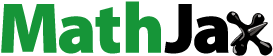
Abstract
Background
Inter-fractional anatomical changes challenge robust delivery of whole-pelvic proton therapy for high-risk prostate cancer. Pre-treatment robust evaluation (PRE) takes uncertainties in isocenter shifts and distal beam edge in treatment plans into account. Using weekly control computed tomography scans (cCTs), the aim of this study was to evaluate the PRE strategy by comparing to an off-line during-treatment robust evaluation (DRE) while also assessing plan robustness with respect to protocol planning constraints.
Material and methods
Treatment plans and cCTs from ten patients included in the pilot phase of the PROstate PROTON Trial 1 were analysed. Treatment planning followed protocol guidelines with 78 Gy to the primary clinical target volume (CTVp) and 56 Gy to the elective target (CTVe) in 39 fractions. Recalculations of the treatment plans were performed for a total of 64 cCTs and dose/volume measures corresponding to clinical constraints were evaluated for this DRE against the simulated scenario interval from the PRE.
Results
Of the 64 cCTs, 59 showed DRE CTVp measures within the robustness range from the PRE; this was also the case for 39 of the cCTs for the CTVe measures. However, DRE CTVe coverage was still within constraints for 57 of the 64 cCTs. DRE dose/volume measures for CTVp fulfilled target coverage constraints in 59 of 64 cCTs. All DRE measures for the rectum, bladder, and bowel were inside the PRE range in 63, 39, and 31 cCTs, respectively.
Conclusion
The PRE strategy predicted the DRE scenarios for CTVp and rectum. CTVe, bladder, and bowel showed more complex anatomical variations than simulated by the PRE isocenter shift. Both original and recalculated nominal treatment plans showed robust treatment delivery in terms of target coverage.
Background
The rapid distal dose fall off of a proton beam that makes this radiotherapy modality attractive for normal tissue sparing [Citation1–3] also makes it potentially sensitive to anatomical changes. For high-risk prostate cancer, both the targeted prostate and the surrounding organs at risk such as the rectum, bladder, and bowel are soft tissue structures that display large anatomical variation relative to the pelvic bones. In contrast, the pelvic lymph nodes are usually considered to be stationary relative to bony anatomy. Co-irradiation of the lymph nodes in high-risk prostate cancer patients [Citation4,Citation5] thus further enhances the need for motion management and detailed image-guidance strategies in order to achieve accurate dose delivery for these patients.
Robust treatment planning and delivery is a long-standing issue in radiotherapy [Citation6]. To accommodate the many sources of potential uncertainties [Citation7], robust optimisation is generally used for proton therapy. In clinical proton treatment planning, this includes distal edge uncertainty and simulated isocenter shifts to counter the potentially dose degrading effect [Citation8,Citation9]. Robust optimisation is complemented by a simulation of the final plan robustness in a process termed robust evaluation.
Pre-treatment robust evaluation (PRE) simulates the effects of daily setup uncertainties and distal edge uncertainty [Citation10] and, like in robust optimisation, involves mirroring of rigid anatomical changes through an isocenter shift with a focus on target volumes. It is then evaluated which effect such a shift has on the resulting treatment plan and a potential, realistic dose degradation is assessed.
Previous studies have investigated the target robustness of proton treatment plans both in an isolated setting and in comparison with photon plans for different treatment beam configurations and setups for prostate radiotherapy [Citation11–13]. To our knowledge, however, no previous study has assessed the robustness evaluation strategy itself using clinical data for this group of patients. It is uncertain whether the robust optimisation strategies used are properly taking into account complex target and normal tissue motion patterns – generally, and here specifically for the pelvis.
Hence, the aim of this study was to evaluate an off-line during-treatment robust evaluation (DRE) based on weekly control computed tomography (cCT) scans against the PRE. Further, the overall treatment robustness including target coverage and normal tissue sparing was also evaluated.
Material and methods
Clinical treatment plans and six to eight verification scans from the ten first, consecutive patients treated in the pilot phase of the randomised clinical trial, PROstate PROTON trial 1 (PRO-PROTON 1, NCT05350475) [Citation14], were included in this study. Details regarding treatment planning and image-guidance principles followed for the patients in this trial have previously been described [Citation15].
