Abstract
Research on fullerenes occupies a unique position in the scientific arena. Synthesis and characterisation of this nanomaterial blur the line between materials science and chemistry; careful tuning of the processing methods gives birth to a whole family of molecules and their functionalised derivatives, whose unusual properties at this nanoscopic scale can be exploited in cutting-edge technological applications. This review focuses on the functionalisation of fullerenes for use in medical applications. The first half gives an introduction to the fullerenes themselves and how their fundamental properties lead to a very rich chemistry, enabling both exohedral (external) and endohedral (internal) functionalisations of the cage. Emphasis is placed on the need for safe and reproducible synthesis routes if fullerenes are ever going to make it to the pharmaceutical market. In line with this, a selection of exohedral functionalisation protocols receives particular attention. Coverage of endohedral fullerene synthesis routes is limited to the endohedral metallofullerenes. In the second half, myriad applications of fullerenes in biomedical contexts are introduced and certain synthesis routes are critically evaluated. Discussion of the need to water solubilise the hydrophobic fullerene cages precedes an overview of fullerene-based diagnostic and therapeutic technologies. A final moment is spent on toxicity studies of fullerenes. The concluding remarks emphasise the positive effects of incorporating fullerenes into biomedical technologies, while looking at how these are perceived by the general public. A case is made for fullerenes being the optimal choice as standard bearers in the advance of nanomaterials into the medical field.
This is the winning review of the 2016 Materials Literature Review Prize of the Institute of Materials, Minerals and Mining, run by the Editorial Board of MST. Sponsorship of the prize by TWI Ltd is gratefully acknowledged.
Fullerenes
Why so interesting?
The fullerenes constitute a distinct allotrope in the family of carbon nanomaterials. Their unique cage-like structure and electron-deficient nature lead to fascinating properties which have made them a promising focus of research in an array of fields. The most abundant fullerene, C60, has a van der Waals diameter of 10.18 Å,Citation1 positioning it well within the realm of nanotechnology. The origin of the fullerenes' key properties and rich chemistry lies in the unique hybridisation of the strained carbon–carbon bonds; while the carbon atoms in graphene and carbon nanotubes (CNTs) are sp2-hybridised to give hexagonal networks, and the tetrahedral network of diamond is sp3-hybridised, the fullerenes are made up of a network of both hexagons (1,3,5-cyclohexatrieneFootnote1) and pentagons ([5]radialene), as shown in Fig. , leading to an average hybridisation of sp2.278.Citation3 The presence of pentagonal rings introduces a significant amount of strain into the network,Footnote2 leading to a rehybridised three-dimensional structure with asymmetric π orbitals and thus three crucial properties.Citation4 First, the fullerenes are excellent electron acceptors, with C60 exhibiting six reversible reductions. Citation5 Second, the exterior of the cages can be decorated using a vast range of chemical reactions. Finally, the relative inertness of the interior of the cages facilitates the capture and isolation of ionic, atomic and even small molecular species. Considerations of both topology and orbital rehybridisation give the fullerenes their unique chemical properties.
1 1,3,5-Cyclohexatriene and [5]radialene sub-units, as found in C60 (double bonds are emitted for clarity)
![1 1,3,5-Cyclohexatriene and [5]radialene sub-units, as found in C60 (double bonds are emitted for clarity)](/cms/asset/e552cee0-38b9-4ac6-9fac-5a1f90cc9d75/ymst_a_1198114_f0001_c.jpg)
Size differences
While the fullerenes are a distinct allotrope of carbon, there is considerable variation within the family: the cages can consist of different numbers of carbon atoms, leading to different shapes and sizes. This increases their versatility and gives researchers considerably greater freedom in tailoring the molecules' properties. C60 is the smallest stable fullerene which obeys the isolated pentagon rule (IPR) – its football-like truncated icosahedron structure (Ih symmetry point group) leads to every carbon atom environment being identical, as elegantly shown by the sole peak in the13C NMR spectrum (Fig. a). This is in contrast to the five peaks for C70 (Figs. b and c). Higher fullerenes exist, with adherence to the IPR a necessity for stability. Bigger cages have an increased number of isomers. This variation leads to differences in shape and symmetry, and makes isomeric isolation difficult and costly.Footnote3Citation4
2 13C nuclear magnetic resonance (NMR) spectra of C60 a and C70 b. Every carbon environment in C60 is identical (all are equally strained in the spherical structure), while there are five environments in C70, corresponding to each successive, increasingly strained ‘layer’ of carbon atoms away from the equatorial plane c. NMR spectra were taken in carbon disulphide (CS2) on a Bruker AVIII 400MHz machine
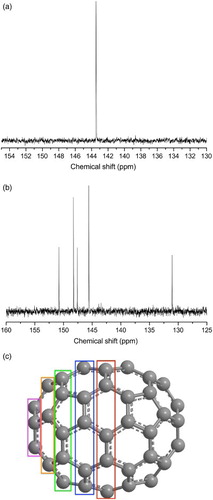
These differences in size and shape have significant effects on both the optical properties (Fig. ) and chemical behaviour of the fullerenes. Solubility decreases with increasing cage size.Citation8 Fullerenes are generally soluble in aromatic hydrocarbons and halogenated solvents, as well as CS2.Citation9, Citation10 The final size effect regards the reactivities of the fullerenes. The primary driving force for addition reactions is the relief of strain in the cage structure and the ensuing return to local sp3 hybridisation. Thus, fullerenes generally exhibit reduced reactivity as the cage size increases; the departure from a curved, strained surface to a more planar, graphitic topology explains this.Citation11
3 The absorption spectra of the fullerenes change as the size of the conjugated system increases: with slight variations depending on solvent, solutions of C60 are an intense purple colour a, C70 red like wine b and C84 a green-yellow. (All above solutions in toluene). Generally speaking, the gap between the highest occupied molecular orbital and the lowest unoccupied molecular orbital decreases with increasing cage size leading to optical absorptions of lower energy, i.e. longer wavelengthCitation7
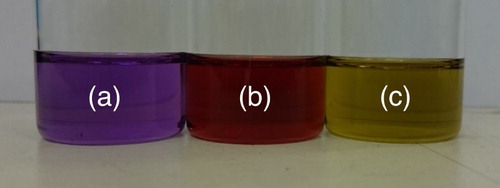
Synthesis
The mechanism of fullerene formation still remains a mystery despite numerous attempts at explanation. Citation12 That has not, however, precluded our ability to produce tens of tons of C60 per year. Citation13 This process put forward by Murayama et al. for Frontier Carbon Corporation (FCC) gives a soluble product mixture of 60% C60 and 25% C70, while the remaining 15% are higher fullerenes up to C96. These are soluble in the organic solvents used for fullerene separation and constitute only 20% of the solid mass retrieved from the reaction chamber; the remaining 80% is insoluble carbonaceous soot. This compares favourably to the first process to produce fullerenes on the gram scale, the standard Krätschmer–Huffman arc discharge, where the fullerene content was and the relative intensities of the fullerene species were similar.Citation14 In this now-standard process, electrical discharge creates a plasma which evaporates graphite in a helium atmosphere. That pioneering work built upon the initial discovery of C60 5 years earlier where it was produced using a low-yield laser ablation method.Citation15 While it is now evident that the production of C60 and C70 has been successfully scaled-up, thus significantly driving down their cost,Footnote4 access to large quantities of higher fullerenes still remains an issue. Even more pressing is the difficulty in scalably synthesising endohedral fullerenes, something of crucial importance to potential future medical technologies.
Both size and synthesis issues impact the processability of different fullerenes and, as will be seen, their derivatives. Practical considerations of solubility, reactivity and scalable production methods play a great role in determining whether a fullerene-based technology can feasibly go to market; this is of particular importance in the pharmaceutical industry and will be touched upon throughout this review.
Exohedral functionalisation
The number of different reactions which have been carried out on fullerenes is immense and barely a fraction of them can be covered in this review.Footnote5 A selection of four reactions which are particularly relevant to the development of water-soluble fullerene derivatives for biomedical applications will be highlighted herein. Each will be examined in more general terms, with specific examples appearing later. It should also be noted that, unless stated otherwise, all reactions in this section were done with C60.
The first two reactions, amination and hydroxylation, are each simple additions of a small polar functional group to the fullerene cage. They both give reasonable water solubilities but suffer from a total lack of control of the precise number of functional groups which are added to the cage.Footnote6 This is a major drawback for their deployment as pharmaceutically relevant reactions as this industry requires stringent control over its chemical structures; each batch of product synthesised must be as pure as possible, not only with respect to reagent impurities.
The second two reactions – the PratoCitation17 and BingelCitation18 reactions, named after their discoverers – are very popular fullerene reactions, lauded for their ability to introduce a vast array of complex functional groups to the fullerene derivative in simple, one-pot processes. For this reason, they have been employed in numerous application areas besides water solubilisation.Citation16 They are such robust reaction protocols that they have also been applied to functionalise CNTs. Citation19,Citation20
Amination
One of the first reactions to be carried out with fullerenes was the direct amination of C60. Citation21 Wudl et al. simply mixed C60 in a series of neat aliphatic primary amines (n-propylamine, t-butylamineFootnote7 and dodecylamine) for 16–24 h, observing a colour change through blue/green to brown. Precise elucidation of the number of adducts in each reaction was not carried out, although an average of six adducts was calculatedFootnote8 for the n-propylamine reaction. Elsewhere, reaction of C60 and C70 with neat, excess, shorter chain primary and secondary amines (methylamine and diethylamine) yielded products containing up to 14 amine groups, as shown by mass spectrometry.Citation22 Amination is an important reaction as it can lead to water-soluble derivatives, as demonstrated by the reaction of C60 with ethylenediamine.Citation21 The reaction mechanism for amination of C60 is described in Fig. ; addition occurs across the [6,6] bond. Interestingly, a density functional theory-based study has shown that the addition of methylamine to the larger C80 cage takes place across the [6,5] bond, with subsequent additions preferentially occurring at [6,5] bonds in pentagons adjacent to the previous attachment site.Citation24 This could have useful consequences for the control of addition reactions to larger fullerenes.
4 Amination mechanism of C60. The first step is single-electron transfer (a fast process) to produce the C60 anion radical which has a characteristic green colour.Citation23 Radical recombination gives a zwitterion which can be stabilised by proton transfer to give the final product (a slow process giving a brown solution)
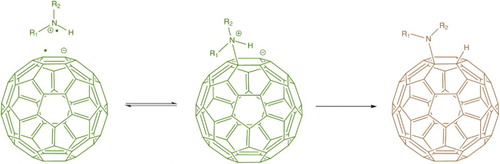
Hydroxylation
The synthesis of polyhydroxylated fullerenes (interchangeably called PHFs, fullerenols and fullerolsFootnote9) was first achieved by Chiang et al.Citation25 who mixed C60 and C70 in an aqueous solution of sulphuric acid and nitric acid (optimum temperatures of 85–115C). An average of 14–15 hydroxyl groups attached to each cage. The more common method of hydroxylation involves stirring a solution of fullerenes in basic conditions. An exceptionally quick reaction time (three minutes) can be achieved by addition of excess aqueous NaOH to a benzene solution of C60 in the presence of a small amount of tetrabutylammonium hydroxide, which acts as a phase-transfer catalyst.Citation26 Other quaternary ammonium hydroxides were tested as catalysts with a markedly less pronounced effect; the reaction took over 96 h in the absence of any catalyst. The average number of hydroxyl groups was
, greater than the product from the acidic conditions of Chiang et al., and this corresponded to an increased water solubility: the greater the number of hydrogen bond donors, the greater the water solubility.
