Introduction
Radiation-induced brainstem necrosis is a severe toxicity that can occur following radiotherapy of tumours in the vicinity of the brainstem [Citation1,Citation2]. Paediatric patients may be particularly at risk of brainstem necrosis since central nervous system (CNS) tumours are common paediatric diagnoses and the brainstem is central to children’s neurological and cognitive development. Due to increased normal tissue sparing, paediatric patients are often referred to proton therapy [Citation3]. However, while proton therapy currently uses a constant relative biological effectiveness (RBE) of 1.1, pre-clinical results have shown the RBE to vary with several factors including linear energy transfer (LET) [Citation4], although clinical evidence of a variable RBE is still inconclusive [Citation5].
In a previous study, normal tissue complication probability (NTCP) models were fitted that describe symptomatic brainstem necrosis in paediatric ependymoma patients [Citation6]. The models were based on a case-control study of paediatric patients treated with proton therapy where the cases developed symptomatic brainstem necrosis following treatment [Citation7]. In the study, a trend of increased dose-averaged LET (LETd) in the high dose volume of the brainstem for the cases was identified. However, NTCP models are specific to the patients on which the models are fitted and an important part of NTCP modelling is to investigate how the models perform in an independent cohort [Citation8,Citation9]. In this study, we therefore aimed to apply the models in an independent paediatric patient cohort. Model performance was analysed both between and within patients, in three main parts: first, the delivered dose and LETd-distributions for individual patients were used to calculate brainstem NTCPs using the risk models, second, we investigated how the models performed for LETd-optimised plans specifically aiming to lower the estimated NTCP as much as possible and, finally, variation in model performance and NTCP estimates within patients due to range and setup uncertainties was considered both for the delivered plans and the LETd-optimised plans.
Materials and method
Patient cohort and dose/LETd calculations
Seven paediatric patients treated for brain tumours with a prescription dose of 54 Gy(RBE) were selected for this study. The patients were selected from a larger cohort of 47 paediatric CNS tumour patients treated with pencil beam scanning proton therapy at the Danish Centre for Particle Therapy (DCPT). Patients were excluded if they did not undergo focal irradiation or if no part of the brainstem received at least 54 Gy(RBE). Dose and LETd-distributions were recalculated in the TOPAS v3.5 Monte Carlo code with the protonLET scorer and the simulations were performed with 15–25 million primary particles per field. LETd considered primary and secondary protons and was scored in water. The study was approved by the internal review board at the DCPT.
Implementation of model
Previously constructed NTCP models describing the risk of brainstem necrosis were applied [Citation6]. The models were based on a case-control cohort (28 patients in total, including 7 brainstem necrosis cases) selected from a total of 954 paediatric brain tumour patients treated at the University of Florida Health Proton Therapy Institute between 2009 and 2017 using double scattering proton therapy [Citation7]. The models used the L10% (the LETd to the 10% of voxels with the highest LETd) of the brainstem receiving dose over either 54 Gy(RBE) or 50 Gy(RBE), referred to as L10% (D > 54 Gy(RBE)) and L10% (D > 50 Gy(RBE)), respectively. Further information on the models as well as the model equations are provided in the supplementary material.
Brainstem risk calculations for clinically delivered treatment
The L10% (D > 54 Gy(RBE)) and L10% (D > 50 Gy(RBE)) were calculated for all seven patients. Equations A1, A2 (supplementary material) were subsequently used to calculate NTCP estimates for all patients from the delivered plans.
LETd-optimisation
To minimise risk of brainstem necrosis and assess model performance in the lower limit of LETd-values, all treatment plans were re-optimised to lower LETd in the high dose volume of the brainstem. The LETd-optimisation was performed using Raystation version 11B (RaySearch Laboratories, Stockholm, Sweden). First, the delivered plans, which were robustly optimised considering range and setup uncertainty, were imported to Raystation and the dose was normalised to an average dose of 54 Gy(RBE) to the clinical target volume (CTV). These recalculated plans served as reference plans. The plans were then re-optimised with varying dose criteria to the brainstem, ensuring the maximum brainstem dose did not increase from the reference plans, as well as LETd-criteria to the parts of the brainstem receiving dose over 50 and 54 Gy(RBE). The planning target volume (PTV) had an isotropic 3 mm CTV to PTV margin to act as a surrogate for range and setup uncertainty. The LETd-optimised plans were PTV-based since robust LETd-optimisation was not available in Raystation 11B at the time this work was performed. The LETd-optimisation caused two of the patients (Patients 4 and 6) to have no or negligible volumes with a dose over 54 Gy(RBE) in the brainstem and these were consequently removed from the analysis of the 54 Gy(RBE) threshold LETd-optimised plans.