Treatment planning and image-guidance
The clinical target volume was divided into a high-dose and a low-dose target: The high-dose/primary clinical target volume (CTVp) included the prostate and involved seminal vesicles, while the low-dose/elective clinical target volume (CTVe) included the pelvic lymph nodes and the proximal 2 cm of un-involved seminal vesicles. CTVp and CTVe were delineated in all CT scans for all patients. For treatment planning, margins of 4 mm in the superior and inferior directions and 2 mm in all other directions were added to CTVp to form an internal target volume (ITV). The ITV margins compensates for internal organ motion to secure a robust target coverage. The ITV structure was modified to avoid overlap with the rectum and resulting dose constraint conflicts. Doses of 78 Gy and 56 Gy were prescribed to ITV and CTVe respectively using a simultaneous integrated boost technique.
Treatments were planned based on planning CT scans (pCT) as well as pre-treatment magnetic resonance imaging. Treatment plans were robust optimised with 5 mm isocentre shift and 3.5% distal edge uncertainty. An example of a clinically accepted, original treatment plan for one of the patients included in this study is shown in .
Figure 1. Original nominal treatment plan for one of the included patients. Transversal views at the level of the lymph nodes (top), seminal vesicles (Middle) and prostate (bottom). dose shown in color wash with a lower limit of 53.2 Gy and an upper limit of 81.5 Gy. Primary target outlined in red, elective target in light red, rectum in brown, bladder in yellow, bowel bag in green. Markers within the prostate volume in red, blue and green.

Cone-beam CT scans (CBCTs) were obtained for on-line image-guidance. The CBCT was matched to the pCT by first matching to the bony anatomy including both translations and rotations and afterwards performing a translational match to fiducial markers implanted in the patient’s prostate. For rotations above 1°, CBCT validation was required. Translational differences between bones and patient outline in CBCT and pCT images after the marker match were checked to be within 5 mm before starting the beam delivery in each fraction. All markers were checked to be within 3 mm from their position at the pCT allowing for small variations in marker positions. The patients were instructed to follow a drinking protocol, and before the delivery of each fraction, also volumes of the rectum and bladder were checked.
The included patients underwent repeated control CT (cCT) scanning every week. The cCTs were clinically assessed upon recording of each scan to evaluate treatment quality and robustness with respect to treatment planning constraints. The off-line matching of the pCT to the cCTs for this clinical evaluation mirrored the on-line clinical procedure. These registrations between pCTs and cCTs were used in the present study.
In the case of plan adaptation during the course of treatment, the treatment plan with the largest number of associated cCTs were included in this study. Treatment plans for three of the ten patients were adapted during the course of treatment as a result of a clinical evaluation during the course of treatment. None of the plans included in this study were subsequently adapted. A total of 64 cCTs were included.
Robust evaluation strategies
Robust evaluations were performed for all patients both before and during the course of treatment. The PRE was calculated for the nominal treatment plan and pCT with the most associated cCTs. The PRE also included 14 uncertainty scenarios with uncertainty parameters; 5 mm isocentre shift and 3.5% distal edge uncertainty. The DRE was based on recalculations of the original treatment plan on each of the repeated cCTs. For the DRE, the cCT constituted the variations that the isocentre shift uncertainty parameter simulated – moreover, distal edge uncertainty was included by the 3.5% uncertainty parameter.
The total 15 PRE scenarios; one nominal plan and 14 uncertainty scenarios, spanned an interval of treatment scenarios likely to occur during the course of treatment. The treatment plans were thus optimised to a point where the CTV scenarios within this interval constituted treatments that were clinically acceptable for a few treatment fractions. PRE scenarios for organs at risk were checked for the final nominal treatment plan but not used for treatment plan optimisation. shows the dose/volume histogram corresponding to the original nominal treatment plan in including the 14 PRE uncertainty scenarios for each volume of interest.
Figure 2. Dose/volume histogram for the original treatment plan for a patient including 14 pre-treatment robust evaluation scenarios for each volume of interest. Primary clinical target volume (CTVp) in red, elective clinical target volume (CTVe) in light red, rectum in brown, bladder in yellow, and bowel in green.

