Int J Hyperthermia. 2023;40(1):2233720.
- Li C, Zhang W, Fan W, et al. Noninvasive treatment of malignant bone tumors using high-intensity focused ultrasound. Cancer. 2010;116(16):3934–3942. doi: 10.1002/cncr.25192.
- Yu W, Tang L, Lin F, et al. Significance of HIFU in local unresectable recurrence of soft tissue sarcoma, a single-center, respective, case series in China. Surg Oncol. 2019;30:117–121. doi: 10.1016/j.suronc.2019.06.004.
- Arrigoni F, Napoli A, Bazzocchi A, et al. Magnetic-resonance-guided focused ultrasound treatment of non-spinal osteoid osteoma in children: multicentre experience. Pediatr Radiol. 2019;49(9):1209–1216. doi: 10.1007/s00247-019-04426-0.
- Chen W, Zhu H, Zhang L, et al. Primary bone malignancy: effective treatment with high-intensity focused ultrasound ablation. Radiology. 2010;255(3):967–978. doi: 10.1148/radiol.10090374.
- Dababou S, Marrocchio C, Scipione R, et al. High-intensity focused ultrasound for pain management in patients with cancer. Radiographics. 2018;38(2):603–623. doi: 10.1148/rg.2018170129.
- Napoli A, Anzidei M, Marincola BC, et al. Primary pain palliation and local tumor control in bone metastases treated with magnetic resonance-guided focused ultrasound. Invest Radiol. 2013;48(6):351–358. doi: 10.1097/RLI.0b013e318285bbab.
- Horise Y, Maeda M, Konishi Y, et al. Sonodynamic therapy with anticancer micelles and high-intensity focused ultrasound in treatment of canine cancer. Front Pharmacol. 2019;10:545. doi: 10.3389/fphar.2019.00545.
- Seward MC, Daniel GB, Ruth JD, et al. Feasibility of targeting canine soft tissue sarcoma with MR-guided high-intensity focused ultrasound. Int J Hyperthermia. 2019;35(1):205–215. doi: 10.1080/02656736.2018.1489072.
- Antoniou A, Evripidou N, Panayiotou S, et al. Treatment of canine and feline sarcoma using MR-guided focused ultrasound system. J Ultrasound. 2022;25(4):895–904. doi: 10.1007/s40477-022-00672-5.
- Kulkarni SS, Shetty NS, Gala KB, et al. Percutaneous radiofrequency ablation of appendicular skeleton chondroblastoma-an experience from a tertiary care cancer center. J Vasc Interv Radiol. 2021;32(4):504–509. doi: 10.1016/j.jvir.2020.07.027.
- Chahal A, Rajalakshmi P, Khan SA, et al. CT-guided percutaneous radiofrequency ablation of osteoid osteoma: our experience in 87 patients. Indian J Radiol Imaging. 2017;27(2):207–215. doi: 10.4103/ijri.IJRI_260_16.
- Lindner NJ, Ozaki T, Roedl R, et al. Percutaneous radiofrequency ablation in osteoid osteoma. J Bone Joint Surg Br. 2001;83-B(3):391–396. doi: 10.1302/0301-620X.83B3.0830391.
- Prud’homme C, Nueffer JP, Runge M, et al. Prospective pilot study of CT-guided microwave ablation in the treatment of osteoid osteomas. Skeletal Radiol. 2017;46(3):315–323. doi: 10.1007/s00256-016-2558-5.
- Thanos L, Mylona S, Galani P, et al. Radiofrequency ablation of osseous metastases for the palliation of pain. Skeletal Radiol. 2008;37(3):189–194. doi: 10.1007/s00256-007-0404-5.
- Yamakado K, Matsumine A, Nakamura T, et al. Radiofrequency ablation for the treatment of recurrent bone and soft-tissue sarcomas in non-surgical candidates. Int J Clin Oncol. 2014;19(5):955–962. doi: 10.1007/s10147-013-0640-8.