Despite the lack of precise control on the number of addends, hydroxylation has proved a very popular method of water solubilisation for both empty-cage and endohedral fullerenes, and a wide range of synthetic routes exist. Citation27,Citation28
Prato reaction
This 1,3-dipolar cycloaddition of an azomethine ylide to a fullerene gives a stable pyrrolidinofullerene product in good yield. The ylide is a reactive intermediate produced in situ by the condensation of an α-amino acid with an aldehyde or ketone, followed by decarboxylation (Fig. ). The thermodynamically favoured product of this reaction involving C60 has the pyrollidine ring attached across a [6,6] bond, with the kinetically favoured attachment being across the [6,5] bond. Cardona et al.Citation30 showed that these products were reversed (i.e. [6,6] adduct is kinetically favoured, [6,5] thermodynamically) in the case of endohedral metallofullerenes (EMFs) M3N@C80, leading to drastically different electrochemical behaviour.
5 Prato reaction mechanism (after ScarelCitation29). Initial attack of the aldehyde/ketone's polar carbonyl group by the nitrogen lone pair of the amino acid leads to the expulsion of water, before decarboxylation gives the reactive ylide intermediate
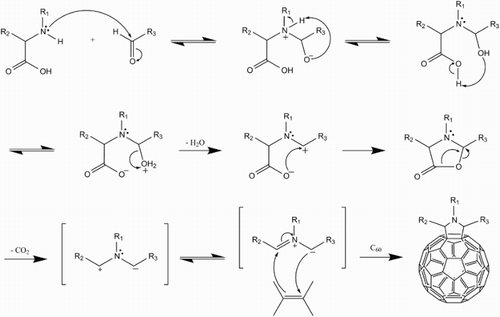
The beauty of the Prato reaction is its ability to introduce multiple functional groups to the fullerene moiety. Referring to Fig. , groups R1, R2 and R3 can be tailored to the desired function. In particular, the possible variability of R1 means the Prato reaction can proceed not only with primary but also secondary amino acids, thus expanding the reaction's applicability.
In contrast with the lack of control of the number of addends in the amination and hydroxylation reactions, good control can be achieved with the Prato reaction by tuning the stoichiometry of reagents and reaction time. Given that all [6,6] bonds on pristine C60 are identical, all mono-adducts of the same addend are likewise identical. Significant regioisomerism and lack of control is, however, introduced on addition of a second addend to form a bis-adduct.Citation31 The second addend has a choice of nine different [6,6] bonds at which to attach, as shown in Fig. , leading to a possible eight regioisomers (presuming the second addend is the same as the first). The difficulty in product purification which this regioisomerism introduces is compounded by the cis/trans isomerism present in pyrollidinofullerenes due to the chiral carbon atoms in the ring either side of the amino group (provided R2, ). This could be of significance when looking at using fullerene derivatives in biomedical contexts as regioisomerism is known to have a significant effect on pharmaco-kinetics and -dynamics. Citation32 In a critical study – significant in deciding which route to take to well-defined fullerene multi-adducts – Lu et al. concluded that bis-addition using the Prato reaction cannot be as well controlled as with the Bingel reaction.Citation33 However, Zhou and WilsonCitation34 used a rigid tether between two azomethine ylides to controllably and selectively synthesise regioisomeric bis-adducts. This technique has found plenty of use with the Bingel reaction, but with the Prato reaction the chiral centres in the pyrrolidine rings introduce stereoisomeric complications.
6 Possible second addend addition sites (adapted after Citation31). There are three sites in the same hemisphere as the initial site of addition, giving rise to cis-regioisomers ,
and
; two equatorial sites, e' and e”; and four addition sites in the other hemisphere, giving trans-regioisomers
,
,
and
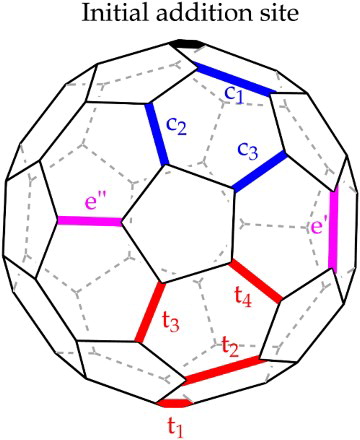
Bingel reaction
Arguably the most popular reaction for exohedral functionalisation of fullerenes, this cyclopropanation involves the addition of an α-halo ester/ketone to the cage under strongly basic conditions (1,8-diazabicyclo[5.4.0]undec-7-ene (DBU) and NaH are most commonly used), yielding a methanofullerene.Citation18 The mechanism of the classic reaction using a bromomalonate is given in Fig. . As with the Prato reaction, functional groups R1 and R2 can be specifically tailored to purpose; on the downside, the issue of regioisomerism in bis-adducts is also common. The popularity of the Bingel reaction, however, arises from versatile modifications of the original reaction, not least the ability to produce the halogenated intermediates in situ, which negates the need for their often time-consuming purification. IodineCitation35 and tetrabromomethane (CBr4)Citation36 have been effectively used for this purpose, with reaction yields of generally 30–60%. Also, unlike the Prato reaction which proceeds at , the Bingel reaction needs mild room temperature conditions.
7 Bingel reaction mechanism. Deprotonation by the strong base gives the nucleophilic malonate anion which then attacks the [6,6] bond. Bromine is then expelled as cyclisation is completed
![7 Bingel reaction mechanism. Deprotonation by the strong base gives the nucleophilic malonate anion which then attacks the [6,6] bond. Bromine is then expelled as cyclisation is completed](/cms/asset/c69eb956-ba6d-444a-84a8-e7ea6342a972/ymst_a_1198114_f0007_b.gif)
Hirsch et al.Citation37 addressed the issue of regioisomerism arising from the Bingel reaction using a somewhat circuitous route to well-defined C60 tris-adducts. After first synthesising the expected range of bis-adducts, the trans-3 (12.0% yield) and e (15.5% yield) regioisomers were isolated and further cyclopropanated. This yielded the tris-adducts seen in Fig. in an impressive 40% yield. The tris-adduct from the trans-3 bis-adduct has all attachments at the trans-3 position (trans-3,trans-3,trans-3) and so symmetry; that from the e bis-adduct has all attachments at the equatorial positions (e,e,e) and so
symmetry. A visible colour difference was also observed: the
product being a more intense orange compared to the red of the
product. These results indicate that functionalisation history affects the subsequent addition of other groups onto the fullerene cage and so can be used to control spatial functionalisation.
8 C60 diethyl malonate tris-adducts. Addition at the trans-3 positions imparts symmetry and produces a red solution; at the equatorial positions,
symmetry and an intense orange solution
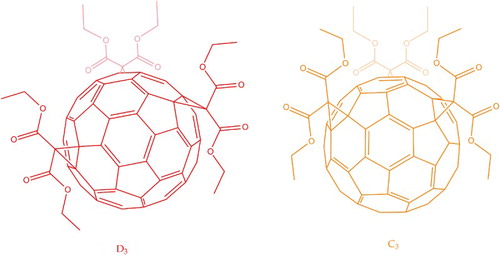
Tethered moieties can also be used to direct functionalisation to specific points on the cage. Sigwalt et al.Citation38 successfully used tert-butyl(trialkoxy)silane tris-malonates to synthesise e,e,e tris-adducts (Fig. ) in 8–26% yield. This method gives a notably increased yield in pure e,e,e tris-adduct final product compared to the functionalisation route via isolation of bis-adducts of Hirsch et al. above. Another attempt at e,e,e tris-adduct isolation, involving a protection–deprotection sequence based on the high-temperature () Diels–Alder reaction, achieved a meagre 4.2% yield in much harsher conditions.Citation39
Fullerene derivatives with even more adducts might be desired for biomedical applications as a greater number of polar groups should give increased solubility. Hexakis-adducts of high symmetry with structurally complex malonatesFootnote10 have been synthesised in good yield (40–68%) by Li et al.Citation40 who used a deceptively simple modification of the standard Bingel reaction. Instead of stoichiometric ratios specific for the desired product, they found that a 100-fold excess of the halogenating agent (in this case, CBr4) preferentially yielded the hexakis adduct.
The introduction of carboxylic acid groups to the fullerene cage is an effective way of increasing the water solubility. However, as in the exemplary case of fullerene malonic acids,Citation41 the carboxylic acid group is often introduced by base hydrolysis after the Bingel reaction has already taken place with a neutral diethyl malonate, as there would otherwise be unwanted acid base interaction. This represents a general issue of how to apply the Bingel reaction when incorporating acidic and base-labile functional groups. A work-around was discovered by Jin et al. who scrapped the need for a basic catalyst.Citation42 Instead they used an amino acid (sarcosine) and DMSO to catalyse the reaction in chlorobenzene (PhCl) solution (Fig. ), giving methanofullerene mono adducts in good yield (33–53%). A potential down side is that relatively large amounts (20 equivalents) of malonate were needed, so the synthesis of these must be scalable with that of the fullerene derivatives.
10 C60 Bingel reaction in the presence of dimethyl sulphoxide (DMSO), with basic catalyst replaced by amino acid (adapted from Jin et al.Citation42). DMSO facilitates single-electron transfer from the amino acid to the fullerene to give the radical cation and radical anion respectively. The fullerene anion then attacks a brominated active methylene, expelling the bromine and forming a fullerenyl radical. Interaction between this and the amino acid radical cation from the first step then finalises cyclisation to give the desired methanofullerene
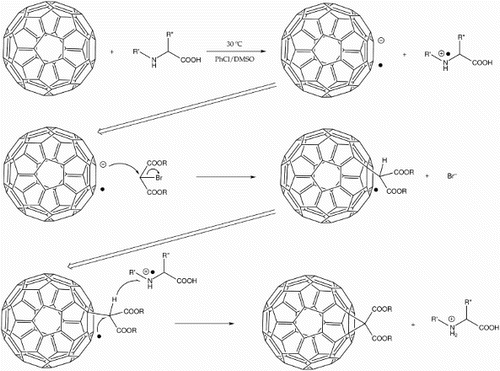
Endohedral functionalisation
The relatively inert interiors of the fullerenes render them able to capture atoms and atomic clusters within. The standard notation X@C2n was introduced by Chai et al.Citation43 to denote endohedral fullerenes, where X is the incarcerated species and n specifies the cage size. Recently, a ‘molecular surgery’ approach, using a series of cage-opening and -closing chemical reactions, has led to the encapsulation of single molecules of hydrogen and water in C60 to give H2@C60 and H2O@C60 (60–78% incorporation, respectively).Citation44 The focus of this section, however, will be on the EMFs, which are synthesised by more established procedures and have found extended use in research on fullerenes for medical applications.Footnote11
Endohedral Metallofullerenes
This is a broad family of endohedral fullerenes where the cage plays host to either one (monometallic) or two (dimetallic) metal atoms or a cluster of metal atoms with another atom, often nitrogen. The presence of large metal atoms within the cage leads to differing reactivities and properties which can be exploited for numerous applications, including those in the biomedical realm.Citation46 In that field, the majority of literature covers the use of gadolinium-containing EMFs as contrast agents (CAs) in magnetic resonance imaging (MRI).