Comparison of during-treatment robust evaluation to pre-treatment robust evaluation
The comparison of PRE vs DRE was based on predefined dose/volume measures corresponding to treatment planning dose constraints (Supplementary Table A1). The percentage of the target structure volume receiving 95% of the prescribed dose (V95%) was determined from the treatment planning system for each CTV structure; CTVp and CTVe. For rectum, the percentages of the structure volume receiving 75 Gy, 70 Gy, 65 Gy, 50 Gy, 40 Gy, and 30 Gy (V75Gy, V70Gy, V65Gy, V50Gy, V40Gy, and V30Gy) were determined. Correspondingly, V80Gy, V70Gy, and V65Gy were assessed for bladder; V35Gy was assessed for bowel.
The three DRE scenarios for each cCT were compared to the interval spanned by the 14 PRE scenarios via the predefined dose/volume measures. If just one of the dose/volume measures for a structure for one of the DRE scenarios for a cCT was outside of the interval of PRE scenarios; that is lower for the target measures or higher for the organs at risk measures, the cCT was labelled as ’outside’ of the PRE interval. The CTVp DRE was compared to the ITV PRE since the ITV takes into account anatomical changes for the CTVp.
The whole course of treatment was analysed from a geometrical point of view to isolate the effects of anatomical and setup variations manifested by the cCTs and simulated by the isocenter shifts. Moreover, the three DRE scenarios with distal edge uncertainty of 0% and ±3.5% for each cCT were compared across cCTs for each patient to determine if distal edge uncertainty in one direction was systematically associated with a more detrimental effect on the treatment. This was investigated for both targets and the three organs at risk, the rectum, bladder and bowel.
Comparison of during-treatment robust evaluation to clinical constraints
A second comparison between the DRE and the clinical dose constraints was performed for this study. The dose constraints were the same as were used for treatment plan optimisation (Supplementary Table A1). Like for the PRE vs DRE comparison, a cCT was labelled as ’outside’ the constraint level if just one of the dose/volume measures for a structure for one of the DRE scenarios for a cCT violated the constraint.
Additionally, a time trend analysis was performed looking at one representative dose/volume measure for each volume of interest. The same endpoints were evaluated and results were collected across all patients.
Results
Comparing the CTVp DREs through the whole course of treatment to the ITV PRE, we found 59 of the total 64 cCTs to exhibit DRE scenarios within the PRE interval. This is illustrated by the patient case in where the ITV PRE corresponding to the CTVp DREs span an interval including all the DRE scenarios for all cCTs. With respect to the planning constraint, we found the CTVp dose/volume measure across all DRE scenarios for pCTs and cCTs to be within the constraint limit (V95% 98%) for 59 of the 64 cCTs. The five constraint events exhibited values above 97% in four of the cases and above 94% in the last case.
Figure 3. Figure of dose/volume measures for the Pre-treatment robust evaluation associated with the planning computed tomography scan (pCT) and the during-treatment robust evaluations associated with the weekly control computed tomography scans (cCTs) for one patient. The red dashed lines mark the constraint values corresponding to the dose/volume measures. A circular dot signifies a nominal treatment plan with no distal edge uncertainty, a cross means that the value corresponds to a scenario with distal edge uncertainty +3.5% and equivalently, a square signifies a −3.5% distal edge uncertainty scenario. Note that a DRE measure outside the PRE interval does not necessarily signify a constraint violation for the dose/volume measure and DRE scenario in question – or vice versa.

For CTVe, 39 of the total 64 cCTs had DRE scenarios within the PRE interval. However, DRE scenarios for 57 of the 64 cCTs showed dose/volume measures which complied with the constraint level. This is again exemplified by the patient case in which shows one of the DRE scenarios to be outside the PRE interval but still inside the constraint limit. The seven CTVe constraint events corresponded to dose volume measure values above 95% in five cases and of 93% and 89% in the remaining two cases. The two cases with CTVe V95% < 95% were observed for two different patients.
The rectum volume showed all DRE scenarios within the PRE interval in 63 of 64 cCTs. Considering the rectum dose/volume measures against the constraint level, 36 of the 64 cCTs had all DRE scenarios below the constraint. as well shows all DRE scenarios within the PRE interval but two cCTs with scenarios above the constraint. For the bladder and bowel, the situation was reversed: Across the whole cohort, the bladder DRE scenarios were within the PRE interval in 39 of the 64 cCTs, but within the constraints in 62 of the 64 cCTs. Both cCTs with bladder constraint violation belonged to the same patient. Evaluation of the bowel dose/volume measures showed 31 of the 64 cCTs to have DRE scenarios within the PRE interval. All 64 cCTs had all DRE scenarios below the constraint limits for the bowel constraint. The above results are summarised in .
