Abstract
Objectives: The ecosystem of the intestinal flora is always dynamic and it is maintained even in the changing chemical and physical environment of the host. The effects of the physical environment such as atmospheric pressure on the composition of intestinal flora has not been studied previously. Considering this, an in vitro experiment was designed to explore the effects of hyperbaromatric pressure on the faecal flora of the rat. Materials and methods: Faecal samples from male albino rat were exposed to graded hyperbaric conditions (122 and 170 kilopascal, kPa) for different time periods (1, 3 and 5 h). Total aerobes, total anaerobes, Escherichia coli, Bifidobacterium spp. and Clostridium perfringens present in the faecal samples were quantified by specific culture-based methods. Variation in their number in comparison to control conditions was analysed statistically (ANOVA and Tukey t test). Results: The numbers of total aerobes and E. coli were increased with the increase in air pressure, whereas a reduction in numbers was recorded for total anaerobes, Bifidobacterium spp. and C. perfringens. Variations of these groups of bacteria in relation to dose and duration of hyperbaric treatment were also recorded. Conclusion: Air pressure is an important exogenous factor that strongly regulates the composition of the faecal flora.
Introduction
The ecology of microbes in the intestine is fascinating. Numerous interactions between host and microorganism are continually occurring there in a complex fashion Citation[1], Citation[2]. Bacteria are the predominant inhabitants of the alimentary tract of mammals and they are designated as indigenous microflora or popularly known as normal flora Citation[3]. They perform several beneficial roles for the host, such as the breakdown of undigested food, enhanced absorption of foodstuffs, metabolism of drugs, synthesis of vitamins, creation of resistance against pathogenic bacteria by colonization resistance, induction of host immunity and stimulation of intestinal maturation Citation[4], Citation[5]. The gastrointestinal microecosystem is always fluctuating. This condition arises due to the high sensitivity of microflora to numerous host-induced physio-chemical and environmental factors such as antimicrobial agents, disorders of peristalsis, inflammatory bowel diseases, cancer, stress, redox potential, drugs, temperature and nutrients Citation[6], Citation[7]. The microflora is also highly sensitive to oxygen tension Citation[8], Citation[9] and that can be correlated with the atmospheric pressure.
During activities such as deep-sea diving, digging tunnels beneath a river or mining, individuals are exposed to hyperbaric atmosphere (an increase of 0.1 kilopascal (kPa) air pressure per 1 cm drop in sea level; with a sea level pressure of 101.3 kPa. This can create various kinds of health hazards Citation[6]. Among them, the major problems are indigestion and gas formation (flatulence). Although such problems are mainly associated with the alteration of the composition of gastrointestinal microflora Citation[4], there is still no detailed record that can correlate the variations in composition of indigenous microflora with atmospheric pressure.
The bacterial community of the gastrointestinal tract is not well understood owing to the inadequacy of classic culture-dependent methods. Recently many advanced techniques have been developed but no single technique could give an overall view of the total bacteria Citation[4]. In the present study, selective oxygen-sensitive culturable bacteria like total aerobes and anaerobes, and some indicator bacteria like Escherichia coli, Bifidobacterium spp. and Clostridium perfringens of the faecal matter (normally found in the gastrointestinal tract of the rat) were selected and their quantitative variation was studied by exposing them to graded hyperbaric pressure.
Materials and methods
Animals and diet
Healthy male albino rats with an average body weight of 115±7 g were used in the present study. They were housed in metal cages (34×28×19 cm). All animals had access to boiled rat feed (carbohydrates, 74.05%; proteins, 10.38%; fibre, 2.20%; iron, 56 ppm; calcium, 400 ppm and sodium, 500 ppm) and water ad libitum. The animals were maintained without interrupting their normal activity.
Sample collection and treatment
Rat faeces were collected just after dropping onto clean paper underlying the cage. Fresh faecal sample was suspended in sterilized phosphate-buffered saline (PBS; pH 7.0 and 9 g l−1 NaCl) using a manual glass homogenizer for 5 min. The faecal homogenates were exposed to two different simulated hyperbaromatric pressures (122 and 170 kPa, these are 1.2 and 1.7 times higher than normal atmospheric pressure, respectively) for different time periods (1, 3 and 5 h). The suspension was then centrifuged (1000 g for 5 min) and clear supernatant was used for microbial analysis.