Synthesis. Much like C60, the first EMFs were synthesised using expensive and low-yielding laser ablation.Citation47 Adaptations of the now-standard Krätschmer–Huffman arc discharge method have led to scaled-up synthesis of EMFs: composite graphite rods containing the desired metal oxide (most often lanthanide oxides) are used, however, yields are still generally less than 2% of the total carbonaceous material produced.Footnote12,Citation49 The major product of such a synthesis is monometallic fullerene, M@C82, which has good solubility in common organic solvents and so can be separated from other products using HPLC. It should be noted that the first step of EMF isolation before HPLC separation is its extraction, using a standard Soxhlet method, from the carbon soot mixture by use of polar solvents such as dimethyl formamide (DMF). This is because the incarcerated metal atom does not sit centrally in the elongated cages of larger fullerene cages, giving the molecule an overall electric dipole moment. Kozlov et al.Citation50 optimised this standard process to produce Gd@C82 of excellent purity (99%).
Stevenson et al.Citation51 synthesised Sc3N@C80, the first trimetallic nitride template (TNT) EMF, in a marginally better 3–5% yield by introducing a small amount of nitrogen to the arc reactor. Their synthesis was vastly improved by Dunsch et al.Citation52 whose ‘reactive gas atmosphere’ concept – the addition of ammonia into the reactor – gave EMFs as the majority fullerene product for the first time. The composition of the TNT EMFs can be generalised to XxYyM3-x-yN@C80, where X, Y and M are different metallic species. These species exist because the nitride clusters have been found to stabilise the high-symmetry -C80 cage.
A further step towards commercial synthesis of TNT EMFs was achieved by Bezmelnitsyn et al.Citation53 whose novel approach to arc discharge, they estimate, has reduced the cost of lutetium-containing TNT EMF synthesis by 70–80%. A three-fold increase in yield was achieved by using a pyramidal three-phase AC arc discharge setup (cf. the standard two-electrode DC setup) along with a pure nitrogen atmosphere (negating the need for expensive helium) and metal oxide powder injection with solid graphite electrodes.
Exohedral functionalisation. The exohedral functionalisation of EMFs is even less trivial than that of empty-cage fullerenes.Footnote13 Entrapped metallic species transfer electrons to the carbon cage, with this excess electron density inhomogeneously distributed over the cage, and even extending out of it – a phenomenon dubbed ‘spin leakage’. Citation55 This has profound effects on the chemistry of the EMFs.Citation46 Prato reactions have been carried out on La@C82 to yield mono- and bis-adducts, with electron paramagnetic resonance measurements showing slight modification of the electronic structure upon mono-addition, and significant modification upon bis-addition.Citation56 Bingel cyclopropanation was used by Bolskar et al.Citation57 to isolate a derivative of the otherwise insoluble Gd@C60. This is of value as the Gd@C60 fraction of the arc discharge soot is more abundant than Gd@C82 but could never be isolated due to its insolubility. A 15-fold excess of diethyl bromomalonate yielded primarily the decakis-adduct, Gd@C60[C(COOCH2CH3)2]10, which was hydrolysed to the water-soluble Gd@C60[C(COOH)2]10. This has been investigated as an MRI CA. Other Gd-EMF MRI CAs, which are examined later, have primarily been hydroxylated to achieve water solubility.
Fullerenes in medicine
Herein, a selection of water solubilisation methods are first covered in an attempt to discover which offer the most promise as potential platforms for medical tools. We then give a run-down of medical uses for which fullerenes have been investigated, offering a critical opinion as to which seem to show the most promise.
Water solubilisation
Pristine fullerenes are highly hydrophobic and so cannot be deployed in the body given that water is the primary constituent of blood into which medicines are delivered intravenously.Footnote14 Addition of hydrophilic, polar groups which are capable of hydrogen bonding with water molecules are the key to achieving water solubility; in turn, water solubility is the key to a longer retention time in the bloodstream due to decreased recognition as a foreign body by the immune system.
Covalent functionalisation is the surest way to producing fullerene-based systems viable for biomedical application as the structures of such molecules can be precisely defined (using X-ray crystallography) and their pharmaco-kinetic and -dynamic behaviour better understood and fine-tuned. This is in comparison with two other methods of water solubilisation:
Encapsulation in water-soluble hosts – Calixarenes are a good example of this type;Citation58
Supramolecular/covalent incorporation into water-soluble polymers – Giacalone et al.Citation59 give a good overview.
The major issues with these two methods are: the lack of definition of molecular structure in solution; inhomogeneity of samples;Footnote15 and unpredictable side effects from the water-solubilising component(s). Iwamoto and YamakoshiCitation60 synthesised C60–NVP (Fig. ) with one of the highest water-solubilities (7.8 mM) of any fullerene derivative. However, being a polymeric system, it contains a spread of differently sized molecules ranging from 30 to 50 kDa, which is not specific enough to be scaled up to pharmaceutical scale.
An easy route to water-soluble C60 was first devised by Lamparth and HirschCitation41, who recognised the need to isolate single regioisomers for investigation in biomedical studies. Thus, they took their previously synthesised and
tris-adducts and hydrolysed them to the respective malonic acids by stirring in 20-fold excess of NaH for 3 h at
. These products were very soluble, even in neutral water, and bis- and mono-adducts were also hydrolysed to confirm that water solubility did indeed increase with the number of carboxylic acid groups. These tris-adducts have been extensively studied for a variety of biomedical applicationsFootnote16 but there is a fear that the malonic acid groups are actually unstable under physiological conditions, leading to cytotoxic side products.Citation61
Another successful water solubilisation via the Bingel reaction was achieved by Brettreich and Hirsch.Citation62 Their dendro[60]fullerene mono-adduct (Fig. ) gave the highest water solubility to date (12 mM). It signifies a different approach to the water solubilisation of fullerenes as it utilises the dendritic nature of the addend to introduce many polar groups and hydrophilicity, rather than adding the same small number of polar groups via multiple addends.
An early attempt to synthesise water-soluble fullerenes via the Prato reaction was not completely successful.Citation63 The introduction of two triethylene glycol chains off the pyrrolidine ring of the mono-adduct (34% yield) gave a poor upper limit to solubility of in a 9:1 water-DMSO binary solvent. Positively, however, this derivative showed promising anti-bacterial and anti-fungal activity. Aroua et al. used the Prato reaction to synthesise a versatile mono-adduct bis-carboxylic acid building block (Fig. ) which was made water soluble (
) by subsequent PEGylation or reaction with the peptide sequence, GABA-GPLGVRGA.Footnote17,Citation64 Dynamic light scattering measurements confirmed a mono-modal distribution of aggregate particle diameter of
. This small size and mono-modal distribution is required for biomedical applications so that the fullerene derivative can act efficiently and without unwanted aggregation effects.
Hydroxylation is a very popular water-solubilisation method despite its lack of specificity. In their synthesis of Gd@C82(OH)x, Chen et al.Citation65 obtained a final product with a narrow distribution in the number of hydroxyl groups by selectively collecting only a cropped portion of HPLC fraction.
Diagnostics
Two non-invasive imaging techniques are investigated below, with readers directed elsewhere for a comprehensive discussion of such techniques.Citation66
Magnetic resonance imaging
The paramagnetic gadolinium(III) ion (Gd3+) is used in MRI CAs because its large magnetic moment and seven unpaired, isotropically distributed electrons (the highest number for any element) dramatically reduce the
and
relaxation timesFootnote18 of any surrounding water protons, thus giving increased contrast in images. Citation67,Citation68 The percentage change in
is greater than in
and so the best visualisation is in so-called
-weighted images.Citation69 In order for Gd3+ to enter the body, chelating agents are used to strongly bind the ion; several different chelating agents are currently available on the market and are used every day in hospitals across the world.Citation70 However, these chelating agents have limitations which it is hoped Gd-EMFs can alleviate. First, there is evidence that the highly toxic gadolinium ion can escape chelates in sufficient quantities to cause fatal damage to patients with renal failure. Citation71,Citation72 By capturing Gd3+ within a stable fullerene cage, there is no chance of escape and so that problem is negated. Second, as shown below, the relaxivity effects of Gd-EMFs can be over an order of magnitude greater than clinically available chelate-based CAs, meaning that lower doses can be administered for the same improvement in image contrast.Citation73 This might at first seem unexpected given that water molecules cannot directly interact with the gadolinium ion as it is shielded by the carbon cage, thus getting rid of all inner-sphere contributions to the relaxivity. Sitharaman et al.Citation74 found that Gd@C60[C(COOH)2]10 and Gd@C60(OH)x form larger aggregatesFootnote19 as the pH of the aqueous solution decreases. Thus they proposed that the large outer-sphere relaxivities observed are due to the slow tumbling of the aggregates formed.
Adding further evidence to this hypothesis, Zhang et al.Citation75 also observed a dependence of the relaxivities of the Gd3N@C80[DiPEG(OH)x] on aggregate size. An optimum PEG chain weight of 350 Da gave aggregates of 95-,nm size and an of 237 mM−1 s−1 (2.4 T magnetic field), which compares very favourably to the r
1 values of low-molecular weight Gd3+-chelates which are typically 3–5 mM−1 s−1.Citation76 Laus et al.Citation77 note, however, that the formation of aggregates of Gd-EMFs can be disrupted, and so relaxivities reduced, by the addition of salts commonly found in the body (e.g. phosphates). Relaxivities were though still generally an order of magnitude greater than commercially available chelates.
This phenomenon of exceptional values is not limited to Gd-TNT EMFs, as Mikawa et al.Citation78 obtained an
value of 81 mM−1 s−1 (1.0 T magnetic field) using Gd@C82(OH)x. Other examples of promising Gd-EMF MRI CAs have been synthesisedCitation79,Citation80 and Ghiassi et al.Citation81 give a comprehensive outlook on the field.
Zhang et al.Citation82 synthesised two novel water-soluble Gd-EMFs also containing scandium, which again showed improved relaxivities compared to commercial agents. ScxN@C80
(OH)n (where
;
), respectively, gave
values of 20.7 and 17.6 mM−1 s−1. This is an important result as Scx
N@C80 was synthesised in greater yields than both Gd@C82 and Gd3N@C80, and was actually the third most abundant fraction from the arc discharge soot, after C60 and C70.
A remarkably high relaxivity of 368.7 mM−1 s−1 (1.5 T magnetic fieldFootnote20) was achieved by combining pristine Gd@C82 and water-soluble graphene oxide (GO).Citation83 π–π interactions between the GO and Gd-EMF are thought to facilitate electron transfer between the two and so help increase the observed relaxivity. While this nanohybrid is an exemplary system for achieving high relaxivities, it suffers from a lack of precise definition of what comprises the sample (e.g. the number of fullerenes per GO sheet).
Finally, Wang et al.Citation84 utilised C60 as a central tethering moiety for the attachment of 4–5 Gd-DOTAFootnote21 chelates (Fig. ). While admirable for exploiting C60 as a three-dimensional chemical anchor and achieving an improved relaxivity of 49.7 mM−1 s−1, this novel derivative does not solve the issue of gadolinium escaping from its chelated state.