Table 1. Results from the during-treatment robust evaluation (DRE) versus pre-treatment robust evaluation (PRE) analysis.
Considering anatomical variations and distal edge uncertainty as two independent contributions to changes in dose/volume measures, we found different impacts on different structure volumes. For the rectum, the variations in the DRE scenarios were dominated by anatomical variations. This is exemplified in by small variations between scenarios for the same cCT compared to variations between DRE scenarios from different cCTs. For the bladder, the distal edge uncertainty was relatively more prominent though anatomical variations still was the dominating effect for most patient treatment plans. The bowel dose/volume measures were influenced even more by the distal edge uncertainty and this contribution and the anatomical variations were comparable for this organ at risk. For the bowel, the distal edge uncertainty constituted a systematic effect where robustness scenarios with +3.5% distal edge uncertainty always showed lower dose/volume measures than their −3.5% counterparts.
Evaluating each of the dose/volume endpoints across all scans for all patients revealed no systematic time trends (illustrated in Supplementary Figures A3–A7).
Discussion
This study was performed in the context of an ongoing randomised clinical trial on proton therapy for high-risk prostate cancer patients which aims to reduce gastrointestinal toxicities while robustly treating the primary prostate target [Citation14]. In the present study we have therefore shown that the dose coverage of the primary target, CTVp, and the closest neighbouring organ at risk, the rectum, was robustly predicted by the PRE. On the other hand, the elective target, CTVe, and the organs at risk further from the pivotal point of the image-guidance; the bladder and bowel, exhibited variations more complex than predicted by the PRE.
Robust optimisation of treatment plans are often compared to the use of planning target volume margins [Citation8]. The planning target volume concept has been criticised [Citation16] but is still a widely accepted practice in photon radiotherapy and some of the same considerations regarding margin sizes apply to the ITV margin and robust optimisation and robust evaluation parameters. If the robust optimisation strategy is to apply also to deformable volumes in complex treatment locations; as CTVe, bladder, and bowel in the pelvis, this study points toward an adjustment of the parameters, or a sophistication of the approach, to accommodate the varying motion complexity of the anatomical structures.
Previous studies on robust optimisation and evaluation have generally focused on the trade-off between nominal treatment plan quality and robustness [Citation11,Citation12]. However, they did not evaluate if the treatment plan quality and robustness of the original treatment plan was carried through the whole treatment which is a key element in evaluating during-treatment plan robustness. Busch et al. proposed a range-guidance based robustness assessment to evaluate treatment robustness through the course of treatment [Citation13], but this approach is not as readily available as the strategies described in this study.
An inherent challenge with dose recalculations using cCTs is that these scans were not obtained in an actual treatment situation. One could argue, that the same is true for the pCT which the original treatment plan is based on but nonetheless, we observed some deviations between some of the cCTs and the same day CBCT that was obtained in the treatment situation. This constitutes a limitation to the present analysis and will therefore be the subject of future CBCT-based studies. In the present study, we directly assessed the effects of anatomical changes on relevant dose/volume endpoints. The future CBCT based study also provides the opportunity to relate these dose/volume measures to inter-fractional anatomical changes that can function as signals or triggers for treatment adaptations.
The deviations between cCTs and same-day CBCTs were observed already during the routine clinical assessment of the cCTs and recalculated treatment plans. The adaptation strategy involved evaluation of the recalculated treatment plan on the cCT. Moreover, the clinical evaluation of the match between positioning CBCT and pCT before delivery of each treatment fraction revealed if there were any systematic deviations in set-up or internal anatomy. This also contributed to the clinical assessment of when to adapt the treatment plan as well as the clinical status of the patient. This is why a CTVp constraint event did not necessarily catalyse a rescan and replan. In this evaluation it is also worth noting another reason that slight under-dosage of CTVe did not necessarily catalyse a rescan and replan. The evidence for co-irradiation of pelvic lymph nodes in high-risk prostate cancer has been interpreted differently in different recent trials [Citation5,Citation17] in terms of the prescribed dose to the lymph nodes. Results from the recent Mayo Clinic study [Citation17] shows clinical benefit for co-irradiation of pelvic lymph nodes with a lower dose than prescribed in the POP-RT trial [Citation5] which is equivalent to the dose prescribed in our trial [Citation14]. This justifies an adaptation strategy in our trial where we do not automatically adapt the treatment in case of slight CTVe under-dosage in a few fractions. It is also worth noting that systematic CTVe under-dosage was not observed for any of the patients.