Robustness assessment
The variation in LETd and estimated brainstem necrosis risk with range and setup uncertainties was also assessed using Raystation. Range uncertainties were considered by scaling the computed tomography calibration curves with 3.5%, while setup uncertainties were considered by changing the position of the isocentre by 2 mm in each spatial direction. The scenarios with the highest and lowest LETd were considered both for the robustly optimised reference plans and the PTV-based LETd-optimised plans with range and setup uncertainties in one spatial direction considered simultaneously.
Results
The simulated LETd-distributions from the delivered plans showed NTCP54Gy(RBE) ranging from 6.7 to 10.3% [95% confidence interval: 3.0, 19.8] () corresponding to L10% (D > 54 Gy(RBE)) varying between 2.9 and 4.1 keV/µm. The NTCP50Gy(RBE) estimates were between 3.8 and 5.2% [3.0, 17.4] (), with L10% (D > 50 Gy(RBE)) values ranging from 2.8 to 4.2 keV/µm. Similar LETd-values were seen for the reference plans recalculated in Raystation ().
Figure 1. NTCP estimates for all seven patients. a) NTCP from the delivered plans simulated in TOPAS. The point estimate is given by the tallest vertical line while the 95% confidence interval is shown with the slightly transparent line and shorter vertical lines. NTCP54Gy(RBE) is shown with a full line while NTCP50Gy(RBE) is shown with a dashed line. b) NTCP from the LETd-optimised plans where the lines represent the same as in subfigure 1a. c) NTCP from the reference plan in Raystation where robustness scenarios are included with the full horizontal line and the inner short vertical lines. The 95% confidence intervals correspond to the lower and higher robustness scenarios and are, as in subfigures 1a and 1b, also shown by a slightly transparent line. d) NTCP from the LETd-optimised plans in Raystation where the representation is the same as subfigure 1c.
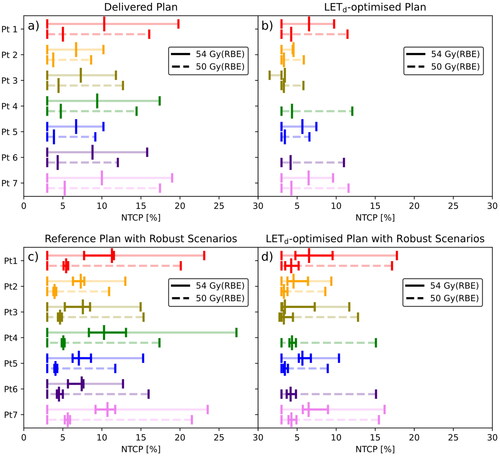
Figure 2. LETd-volume histograms showing brainstem LETd for all patients. a) is the LETd in the brainstem volume receiving dose over 54 Gy(RBE) from the reference plans recalculated in Raystation. NTCP54Gy(RBE) is shown with the horizontal line and shows the L10% for each Curve. b) is the LETd in the brainstem volume with dose over 50 Gy(RBE) from the recalculated reference plans and NTCP50Gy(RBE) shown as a second axis. c) shows a similar plot as subfigure 2a except it shows the curves for the LETd-optimised plans instead while d) is a corresponding plot to subfigure 2b for the LETd-optimised plans.