- Belfiore MP, Ronza FM, Della Volpe T, et al. Long-term local disease control in a recurrent soft-tissue sarcoma of the thigh treated by radiofrequency ablation. J Surg Oncol. 2015;111(6):708–710. doi: 10.1002/jso.23877.
- Kim C. Understanding the nuances of microwave ablation for more accurate post-treatment assessment. Future Oncol. 2018;14(17):1755–1764. doi: 10.2217/fon-2017-0736.
- Rinzler ES, Shivaram GM, Shaw DW, et al. Microwave ablation of osteoid osteoma: initial experience and efficacy. Pediatr Radiol. 2019;49(4):566–570. doi: 10.1007/s00247-018-4327-1.
- Fan QY, Ma BA, Zhou Y, et al. Bone tumors of the extremities or pelvis treated by microwave-induced hyperthermia. Clin Orthop Relat Res. 2003;406(406):165–175. doi: 10.1097/01.blo.0000037439.23683.9c.
- Fan QY, Zhou Y, Zhang M, et al. Microwave ablation of malignant extremity bone tumors. Springerplus. 2016;5(1):1373. doi: 10.1186/s40064-016-3005-8.
- Zheng K, Yu X, Hu Y, et al. Clinical guideline for microwave ablation of bone tumors in extremities. Orthop Surg. 2020;12(4):1036–1044. doi: 10.1111/os.12749.
- Shu C, Lim M, Fang A. Transarterial embolization and percutaneous ablation of primary and metastatic soft tissue tumors. Life. 2023;13(7). doi: 10.3390/life13071485.
- Ringdahl-Mayland B, Thamm DH, Martin TW. Retrospective evaluation of outcome in dogs with appendicular osteosarcoma following hypofractionated palliative radiation therapy with or without bisphosphonates: 165 cases (2010-2019). Front Vet Sci. 2022;9:892297. doi: 10.3389/fvets.2022.892297.
- Tollett MA, Duda L, Brown DC, et al. Palliative radiation therapy for solid tumors in dogs: 103 cases (2007-2011). J Am Vet Med Assoc. 2016;248(1):72–82. doi: 10.2460/javma.248.1.72.
- Cancedda S, Marconato L, Meier V, et al. Hypofractionated radiotherapy for macroscopic canine soft tissue sarcoma: a retrospective study of 50 cases treated with a 5 × 6 GY protocol with or without metronomic chemotherapy. Vet Radiol Ultrasound. 2016;57(1):75–83. doi: 10.1111/vru.12308.
- Lawrence J, Forrest L, Adams W, et al. Four-fraction radiation therapy for macroscopic soft tissue sarcomas in 16 dogs. J Am Anim Hosp Assoc. 2008;44(3):100–108. doi: 10.5326/0440100.
- Frisch S, Timmermann B. The evolving role of proton beam therapy for sarcomas. Clin Oncol (R Coll Radiol). 2017;29(8):500–506. doi: 10.1016/j.clon.2017.04.034.
- Ciernik IF, Niemierko A, Harmon DC, et al. Proton-based radiotherapy for unresectable or incompletely resected osteosarcoma. Cancer. 2011;117(19):4522–4530. doi: 10.1002/cncr.26037.
- Livi L, Santoni R, Paiar F, et al. Late treatment-related complications in 214 patients with extremity soft-tissue sarcoma treated by surgery and postoperative radiation therapy. Am J Surg. 2006;191(2):230–234. doi: 10.1016/j.amjsurg.2005.09.007.
- Paulino AC, Nguyen TX, Mai WY. An analysis of primary site control and late effects according to local control modality in non-metastatic Ewing sarcoma. Pediatr Blood Cancer. 2007;48(4):423–429. doi: 10.1002/pbc.20754.
- Tierce R, Martin T, Hughes KL, et al. Response of canine soft tissue sarcoma to stereotactic body radiotherapy. Radiat Res. 2021;196(6):587–601.
- Gagnon J, Mayer MN, Belosowsky T, et al. Stereotactic body radiation therapy for treatment of soft tissue sarcomas in 35 dogs. J Am Vet Med Assoc. 2020;256(1):102–110. doi: 10.2460/javma.256.1.102.