An explanation of the impact of the distal edge uncertainty on the dose/volume measures of the different organs at risk can be found in the treatment beam configuration. As detailed in our previous paper [Citation15], two posterior oblique fields and two lateral oblique fields are used in our trial. None of these beams have their distal edges in the rectum, whereas the bowel follows the elective target in all its extent and receive distal edge contributions from the lateral oblique fields aimed at CTVe for every marginal overshoot. Hence, these results are depending on the chosen beam configuration.
In our results we showed anatomical variations that were not accurately described by rigid isocenter shifts from the PRE. With a total target covering the majority of the pelvis and organs at risk including several moveable and deformable soft tissue structures, however, this was expected. Nevertheless, rectum dose/volume measures were within the DRE scenarios predicted by the PRE interval with one single exception. This was due to the fact that the rectum neighbours the primary target and hence is positioned with close to the same precision as CTVp leading to mainly deviations within the 5 mm optimisation margin. It is also an indication of that our strategy for controlling rectum filling managed to limit deformation uncertainties for the rectum. As for the bladder and bowel, the original proton treatment plans were optimised to be well within the clinical constraints causing the bladder and bowel dose/volume measures to be within the constraints even if a DRE scenario measure was outside of the PRE interval. It is, however, still important to note what the goal of the PRE analysis is. As the main focus of the radiotherapy treatment for this patient group is primary target coverage, the PRE strategy proves useful. Further analysis of robust evaluation strategies for this patient group should in addition include CBCTs obtained in the treatment position for enhanced anatomical precision as well as dose accumulation for each volume of interest. The latter to assess the implications of treatment scenarios where the anatomical variations are more complex than simulated by the PRE.
The present study supports our trial in contributing to the accumulating evidence of proton therapy for high-risk prostate cancer. Several ongoing clinical trials on proton therapy for prostate cancer will add to the clinical evidence, though to our knowledge only our trial is a randomised, comparative study of protons versus photons for whole-pelvic irradiation of primary disease. The primary endpoint of our trial is a five point reduction in delta EPIC-26 bowel score [Citation18] – based on a minimally important difference study [Citation19]. We assume that this reduction will impact the clinical outcome for this patient group.
In conclusion, we found the PRE to reliably predict scenarios for the treatment of the primary target and the adjacent rectum volume. DRE of the elective target, bladder, and bowel showed that still some variations were not simulated by the PRE for these volumes and further investigation and DREs might be necessary to more precisely monitor elective target coverage and organs at risk sparing with the delivered treatment. In terms of treatment robustness, we found the CTVp and even CTVe to be covered robustly securing the primary goal of the treatment to be fulfilled.
Supplemental Material
Download Zip (1.5 MB)Disclosure statement
No potential conflict of interest was reported by the author(s).
Data availability statement
The participants of this study did not give written consent for their data to be shared publicly, so due to the sensitive nature of the research supporting data is not available.
Additional information
Funding
References
- Dowdell S, Metcalfe P, Morales J, et al. A comparison of proton therapy and IMRT treatment plans for prostate radiotherapy. Australas Phys Eng Sci Med. 2008;31(4):325–331. doi: 10.1007/BF03178602.
- Widesott L, Pierelli A, Fiorino C, et al. Helical tomotherapy vs. intensity-modulated proton therapy for whole pelvis irradiation in high-risk prostate cancer patients: dosimetric, normal tissue complication probability, and generalized equivalent uniform dose analysis. Int J Radiat Oncol Biol Phys. 2011;80(5):1589–1600. doi: 10.1016/j.ijrobp.2010.10.005.
- Schiller KC, Habl G, Combs SE. Protons, photons, and the prostate – is there emerging evidence in the ongoing discussion on particle therapy for the treatment of prostate cancer? Front Oncol. 2016;6:8. doi: 10.3389/fonc.2016.00008.
