Abstract
Background
The purpose of this study was to investigate acute normal tissue responses in the head and neck region following proton- or X-irradiation of a murine model.
Materials and methods
Female C57BL/6J mice were irradiated with protons (25 or 60 MeV) or X-rays (100 kV). The radiation field covered the oral cavity and the major salivary glands. For protons, two different treatment plans were used, either with the Bragg Peak in the middle of the mouse (BP) or outside the mouse (transmission mode; TM). Delivered physical doses were 41, 45, and 65 Gy given in 6, 7, and 10 fractions for BP, TM, and X-rays, respectively. Alanine dosimetry was used to assess delivered doses. Oral mucositis and dermatitis were scored using CTC v.2.0-based tables. Saliva was collected at baseline, right after end of irradiation, and at day 35.
Results
The measured dose distribution for protons (TM) and X-rays was very similar. Oral mucositis appeared earlier, had a higher score and was found in a higher percentage of mice after proton irradiation compared to X-irradiation. Dermatitis, on the other hand, had a similar appearance after protons and X-rays. Compared to controls, saliva production was lower right after termination of proton- and X-irradiation. The BP group demonstrated saliva recovery compared to the TM and X-ray group at day 35.
Conclusion
With lower delivered doses, proton irradiation resulted in similar skin reactions and increased oral mucositis compared to X-irradiation. This indicates that the relative biological effectiveness of protons for acute tissue responses in the mouse head and neck is greater than the clinical standard of 1.1. Thus, there is a need for further investigations of the biological effect of protons in normal tissues.
Background
Radiotherapy of head and neck (H&N) cancer may damage normal tissue surrounding the tumor, thereby causing detrimental acute and late side effects [Citation1]. Acute side effects such as skin dermatitis and oral mucositis usually occur during or immediately after treatment and may cause an unfavorable treatment halt [Citation2,Citation3]. Therefore, approaches that can reduce normal tissue damage and thus decrease side effects from radiation therapy are warranted, and proton therapy has emerged as an important tissue-sparing approach.
Compared to conventional X-irradiation, proton irradiation has a more favorable dose distribution where a high dose is deposited in the so-called Bragg peak with no dose deposited further into the tissue [Citation4]. Thus, proton irradiation can reduce the radiation dose to normal tissues, especially behind the tumor. However, published studies point toward differences in biological responses between protons and X-rays, such as in DNA damage repair, gene expression modulation, and inflammatory regulation [Citation5–10]. Relative biological effectiveness (RBE) is defined as the ratio of a reference X-ray dose to the proton dose that induce the same biological effect. It depends on several variables such as the dose per fraction, linear energy transfer (LET), tissue type, and biological endpoint [Citation11–13]. Despite accumulated experimental data on the variability of proton RBE, a fixed standard value for RBE of 1.1 for protons is used at most treatment centers, but there is a growing awareness of RBE variation along the proton path [Citation14–17].
Increasing clinical evidence shows that proton therapy is superior to X-ray therapy in lowering the radiation dose to normal tissue and thus reducing side effects [Citation18–20]. Still, for H&N cancer, there is a scarcity of randomized clinical studies directly comparing acute or late side effects from proton and X-therapy, and more knowledge regarding the differences in normal tissue responses between X-ray and proton irradiation is needed. The purpose of this preclinical study was thus to investigate acute normal tissue responses following H&N proton- and X-irradiation to gain new knowledge on the differences in biological effects between the two radiation types.
Materials and methods
Animals
Female C57BL/6J mice purchased from Janvier (France) were used in this study. Mice were kept in a 12-h light/12-h dark cycle under pathogen-free conditions and fed standard commercial fodder with water given ad libitum. Standard housing with nesting material and refuge was provided. Animals were 12 weeks old at the onset of experiments. All animal experiments in Norway were performed in accordance with directive 2010/63/EU on the protection of animals used for scientific purposes and approved by the Norwegian Food Safety Authority (ID 27931). All animal experiments in Denmark were performed in accordance with the animal welfare policy of Aarhus University and approved by the Danish Animal Experiments Inspectorate.
