Background
Sleep disturbances are highly prevalent in patients treated for a primary brain tumor, with reported prevalence estimates ranging between 30 and 50% [Citation1–3]. Sleep disturbances may contribute to the development and exacerbation of other common co-occurring symptoms in brain cancer patients, including fatigue, depressed mood, and cognitive impairment [Citation4].
Despite advancements in precision radiotherapy (RT), it is often inevitable that healthy brain tissue is exposed to radiation. Although the exact neurobiological circuitry underlying the regulation of sleep–wake behaviors is not fully understood, evidence points to complex interactions between various neuroanatomical structures, including the brain stem, hypothalamus, and thalamus [Citation5–8]. Furthermore, since sleep is partly regulated by a circadian process [Citation9], structures relevant to the secretion of circadian and endocrine markers, such as the pineal and pituitary glands, are important [Citation10,Citation11]. Indeed, results from a recent cross-sectional study found that radiation to midline brain structures, including the pineal gland, was associated with lower levels of overnight melatonin secretion [Citation12].
While previous studies have investigated associations between radiation to specific brain structures and cognitive- and quality of life outcomes [Citation13–15], no study has yet investigated whether radiation dose to the brain is a risk factor for impaired sleep quality. Thus, the objective of the present study was to explore the potential associations between radiation dose to sleep-relevant brain structures and sleep quality in patients treated for a non-glioblastoma primary brain tumor.
Methods
Study design and participants
The data were collected as part of a pre-registered cross-sectional study with the primary aim of investigating associations between irradiation to the brain and cognitive functions (ClinicalTrials.gov: NCT04118426). The Research Ethics Committee of Central Denmark Region approved this study (no. 1-10-72-367-15). We have previously reported results on the primary outcomes [Citation13].
Patients who had previously received RT for brain tumors were invited. Applied inclusion criteria were: primary non-glioblastoma brain tumor according to WHO 2016 guidelines [Citation16]; RT between 2006 and 2016 at Aarhus University Hospital (AUH) or proton therapy; progression-free since RT; age >18 years; Karnofsky performance status = 60–100. Exclusion criteria were a diagnosis of glioblastoma and inadequate Danish language proficiency.
Study procedures
Consenting participants were scheduled for an in-person assessment, which included a questionnaire package and neurocognitive assessment with a battery of standardized neuropsychological tests [Citation13]. The data were collected from February 2017 to March 2018.
Sleep and other self-reported outcomes
Sleep quality was assessed with the Pittsburgh Sleep Quality Index (PSQI), a self-reported questionnaire designed to measure sleep quality and quantity over the past month [Citation17]. It consists of 19 items that make up seven subcomponents, each ranging from 0 to 3 and pertaining to: subjective sleep quality, sleep latency, sleep duration, habitual sleep efficiency, sleep disturbances, use of sleeping medication, and daytime dysfunction. A global sleep quality score (0–21) is calculated with higher scores indicating worse sleep. PSQI is a reliable and valid measure of sleep quality, including in cancer populations [Citation18], and has been used to assess sleep quality in brain tumor patients [Citation19]. Other self-reported outcomes were also collected [Citation13,Citation14].
Radiation dose to sleep/wake-relevant brain structures
Details about the RT regimen have been reported elsewhere [Citation14]. In brief, participants received three-dimensional conformal RT (2006–2008; n = 5), or intensity-modulated RT (IMRT) (after 2008; n = 64). RT was given with 1.8–2.0 Gray (Gy) fractions to total doses of 45–60 Gy. Nine patients were referred for proton therapy. Doses for these patients were converted to cobalt gray equivalents (GyRBE) assuming a constant relative biological effectiveness (RBE) factor of 1.1.
The following sleep/wake-related structures were delineated on the computerized tomography (CT) scan co-registered with a contrast enhanced 3D T1-weighted magnetic resonance imaging (MRI) scan according to national and European Particle Therapy Network guidelines [Citation20,Citation21]: brain stem, hypothalamus, thalamus, pineal gland, and pituitary gland. All contouring was performed by the same oncologist and reviewed by a neurosurgeon and a neuro-oncologist. Mean doses from these contours were converted to equivalent doses in 2 Gy fractions (EQD2) assuming an α/β ratio of 3 Gy [Citation22].