- Mottet N, van den Bergh R, Briers E, et al. EAU-EANM-ESTRO-ESUR-SIOG guidelines on prostate cancer-2020 update. Part 1: screening, diagnosis, and local treatment with curative intent. Eur Urol. 2021;79(2):243–262. doi: 10.1016/j.eururo.2020.09.042.
- Murthy V, Maitre P, Kannan S, et al. Prostate-only versus whole-pelvic radiation therapy in high-risk and very high-risk prostate cancer (POP-RT): outcomes from phase III randomized controlled trial. J Clin Oncol. 2021;39(11):1234–1242. doi: 10.1200/JCO.20.03282.
- Goitein M. Calculation of the uncertainty in the dose delivered during radiation therapy. Med Phys. 1985;12(5):608–612. doi: 10.1118/1.595762.
- Paganetti H. Range uncertainties in proton therapy and the role of Monte Carlo simulations. Phys Med Biol. 2012;57(11):R99–R117. doi: 10.1088/0031-9155/57/11/R99.
- Unkelbach J, Alber M, Bangert M, et al. Robust radiotherapy planning. Phys Med Biol. 2018;63(22):22TR02. doi: 10.1088/1361-6560/aae659.
- Butala AA, Ingram WS, O'Reilly SE, et al. Robust treatment planning in whole pelvis pencil beam scanning proton therapy for prostate cancer. Med Dosim. 2020;45(4):334–338. doi: 10.1016/j.meddos.2020.04.001.
- Killoran JH, Kooy HM, Gladstone DJ, et al. A numerical simulation of organ motion and daily setup uncertainties: implications for radiation therapy. Int J Radiat Oncol Biol Phys. 1997;37(1):213–221. doi: 10.1016/s0360-3016(96)00477-4.
- Ong ALK, Ang KW, Master Z, et al. Intensity-modulated radiotherapy for whole pelvis irradiation in prostate cancer: a dosimetric and plan robustness study between photons and protons. Tech Innov Patient Support Radiat Oncol. 2018;6:11–19. doi: 10.1016/j.tipsro.2018.02.001.
- Shan J, Sio T, Liu C, et al. A novel and individualized robust optimization method using normalized dose interval volume constraints (NDIVC) for intensity-modulated proton radiotherapy. Med Phys. 2019;46(1):382–393. doi: 10.1002/mp.13276.
- Busch K, Andersen A, Petersen J, et al. Towards range-guidance in proton therapy to detect organ motion-induced dose degradations. Biomed Phys Eng Express. 2022;8(2):025018. doi: 10.1088/2057-1976/ac5151.
- Lymph node radiation therapy with integrated boost to prostate for high-risk prostate cancer, a randomized phase 3 trial comparing photons vs. protons. 2022. [cited 2023 Feb 14]. https://clinicaltrials.gov/ct2/show/NCT05350475
- Tilbæk S, Muren LP, Vestergaard A, et al. Proton therapy planning and image-guidance strategies within a randomized controlled trial for high-risk prostate cancer. Clin Transl Radiat Oncol. 2023;41:100632. doi: 10.1016/j.ctro.2023.100632.
- Biston MC, Chiavassa S, Grégoire V, et al. Time of PTV is ending, robust optimization comes next. Cancer Radiother. 2020;24(6–7):676–686. doi: 10.1016/j.canrad.2020.06.016.
- Choo R, Hillman D, Mitchell C, et al. Late toxicity of moderately hypofractionated intensity modulated proton therapy treating the prostate and pelvic lymph nodes for high-risk prostate cancer. Int J Radiat Oncol Biol Phys. 2023;115(5):1085–1094. doi: 10.1016/j.ijrobp.2022.11.027.
- Szymanski K, Wei J, Dunn R, et al. Development and validation of an abbreviated version of the expanded prostate cancer index composite instrument for measuring health-related quality of life among prostate cancer survivors. Urology. 2010;76(5):1245–1250. doi: 10.1016/j.urology.2010.01.027.
- Skolarus T, Dunn R, Sanda M, et al. Minimally important difference for the expanded prostate cancer index composite short form. Urology. 2015;85(1):101–105. doi: 10.1016/j.urology.2014.08.044.