Deploying EMFs as MRI CAs is without doubt one of the most saturated spaces in biomedical fullerene research. Given the significant improvement in relaxivity which Gd-EMFs offer when compared with currently used Gd-chelates, and the lack of Gd-toxicity due to incarceration within the fullerene cage, it seems likely that this could be one of the first places we see fullerenes deployed as a biomedical tool. Improvements in synthesis routes, however, need to be achieved – specifically, higher-yielding arc discharge methods need to be scaled-up and controllable exohedral functionalisation must be achieved.
X-ray imaging
Wharton and WilsonCitation85 first developed a highly iodinated C60 derivative (Fig. ) for use as an X-ray imaging CA, mimicking the highly iodinated and highly water-soluble structure of commercially available Iohexol®. Using C60 as a three-dimensional central anchor, Bingel cyclopropanation was initially used to add the highly iodinated addend before four further cyclopropanations introduced polar groups responsible for an impressive water-solubility of .
In contrastFootnote22 to this exohedral introduction of multiple iodine atoms, Miyamoto et al.Citation86 investigated a range of fullerenol EMFs as X-ray CAs – it was hoped that these would abolish the need for iodinated agents, which can cause severe issues for patients with an allergy to iodine. The structures investigated – M@C82(OH)40 where M = Dy, Er, Gd, Eu or Lu2 – unfortunately, however, did not produce contrast comparable to that of commercially available agents.
Therapeutics
Human immunodeficiency virus-1 (HIV-1) protease inhibitor
The first posited medical application of C60 was as an inhibitor of human immunodeficiency virus-1 (HIV-1) protease, as the fullerene core is ideally suited to interact with the receptor site of the enzyme due to its hydrophobic surface and spherical shape.Citation87 By blocking the receptor site, the cleaving of proteins required for HIV replication and infection can no longer take place. In this initial work, Friedman et al. used water-soluble C60 derivatised with bis(phenethyl-aminosuccinate) before suggesting, with the evidence of modelling, that direct amine additions to the cage could give better performance. A further modelling study by Tzoupis et al.Citation88 aimed to design fullerene derivative inhibitors by considering hydrogen-bond patterns, the regions of the receptor site most important for binding and contributions to the binding free energy. Recent work by Strom et al.Citation89 has shown that C60-based amino acids can be further functionalised and their inhibition capability tuned by adding specially designed peptide sequences. Aside from inhibition of the protease enzyme, Tanimoto et al.Citation90 actually managed to degrade and thus render inactive HIV-1 protease by irradiating (365 nm) sugar-functionalised C60 which had docked in the enzyme. This photodynamic effect is discussed further below.
Antioxidants
The role of oxidative stress – the unregulated and excessive proliferation of radicals, such as reactive oxygen species (ROS), within the body – in the pathogenesis of human disease cannot be overstated.Citation91 While ROS, created by the partial reduction of oxygen,Footnote23 are naturally produced by metabolic processes within the body and have even been found to play a key part in cellular signalling, deviation from homeostasis due to an increase in ROS levelsFootnote24 or a decrease in inherent antioxidant capability leads to problems.Citation93 Such problems include promotion of tumour metastasis, Citation94 neurodegenerationCitation95 and other degenerative diseases associated with aging. Citation96
Fullerenes have attracted much attention as potential antioxidant therapeutic agents due to their high capacity for radical quenching – they readily accept the lone electrons of radicals into their extended conjugated system. Beuerle et al.Citation61 offer an excellent discussion on the efficacies of various fullerene derivatives in quenching a range of ROS. In groundbreaking work, Dugan et al.Citation97 showed that solutions of tris-malonic acids
and
eliminated all superoxide radicals generated in situ, and that the
derivative in particular showed promising neuroprotective behaviour thanks to its antioxidant ability.Citation98 Further work from the same group showed that
, when administered to non-human primates with Parkinson's disease, was responsible for improved motor function. Citation99 These results and a myriad other studies looking at
have established it as the poster-boy fullerene antioxidant, thus arguably propelling the field closer to market.Citation61
Dendrofullerenes of first and second generation (see Figs. and , respectively) were required in lower doses than both and
for effective superoxide quenching, seemingly confirming that mono-adducts provide better antioxidant functionality thanks to a more intact conjugated system.Citation100
Away from protection of the central nervous system and only superoxide quenching, Yin et al.Citation101 found that PHFs offered better cytoprotection than bis-malonic acid, C60(C(COOH)2)2. The protective effects of Gd@C82(OH)22 were found to be greater than its empty-cage C60 analogue, C60(OH)22 and both were found to scavenge a range of physiologically relevant ROS. The radical scavening ability of fullerenols has even been used to treat lower back pain effectively.Citation102
Photodynamic therapy (PDT)
Photodynamic therapy (PDT) is lauded as an attractive cancer therapy for localised smaller lesions and carcinomas, as well as an aid during surgery of larger masses, thanks to its relative non-invasiveness, precise targeting and lack of long-term side effects.Citation103 It works by irradiating an intravenously administered photosensitising molecule at a particular wavelength, which excites it into its very short-lived (nanosecond scale) first excited singlet state, S1. Non-radiative decay of this S1 state gives the longer-lived (up to milli-second scale) triplet state, T1, which is subsequently quenched.Citation104 In the case of molecular oxygen, quenching by energy transfer leads to the formation of a highly reactive and cytotoxic ROS: singlet oxygen ().Citation105 Thus, the effectiveness of PDT relies on the evolution of singlet oxygen in areas with a high density of cancerous cells.
Fullerene derivatives hold great promise as PDT photosensitisers due to their unprecedented efficiency of formation – for C60 irradiated at 532 nm, this is unity.Citation106 There are, however, some drawbacks. The first is that C60 absorbs well in the UV range and poorly at the red end of the electromagnetic spectrum; this matters as these are the wavelengths of the light sources typically used in PDT. The second potential drawback concerns the effect of multiple functionalisation of the fullerene cage. Hamano et al.Citation107 showed that increasing the number of addends decreased the efficiency of
production. At the extreme end, a PHF exhibited zero photosensitisation. A final drawback is the solvent dependency of
generation. Yamakoshi et al.Citation108 showed that, while
is easily generated in non-polar solvents,
and
are the preferential products in polar solvents such as water and that these are the species responsible for DNA cleavage in physiological conditions, thus casting shadow over the precise mechanism(s) of PDT. Readers are directed to the review of Mroz et al.Citation109 for a fuller treatment of this topic.
Chemotherapy
PHFs are prevalent in the literature on cancer therapeutics, despite the specifics of their mechanism of action not being fully understood. Kang et al. and Pan et al. provided a detailed mechanistic insight into the action of Gd@C82(OH)22 on pancreatic tumour metastasis,Footnote25 concluding that the amphiphilic nature of the PHF (hydrophobic cage carbons and hydrophilic hydroxyl groups) led to specific binding and enzymatic inhibition. Citation110,Citation111 Liu et al. recognised that, while sharing the same number of hydroxyl groups, Gd@C82(OH)22 outperformed C60(OH)22 in the inhibition of breast cancer metastasis. Citation112 This is a good demonstration of the effect of the incarcerated species in EMFs on their reactivity, structure and subsequent function. Chen et al. found the antineoplastic activity of Gd@C82(OH)22 was considerably greater than that of commonly used cis-platin, thus adding weight to the claim for fullerenes to be used as chemotherapeutic agents.Citation113
Theranostics
A distinct selling point of the fullerenes is their ability to combine multiple functionalities, thus making them viable theranosticFootnote26 platforms.Citation114 Theranostics offers an attractive route to personalised nanomedicines where exposure to treatment can be monitored and thus tailored for each patient individually.Citation115,Citation116
Photoacoustic imaging and photothermal therapy
This is a rather novel example of nanoparticles exhibiting useful theranostic qualities, with benefits of deeper tissue penetration and lower cost when compared with more traditional imaging modalities, e.g. MRI.Citation117 Fullerenols (C60(OH)xNaz) and carboxy-fullerene tris-adducts (C60(C(COOH)2)3) were exposed to low-intensity near-IR laser irradiation (10 mJ cm−2) in both in vitro and in vivoFootnote27 studies. A scanning ultrasound detection system detected a popping sound arising from the bursting of mesothelial lung carcinoma (A549) cells due to induced local heating of at least
. Thus, not only were cancer cells destroyed, but the process could also be tracked by a cheap, non-invasive imaging technique using a laser power well within safety limits.
Brachytherapy, chemotherapy and dual-modal imaging
Shultz et al. synthesised a novel theranostic platform for MRI and brachytherapyFootnote28 of brain tumours wherein Gd3N@C80 (water solubilised by both carboxyl and hydroxyl groups) was covalently linked to the DOTA chelating agent labelled with radioactive β-emitter,177Lu.Footnote29,Citation118 Retention time in the tumour was good, allowing for good T1-weighted imaging and effective delivery of brachytherapy. In a similar vain,177LuxN@C80, with the radiolabel within the fullerene cage, was developed by the same group.Citation119 Fears of β decay destroying the fullerene cage were alleviated as there appeared to be little evidence of it after one half-life (6.7 days).
In the field of multi-modal imaging, Iezzi et al.Citation120 synthesised mixed-metal TNT EMFs, N@C80 (M = Gd or Ho), where the lutetium atom provided X-ray imaging contrast on top of the MRI contrast provided by M. This is a promising result as these mixed-metal TNT EMFs were actually synthesised in higher yield than the mono TNT EMFs, e.g. Lu3N@C80. Another multi-modal imaging platform has been developed by Luo et al. for combined positron emission tomography (PET) and MRI.Citation121 Exohedral addition of 124I to hydroxylated Gd3N@C80 conferred the PET ability although, as with the X-ray CA of Wharton et al.,Citation85 iodine must be stably attached to the cage to avoid allergic reactions. A lack of iodine uptake in the thyroid gland seemed to suggest good stability of this molecule, giving it promise as a future dual-modal imaging tool.
Toxicity
The toxicity of fullerenes and their derivatives is a hotly debated topic amongst both researchers and regulatory bodies.Citation122,Citation123 Particularly when dealing with synthetic routes to potential pharmaceutical products, low levels of genotoxic impurities will be introduced but must be minimised. Citation124 As has been shown in the preceding sections, aggregation can have a great effect on the efficacy of administered nanoparticlesFootnote30 and its effects in physiological systems must be understood.Citation125
Kyzyma et al.Citation126 showed that aqueous solutionsFootnote31 of C60 had no toxic effects on Chinese-hamster V79 cells. However, cytotoxicity of C60 in aqueous solution, mediated by singlet oxygen production, was seen in another study.Citation127 Most importantly, this study found that cytotoxicity is a sensitive function of the number of functional addends attached to the cage, with increased exohedral functionalisation decreasing cytotoxic effects by over seven orders of magnitude. This is in keeping with the results of Hamano et al.Citation107 discussed earlier.