Statistical analysis
Descriptive statistics were computed for demographic, clinical, and treatment variables. Possible differences in sleep quality between tumor types were tested with ANOVAs. For each PSQI sub-component, a dichotomized score was calculated indicating a good or poor outcome. Patients characterized as poor sleepers were determined using established cutoffs for the PSQI global score (>5) [Citation17]. Mean radiation dose (Dmean) was compared between patients with good or poor sleep using independent t-tests with 1000 bootstrapped re-samplings. Due to the exploratory nature of the analyses, no adjustments for multiple comparisons were undertaken [Citation23]. Between-group differences with effect-sizes (d) exceeding ±0.4 were highlighted. Statistical analyses were performed using SPSS Statistics 28.0 (SPSS Inc., Chicago, IL).
Results
Participants
A total of 78 (64%) of 121 eligible patients (glioma, grade 1–3 = 28; meningioma = 22, pituitary = 16, other = 12) consented to participate in the present study. Median time since RT was 4.6 years (range 1–9 years). For details about demographic and clinical characteristics, see .
Table 1. Mean PSQI component and global scores and radiation doses to sleep- and wake-related brain structures.
Sleep quality
Mean PSQI global score for the total group was 5.13 (SD = 3.13). No differences were observed in overall sleep quality between tumor types (F[3, 74] = 0.37, η2 = .02). Poor sleep quality (PSQI >5) was reported by 37.2% (n = 29) of the sample. The frequency across different tumor types was 35.7% (n = 10) in patients with glioma, 40.9% in patients with meningioma, 50% (n = 8) in patients with a pituitary tumor, and 16.7% (n = 2) in patients with other tumor types. No associations were observed between the PSQI global score and years since diagnosis (r = –0.01, 95% CI [–0.22, .21]), years since RT (r = .08, 95% CI [–0.30, .15]), or age (r = –0.06, 95% CI [–0.28, .17]). For details regarding PSQI sub-components, see .
Radiation dose
Mean radiation doses to the brainstem, hypothalamus, thalamus, the pineal and pituitary glands are reported in .
Associations between radiation dose and sleep outcomes
The means, standard deviations, effect size estimates, 95% bias-corrected confidence intervals, and significance levels based on the bootstrapped t-tests for all PSQI components and global PSQI score in each brain region are presented in .
Table 2. Radiation dose to sleep- and wake-relevant structures in patients with good and poor outcomes on PSQI components and global score.
Patients reporting high levels of daytime dysfunction had received greater radiation doses to all of the brain regions examined, including the brain stem, hypothalamus, thalamus, pineal gland, and pituitary gland with medium to large effect sizes (ds = –0.54 to –0.90). All associations, except the association with radiation doses to the pineal gland, were statistically significant. Dose–volume histograms for high and low daytime dysfunction for significant brain structures are presented in .
Figure 1. Individual (iDVH) and mean dose volume histograms (DVH) for good (blue) and poor (red) daytime dysfunction across selected brain structures.
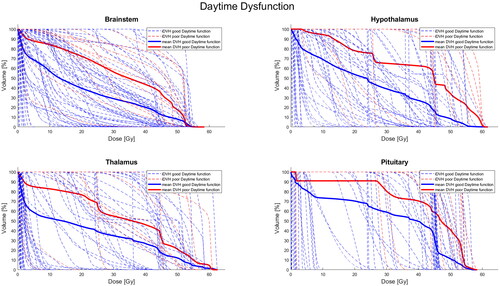
Associations corresponding to small-to-medium effect sizes were found across several PSQI sub-component scores and the pituitary gland. Specifically, those with poorer outcomes in subjective sleep quality, sleep latency, sleep disturbance, daytime dysfunction, as well as the global PSQI score tended to have received higher radiation doses to the pituitary gland than those who did not exceed the cutoffs. As noted, the association between daytime dysfunction and radiation dose to this region was also statistically significant. To investigate whether these findings were specifically driven by patients with pituitary tumors, sensitivity analyses were conducted excluding these patients from the analyses. The analyses revealed similar results in terms of the direction and magnitude of effect sizes, and the significant association with daytime dysfunction remained statistically significant (Cohen’s d = –0.80, 95% CI [–1.49, –0.10], p = .011).