- Martin TW, Griffin L, Custis J, et al. Outcome and prognosis for canine appendicular osteosarcoma treated with stereotactic body radiation therapy in 123 dogs. Vet Comp Oncol. 2021;19(2):284–294. doi: 10.1111/vco.12674.
- Martin TW, LaRue SM, Griffin L. CT characteristics and proposed scoring scheme are predictive of pathologic fracture in dogs with appendicular osteosarcoma treated with stereotactic body radiation therapy. Vet Radiol Ultrasound. 2022;63(1):82–90. doi: 10.1111/vru.13033.
- Vlaisavljevich E, Lin KW, Maxwell A, et al. Effects of ultrasound frequency and tissue stiffness on the histotripsy intrinsic threshold for cavitation. Ultrasound Med Biol. 2015;41(6):1651–1667. doi: 10.1016/j.ultrasmedbio.2015.01.028.
- Vlaisavljevich E, Maxwell A, Mancia L, et al. Visualizing the histotripsy process: bubble Cloud-Cancer cell interactions in a tissue-mimicking environment. Ultrasound Med Biol. 2016;42(10):2466–2477. doi: 10.1016/j.ultrasmedbio.2016.05.018.
- Vlaisavljevich E, Greve J, Cheng X, et al. Non-invasive ultrasound liver ablation using histotripsy: chronic study in an in vivo rodent model. Ultrasound Med Biol. 2016;42(8):1890–1902. doi: 10.1016/j.ultrasmedbio.2016.03.018.
- Knott EA, Zlevor AM, Hinshaw JL, et al. A comparison study of microwave ablation vs. histotripsy for focal liver treatments in a swine model. Eur Radiol. 2023;33(2):1050–1062. doi: 10.1007/s00330-022-09112-8.
- Hall TL, Hempel CR, Wojno K, et al. Histotripsy of the prostate: dose effects in a chronic canine model. Urology. 2009;74(4):932–937. doi: 10.1016/j.urology.2009.03.049.
- Hall TL, Kieran K, Ives K, et al. Histotripsy of rabbit renal tissue in vivo: temporal histologic trends. J Endourol. 2007;21(10):1159–1166. doi: 10.1089/end.2007.9915.
- Hendricks-Wenger A, Hutchison R, Vlaisavljevich E, et al. Immunological effects of histotripsy for cancer therapy. Front Oncol. 2021;11:681629. doi: 10.3389/fonc.2021.681629.
- Pepple AL, Guy JL, McGinnis R, et al. Spatiotemporal local and abscopal cell death and immune responses to histotripsy focused ultrasound tumor ablation. Front Immunol. 2023;14:1012799. doi: 10.3389/fimmu.2023.1012799.
- Qu S, Worlikar T, Felsted AE, et al. Non-thermal histotripsy tumor ablation promotes abscopal immune responses that enhance cancer immunotherapy. J Immunother Cancer. 2020;8(1):e000200. doi: 10.1136/jitc-2019-000200.
- Worlikar T, Zhang M, Ganguly A, et al. Impact of histotripsy on development of intrahepatic metastases in a rodent liver tumor model. Cancers. 2022;14(7):1612. doi: 10.3390/cancers14071612.
- Vlaisavljevich E, Gerhardson T, Hall T, et al. Effects of f-number on the histotripsy intrinsic threshold and cavitation bubble cloud behavior. Phys Med Biol. 2017;62(4):1269–1290. doi: 10.1088/1361-6560/aa54c7.
- Bazzocchi A, Aparisi Gómez MP, Taninokuchi Tomassoni M, et al. Musculoskeletal oncology and thermal ablation: the current and emerging role of interventional radiology. Skeletal Radiol. 2023;52(3):447–459. doi: 10.1007/s00256-022-04213-3.
- Xu Z, Fowlkes JB, Rothman ED, et al. Controlled ultrasound tissue erosion: the role of dynamic interaction between insonation and microbubble activity. J Acoust Soc Am. 2005;117(1):424–435. doi: 10.1121/1.1828551.