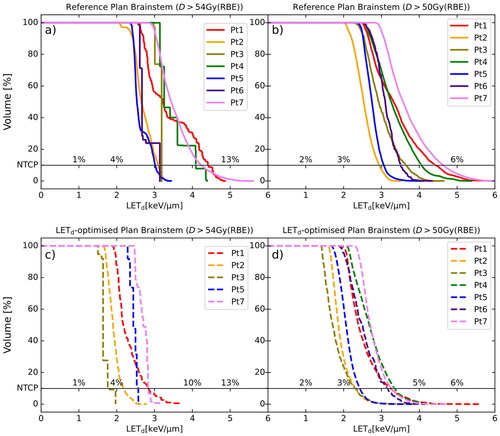
With LETd-optimisation, the L10% (D > 54 Gy(RBE)) was reduced to between 1.8 and 2.8 keV/µm () with NTCP54Gy(RBE) ranging between 3.4% [3.0, 1.5] and 6.5% [3.0, 9.7] (). The L10% (D > 50 Gy(RBE)) varied between 2.3 and 3.3 keV/µm () with NTCP50Gy(RBE) ranging from 3.3% [3.0, 5.8] to 4.3% [3.0, 12.1] (). CTV and brainstem dose remained similar between the reference plans and the LETd-optimised plans ().
Figure 3. Dose-volume histograms for all seven patients showing the CTV and brainstem dose from the delivered reference plan to the left and for the LETd-optimised plans to the right. CTV doses are shown with the full while the brainstem is given by the dashed lines.
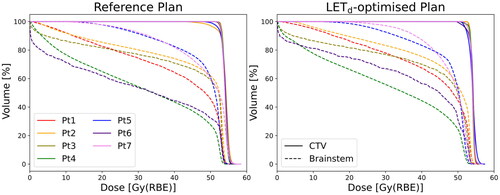
Robustness assessment of the reference plans showed intra-patient NTCP54Gy(RBE) varying between 1.5 and 4.7 percentage points, and intra-patient NTCP50Gy(RBE) varying between 0.4 and 0.8 percentage points due to range and setup uncertainties ( and Figure A1). This corresponded to L10% (D > 54 Gy(RBE)) variation between 0.5 and 1.6 keV/µm, and L10% (D > 50 Gy(RBE)) variation between 0.1 and 0.8 keV/µm. The LETd robustness for the LETd-optimised plans was similar for the 54 Gy(RBE) threshold but slightly worse for the 50 Gy(RBE) threshold. NTCP54Gy(RBE) varied between 1.5 and 4.8 percentage points while NTCP50Gy(RBE) varied between 0.5 and 1.7 percentage points (). The corresponding L10% (D > 54 Gy(RBE)) differences were between 0.5 and 1.6 keV/µm and the L10% (D > 50 Gy(RBE)) differences were between 0.6 and 1.7 keV/µm.
Discussion
In this study, we have applied LETd-inclusive brainstem NTCP models in an independent cohort. Variations in LETd both between patients and within patients based on robustness and re-optimisation showed NTCP54Gy(RBE) to vary with under five percentage points while NTCP50Gy(RBE) variance stayed below two percentage points. Across all patients and scenarios, the NTCP54Gy(RBE) was in the range of 3.1% to 13.1% [3.0%, 27.2%] while NTCP50Gy(RBE) was in the range of 2.7% to 5.9% [3.0%, 21.5%]. These risk estimates are high compared to incidence rates found for larger paediatric cohorts in the literature [Citation2,Citation10,Citation11] partly explained by the model basing incidence on a study with a relatively high incidence rate of 5.5% [Citation12]. The NTCP estimates achieved with LETd-optimisation also demonstrated the limited range of model applicability.
LET-optimisation both using the Raystation treatment planning system [Citation13] and other in-house software [Citation14] has been a topic of interest in recent publications and has shown the potential to lower LET in OARs without compromising dose. However, the evaluation of achieved LET distributions is either purely based on LET values or linked to NTCP using variable RBE models. The uncertainty surrounding current state-of-the-art RBE models means the latter approach is associated with uncertain NTCP estimates. The development of models associating LET with common NTCP endpoints may therefore be an important step towards clinical implementation of LET-optimisation. Here an example is demonstrated, with NTCP based on a subvolume of high dose and LETd in the brainstem.