Finally, two small-to-medium associations were also detected in the opposite direction from our predictions. Participants who reported poor subjective sleep quality received lower doses of radiation to the thalamus than those with good sleep quality. Furthermore, those who reported using sleep medicine received lower radiation doses to the hypothalamus than those who did not.
Discussion
To the best of our knowledge, no previous studies have investigated the association between radiation dose to specific brain structures and sleep quality. While the prevalence of long-term sleep disturbances in patients with primary brain tumors (37%) was comparable to other findings within this population [Citation19,Citation24], as well as with the general population [Citation25], the present results indicated that a higher radiation dose to the pituitary gland, brain stem, thalamus, and pineal gland could represent a risk factor for poor sleep quality. Daytime dysfunction may be particularly affected by higher radiation doses to these sleep-related brain areas. The daytime dysfunction sub-component of the PSQI relates to the consequences of sleep disturbances, including an impaired ability to maintain wakefulness and motivation during daytime activities. The component has shown good convergent validity with other measures of daytime fatigue and insomnia severity [Citation26].
Among the different sleep- and wake-related brain structures investigated, a higher radiation dose to the pituitary gland appeared to be the most substantial risk factor for poor sleep outcomes. Patients with poorer subjective sleep quality, longer sleep latency, more sleep disturbances, greater daytime dysfunction, and lower global sleep quality tended to have received higher mean radiation doses to the pituitary gland compared to patients who reported better sleep outcomes. Mean doses to the pituitary gland for each impaired sleep outcome ranged from 32.6 to 38.9 Gy, while patients with better sleep outcomes typically received mean radiation doses below 30 Gy. These findings remained consistent even when excluding patients with pituitary tumors in sensitivity analyses. A key regulator of the HPA axis is corticotropin releasing hormone which, when released, results in secretion of adrenocorticotropic hormone (ACTH) from the anterior pituitary triggering the production of cortisol [Citation27]. Cortisol and ACTH follow a diurnal pattern with a steep rise after awakening and a decline across the day [Citation11]. Previous studies in primary brain tumors patients have reported a high frequency of hypopituitarism following brain radiation with lower serum cortisol [Citation28–31]. Importantly, chronic ACTH deficiency can cause tiredness and lethargy [Citation32]. Thus, higher radiation doses to the pituitary gland may potentially disrupt HPA axis function and contribute to disturbed sleep and daytime dysfunction.
Two unexpected trends were also observed. First, participants who reported poor subjective sleep quality had received lower radiation doses to the thalamus, and patients taking sleep medication had received lower radiation to the hypothalamus, although these differences were not statistically significant. Given the lack of consistent patterns in other brain regions related to these sleep components, these findings may be chance occurrences due to multiple testing.
The strengths of our study include the long-term assessment of sleep quality in a relatively large sample (n = 78), detailed information on radiation doses to sleep-related regions of interest, the use of a validated measure of sleep quality, and robust statistical estimations based on random resampling. However, some limitations should be considered when interpreting our findings. The cross-sectional design limits our ability to establish causality. Further, we could not assess the contribution of other potential causal factors to sleep dysfunction, such as the impact of the tumor, surgery, chemotherapy, other medications, as well as radiation to additional brain structures that could be relevant for sleep. Additionally, objective sleep measures were not assessed, and multiple testing was conducted without correction due to the exploratory nature of the analysis [Citation23]. To address the latter, we have presented effect sizes and associated 95% confidence intervals.
In summary, our study suggests that more severe daytime dysfunction is potentially associated with higher radiation doses to sleep- and wake-relevant structures. Furthermore, a higher radiation dose to the pituitary gland appears to have detrimental effects on multiple sleep outcomes. While the long-term goal is to develop dose–toxicity profiles for each brain structure, specific recommendations regarding radiation doses to the pituitary gland and other structures to protect sleep are premature. However, our findings indicate that impaired sleep outcomes were more evident when the dose to the pituitary gland exceeded 30 Gy. This dose aligns with published data showing ACTH deficiency and increased risk for hypopituitarism at doses greater than 30 Gy [Citation33,Citation34].