Irradiation procedure
X-irradiation was performed with a Faxitron Multirad225 irradiation system (Faxitron Bioptics, Tucson, AZ, USA) at the Radium Hospital in Oslo, Norway. Mice in the X-ray group were irradiated with a total dose of 65 Gy given in 10 fractions over 10 days with the following settings: 100 kV X-ray potential, 15 mA current, 2.0 mm Al filter, and 0.68 Gy/min dose rate.
Absolute dosimetry of the X-ray system was conducted using an FC65-G Farmer type ionization chamber (IBA Dosimetry, Germany) together with a MAX-4000 electrometer (Standard Imaging, USA) according to IAEA report TRS-277 [Citation21]. Dosimetry for the collimated case was performed using GafchromicTM EBT3 dosimeter films at a source-surface distance representing the skin entrance of the mouse. Calibration was done using an unshielded beam. EBT3 films (lot No. 02122001) were irradiated and processed according to the recommended protocols specified for radiochromic film dosimetry in the report of AAPM Task Group 235 [Citation22]. The reported dose for the X-ray group is the mean dose at the midpoint in the X-ray path through the mouse. Alanine dosimetry was used to measure delivered X-ray and proton depth dose profiles, and the Monte Carlo method was used to simulate the dose in 3D () (see Supplementary Materials).
Proton irradiation was performed using the Varian ProBeam clinical gantry with a pencil beam scanning dedicated nozzle (Varian, Medical Systems, Palo Alto, CA, USA) at the Danish Center for Particle Therapy at Aarhus University Hospital in Aarhus, Denmark. Two different proton treatment groups were included. The first proton treatment group (BP group) was irradiated with a total physical dose of 40.8 Gy given in six fractions over six days using a single-energy pristine Bragg peak (70 MeV degraded by 3 cm solid water). For this group, the distal edge of Bragg peak is located in the middle of the mouse). The second proton treatment group (TM group) was irradiated with a total physical dose of 44.8 Gy given in seven fractions over seven days with a single energy pristine Bragg peak (70 MeV degraded by 1 cm solid water). In this ‘transmission mode’, the Bragg peak ended up outside the mouse (). The proton entrance energy/range was estimated to be 25 MeV/6.8 mm and 60 MeV/31 mm for the BP and TM groups, respectively.
Figure 1. Timeline (A), Graphical treatment plan (B) and corresponding FLUKA MC simulations (C) of the X-ray and proton irradiation experiments.
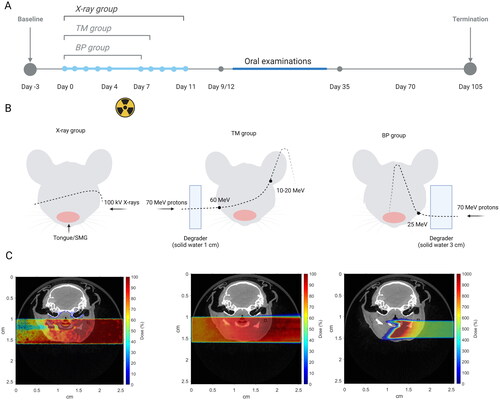
Day 0 was the time point where the first irradiation was performed. For X-ray and proton irradiation, mice were anesthetized using gas anesthesia with 4% Sevoflurane in O2. Mice were positioned laterally, on the right side for the X-ray and BP groups and on the left side for the TM group, in a custom-made foam holder with the beam entering on the opposite side (Supplementary Figure 1). The mice in the TM group were positioned on the left side to approximately match the proton and X-ray dose distributions. A lead (for X-rays) or brass (for protons) collimator was custom-built to define a radiation field of 25 × 20 mm covering the oral cavity, pharynx, and major salivary glands and placed on top of the foam holder. The radiation field was carefully planned to only irradiate the tissues of interest and avoid exposure to the eyes and brain.
Experimental protocol and study groups
The experimental timeline for proton and X-ray experiments is presented in . On day −3, saliva collections were performed in all animals as baseline measurements. On days 0–4 and 7–11, X-ray-fractionated treatment was given once a day around 4 pm, as explained above. During the acute phase (days 8–35), mice were monitored daily, and examinations of the oral cavity were performed every second day in order to obtain documentation of the acute tissue responses. Oral mucositis and lip dermatitis were scored using modified scoring tables (Supplementary Tables 1 and 2) based on CTC v. 2.0 [Citation23–25].