Gao et al.Citation128 showed that C60(OH)24-26 presented no acute or chronic toxic effects in model organisms from four different kingdoms;Footnote32 on the contrary, there was evidence of increased growth and extended lifespan, which could have profound effects in environmental research areas such as biofuel production. With regard to cancer therapy, the antioxidant ability of C60(OH)24 has been shown to modulate cytotoxic effects of chemotherapeutic agent, doxorubicin (DOX), which induces ROS-mediated oxidative stress. Citation129,Citation130
Upon intravenous administration of future fullerene derivatives, the pharmaceutical must efficiently perform its function at the desired site, rather than just circulate throughout the body without specificity. Thus, a flagship targeted fullerene system was synthesised by Fan et al. who used a folic acid ligand to target tumour cells.Citation131 Chemotherapy was delivered on-site by DOX as well as PDT thanks to the fullerene moiety. In an elegant twist, the intracellular release of DOX could be tracked as its fluorescence was quenched by C60's excellent affinity for electrons.
Concluding remarks
This review has taken a holistic approach to the deployment of fullerene derivatives in biomedical contexts. The unique electronic structure of a fullerene cage is shown to be the basis of its salient properties. A number of exohedral synthesis protocols have been critically examined, identifying the need for reproducible and scalable methods which yield well-defined addition patterns, in line with requirements of the pharmaceutical industry. Endohedral fullerenes remain a relatively exotic commodity; despite their numerous varieties and subsequent biomedical applications, even gram-scale quantities are hard to produce. Effective methods of water solubilisation have been critically evaluated, taking into account the need for well-defined addition patterns. The highest water-solubility to date has been achieved by a mono-functionalised dendrofullerene via the popular Bingel reaction, and this same reaction protocol proves robust for synthesising multi-adduct derivatives with well-defined structure. With this knowledge of product synthesis, applications of fullerene derivatives in diagnosis, therapy and theranostics were discussed. The literature on fullerenes as MRI CAs and antioxidants is vast and these appear to be very likely to make it to market soonest, given the marked improvements on technologies currently used in-clinic. Novel multifunctional theranostic platforms, however, demonstrate the fuller beauty and power of nanotechnology when applied to medicine. In spite of cytotoxicity arising from the efficient generation of singlet oxygen, fullerenes seem to exhibit little toxicity in their functionalised form.
As with all vying nanomaterials, if fullerenes are to reach the pharmaceutical market, and if fullerene-based nanomedicineCitation132 is to find ubiquitous, daily application, then an understanding of the fundamental physical and chemical behaviour of these fascinating molecules necessarily precedes their technological exploitation.Footnote33 Unlike other nanomaterials – such as CNTs,Citation137 graphene,Citation138 quantum dots (QDs) Citation139 and superparamagnetic iron oxide nanoparticles (SPIONs)Citation140 – there is not an inherent heterogeneity of the base product. Each fullerene has a precisely defined structure in three-dimensional space. The importance of this cannot be overstated as, in drug discovery, it is the molecular structure which pharmaceutical companies patent. Thus, there is a significant distinction between fullerenes and graphene (whose sheets are of different size and termination), CNTs (whose length and chirality vary) and QDs and SPIONs (whose morphologies and topographies vary).
The benefits conferred through using fullerenes as medical tools could be immense, and it is the author's belief that they should and will soon be on the market. This will, however, require further interdisciplinary collaborations and a willing dialogue between academia and the pharmaceutical industry.
Acknowledgments
The author wishes to thank Prof. Kyriakos Porfyrakis for his support as DPhil supervisor, as well as the EPSRC for funding [EP/K030108/1].
Disclosure statement
No potential conflict of interest was reported by the author.
ORCiD
I. Rašović http://orcid.org/0000-0001-9466-6281
Notes
1 Differentiated from benzene due to the difference in bond lengths: the [6,6] bonds (double) between adjacent hexagons are 1.38 Å, while the [6,5] bonds (single) which form the borders between hexagons and pentagons are 1.45 Å.Citation2
2 This strain accounts for approximately 80% of the heat of formation of C60.Citation3
3 It is worth mentioning at this point that the primary technique used to separate fullerenes from one another is high performance liquid chromatography (HPLC). Careful selection of the stationary phase and solvent system can lead to good separation of not only different sized cages, but also isomers containing the same number of atoms.Citation6
4 C60 can now be easily bought in large quantities at a price comparable to any standard laboratory chemical. MER Corporation of Arizona, USA sells 99+% pure batches of at $20 per gram. As a good example to see how the price and rarity increases with cage size, the same company sells C70 of the same purity for $325 per gram, up to 25 g (Prices correct as of June 2016).
5 Readers are directed to the excellent monograph by Hirsch and BrettreichCitation16 for a more complete overview of the library of fullerene reactions.
6 The ‘small polar functional group’ for amination here refers to the addition of methylamine.
7 Actually 1:3 t-butylamine:DMF.
8 Based on the ratios H:C and H:N.
9 The fact that there is no consensus on the name of this type of derivative should give an idea as to the lack of specificity associated with its structure and thus definition. This could conceivably cause regulatory issues and is a representative example of one of the stumbling blocks to fullerenes reaching the pharmaceutical market.
10 Compared to the simplicity of the prototypical diethyl malonate used in Bingel reactions.
11 Readers are directed to the review of Popov et al.Citation45 for a broader and more comprehensive outlook on the synthesis, properties and applications of endohedral fullerenes.
12 EMF yields are found to be dependent on many different parameters in the arc discharge reactor, with a strong dependence on the pressure of the He atmosphere.Citation48 Reactor size thus obviously has a major effect, as do rod size, distance between rods and current.
13 Researchers have attempted to make sense of this complex field in both theoryCitation54 and experiment.
14 The favoured method of administering medicines as it is fastest and gives 100% bioavailability of the administered species.
15 This is particularly true when long-chain hydrophilic polymers are involved and control over the precise length of polymer chains is impossible. While more ethylene glycol units, for example, will lead to an increased solubility in polyethylene glycol (PEG)-based systems, the pharmaco-kinetic behaviour grows in complexity with increasing chain length due to increasing size and configurational entropy of the polymer chain. With a mixture of chain lengths in the sample, predicting behaviour within the body becomes even more difficult.
16 A full list would warrant further discussion beyond the scope of this review, so readers are directed to the extensive list offered by Beuerle et al.Citation61
17 Each letter in this sequence corresponds to a different amino acid building block: G = glycine; A = alanine; B = asparagine or aspartic acid (ambiguous); P = proline; L = leucine; V = valine; R = arginine.
18 Thus increasing the relaxivities, respectively and
.
19 Diameter increase from 30–90 to 600–1000 nm.
20 An even higher of 439.7 mM−1 s−1 was seen at 4.7 T.
21 DOTA = 1,4,7,10-tetraazacyclododecane-1,4,7,10-tetraacetic acid)
22 Pun not intended.
23 There are many ROS but two of the most studied are the superoxide () and hydroxyl (
) radicals. Reduction of molecular oxygen yields the superoxide radical (
) which can dismutate to hydrogen peroxide (
), which can then be fully reduced to water or partially reduced to the hydroxyl radical (
).Citation92
24 This increase can be a result of endogenous generation (i.e. standard cellular activity) or exogenous sources (e.g. through smoking tobacco).
25 Through a combination of in vivo, in vitro and in silico observations.
26 Capable of both therapeutic and diagnostic functions.
27 However, given the lack of a targeting moiety on the fullerene cage, the in vivo study saw the fullerenols encapsulated within functionalised silica or chitosan nanoparticles.
28 Delivery of a radioactive source within the body so as to allow localised tumour targeting with minimal-radiation side effects cf. traditional radiotherapy using external X-ray sources.
29 177Lu also emits γ radiation which could be detected by, for example, single-photon emission computed tomography (SPECT) for imaging purposes.
30 Although the size and length of an individual macromolecular fullerene might be well characterised, the average size and charge of many of them agglomerated together will vary from solvent to solvent, and could well lead to different toxic behaviour.
31 C60 was first dissolved in N-methylpyrrolidine which also showed no cytotoxicity.
32 These were: algae (Pseudokirchneriella subcapitata); a plant (Arabidopsis thaliana); a fungus (Aspergillus niger); and an invertebrate (Ceriodaphnia dubia).
33 Science aside, the opinions of the public and key players in both policy and business (who may not have a specialist background) cannot be ignored.Citation133 Surveys have shown that the UK and US public view medical applications of nanotechnology in a less favourable light than energy applications. Citation134 In one study, industry perceives fewer risks associated with nanotechnology than the public, and so does not enforce the regulations the public expects of such an industry.Citation135 This is likely to change in the near future as stricter definitions of a nanomaterial have been implemented by the European Union and include fullerenes within their scope.Citation122,Citation136
References
- R. Taylor and D. R. M. Walton: Nature, 1993, 363, (6431), 685–693.
- K. M. Kadish and R. S. Ruoff: ‘Fullerenes: chemistry, physics, and technology’, 2000, New York, Wiley.
- R. C. Haddon, R. E. Palmer, H. W. Kroto and P. A. Sermon: ‘The fullerenes: powerful carbon-based electron acceptors’, Philos. Trans. R. Soc. A: Math. Phys. Eng. Sci., 1993, 343, (1667), 53–62.
- P. W. Fowler and D. E. Manolopoulos: ‘An Atlas of fullerenes’, ‘Dover books on chemistry’, 2006, New York, Dover Publications.
- Q. Xie, E. Perez-Cordero and L. Echegoyen: ‘Electrochemical detection of C606- and C706-: enhanced stability of fullerides in solution’, J. Am. Chem. Soc., 1992, 114, (10), 3978–3980.
- M. P. Gasper and D. W. Armstrong: ‘A comparative study of buckminsterfullerene and higher fullerene separations by HPLC’, J. Liq. Chromatogr., 1995, 18, (6), 1047–1076.
- F. Diederich, R. Ettl, Y. Rubin, R. L. Whetten, R. Beck, M. Alvarez, S. Anz, D. Sensharma, F. Wudl, K. C. Khemani and A. Koch: ‘The Higher Fullerenes: Isolation and Characterization of C76, C84, C90, C94, and C70O, an Oxide of D5h-C70’, Science, 1991, 252, (5005), 548–551.
- F. Cataldo and T. Da Ros: ‘Medicinal chemistry and pharmacological potential of fullerenes and carbon nanotubes, ‘Carbon materials: chemistry and physics’, 2008, Berlin, Springer Science+Business Media.
- K. N. Semenov, N. A. Charykov, V. A. Keskinov, A. K. Piartman, A. A. Blokhin and A. A. Kopyrin: ‘Solubility of light fullerenes in organic solvents’, J. Chem. Eng. Data, 2010, 55, (1), 13–36.
- X. Zhou, J. Liu, Z. Jin, Z. Gu, Y. Wu and Y. Sun: ‘Solubility of fullerene C60 and C70 in toluene, o-xylene and carbon disulfide at various temperatures’, Fullerene Sci. Technol., 1997, 5, (1), 285–290.
- C. Bingel and H. Schiffer: ‘Biscyclopropanation of C70’, Liebigs Ann., 1995, 1551–1553.
- N. S. Goroff: ‘Mechanism of fullerene formation’, Acc. Chem. Res., 1996, 48824, (8), 77–83.
- H. Murayama, S. Tomonoh, J. M. Alford and M. E. Karpuk: ‘Fullerene production in tons and more: from science to industry’, Fuller. Nanotub. Car. N., 2005, 12, (February 2015), 1–9.