Clearly, research is needed to confirm these initial findings using more specific measures to assess both sleep quantity and daytime dysfunction. We are currently undertaking a nationwide prospective study of brain tumor patients, assessing cognition and self-reported outcomes including sleep quality up to 10 years post-treatment. Given the deleterious impact of daytime dysfunction on quality of life in this population, and the established importance of sleep for general brain health, established non-pharmacological interventions, such as cognitive-behavioral therapy for insomnia, should specifically be tested in this population.
Disclosure statement
The authors report no competing interests.
Data availability statement
The data that support the findings are available from the corresponding author, AA, upon reasonable request.
Additional information
Funding
References
- Willis KD, Ravyts SG, Lanoye A, et al. Sleep disturbance in primary brain tumor: prevalence, risk factors, and patient preferences. Support Care Cancer. 2022;30(1):741–748.
- Armstrong TS, Shade MY, Breton G, et al. Sleep–wake disturbance in patients with brain tumors. Neuro Oncol. 2017;19(3):323–335.
- Martin JA, Hart NH, Bradford N, et al. Prevalence and management of sleep disturbance in adults with primary brain tumours and their caregivers: a systematic review. J Neurooncol. 2023;162(1):25–44. doi: 10.1007/s11060-023-04270-1.
- Amidi A, Wu LM. Circadian disruption and cancer- and treatment-related symptoms. Front Oncol. 2022;12:1009064.
- Saper CB, Fuller PM, Pedersen NP, et al. Sleep state switching. Neuron. 2010;68(6):1023–1042.
- Taran S, Gros P, Gofton T, et al. The reticular activating system: a narrative review of discovery, evolving understanding, and relevance to current formulations of brain death. Can J Anaesth. 2023;70(4):788–795. doi: 10.1007/s12630-023-02421-6.
- Saper CB, Scammell TE, Lu J. Hypothalamic regulation of sleep and circadian rhythms. Nature. 2005;437(7063):1257–1263.
- De Luca R, Nardone S, Grace KP, et al. Orexin neurons inhibit sleep to promote arousal. Nat Commun. 2022;13(1):1–15. doi: 10.1038/s41467-022-31591-y.
- Borbély AA, Daan S, Wirz-Justice A, et al. The two-process model of sleep regulation: a reappraisal. J Sleep Res. 2016;25(2):131–143. doi: 10.1111/jsr.12371.
- Claustrat B, Leston J. Melatonin: physiological effects in humans. Neurochirurgie. 2015;61:77–84.
- Hirotsu C, Tufik S, Andersen ML. Interactions between sleep, stress, and metabolism: from physiological to pathological conditions. Sleep Sci. 2015;8(3):143–152. doi: 10.1016/j.slsci.2015.09.002.
- Rosenkranz E, Thissen A, Siegel S, et al. Melatonin secretion following brain midline irradiation is diminished, but not correlated with subjective sleep disturbances. Clin Endocrinol. 2018;89(6):870–877. doi: 10.1111/cen.13814.
- Haldbo-Classen L, Amidi A, Lukacova S, et al. Cognitive impairment following radiation to hippocampus and other brain structures in adults with primary brain tumours. Radiother Oncol. 2020;148:1–7. doi: 10.1016/j.radonc.2020.03.023.
- Haldbo-Classen L, Amidi A, Wu LM, et al. Associations between patient-reported outcomes and radiation dose in patients treated with radiation therapy for primary brain tumours. Clin Transl Radiat Oncol. 2021;31:86–92. doi: 10.1016/j.ctro.2021.09.006.
- Brown PD, Gondi V, Pugh S, et al. Hippocampal avoidance during whole-brain radiotherapy plus memantine for patients with brain metastases: phase III trial NRG Oncology CC001. J Clin Oncol. 2020;38(10):1019–1029. doi: 10.1200/JCO.19.02767.