On days 0–4 and 7, proton-fractionated treatment was given once a day around 4 pm to both proton groups, as explained above. On day 8, irradiation treatment was terminated for the BP group due to substantial weight loss and observed oral mucositis. The TM group received one more fraction on day 8 and irradiation treatment was then terminated on day 9 due to substantial weight loss. To alleviate the pain from oral mucositis, analgesic treatment using Temgesic injections was started right after saliva collection (day 12 for the X-ray group and day 9 for the TM and BP groups) and administered to all mice through intraperitoneal injections every 8 h for 5 days. In addition, easily digestible and nutrition-rich gel fodder (DietGel Recovery, Clear H2O) and saline injection (0.1–0.3 ml subcutaneously administered) were provided to all mice to avoid unnecessary mortality. However, several mice in the proton groups reached humane endpoints and were euthanized between days 12–18 according to the protocol approved by the national animal authorities. Additional samples of saliva were collected immediately after termination of irradiation treatment (day 12 for the X-ray group and day 9 for the BP and TM groups), and at the end of the acute phase on day 35. The maximum follow-up period was day 105 after the onset of fractionated irradiation. However, the acute phase was considered to be over on day 35, so only this period is included in the current study.
Saliva collection
Saliva volume measurements were used to assess salivary gland function and saliva production. Saliva collection was performed as previously described [Citation26,Citation27]. Briefly, 0.375 mg/kg of pilocarpine (pilocarpine hydrochloride, Sigma) was intraperitoneally administered to the mice under anesthesia to stimulate saliva production. Saliva was collected into a cotton swab for 15 min and was then centrifuged at 7500 g and 4 °C for 2 min. The obtained volume was measured and stored at −80 °C. Data from two nonirradiated control groups from the involved research facilities were pooled.
Statistical analysis
Statistical analysis was performed using Prism 8 for Windows (Version 8.3.0, GraphPad Software, LLC). Saliva volume measurements were compared using a Mann–Whitney test with a significance level of 0.05.
Results
The dose distributions for X-ray and proton irradiations were measured using alanine dosimetry (). The results for X-ray and TM groups showed that the depth dose profiles within the region of the irradiation field are virtually the same for two different radiation types with approximately the same dose per fraction. Therefore, mice from X-ray and TM groups received the same dose distribution, gradually decreasing from the left to the right side. Because of the alanine pellet thickness and the narrow width of Bragg peak, the depth dose profile for BP proton irradiation cannot display the Bragg peak but a steep dose gradient is indeed seen. Thus, as also indicated by the Monte Carlo simulations, the distal edge of Bragg peak was likely located around the midplane of the mouse in the BP group, as planned.
Figure 2. Depth dose profiles based on alanine dosimetry. All points represent the mean dose per pellet with 1 standard error indicated as error bars.
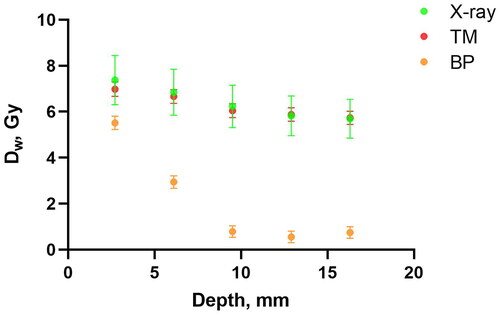
The onset of lip dermatitis was seen on day 8 for all groups. The mean lip dermatitis severity score was quite similar in the X-ray and proton groups (); however, this score only represented the highest value observed locally in each mouse. In the BP group, we observed less severe dermatitis on the left side than on the right side of the lip, confirming that the Bragg peak was deposited in the middle of the mouse. The severity score on the right side of the lip in the BP group was equal to the severity score observed in the entire lip of the TM and X-ray groups. Moreover, we observed large areas of dry and moist desquamation followed by fur loss on the chest of TM and X-ray groups. In contrast, only dry desquamation followed by fur loss was observed in similar areas of the chest in the BP group.