- W. Kratschmer, L. D. Lamb, K. Fostiropoulos and D. R. Huffman: ‘Solid C60: a new form of carbon’, Nature, 1990, 347, 354–358.
- H. W. Kroto, J. R. Heath, S. C. O'Brien, R. F. Curl and R. E. Smalley: ‘C60: buckminsterfullerene’, Nature, 1985, 318, (6042), 162–163.
- A. Hirsch, M. Brettreich and F. Wudl: ‘Fullerenes: chemistry and reactions’, 2006, Weinheim, Wiley.
- M. Maggini, G. Scorrano and M. Prato: ‘Addition of azomethine ylides to C60: synthesis, characterization, and functionalization of fullerene pyrrolidines’, J. Am. Chem. Soc., 1993, 115, (21), 9798–9799.
- C. Bingel: ‘Cyclopropanierung von fullerenen’, Chemische Berichte, 1993, 126, (8), 1957–1959.
- V. Georgakilas, K. Kordatos, M. Prato, D. M. Guldi, M. Holzinger and A. Hirsch: ‘Organic functionalization of carbon nanotubes’, J. Am. Chem. Soc., 2002, 124, (5), 760–761.
- K. S. Coleman, S. R. Bailey, S. Fogden and M. L. H. Green: ‘Functionalization of single-walled carbon nanotubes via the bingel reaction’, J. Am. Chem. Soc., 2003, 125, (29), 8722–8723.
- F. Wudl, A. Hirsch, K. C. Khemani, T. Suzuki, P-M. Allemand, A. Koch, H. Eckert, G. Srdanov and H. M. Webb: ‘Survey of chemical reactivity of C60, electrophile, and dienopolarophile par excellence’ in ‘Synthesis, properties, and chemsitry of large carbon clusters’, (ed. G. S. Hammond and V. J. Kuck), chap. 11, ACS Symposium Series 481, 161–176; 1992, Washington, DC, American Chemical Society.
- R. Seshadri, A. Govindaraj, R. Nagarajan, T. Pradeep and C. N. R. Rao: ‘Addition of amines and halogens to fullerenes C60 and C70’, Tetrahedron Lett., 1992, 33, (15), 2069–2070.
- V. Brezova, A. Stasko, P. Rapta, G. Domschke, A. Bartl and L. Dunsch: ‘Fullerene anion formation by electron transfer from amino donor to photoexcited C60. Electron paramagnetic resonance study’, J. Phys. Chem., 1995, 99, (44), 16234–16241.
- O. Amelines-Sarria and V. A. Basiuk: ‘A DFT study of methylamine polyaddition to C80 fullerene’, Superlattice Microst., 2009, 46, (1–2), 302–305.
- L. Y. Chiang, J. W. Swirczewski, C. S. Hsu, S. K. Chowdhury, S. Cameron and K. Creegan: ‘Multi-hydroxy additions onto C60 fullerene molecules’, J. Chem. Soc. Chem. Commun., 1992, 1791–1793.
- J. Li, A. Takeuchi, M. Ozawa, X. Li, K. Saigo and K. Kitazawa: ‘C60 fullerol formation catalysed by quaternary ammonium hydroxides’, J. Chem. Soc. Chem. Commun., 1993, 1784–1785.
- N. S. Schneider, A. D. Darwish, H. W. Kroto, R. Taylor and D. R. M. Walton: ‘Formation of fullerols via hydroboration of fullerene-C60’, J. Chem. Soc. Chem. Commun., 1994, 463–464.
- A. Arrais and E. Diana: ‘Highly water soluble C60 derivatives: a new synthesis’, Fullerene Nanotub. Carb. N., 2003, 11, (1), 35–46.
- F. Scarel: ‘Fullerene-stoppered rotaxane : a multifunctional supramolecular material’, PhD thesis, Freiburg Institute of Advanced Studies, 2012.
- C. M. Cardona, B. Elliott and L. Echegoyen: ‘Unexpected chemical and electrochemical properties of M3N@C80 (M = Sc, Y, Er)’, J. Am. Chem. Soc., 2006, 128, (19), 6480–6485.
- D. Milic and M. Prato: ‘Fullerene unsymmetrical bis-adducts as models for novel peptidomimetics’, Eur. J. Org. Chem., 2010, 476–483.
- N. Chhabra, M. L. Aseri and D. Padmanabhan: ‘A review of drug isomerism and its significance’, Int. J. Appl. Basic Med. Res., 2013, 3, (1), 16–18.
- Q. Lu, D. I. Schuster and S. R. Wilson: ‘Preparation and characterization of six bis(N-methylpyrrolidine)-C60 isomers: magnetic deshielding in isomeric bisadducts of C60’, J. Organ. Chem., 1996, 61 (24), 4764–4768.
- Z. Zhou and S. Wilson: ‘Tether-directed multiple functionalization of fullerene[60]’, Curr. Organ. Chem., 2005, 9, (8), 789–811.
- J.-F. Nierengarten and J.-F. Nicoud: ‘cyciopropanation of C60 with malonic acid mono-esters’, Tetrahedron Lett., 1997, 38, (44), 7737–7740.
- X. Camps and A. Hirsch: ‘Efficient cyclopropanation of C60 starting from malonates’, J. Chem. Soc. Perkin Trans. 1, 1997 (11), 1595–1596.
- A. Hirsch, I. Lamparth and H. R. Karfunkel: ‘Fullerene chemistry in three dimensions: isolation of seven regioisomeric bisadducts and chiral trisadducts of C60 and Di(ethoxycarbonyl)methylene’, Angew. Chem. Int. Ed. Engl., 1994, 33, (4), 437–438.
- D. Sigwalt, F. Schillinger, S. Guerra, M. Holler, M. Berville and J-F. Nierengarten: ‘An expeditious regioselective synthesis of [60]fullerene e,e,e tris-adduct building blocks’, Tetrahedron Lett., 2013, 54, (32), 4241–4244.
- Á. Duarte-Ruiz, L. Echegoyen, A. Aya and F. Gomez-Baquero: J. Mex. Chem. Soc., 2009, 53, (3), 169–173.
- H. Li, S. A. Haque, A. Kitaygorodskiy, M. J. Meziani, M. Torres-Castillo and Y. P. Sun: ‘Alternatively modified Bingel reaction for efficient syntheses of C60 hexakis-adducts’, Org. Lett., 2006, 8, (24), 5641–5643.
- I. Lamparth and A. Hirsch: ‘Water-soluble malonic acid derivatives of C60 with a defined three-dimensional structure’, J. Chem. Soc. Chem. Commun., 1994, 1727–1728.
- B. Jin, J. Shen, R. Peng, R Zheng and S. Chu: ‘Efficient cyclopropanation of [60]fullerene starting from bromo-substituted active methylene compounds without using a basic catalyst’, Tetrahedron Lett., 2014, 55, (36), 5007–5010.
- Y. Chai, T. Cuo, C. Jin, R. E. Haufler, L. P. F. Chibante, J. Fure, L. Wang, J. M. Alford and R. E. Smalley: ‘Fullerenes wlth metals inside’, J. Phys. Chem., 1991, 95, (9), 7564–7568.
- A. Krachmalnicoff, M. H. Levitt and R. J. Whitby: ‘An optimised scalable synthesis of H2O@C60 and a new synthesis of H2@C60’, Chem. Commun., 2014, 50, (86), 13037–13040.
- A. Popov, S. Yang and L. Dunsch: Endohedral Fullerenes, Chem. Rev., 2013, 113, (8), 5989–6113.
- P. Dallas, I. Rasovic, G. Rogers et al.: in ‘Carbon nanomaterials sourcebook: graphene, fullerenes, nanotubes and nanodiamonds,’ (ed. K. Sattler), chap. 10, 255–270; 2016, Boca Raton, Taylor & Francis.
- J. R. Heath, S. C. O'Brien, Q. Zhang, Y. Liu, R. F. Curl, F. K. Tittel and R. E. Smalley: ‘Lanthanum complexes of spheroidal carbon shells’, J. Am. Chem. Soc., 1985, 107, (25), 7779–7780.
- Y. Saito, S. Yokoyama, M. Inakuma and H. Shinohara: ‘An ESR study of the formation of La@C82 isomers in arc synthesis’, Chem. Phys. Lett., 1996, 250, (1), 80–84.
- H. Shinohara: ‘Endohedral metallofullerenes’, Rep. Prog. Phys., 2000, 63, (6), 843–892.
- V. S. Kozlov, M. V. Suyasova and V. T. Lebedev: ‘Synthesis, extraction, and chromatographic purification of higher empty fullerenes and endohedral gadolinium metallofullerenes’, Russ. J. Appl. Chem., 2014, 87, (2), 121–127.
- S. Stevenson, G. Rice, T. Glass, K. Harich, F. Cromer, M. R. Jordan, J. Craft and H. C. Dorn: ‘Metallofullerenes in high yield and purity’, Nature, 1999, 80, 80–82.
- L. Dunsch, M. Krause, J. Noack and P. Georgi: ‘Endohedral nitride cluster fullerenes: formation and spectroscopic analysis’, J. Phys. Chem. Solids, 2004, 65, 309–315.
- V. Bezmelnitsyn, S. Davis and Z. Zhou: ‘Efficient synthesis of endohedral metallofullerenes in 3-phase arc discharge’, Fullerene Nanotub. Carb. N., 2014, 23, 612–617.
- Q. Deng and A. A. Popov: ‘Prato and bingel-hirsch cycloaddition to heptagon-containing LaSc2N@Cs(hept)-C80: importance of pentalene units’, Chem. Commun., 2015, 51, (26), 5637–5640.
- V. K. Koltover: ‘Spin-leakage of the fullerene shell of endometallofullerenes: EPR, ENDOR and NMR evidences’, Carbon, 2004, 42, 1179–1183.
- B. Cao, T. Wakahara, Y. Maeda, A. Han, T. Akasaka, T. Kato, K. Kobayashi and S. Nagase: ‘Lanthanum endohedral metallofulleropyrrolidines: synthesis, isolation, and EPR characterization’, Chem. Eur. J., 2004, 10, (3), 716–720.
- R. Bolskar, A. Benedetto, L. Husebo, R. Price, E. Jackson, S. Wallace, L. Wilson and M. Alford: ‘First soluble M@C60 derivatives provide enhanced access to metallofullerenes and permit in vivo evaluation of Gd@C60 [C(COOH)2]10 as a MRI contrast agent’, J. Am. Chem. Soc., 2003, 125, (18), 5471–5478.
- S. Kunsági-Máté, K. Szabó, I. Bitter, G. Nagy and L. Kollar: ‘Complex formation between water-soluble sulfonated calixarenes and C60 fullerene’, Tetrahedron Lett., 2004, 45, (7), 1387–1390.
- F. Giacalone, N. Martín and F. Wudl: in ‘Fullerene polymers,’ chap. 1, 1–14; 2009, Weinheim, Wiley-VCH.
- Y. Iwamoto and Y. Yamakoshi: ‘A highly water-soluble C60-NVP copolymer: a potential material for photodynamic therapy’, Chem. Commun., 2006, 4805–4807.
- F. Beuerle, R. Lebovitz and A. Hirsch: in ‘Medicinal chemistry and pharmacological potential of fullerenes and carbon nanotubes’, (ed. F. Cataldo & T. Da Ros), Vol. 1, chap. 3, 51–78; 2008, Berlin, Springer Science+Business Media.