Analytical measurements
The total aerobic and anaerobic faecal bacteria were enumerated by standard pour-plate technique in single-strength trypticase soya agar (TSA, Himedia, India) and reduced Wilkins Chalgren agar (WCA, Micromaster, India), respectively. An anaerobic jar was employed for anaerobic culture, from which oxygen was removed catalytically and replaced with 10% of both CO2 and H2 gas (Micromaster, Mumbai). Enumeration of E. coli and Bifidobacterium spp. was carried out using selective media – MacConkey and bifidobacterium agar (Himedia), respectively. Reduced perfringens agar base (Himedia) was used for selective enumeration of C. perfringens.
Statistical analysis
Collected data are presented as the arithmetic mean of three replicas (mean±SE). The variations in microbial count were examined by one-way ANOVA and the multiple comparisons of all possible pairs were done by Tukey t test (SPSS-10.0). The alteration in bacterial quantity at different air pressures (122 and 170 kPa) for each specific time period (1, 3 and 5 h) was tested by Fisher's t test. Significant variation was accepted at the level of 5%, i.e. p<0.05.
Results
The effect of graded hyperbaric pressures (122 and 170 kPa) for different durations (1, 3 and 5 h) on the faecal flora was evaluated and is presented in . In control conditions (normobaric), rat faeces (per gram) contained total aerobes 1.9×106, total anaerobes 3.7×1011, E. coli 9.5×105, Bifidobacterium spp. 4.0×104 and C. perfringens 3.7×105.
Table I. Quantification of gastrointestinal flora after hyperbaric treatment for various time periods.
When the faecal matter was subjected to higher atmospheric pressure of 122 and 170 kPa for 1, 3 and 5 h, the quantity of total aerobic bacteria was increased up to 10-fold (3.2×107) polynomially (R2=0.9389), step by step (−0.05-fold, 0.25-fold and 0.42-fold); and about 100-fold (1.4×108) exponentially (R2=0.9728), step by step (3.74-fold, 9.0-fold and 72.68-fold) ( and ). The alterations in aerobic bacteria at both the atmospheric pressures were statistically significant (p<0.05). Increasing the pressure by about 40% favoured the bacterial population to multiply by 3.74, 7.44 and 3.38 times at the incubation times of 1, 3 and 5 h, respectively (t1h=426.34, p<0.05; t3h=981.46, p <0.05 and t5h=29090.35, p<0.05).
Figure 1. Comparative study of population size of total aerobes with time at 122 and 170 kPa. , regression coefficient (122 kPa-time);
, regression coefficient (170 kPa-time); *step-wise; –, reduction in population size; +, increase in population size; #, time-specific, ⊺, standard error of mean.
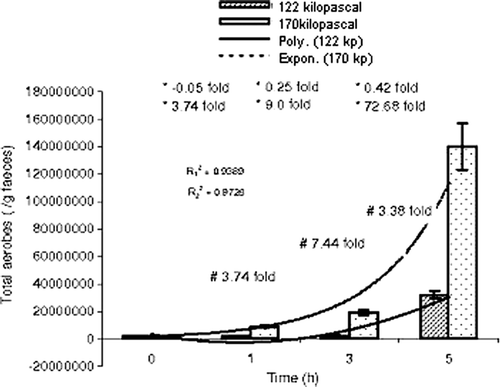
Interestingly, the count of E. coli mounted gradually and reached the level of the total aerobic population polynomially (=0.9794 and
=0.945) after graded hyperbaric treatment ( and ).
Figure 2. Comparative study of population size of E. coli with time at 122 and 170 kPa. , regression coefficient (122 kPa-time);
, regression coefficient (170 kPa-time); *step-wise; –, reduction in population size; +, increase in population size; #, time-specific; ⊺, standard error of mean.