One of the most central limitations of this study is the lack of outcome data in the cohort, preventing this work from being a true validation of the models. Since the models describe relative risk, the validation of the models is also difficult due to the estimated risk being low for the typical LETd-ranges seen in proton therapy.
The lack of robustness considerations in the LET optimisation was shown to have relatively minor impact on the resulting LET scenarios. Previous studies have also assessed the robustness of LET-distributions following proton treatment planning [Citation15,Citation16]. The studies show that range and setup uncertainties do affect LET-distributions and taking the robustness of LET into account in LET optimisation is likely to strengthen the treatment plans achievable with this technique.
Another aspect to consider for LET optimisation is that dose may already be slightly compromised in favour of LET-distributions when avoiding several distal edges of proton beams in an OAR [Citation17]. This work did not explore altered beam positions with LETd-optimisation but the technique may allow greater flexibility in how beams are positioned in proton therapy.
A limitation of the NTCP models studied here that becomes evident for certain patients in this cohort is the unreliability of NTCP values when the volumes become very small, which is a consequence of the choice of dose thresholds. The small size of the volume may make it more sensitive to errors in dose calculation and contouring despite the brainstem being a serial organ and sensitive to hotspots in small volumes. The 54 Gy(RBE) threshold is also a clinically relevant dose threshold since it is a common prescription dose for paediatric CNS tumours and the safety of subjecting the brainstem to a dose of 54 Gy(RBE) is a topic discussed in the QUANTEC report [Citation18], but the 50 Gy(RBE), while containing a larger volume, has no explicit clinical relevance. LETd in high dose volumes was also prioritised in the LETd-optimisation leading to increased brainstem mean dose for most patients compared to the reference plans (), which may be impactful despite having no effect on the NTCP estimates [Citation6].
The limitation in applicable LETd-range for the patients is also demonstrated in this model application. When no part of the brainstem received dose over 54 Gy(RBE) which was the case for the LETd-optimised plan of patient 6, the NTCP54Gy(RBE)-estimate was below zero (Table A1 in supplementary materials). Additionally, the upper and lower confidence intervals were reversed relative to the actual model estimate for the LETd-optimised plans of certain patients. Consequently, these models were difficult to use as a means of quantifying NTCP improvements from LETd optimisation since the actual LETd values became too low for the model to give reasonable estimates.
A factor that may also impact the risk estimates is that the models were based on double scattering proton therapy data, while the patients in this study were treated with intensity-modulated proton therapy. LET-distributions differ slightly between the two modalities [Citation19] which may affect how necrosis develops.
In conclusion, we applied two NTCP models associating brainstem necrosis with the LETd in the high dose volumes of the brainstem following proton therapy of paediatric brain tumours. The models gave low estimated risk of brainstem necrosis and demonstrated that the risk could be further reduced using LET optimisation. The importance of robustly optimising proton plans in terms of not only dose but also LET distributions is emphasised since LET may also have an impact on the NTCP of the patient.
Supplemental Material
Download MS Word (405.9 KB)Disclosure statement
No potential conflict of interest was reported by the author(s).
Data availability
The participants of this study did not give written consent for their data to be shared publicly, so due to the sensitive nature of the research supporting data is not available.
Additional information
Funding
References
- Yock TI, Constine LS, Mahajan A. Protons, the brainstem, and toxicity: ingredients for an emerging dialectic. Acta Oncol. 2014;53(10):1279–1282. doi: 10.3109/0284186X.2014.957415.
- Indelicato DJ, Flampouri S, Rotondo RL, et al. Incidence and dosimetric parameters of pediatric brainstem toxicity following proton therapy. Acta Oncol. 2014;53(10):1298–1304. doi: 10.3109/0284186X.2014.957414.
- Stokkevag CH, Indelicato DJ, Herfarth K, et al. Normal tissue complication probability models in plan evaluation of children with brain tumors referred to proton therapy. Acta Oncol. 2019;58(10):1416–1422. doi: 10.1080/0284186X.2019.1643496.
- Paganetti H, Niemierko A, Ancukiewicz M, et al. Relative biological effectiveness (RBE) values for proton beam therapy. Int J Radiat Oncol Biol Phys. 2002;53(2):407–421. doi: 10.1016/s0360-3016(02)02754-2.