- Louis DN, Perry A, Reifenberger G, et al. The 2016 World Health Organization classification of tumors of the central nervous system: a summary. Acta Neuropathol. 2016;131(6):803–820. doi: 10.1007/s00401-016-1545-1.
- Buysse DJ, Reynolds CF, Monk TH, et al. The Pittsburgh Sleep Quality Index: a new instrument for psychiatric practice and research. Psychiatry Res. 1989;28(2):193–213.
- Beck SL, Schwartz AL, Towsley G, et al. Psychometric evaluation of the Pittsburgh Sleep Quality Index in cancer patients. J Pain Symptom Manage. 2004;27(2):140–148. doi: 10.1016/j.jpainsymman.2003.12.002.
- Jeon MS, Dhillon HM, Koh ES, et al. Exploring sleep disturbance among adults with primary or secondary malignant brain tumors and their caregivers. Neurooncol Pract. 2020;8(1):48–59. doi: 10.1093/nop/npaa057.
- Danish Neuro Oncology Group. DNOG – contouring guidelines; 2016. p. 12–19. Available from: www.dnog.dk
- Eekers D, Di Perri D, Roelofs E, et al. EPTN International Neurological Contouring Atlas - 2021 Update CancerData; 2021. doi: 10.17195/candat.2021.02.1.
- Baumann M, Begg AB. Basic clinical radiobiology. Int J Radiat Biol. 2010;86:106–112.
- Bender R, Lange S. Adjusting for multiple testing—when and how? J Clin Epidemiol. 2001;54(4):343–349. doi: 10.1016/s0895-4356(00)00314-0.
- Jeon MS, Dhillon HM, Agar MR. Sleep disturbance of adults with a brain tumor and their family caregivers: a systematic review. Neuro Oncol. 2017;19(8):1035–1046. doi: 10.1093/neuonc/nox019.
- Hinz A, Glaesmer H, Brähler E, et al. Sleep quality in the general population: psychometric properties of the Pittsburgh Sleep Quality Index, derived from a German community sample of 9284 people. Sleep Med. 2017;30:57–63. doi: 10.1016/j.sleep.2016.03.008.
- Dietch JR, Taylor DJ, Sethi K, et al. Psychometric evaluation of the PSQI in U.S. college students. J Clin Sleep Med. 2016;12(8):1121–1129. doi: 10.5664/jcsm.6050.
- Steiger A. Sleep and endocrinology. J Intern Med. 2003;254(1):13–22. doi: 10.1046/j.1365-2796.2003.01175.x.
- Constine LS, Woolf PD, Cann D, et al. Hypothalamic-pituitary dysfunction after radiation for brain tumors. N Engl J Med. 1993;328(2):87–94. doi: 10.1056/NEJM199301143280203.
- Agha A, Sherlock M, Brennan S, et al. Hypothalamic-pituitary dysfunction after irradiation of nonpituitary brain tumors in adults. J Clin Endocrinol Metab. 2005;90(12):6355–6360.
- Arlt W, Hove U, Müller B, et al. Frequency and frequently overlooked: treatment-induced endocrine dysfunction in adult long-term survivors of primary brain tumors. Neurology. 1997;49(2):498–506. doi: 10.1212/wnl.49.2.498.
- Darzy KH, Shalet SM. Hypopituitarism following radiotherapy. Pituitary. 2009;12(1):40–50. doi: 10.1007/s11102-008-0088-4.
- Husebye ES, Pearce SH, Krone NP, et al. Adrenal insufficiency. Lancet. 2021;397(10274):613–629. doi: 10.1016/S0140-6736(21)00136-7.
- Scoccianti S, Detti B, Gadda D, et al. Organs at risk in the brain and their dose-constraints in adults and in children: a radiation oncologist’s guide for delineation in everyday practice. Radiother Oncol. 2015;114(2):230–238. doi: 10.1016/j.radonc.2015.01.016.
- Kyriakakis N, Lynch J, Orme SM, et al. Hypothalamic-pituitary axis irradiation dose thresholds for the development of hypopituitarism in adult-onset gliomas. Clin Endocrinol. 2019;91(1):131–140.