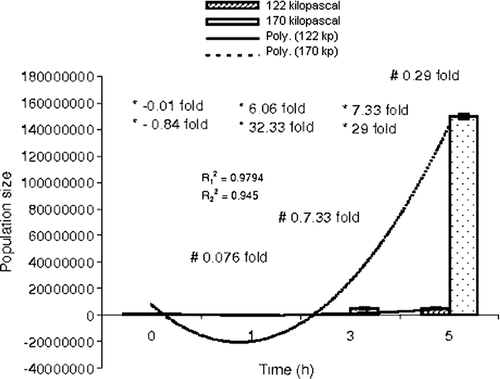
In rat faeces, total anaerobes, Bifidobacterium spp. and C. perfringens were normally present at a ratio of 1:1.08×10−7:1×10−6 (). The quantity of total anaerobes was reduced by 105- and 107-fold at 122 and 170 kPa pressure, respectively, in comparison with their normal counts (p<0.05 and p<0.05, respectively) (). In addition, the count of anaerobes also declined at above atmospheric pressure (120–170 kPa) for the three time periods tested by 57.14%, 89.09% and 99.41%, respectively (t1h=54632.283, p<0.05; t3h=786.64, p<0.05 and t5h=292.09, p<0.05) (). The counts of Bifidobacterium spp. and C. perfringens were abridged up to 102-fold and 103-fold, respectively (p<0.05 and p<0.05), at both the tested pressures (). On increasing the pressure from 122 kPa and 170 kPa, the population of Bifidobacterium spp. declined by 24.4-, 5.11- and 2.73-fold at 1, 3 and 5 h, respectively (t1h=101.17, p<0.05; t3h=367.64, p<0.05 and t5h=63.48, p<0.05) (), but a drastic reduction in number was observed in C. perfringens (10.91%, t1h=120.88, p<0.05; 98.46%, t3h=264.97, p<0.05 and 100%, t5h=52.08, p<0.05) ().
Figure 3. Comparative study of population size of total anaerobes with time at 122 and 170 kPa. , regression coefficient (122 kPa-time);
, regression coefficient (170 kPa-time); *step-wise; –, reduction in population size; +, increase in population size; #, time-specific; ⊺, standard error of mean.
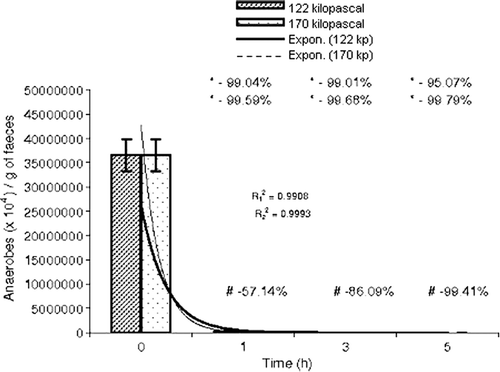
Figure 4. Comparative study of population size of Bifidobacterium spp. with time at 122 and 170 kPa. , regression coefficient (122 kPa-time);
, regression coefficient (170 kPa-time); *step-wise; –, reduction in population size; +, increase in population size; #, time-specific; ⊺, standard error of mean.
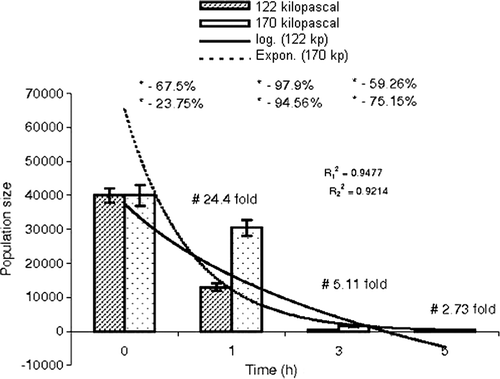
Figure 5. Comparative study of population size of Clostridium perfringens with time at 122 and 170 kPa. , regression coefficient (122 kPa-time);
, regression coefficient (170 kPa-time); *step-wise; –, reduction in population size; +, increase in population size; #, time-specific; ⊺, standard error of mean.