- Underwood TSA, McNamara AL, Appelt A, et al. A systematic review of clinical studies on variable proton relative biological effectiveness (RBE). Radiother Oncol. 2022;175:79–92. doi: 10.1016/j.radonc.2022.08.014.
- Handeland AH, Indelicato DJ, Fjæra LF, et al. Linear energy transfer-inclusive models of brainstem necrosis following proton therapy of paediatric ependymoma. Phys Imaging Radiat Oncol. 2023;27:100466. doi: 10.1016/j.phro.2023.100466.
- Fjæra LF, Indelicato DJ, Handeland AH, et al. A case-control study of linear energy transfer and relative biological effectiveness related to symptomatic brainstem toxicity following pediatric proton therapy. Radiother Oncol. 2022;175:47–55. doi: 10.1016/j.radonc.2022.07.022.
- Engeseth GM, Stokkevåg C, Muren LP. Achievements and challenges in normal tissue response modelling for proton therapy. Phys Imaging Radiat Oncol. 2022;24:118–120. doi: 10.1016/j.phro.2022.11.004.
- Bentzen SM, Constine LS, Deasy JO, et al. Quantitative analyses of normal tissue effects in the clinic (QUANTEC): an introduction to the scientific issues. Int J Radiat Oncol Biol Phys. 2010;76(3 Suppl):S3–S9. doi: 10.1016/j.ijrobp.2009.09.040.
- Gentile MS, Yeap BY, Paganetti H, et al. Brainstem injury in pediatric patients with posterior fossa tumors treated with proton beam therapy and associated dosimetric factors. Int J Radiat Oncol Biol Phys. 2018;100(3):719–729. doi: 10.1016/j.ijrobp.2017.11.026.
- Haas-Kogan D, Indelicato D, Paganetti H, et al. National cancer institute workshop on proton therapy for children: considerations regarding brainstem injury. Int J Radiat Oncol Biol Phys. 2018;101(1):152–168. doi: 10.1016/j.ijrobp.2018.01.013.
- Indelicato DJ, Bradley JA, Rotondo RL, et al. Outcomes following proton therapy for pediatric ependymoma. Acta Oncol. 2018;57(5):644–648. doi: 10.1080/0284186X.2017.1413248.
- Hahn C, Heuchel L, Ödén J, et al. Comparing biological effectiveness guided plan optimization strategies for cranial proton therapy: potential and challenges. Radiat Oncol. 2022;17(1):169. doi: 10.1186/s13014-022-02143-x.
- Liu C, Patel SH, Shan J, et al. Robust optimization for intensity modulated proton therapy to redistribute high linear energy transfer from nearby critical organs to tumors in head and neck cancer. Int J Radiat Oncol Biol Phys. 2020;107(1):181–193. doi: 10.1016/j.ijrobp.2020.01.013.
- Rana S, Traneus E, Jackson M, et al. Quantitative analysis of dose-averaged linear energy transfer (LETd) robustness in pencil beam scanning proton lung plans. Med Phys. 2022;49(5):3444–3456. doi: 10.1002/mp.15569.
- Hahn C, Eulitz J, Peters N, et al. Impact of range uncertainty on clinical distributions of linear energy transfer and biological effectiveness in proton therapy. Med Phys. 2020;47(12):6151–6162. doi: 10.1002/mp.14560.
- Toma-Dasu I, Dasu A, Vestergaard A, et al. RBE for proton radiation therapy – a Nordic view in the international perspective. Acta Oncol. 2020;59(10):1151–1156. doi: 10.1080/0284186X.2020.1826573.
- Mayo C, Yorke E, Merchant TE. Radiation associated brainstem injury. Int J Radiat Oncol Biol Phys. 2010;76(3 Suppl):S36–S41. doi: 10.1016/j.ijrobp.2009.08.078.
- Grassberger C, Trofimov A, Lomax A, et al. Variations in linear energy transfer within clinical proton therapy fields and the potential for biological treatment planning. Int J Radiat Oncol Biol Phys. 2011;80(5):1559–1566. doi: 10.1016/j.ijrobp.2010.10.027.