Figure 3. Timeline of median lip dermatitis score (A) and median oral mucositis score (B) in each treatment group after fractionated irradiation (X-rays n = 9, TM n = 6, BP n = 5, controls n = 14). The X-ray group received daily fractions on days 0–4 and 7–11, to a total dose of 65 Gy. The TM group received daily fractions on days 0–4 and 7–8, to a total dose of 45 Gy. The BP group received daily fractions on days 0–4 and 7, to a total dose of 41 Gy.
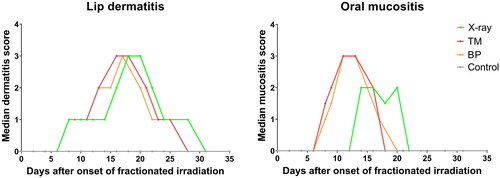
Mucositis of the tongue was observed in 100% of the mice exposed to both proton irradiation schemes, but only in 80% of the mice exposed to X-ray irradiation. The onset of mucositis was observed earlier for proton compared to X-ray-irradiated mice and the median severity score was higher (). In both proton groups, mucositis was observed on the ventral and dorsal surface of the tongue, while it was only observed on the ventral surface in the X-ray group (). Even though the severity of the mucositis was similar in BP and TM mice, the locations were different. In the BP group, mucositis was only observed on the right side of the dorsal surface of the tongue, while it was more consistent on the entire dorsal surface in the TM group () further confirming the dose distributions seen by alanine dosimetry.
Figure 4. Representative images of observed oral mucositis on the ventral and dorsal surface of the tongue after proton- and X-irradiation (dotted lines). a control (nonirradiated) mouse is included for comparison.
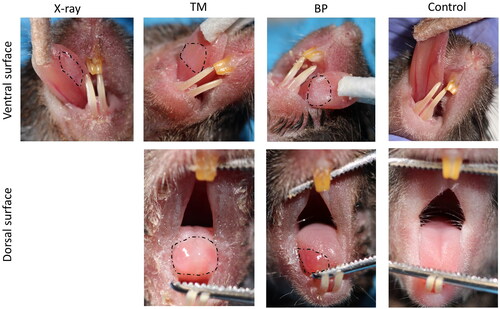
The saliva volume was significantly lower after X-ray and proton irradiation compared to controls right after termination of the radiotherapy (day 9 for BP and TM, day 12 for X-rays). However, at day 35 only the TM and X-ray group demonstrated significantly lower saliva volume compared to controls, while the BP group showed recovery of saliva production ().
Discussion
Normal tissue complications after radiotherapy are dose-limiting and may prevent a successful treatment. Tissue damage can contribute both to interruption of planned treatment and to reduced quality of life for the patient. Proton therapy is considered a tissue-sparing modality because less normal tissue is irradiated compared to conventional X-rays. However, protons deposit their energy with an increasing LET at the end of the Bragg peak. To adjust for this, a constant RBE of 1.1 is used in most proton centers in USA and Europe [Citation28]. However, data from in vitro and in vivo experiments demonstrate that the RBE increases along the proton beam track and that the average value may be higher than 1.1 [Citation14–16]. Protons with dissimilar energies and LET have been shown to induce different biological effects [Citation29], and more experiments to gain fundamental knowledge on biological effects in different tissues are needed to understand how RBE variations should be addressed in treatment planning.
In this study, we used two different proton beam delivery setups to study acute normal tissue responses. The BP group was irradiated with a pristine Bragg peak covering half of the mouse H&N region with the distal side of the Bragg peak located in the area of interest (oral cavity, salivary glands). This may clinically reflect irradiation of normal tissues being located behind the tumor. On the other hand, the TM group was irradiated with a more homogeneous dose distribution where the Bragg peak ended up outside the mouse. We designed this approach to create a proton plan with a similar dose distribution as X-rays to be able to directly compare their biological effectiveness. The depth dose distribution measured by alanine dosimetry showed very similar dose distributions for the TM and X-ray group. Surprisingly, the biological effects were very high for the TM group compared to the X-ray group. The fractionated treatment had to be terminated earlier than planned due to substantial weight loss in the BP and TM groups so that the total dose received was lower than that received by the X-ray group. The animals tolerated 1.5–1.6 times higher doses with X-rays compared to both proton groups. Therefore, for local H&N irradiation, protons were biologically more effective in causing acute tissue damage compared to X-rays. Moreover, the proton RBE for both treatment groups is likely higher than the clinical standard of 1.1.