- M. Brettreich and A. Hirsch: ‘A highly water-soluble dendro[60]-fullerene’, Tetrahedron Lett., 1998, 39, (18), 2731–2734.
- T. Da Ros, M. Prato, F. Novello, M. Maggini and E. Banfi: ‘Easy access to water-soluble fullerene derivatives via 1,3-dipolar cycloadditions of azomethine ylides to C60’, J. Organ. Chem., 1996, 61, (25), 9070–9072.
- S. Aroua, W. B. Schweizer and Y. Yamakoshi: ‘C60 pyrrolidine bis-carboxylic acid derivative as a versatile precursor for biocompatible fullerenes’, Organ. Lett., 2014, 16, 1688–1691.
- C. Chen, G. Xing, J. Wang, Y. Zhao, B. Li, J. Tang, G. Jia, T. Wang, J. Sun, L. Xing, H. Yuan, Z. Chen, F. Zhao, Z. Chai and X. Fang: ‘Multihydroxylated [Gd@C82(OH)22]n nanoparticles: antineoplastic activity of high efficiency and low toxicity’, Nano Lett., 2005, 82(10), 2050–2057.
- S. Kunjachan, J. Ehling, G. Storm, F. Kiessling and T. Lammers: ‘Noninvasive imaging of nanomedicines and nanotheranostics: principles, progress, and prospects’, Chem. Rev., 2015, 115, (19), 10907–10937.
- G. Hagberg and K. Scheffler: ‘Effect of r1 and r2 relaxivity of gadolinium-based contrast agents on the T1-weighted MR signal at increasing magnetic field strengths’, Contrast Media Mol. Imaging, 2013, 8, (6), 456–465.
- A. J. Amoroso and S. J. A. Pope: ‘Using lanthanide ions in molecular bioimaging’, Chem. Soc. Rev., 2015, 44, 4723–4742.
- P. Caravan, J. Ellison, T. McMurry and R. Lauffer: ‘Gadolinium-(III) chelates as MRI contrast agents: structure, dynamics, and applications’, Chem. Rev., 1999, 99, (9), 2293–2352.
- P. Caravan: ‘Strategies for increasing the sensitivity of gadolinium based MRI contrast agents’, Chem. Soc. Rev., 2006, 35, (6), 512–523.
- P. H. Kuo, E. Kanal, A. K. Abu-Alfa and S. E. Cowper: ‘Gadolinium-based MR contrast agents and nephrogenic systemic fibrosis’, Radiology, 2007, 242, 647–649.
- J. G. Penfield and R. F. Reilly: ‘What nephrologists need to know about gadolinium’, Nat. Clin. Pract. Nephrol., 2007, 3, (12), 654–668.
- K. Hartman and L. Wilson: ‘Carbon nanostructures as a new high-performance platform for MR molecular imaging’ in Bio-applications of nanoparticles’, (ed. W. Chan), Vol. 620, chap. 6, 74–84; 2007, New York, Springer-Verlag.
- B. Sitharaman, R. D. Bolskar, I. Rusakova and L. J. Wilson: ‘Gd@C60[C(COOH)2]10 and Gd@C60(OH)x : nanoscale aggregation studies of two metallofullerene MRI contrast agents in aqueous solution’, Nano Lett., 2004, 4, (12), 2373–2378.
- J. Zhang, C. Shu, J. Reid, L. S. Owens, T. Cai, W. Gibson, G. L. Long, F. D Corwin, Z.-J. Chen, P. P. Fatouros and H. C. Dorn: ‘High relaxivity trimetallic nitride (Gd3N) metallofullerene MRI contrast agents with optimized functionality’, Bioconjug Chem., 2010, 21, (4), 610–615.
- D. Yang, Y. Zhao, H. Guo, Y. Li, P. Tewary and G. Xing: ‘Nanoparticles, [Gd@C82(OH)22]n, induces dendritic cell maturation and activates Th1 immune responses’, ACS Nano, 2011, 4, (2), 1178–1186.
- S. Laus, B. Sitharaman, É. Tóth, R. D. Bolskar, L. Helm, S. Asokan, M. S. Wong, L. J. Wilson and A. E. Merbach: ‘Destroying gadofullerene aggregates by salt addition in aqueous solution of Gd@C60(OH)x and Gd@C60 [C(COOH2)]10’, J. Chem. Soc., 2005, 127, (26), 10–13.
- M. Mikawa, H. Kato, M. Okumura, M. Narazaki, Y. Kanazawa, N. Miwa and H. Shinohara: ‘Paramagnetic water-soluble metallofullerenes having the highest relaxivity for MRI contrast agents’, Bioconjugate Chem., 2001, 12, (4), 510–514.
- C. Shu, F. D. Corwin, J. Zhang, Z. Chen, J. E. Reid, M. Sun, W. Xu, J. H. Sim, C. Wang, P. P. Fatouros, R. Alan, H. W. Gibson and H. C. Dorn: ‘Facile preparation of a new gadofullerene-based magnetic resonance imaging contrast agent with high1H relaxivity’, Bioconjug Chem., 2009, 20, (6), 1186–1193.
- P. P. Fatouros, F. D. Corwin, Z.-J. Chen, W. C. Broaddus, J. L. Tatum, B. Kettenmann, Z. Ge, H. W. Gibson, J. L. Russ, A. P. Leonard, J. C. Duchamp and H. C. Dorn: ‘In vitro and in vivo imaging studies of a new endohedral metallofullerene nanoparticle’, Radiology, 2006, 240, (3), 756–764.
- K. B. Ghiassi, M. M. Olmstead and A. L. Balch: ‘Gadolinium-containing endohedral fullerenes: structures and function as magnetic resonance imaging (MRI) agents’, Dalton Trans., 2014, 43, 7346–7358.
- E.-Y. Zhang, C.-Y. Shu, L. Feng and C.-R. Wang: ‘Preparation and characterization of two new water-soluble endohedral metallofullerenes as magnetic resonance imaging contrast agents’, J. Phys. Chem. B, 2007, 111, 14223–14226.
- R. Cui, J. Li, H. Huang, M. Zhang, X. Guo, Y. Chang, M. Li, J. Dong, B. Sun and G. Xing: ‘Novel carbon nanohybrids as highly efficient magnetic resonance imaging contrast agents’, Nano Res., 2015, 8, (4), 1259–1268.
- L. Wang, X. Zhu, X. Tang, C. Wu, Z. Zhou, C. Sun, S.-L. Deng, H. Ai and J. Gao: ‘A multiple gadolinium complex decorated fullerene as a highly sensitive T1 contrast agent’, Chem. Commun., 2015, 51, (21), 4390–4393.
- T. Wharton and L. J. Wilson: ‘Highly-iodinated fullerene as a contrast agent for X-ray imaging’, Bioorg. Med. Chem., 2002, 10, (11), 3545–3554.
- A. Miyamoto, H. Okimoto, H. Shinohara and Y. Shibamoto: ‘Development of water-soluble metallofullerenes as x-ray contrast media’, Eur. Radiol., 2006, 16, (5), 1050–1053.
- S. H. Friedman, D. L. Decamp, R. P. Sijbesma, G. Srdanov, F. Wudl and G. L. Kenyon: ‘Inhibition of the HIV-1 protease by fullerene derivatives: model building studies and experimental verification’, J. Am. Chem. Soc., 1993, 115, (5), 6506–6509.
- H. Tzoupis, G. Leonis, S. Durdagi, V. Mouchlis, T. Mavromoustakos and M. G. Papadopoulos: ‘Binding of novel fullerene inhibitors to HIV-1 protease: insight through molecular dynamics and molecular mechanics Poisson-Boltzmann surface area calculations’, J. Computer Aided Mol. Des., 2011, 25, (10), 959–976.
- T. A. Strom, S. Durdagi, S. S. Ersoz, R. E. Salmas, C. T. Supuran and A. R. Barron: ‘Fullerene-based inhibitors of HIV-1 protease’, J. Peptide Sci., 2015, 21, (12), 862–870.
- S. Tanimoto, S. Sakai, S. Matsumura, D. Takahashi and K. Toshima: ‘Target-selective photo-degradation of HIV-1 protease by a fullerene-sugar hybrid’, Chem. Commun., 2008 (44), 5767.
- A. A. Alfadda and R. M. Sallam: ‘Reactive oxygen species in health and disease’, J. Biomed. Biotechnol., 2012, 2012, 1–14.
- J. F. Turrens: ‘Mitochondrial formation of reactive oxygen species’, J. Physiol., 2003, 552, 335–344.
- P. D. Ray, B. W. Huang and Y. Tsuji: ‘Reactive oxygen species (ROS) homeostasis and redox regulation in cellular signaling’, Cell. Signal., 2012, 24, (5), 981–990.
- K. Ishikawa, K. Takenaga, M. Akimoto, N. Koshikawa, A. Yamaguchi, H. Imanishi, K. Nakada, Y. Honma and J.-I. Hayashi: ‘ROS-generating mitochondrial DNA mutations can regulate tumor cell metastasis’, Science, 2008, 320, (5876), 661–664.
- V. Shukla, S. K. Mishra and H. C. Pant: ‘Oxidative Stress in Neurodegeneration’, Adv. Pharmacol. Sci., 20111–13.
- B. N. Ames, M. K. Shigenaga and T. M. Hagen: ‘Oxidants, antioxidants, and the degenerative diseases of aging’, Proc. Natl. Acad. Sci. U.S.A., 1993, 90, (17), 7915–7922.
- L. L. Dugan, D. M. Turetsky, C. Du, M. Wheeler, C. R. Almli, C. K. Shen, T. Y. Luh, D. W. Choi and T. S. Lin: ‘Carboxyfullerenes as neuroprotective agents’, Proc. Natl. Acad. Sci. U.S.A., 1997, 94, (17), 9434–9439.
- L. L. Dugan, E. G. Lovett, K. L. Quick, J. Lotharius, T. T. Lin and K. L. O'Malley: ‘Fullerene-based antioxidants and neurodegenerative disorders’, Parkinsonism Relat. Disord., 2001, 7, (3), 243–246.
- L. L. Dugan, L. Tian, K. L. Quick, J. I. Hardt, M. Karimi, C. Brown, S. Loftin, H. Flores, S. M. Moerlein, J. Polich, S. D. Tabbal, J. W. Mink and J. S. Perlmutter: ‘Carboxyfullerene neuroprotection postinjury in Parkinsonian nonhuman primates’, Ann. Neurol., 2014, 76, (3), 393–402.
- P. Witte, F. Beuerle, U. Hartnagel, R. Lebovitz, A. Savouchkina, S. Sali, D. Guldi, N. Chronakis and A. Hirsch: ‘Water solubility, antioxidant activity and cytochrome C binding of four families of exohedral adducts of C60 and C70’, Org. Biomol. Chem., 2007, 5, (22), 3599–3613.
- J. J. Yin, F. Lao, P. P. Fu, W. G. Wamer, Y. Zhao, P. C. Wang, Y. Qiu, B. Sun, G. Xing, J. Dong, X. J. Liang and C. Chen: ‘The scavenging of reactive oxygen species and the potential for cell protection by functionalized fullerene materials’, Biomaterials, 2009, 30, (4), 611–621.