- Edsall C, Ham E, Holmes H, et al. Effects of frequency on bubble-cloud behavior and ablation efficiency in intrinsic threshold histotripsy. Phys Med Biol. 2021;66(22):225009. doi: 10.1088/1361-6560/ac33ed.
- Simon A, Robinson F, Anzivino A, et al. Histotripsy for the treatment of uterine leiomyomas: a feasibility study in ex vivo uterine fibroids. Ultrasound Med Biol. 2022;48(8):1652–1662. doi: 10.1016/j.ultrasmedbio.2022.04.214.
- Sukovich JR, Macoskey JJ, Lundt JE, et al. Real-Time transcranial histotripsy treatment localization and mapping using acoustic cavitation emission feedback. IEEE Trans Ultrason Ferroelectr Freq Control. 2020;67(6):1178–1191. doi: 10.1109/TUFFC.2020.2967586.
- Chen W, Wang Z, Wu F, et al. [High intensity focused ultrasound in the treatment of primary malignant bone tumor]. Zhonghua Zhong Liu Za Zhi. 2002;24(6):612–615.
- Yarmolenko PS, Eranki A, Partanen A, et al. Technical aspects of osteoid osteoma ablation in children using MR-guided high intensity focussed ultrasound. Int J Hyperthermia. 2018;34(1):49–58. doi: 10.1080/02656736.2017.1315458.
- Smolock AR, Cristescu MM, Vlaisavljevich E, et al. Robotically assisted sonic therapy as a noninvasive nonthermal ablation modality: proof of concept in a porcine liver model. Radiology. 2018;287(2):485–493. doi: 10.1148/radiol.2018171544.
- Lundt JE, Allen SP, Shi J, et al. Non-invasive, rapid ablation of tissue volume using histotripsy. Ultrasound Med Biol. 2017;43(12):2834–2847. doi: 10.1016/j.ultrasmedbio.2017.08.006.
- Zhang X, Owens GE, Cain CA, et al. Histotripsy thrombolysis on retracted clots. Ultrasound Med Biol. 2016;42(8):1903–1918. doi: 10.1016/j.ultrasmedbio.2016.03.027.
- Lu N, Gupta D, Daou BJ, et al. Transcranial magnetic resonance-guided histotripsy for brain surgery: pre-clinical investigation. Ultrasound Med Biol. 2022;48(1):98–110. doi: 10.1016/j.ultrasmedbio.2021.09.008.
- Lu N, Hall TL, Choi D, et al. Transcranial MR-guided histotripsy system. IEEE Trans Ultrason Ferroelectr Freq Control. 2021;68(9):2917–2929. doi: 10.1109/TUFFC.2021.3068113.
- Wang TY, Xu Z, Hall TL, et al. An efficient treatment strategy for histotripsy by removing cavitation memory. Ultrasound Med Biol. 2012;38(5):753–766. doi: 10.1016/j.ultrasmedbio.2012.01.013.
- Allen SP, Hernandez-Garcia L, Cain CA, et al. MR-based detection of individual histotripsy bubble clouds formed in tissues and phantoms. Magn Reson Med. 2016;76(5):1486–1493. doi: 10.1002/mrm.26062.
- Allen SP, Hall TL, Cain CA, et al. Controlling cavitation-based image contrast in focused ultrasound histotripsy surgery. Magn Reson Med. 2015;73(1):204–213. doi: 10.1002/mrm.25115.
- Scipione R, Anzidei M, Bazzocchi A, et al. HIFU for bone metastases and other musculoskeletal applications. Semin Intervent Radiol. 2018;35(4):261–267. doi: 10.1055/s-0038-1673363.
- Zhang X, Jin L, Vlaisavljevich E, et al. Noninvasive thrombolysis using microtripsy: a parameter study. IEEE Trans Ultrason Ferroelectr Freq Control. 2015;62(12):2092–2105. doi: 10.1109/TUFFC.2015.007268.
- Macoskey JJ, Choi SW, Hall TL, et al. Using the cavitation collapse time to indicate the extent of histotripsy-induced tissue fractionation. Phys Med Biol. 2018;63(5):055013. doi: 10.1088/1361-6560/aaae3b.