In the present study, the saliva volume was significantly lower in all irradiated groups right after treatment termination (day 9/12) and on day 35, compared to controls. However, the BP group showed recovery of saliva production compared to the TM and X-ray groups on day 35. This may indicate a compensatory mechanism in the paired salivary glands that were outside the radiation field (as only half of the pair of glands were hit by the BP approach). Moreover, we observed a greater degree of oral mucositis after proton compared to X-ray irradiation. The oral mucositis mean score was about the same for TM and BP, but the extension on the tongue was different. The observed location and high score of oral mucositis on the dorsal surface of the tongue in BP mice indicates that there is an effect of high LET on normal tissue damage considering the much lower dose received by this area compared to the other irradiation groups. However, the low LET TM proton setup with a lower total dose compared to the X-ray setup also induced pronounced oral mucositis, which indicates that LET might not be the only decisive factor contributing to RBE in this case. Thus, for protons, it would seem that low irradiated volume and high LET (BP) give approximately the same mucositis as high volume and low LET (TM).
It is challenging to compare our results to clinical studies since the latter usually demonstrate a lower mean dose to the oral cavity after protons compared to X-rays as well as less irradiated volume [Citation18–20,Citation30–32]. Thus, in these clinical studies, the lower dose explained the reduced side effects, but the local RBE could still be higher due to differences in LET. No other preclinical data on oral mucositis after protons compared to X-rays have been published. However, Choi et al. studied acute normal tissue responses in the intestinal epithelium [Citation33], which resembles oral epithelium in many ways [Citation34]. They found that in mouse jejunum, protons induced a greater decrease in the number of crypts than X-rays, even when the same physical dose was used [Citation33]. Our study also demonstrates larger acute tissue responses in the oral mucosa after protons compared to X-rays. Surprisingly, we observed this difference in oral mucosal response even using lower total physical doses of protons than X-rays.
In contrast to the increased score of oral mucositis after proton irradiation, we found similar dermatitis scores after proton- and X-irradiation. Similar acute responses in the skin have previously been shown in mice after a single dose of proton or X-irradiation of the leg [Citation13,Citation35]. The observed difference in the acute response of oral mucosa and skin in the present study is, however, intriguing. Oral mucosa and skin are intrinsically different in regard to wound healing, where oral mucosa generally heals faster than skin [Citation36,Citation37]. Relative to X-irradiation, we observed an increase in oral mucositis compared to dermatitis following proton irradiation. This could indicate that the oral mucosa is affected not only by the type of radiation but also by the reduction in saliva production or changes in the saliva content. Differences in the degree of keratinization and proliferation rate between the two tissues can also contribute to the observed differences and need to be further elucidated.
Supplemental Material
Download PDF (978.5 KB)Disclosure statement
No potential conflict of interest was reported by the authors.
Data availability statement
The data that support the findings of this study are available from the corresponding author, EM, upon reasonable request.
Additional information
Funding
References
- Barazzuol L, Coppes RP, van Luijk P. Prevention and treatment of radiotherapy-induced side effects. Mol Oncol. 2020;14(7):1538–1554. doi: 10.1002/1878-0261.12750.
- Chibly AM, Aure MH, Patel VN, et al. Salivary gland function, development, and regeneration. Physiol Rev. 2022;102(3):1495–1552. doi: 10.1152/physrev.00015.2021.
- Siddiqui F, Movsas B. Management of radiation toxicity in head and neck cancers. Semin Radiat Oncol. 2017;27(4):340–349. doi: 10.1016/j.semradonc.2017.04.008.
- Tian X, Liu K, Hou Y, et al. The evolution of proton beam therapy: current and future status. Mol Clin Oncol. 2018;8(1):15–21. doi: 10.3892/mco.2017.1499.
- Fontana AO, Augsburger MA, Grosse N, et al. Differential DNA repair pathway choice in cancer cells after proton- and photon-irradiation. Radiother Oncol. 2015;116(3):374–380. doi: 10.1016/j.radonc.2015.08.014.