- Q. Liu, L. Jin, B. H. Mahon, M. D. Chordia, F. H. Shen and X. Li: ‘Novel treatment of neuroinflammation against low back pain by soluble fullerol nanoparticles’, Spine, 2013, 38, (17), 1443–1451.
- F. Stewart, P. Baas and W. Star: ‘What does photodynamic therapy have to offer radiation oncologists (or their cancer patients)?’, Radiother. Oncol., 1998, 48, (3), 233–248.
- F. Wilkinson, W. P. Helman and A. B. Ross: ‘Quantum yields for the photosensitized formation of the lowest electronically excited singlet state of molecular oxygen in solution’, J. Phys. Chem. Ref. Data, 1993, 22, (1993), 113.
- S. Wang, R. Gao, F. Zhou and M. Selke: ‘Nanomaterials and singlet oxygen photosensitizers: potential applications in photodynamic therapy’, J. Mater. Chem., 2004, 14, (4), 487.
- J. W. Arbogast, A. P. Darmanyan, C. S. Foote, Y. Rubin, F. N. Diederich, M. M. Alvarez, S. J. Anz and R. L. Whetten: ‘Photophysical properties of C60’, J. Phys. Chem., 1991 (95), 11–12.
- T. Hamano, K. Okuda, T. Mashino, M. Hirobe, K. Arakane, A. Ryu, S. Mashiko and T. Nagano: ‘Singlet oxygen production from fullerene derivatives: effect of sequential functionalization of the fullerene core’, Chem. Commun., 1997, 21–22.
- Y. Yamakoshi, N. Umezawa, A. Ryu, K. Arakane, N. Miyata, Y. Goda, T. Masumizu and T. Nagano: ‘Active oxygen species generated from photoexcited fullerene (C60) as potential medicines: O2-• versus 1O2’, J. Am. Chem. Soc., 2003, 125, (42), 12803–12809.
- P. Mroz, G. P. Tegos, H. Gali, T. Wharton, T. Sarna and M. R. Hamblin: ‘Fullerenes as photosensitizers in photodynamic therapy’ in ‘Medicinal chemistry and pharmacological potential of fullerenes and carbon nanotubes’, (ed. F. Cataldo and T. Da Ros), Vol. 1, chap. 4, 79–106; 2008, Berlin, Springer Science+Business Media.
- S.-G. Kang, G. Zhou, P. Yang, Y. Liu, B. Sun, T. Huynh, H. Meng, L. Zhao, G. Xing, C. Chen, Y. Zhao and R. Zhou: ‘Molecular mechanism of pancreatic tumor metastasis inhibition by Gd@C82(OH)22 and its implication for de novo design of nanomedicine’, Proc. Natl. Acad. Sci., 2012, 109, (38), 15431–15436.
- Y. Pan, L. Wang, S.-G. Kang, Y. Lu, Z. Yang, T. Huynh, C. Chen, R. Zhou, M. Guo and Y. Zhao: ‘Gd-metallofullerenol nanomaterial suppresses pancreatic cancer metastasis by inhibiting the interaction of histone deacetylase 1 and metastasis-associated protein 1’, ACS Nano, 2015, 9, (7), 6826–6836.
- Y. Liu, C. Chen, P. Qian, X. Lu, B. Sun, X. Zhang, L. Wang, X. Gao, H. Li, Z. Chen, J. Tang, W. Zhang, J. Dong, R. Bai, P. E. Lobie, Q. Wu, S. Liu, H. Zhang, F. Zhao, M. S. Wicha, T. Zhu and Y. Zhao: ‘Gd-metallofullerenol nanomaterial as non-toxic breast cancer stem cell-specific inhibitor’, Nat. Commun., 2015, 6, 5988.
- C. Chen, G. Xing, J. Wang, Y. Zhao, B. Li, J. Tang, G. Jia, T. Wang, J. Sun, L. Xing, H. Yuan, Y. Gao, H. Meng, Z. Chen, F. Zhao, Z. Chai and X. Fang: ‘Multihydroxylated [Gd@C82(OH)22]n nanoparticles: antineoplastic activity of high efficiency and low toxicity’, Nano Lett., 2005, 5, (10), 2050–2057.
- Z. Chen, L. Ma, Y. Liu and C. Chen: ‘Applications of functionalized fullerenes in tumor theranostics’, Theranostics, 2012, 2, (3), 238–250.
- S. S. Kelkar and T. M. Reineke: ‘Theranostics: combining imaging and therapy’, Bioconjug. Chem., 2011, 22, 1879–1903.
- E.-K. Lim, T. Kim, S. Paik, S. Haam, Y.-M. Huh and K. Lee: ‘Nanomaterials for theranostics: recent advances and future challenges’, Chem. Rev., 2015, 115, (1), 327–394.
- V. Krishna, A. Singh, P. Sharma, N. Iwakuma, Q. Wang, Q. Zhang, J. Knapik, H. Jiang, S. R. Grobmyer, B. Koopman and B. Moudgil: ‘Polyhydroxy fullerenes for non-invasive cancer imaging and therapy’, Small, 2010, 6, (20), 2236–2241.
- M. D. Shultz, J. D. Wilson, C. E. Fuller, J. Zhang, H. C. Dorn and P. P. Fatouros: ‘Metallofullerene-based nanoplatform for brain tumor brachytherapy and longitudinal imaging in a murine orthotopic xenograft model’, Radiology, 2011, 261, (1), 136–143.
- M. D. Shultz, J. C. Duchamp, J. D. Wilson, C. Y. Shu, J. Ge, J. Zhang, H. W. Gibson, H. L. Fillmore, J. I. Hirsch, H. C. Dorn and P. P. Fatouros: ‘Encapsulation of a radiolabeled cluster inside a fullerene cage, 177Lux Lu(3-x)N@C80: an interleukin-13-conjugated radiolabeled metallofullerene platform’, J. Am. Chem. Soc., 2010, 132, (14), 4980–4981.
- E. B. Iezzi, J. C. Duchamp, K. R. Fletcher, T. E. Glass and H. C. Dorn: ‘Lutetium-based trimetallic nitride endohedral metallofullerenes: new contrast agents’, Nano Lett., 2002, 2, (11), 1187–1190.
- J. Luo, J. D. Wilson, J. Zhang, J. I. Hirsch, H. C. Dorn, P. P. Fatouros and M. D. Shultz: ‘A dual PET/MR imaging nanoprobe: 124I Labeled Gd3N@C80’, Appl. Sci., 2012, 2, (2), 465–478.
- J. Potocnik: ‘On a code of conduct for responsible nanosciences and nanotechnologies research’, 2009, EUR 23906, ISBN 978-92-79-11605-6 Luxembourg, European Commission.
- V. Stone, S. Hankin, R. Aitken, K. Aschberger, A. Baun, F. M. Christense, T. F. Fernandes, S. F. Hansen, N. B. Hartmann, G. R. Hutchison, H. Johnson, C. Micheletti, S. Read, B. Ross, B. Sokull-Kluettgen, D. Stark and L. Tran: ‘ENRHES: Engineered nanoparticles: review of health and environmental safety’, 2010, Brussels, European Commission.
- G. Szekely, M. C. Amores de Sousa, M. Gil, F. Castelo Ferreira and W. Heggie: ‘Genotoxic impurities in pharmaceutical manufacturing: sources, regulations, and mitigation’, Chem. Rev., 2015, 115, (16), 8182–8229.
- A. Sharma, S. V. Madhunapantula and G. P. Robertson: ‘Toxicological considerations when creating nanoparticle-based drugs and drug delivery systems’, Expert Opin. Drug Metab. Toxicol., 2012, 8, 47–69.
- E. A. Kyzyma, A. A. Tomchuk, L. A. Bulavin, V. I. Petrenko, L. Almasy, M. V. Korobov, D. S. Volkov, I. V. Mikheev, I. V. Koshlan, N. A. Koshlan, P. Blaha, M. V. Avdeev and V. L. Aksenov: ‘Structure and toxicity of aqueous fullerene C60 solutions’, J. Surf. Invest.: X-ray, Synchrotron Neutron Tech., 2015, 9, (1), 1–5.
- C. M. Sayes, J. D. Fortner, W. Guo, D. Lyon, A. M. Boyd, K. D. Ausman, Y. J. Tao, B. Sitharaman, L. J. Wilson, J. B. Hughes, J. L. West and V. L. Colvin: ‘The differential cytotoxicity of water-soluble fullerenes’, Nano Lett., 2004, 4, (10), 1881–1887.
- J. Gao, Y. Wang, K. M. Folta, V. Krishna, W. Bai, P. Indeglia, A. Georgieva, H. Nakamura, B. Koopman and B. Moudgil: ‘Polyhydroxy fullerenes (fullerols or fullerenols): beneficial effects on growth and lifespan in diverse biological models’, PLoS ONE, 2011, 6, (5), 1–8.
- A. Djordjevic and G. Bogdanovic: ‘Fullerenol: a new nanopharmaceutic?’, Arch. Oncol., 2008, 16, (3–4), 42–45.
- B. Srdjenovic, V. Milic-Torres, N. Grujic, K. Stankov, A. Djordjevic and V. Vasovic: ‘Antioxidant properties of fullerenol C60(OH)24 in rat kidneys, testes, and lungs treated with doxorubicin’, Toxicol. Mech. Method, 2010, 20, (6), 298–305.
- J. Fan, G. Fang, F. Zeng, X. Wang and F. Wu: ‘Water-dispersible fullerene aggregates as a targeted anticancer prodrug with both chemo- and photodynamic therapeutic actions’, Small, 2013, 9, 613–621.
- K. K. Jain: ‘The handbook of nanomedicine’, 2nd edn, 2012, New York, Springer.
- J. Besley: ‘Current research on public perceptions of nanotechnology’, Emerg. Health Threats J., 2010, 3, e8.
- N. Pidgeon, B. H. Harthorn, K. Bryant and T. Rogers-Hayden, ‘Deliberating the risks of nanotechnologies for energy and health applications in the United States and United Kingdom’, Nat. Nanotechnol., 2009, 4, (2), 95–98.
- M. Siegrist, A. Wiek, A. Helland and H. Kastenholz: ‘Risks and nanotechnology: the public is more concerned than experts and industry’, Nat. Nanotechnol., 2007, 2, (2), 67.
- H. Rauscher, B. Sokull-Klüttgen and H. Stamm: ‘The European commission's recommendation on the definition of nanomaterial makes an impact’, Nanotoxicology, 2012, 7, 1–3.
- R. H. Baughman, A. A. Zakhidov and W. A. de Heer: ‘Carbon nanotubes on the route toward applications’, Science, 2002, 297, 787.
- H. Shen, L. Zhang, M. Liu and Z. Zhang: ‘Biomedical applications of graphene’, Theranostics, 2012, 2, (3), 283–294.
- I. L. Medintz, H. Mattoussi and A. R. Clapp: ‘Potential clinical applications of quantum dots’, Int. J. Nanomed., 2008, 3, (2), 151–167.
- M. Mahmoudi, S. Sant, B. Wang, S. Laurent and T. Sen: ‘Superparamagnetic iron oxide nanoparticles (SPIONs): development, surface modification and applications in chemotherapy’,Adv. Drug Deliv. Rev., 2011, 63, (1–2), 24–46.