- Sukovich JR, Cain CA, Pandey AS, et al. In vivo histotripsy brain treatment. J Neurosurg. 2018:1–8. doi: 10.3171/2018.4.JNS172652.
- Zimmer WD, Berquist TH, McLeod RA, et al. Bone tumors: magnetic resonance imaging versus computed tomography. Radiology. 1985;155(3):709–718. doi: 10.1148/radiology.155.3.4001374.
- Zimmer WD, Berquist TH, McLeod RA, et al. Magnetic resonance imaging of osteosarcomas. Clin Orthop Relat Res. 1986;208(208):289–299. ():doi: 10.1097/00003086-198607000-00050.
- Worlikar T, Mendiratta-Lala M, Vlaisavljevich E, et al. Effects of histotripsy on local tumor progression in an in vivo orthotopic rodent liver tumor model. BME Front. 2020;2020:9830304. doi: 10.34133/2020/9830304.
- Allen SP, Vlaisavljevich E, Shi J, et al. The response of MRI contrast parameters in in vitro tissues and tissue mimicking phantoms to fractionation by histotripsy. Phys Med Biol. 2017;62(17):7167–7180. doi: 10.1088/1361-6560/aa81ed.
- Hendricks-Wenger A, Sereno J, Gannon J, et al. Histotripsy ablation alters the tumor microenvironment and promotes immune system activation in a subcutaneous model of pancreatic cancer. IEEE Trans Ultrason Ferroelectr Freq Control. 2021;68(9):2987–3000. doi: 10.1109/TUFFC.2021.3078094.
- Pahk KJ, Shin CH, Bae IY, et al. Boiling histotripsy-induced partial mechanical ablation modulates tumour microenvironment by promoting immunogenic cell death of cancers. Sci Rep. 2019;9(1):9050. doi: 10.1038/s41598-019-45542-z.
- Schade GR, Wang YN, D'Andrea S, et al. Boiling histotripsy ablation of renal cell carcinoma in the eker rat promotes a systemic inflammatory response. Ultrasound Med Biol. 2019;45(1):137–147. doi: 10.1016/j.ultrasmedbio.2018.09.006.
- Singh MP, Sethuraman SN, Miller C, et al. Boiling histotripsy and in-situ CD40 stimulation improve the checkpoint blockade therapy of poorly immunogenic tumors. Theranostics. 2021;11(2):540–554. doi: 10.7150/thno.49517.
- Petitprez F, de Reyniès A, Keung EZ, et al. B cells are associated with survival and immunotherapy response in sarcoma. Nature. 2020;577(7791):556–560. doi: 10.1038/s41586-019-1906-8.
- Weng W, Yu L, Li Z, et al. The immune subtypes and landscape of sarcomas. BMC Immunol. 2022;23(1):46. doi: 10.1186/s12865-022-00522-3.
- O'Brien MA, Power DG, Clover AJ, et al. Local tumour ablative therapies: opportunities for maximising immune engagement and activation. Biochim Biophys Acta. 2014;1846(2):510–523. doi: 10.1016/j.bbcan.2014.09.005.
- Eranki A, Srinivasan P, Ries M, et al. High-intensity focused ultrasound (HIFU) triggers immune sensitization of refractory murine neuroblastoma to checkpoint inhibitor therapy. Clin Cancer Res. 2020;26(5):1152–1161. doi: 10.1158/1078-0432.CCR-19-1604.
- Joiner JB, Pylayeva-Gupta Y, Dayton PA. Focused ultrasound for immunomodulation of the tumor microenvironment. J Immunol. 2020;205(9):2327–2341. doi: 10.4049/jimmunol.1901430.
- Wah TM, Pech M, Thormann M, et al. A multi-centre, single arm, non-randomized, prospective European trial to evaluate the safety and efficacy of the HistoSonics system in the treatment of primary and metastatic liver cancers (#HOPE4LIVER). Cardiovasc Intervent Radiol. 2023;46(2):259–267. doi: 10.1007/s00270-022-03309-6.