- Lupu-Plesu M, Claren A, Martial S, et al. Effects of proton versus photon irradiation on (lymph)angiogenic, inflammatory, proliferative and anti-tumor immune responses in head and neck squamous cell carcinoma. Oncogenesis. 2017;6(7):e354–e354. doi: 10.1038/oncsis.2017.56.
- Nielsen S, Bassler N, Grzanka L, et al. Differential gene expression in primary fibroblasts induced by proton and cobalt-60 beam irradiation. Acta Oncol. 2017;56(11):1406–1412. doi: 10.1080/0284186X.2017.1351623.
- Nielsen S, Bassler N, Grzanka L, et al. Comparison of coding transcriptomes in fibroblasts irradiated with low and high let proton beams and cobalt-60 photons. Int J Radiat Oncol Biol Phys. 2019;103(5):1203–1211. doi: 10.1016/j.ijrobp.2018.11.065.
- Vitti ET, Parsons JL. The radiobiological effects of proton beam therapy: impact on DNA damage and repair. Cancers (Basel). 2019;11(7):946. doi: 10.3390/cancers11070946.
- Paganetti H. Mechanisms and review of clinical evidence of variations in relative biological effectiveness in proton therapy. Int J Radiat Oncol Biol Phys. 2022;112(1):222–236. doi: 10.1016/j.ijrobp.2021.08.015.
- McNamara AL, Schuemann J, Paganetti H. A phenomenological relative biological effectiveness (RBE) model for proton therapy based on all published in vitro cell survival data. Phys Med Biol. 2015;60(21):8399–8416. doi: 10.1088/0031-9155/60/21/8399.
- Paganetti H. Relative biological effectiveness (RBE) values for proton beam therapy. Variations as a function of biological endpoint, dose, and linear energy transfer. Phys Med Biol. 2014;59(22):R419–72. doi: 10.1088/0031-9155/59/22/R419.
- Sorensen BS, Horsman MR, Alsner J, et al. Relative biological effectiveness of carbon ions for tumor control, acute skin damage and late radiation-induced fibrosis in a mouse model. Acta Oncol. 2015;54(9):1623–1630. doi: 10.3109/0284186X.2015.1069890.
- Sorensen BS, Bassler N, Nielsen S, et al. Relative biological effectiveness (RBE) and distal edge effects of proton radiation on early damage in vivo. Acta Oncol. 2017;56(11):1387–1391. doi: 10.1080/0284186X.2017.1351621.
- Sorensen BS, Pawelke J, Bauer J, et al. Does the uncertainty in relative biological effectiveness affect patient treatment in proton therapy? Radiother Oncol. 2021;163:177–184. doi: 10.1016/j.radonc.2021.08.016.
- Saager M, Peschke P, Brons S, et al. Determination of the proton RBE in the rat spinal cord: is there an increase towards the end of the spread-out Bragg peak? Radiother Oncol. 2018;128(1):115–120. doi: 10.1016/j.radonc.2018.03.002.
- Jones B. Why RBE must be a variable and not a constant in proton therapy. Br J Radiol. 2016;89(1063):20160116. doi: 10.1259/bjr.20160116.
- Meijer TWH, Scandurra D, Langendijk JA. Reduced radiation-induced toxicity by using proton therapy for the treatment of oropharyngeal cancer. Br J Radiol. 2020;93(1107):20190955. doi: 10.1259/bjr.20190955.
- Cao J, Zhang X, Jiang B, et al. Intensity-modulated proton therapy for oropharyngeal cancer reduces rates of late xerostomia. Radiother Oncol. 2021;160:32–39. doi: 10.1016/j.radonc.2021.03.036.
- Blanchard P, Garden AS, Gunn GB, et al. Intensity-modulated proton beam therapy (IMPT) versus intensity-modulated photon therapy (IMRT) for patients with oropharynx cancer - A case matched analysis. Radiother Oncol. 2016;120(1):48–55. doi: 10.1016/j.radonc.2016.05.022.
- Andreo P, Cunningham JR, Hohlfeld K, et al. Absorbed dose determination in photon and electron beams. An international Code of Practice. 1987.
- Niroomand‐Rad A, Chiu‐Tsao ST, Grams MP, et al. Report of AAPM task group 235 radiochromic film dosimetry: an update to TG‐55. Med Phys. 2020;47(12):5986–6025. doi: 10.1002/mp.14497.
- Mallick S, Benson R, Rath GK. Radiation induced oral mucositis: a review of current literature on prevention and management. Eur Arch Otorhinolaryngol. 2016;273(9):2285–2293. doi: 10.1007/s00405-015-3694-6.
- Sonis ST, Elting LS, Keefe D, et al. Perspectives on cancer therapy-induced mucosal injury: pathogenesis, measurement, epidemiology, and consequences for patients. Cancer. 2004;100(9 Suppl):1995–2025. doi: 10.1002/cncr.20162.
- Trotti A, Byhardt R, Stetz J, et al. Common toxicity criteria: version 2.0. an improved reference for grading the acute effects of cancer treatment: impact on radiotherapy. Int J Radiat Oncol Biol Phys. 2000;47(1):13–47. doi: 10.1016/s0360-3016(99)00559-3.
- Bagavant H, Trzeciak M, Papinska J, et al. A method for the measurement of salivary gland function in mice. J Vis Exp. 2018;(131):57203. doi: 10.3791/57203.
- Juvkam IS, Zlygosteva O, Arous D, et al. A preclinical model to investigate normal tissue damage following fractionated radiotherapy to the head and neck. J Radiat Res. 2023;64(1):44–52. doi: 10.1093/jrr/rrac066.
- Heuchel L, Hahn C, Pawelke J, et al. Clinical use and future requirements of relative biological effectiveness: survey among all european proton therapy centres. Radiother Oncol. 2022; 172:134–139. doi: 10.1016/j.radonc.2022.05.015.
- Tommasino F, Durante M. Proton radiobiology. Cancers (Basel). 2015;7(1):353–381. doi: 10.3390/cancers7010353.
- Holliday EB, Kocak-Uzel E, Feng L, et al. Dosimetric advantages of intensity-modulated proton therapy for oropharyngeal cancer compared with intensity-modulated radiation: a case-matched control analysis. Med Dosim. 2016;41(3):189–194. doi: 10.1016/j.meddos.2016.01.002.
- McDonald MW, Liu Y, Moore MG, et al. Acute toxicity in comprehensive head and neck radiation for nasopharynx and paranasal sinus cancers: cohort comparison of 3D conformal proton therapy and intensity modulated radiation therapy. Radiat Oncol. 2016;11(1):32. doi: 10.1186/s13014-016-0600-3.
- Romesser PB, Cahlon O, Scher E, et al. Proton beam radiation therapy results in significantly reduced toxicity compared with intensity-modulated radiation therapy for head and neck tumors that require ipsilateral radiation. Radiother Oncol. 2016;118(2):286–292. doi: 10.1016/j.radonc.2015.12.008.
- Choi C, Lee C, Shin SW, et al. Comparison of proton and photon beam irradiation in Radiation-Induced intestinal injury using a mouse model. Int J Mol Sci. 2019;20(8):1894. doi: 10.3390/ijms20081894.
- Suarez LJ, Arboleda S, Angelov N, et al. Oral versus gastrointestinal mucosal immune niches in homeostasis and allostasis. Front Immunol. 2021;12:705206. doi: 10.3389/fimmu.2021.705206.
- Nielsen S, Bassler N, Grzanka L, et al. Proton scanning and X-ray beam irradiation induce distinct regulation of inflammatory cytokines in a preclinical mouse model. Int J Radiat Biol. 2020;96(10):1238–1244. doi: 10.1080/09553002.2020.1807644.
- Turabelidze A, Guo S, Chung AY, et al. Intrinsic differences between oral and skin keratinocytes. PLoS One. 2014;9(9):e101480. doi: 10.1371/journal.pone.0101480.
- Waasdorp M, Krom BP, Bikker FJ, et al. The bigger picture: why oral mucosa heals better than skin. Biomolecules. 2021;11(8):1165. doi: 10.3390/biom11081165.