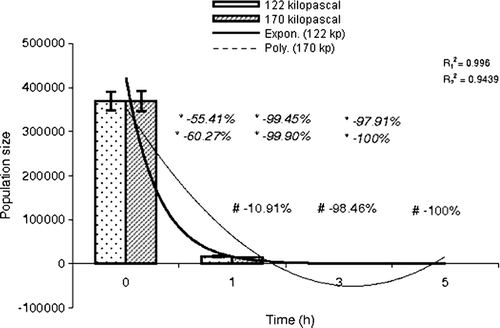
Discussion
Microbial flora present in the microenvironment of the gastrointestinal tract performs several important and essential activities of the host Citation[10]. Diverse types of bacteria are associated in this ecosystem and among them a few have been well characterized Citation[11]. Their homeostasis can be disrupted by a wide variety of indigenous and exogenous factors Citation[1]. The effects of graded hyperbaric pressures on the microbes present in the faecal matter were examined. Two hyperbaric pressures, i.e. 122 and 170 kPa (equivalent to depths of 2.07 m and 6.87 m, respectively, from sea level) were chosen in this study, as humans generally encounter such air pressures during sea-diving and cleaning of underground municipal drainage, wells, tunnels, etc.
In the faeces of rat (normobaric), the aerobic, facultative (E. coli) and anaerobic bacteria were present in a ratio of 2:1:3.89×105. This ratio varies from species to species Citation[12]. Abundance of anaerobic bacteria in faeces is common in all animals due to their expanded large intestine. It has been found that the population of facultative bacteria is half of the total aerobes, whereas Samanta et al. Citation[4] reported that the quantity of E. coli in rat faeces is approximately one-third of the total aerobic microflora.
Faecal matter exposed to graded hyperbaric pressure for different time periods showed an increased number of total aerobes and E. coli. Normally bacteria are very sensitive to atmospheric oxygen and the limit of oxygen tolerance varies from species to species Citation[13], Citation[14]. In this experiment the reasons for elevation of aerobes and facultative bacteria at high atmospheric pressure is not clear but it may be due to accelerated oxidation of cellular metabolites and over-activation of different oxygen-sensitive rate-limiting enzymes of growth-related metabolism in the presence of high levels of molecular oxygen Citation[15]. Our observations are in agreement with the study of Gillmore et al. Citation[16].
The anaerobic bacteria are the predominant inhabitants of the lower intestine; they have several beneficial effects and also maintain the normal health of the colon Citation[3]. In recent years, bifidobacteria and C. perfringens have been of great interest because of their nutritional and health impacts Citation[17–19]. In the present study, the quantity of the anaerobic group of organisms was drastically reduced at graded simulated air pressure in a duration-dependent manner (). In excess oxygenation most of the anaerobic groups of bacteria were eradicated and this may be due to the production of reactive oxygen species (ROS). Normally this group of bacteria creates an anaerobiosis by reducing the action potential of its surroundings Citation[20]. In hyperbaric conditions, alteration of the potential creates an unfavourable environment for their survival.
Conclusion
It is clear from the study results that atmospheric pressure above the ambient level alters the composition of faecal flora. Although the selected groups of bacteria are very limited members of the overall microbial population in the gastrointestinal tract, the observed results indicate that atmospheric pressure has a significant impact on the colonization of such a group of microflora.
Acknowledgements
The authors are thankful to the Director, DIPAS, DRDO, Government of India for financial assistance [112(KCM)/DIPAS/06]. Declaration of interest: The authors report no conflicts of interest. The authors alone are responsible for the content and writing of the paper.
References
- Eckburg PB, Bik EM, Bernstein CN, Purdom E, Dethlefsen L, Sargent M, et al. Diversity of the human intestinal microbial flora. Science 2005; 308: 1635–8
- Hooper LV, Midtvedt T, Gordon JI. How host-microbial interactions shape the nutrient environment of the mammalian intestine. Annu Rev Nutr 2002; 22: 283–307
- Ito H. Are intestinal microbes our friend or foe?. J Gastroenterol 2005; 40: 111–12
- Samanta S, Giri S, Parua S, Nandi DK, Pati BR, Mondal KC. Impact of tannic acid on the gastrointestinal microflora. Microb Ecol Health Dis 2004; 16: 32–4
- Mitsuhara I, Nakajima Y, Natori S, Mitsuoka T, Ohashi Y. In vitro growth inhibition of human intestinal bacteria by sarcotoxin IA, an insect bactericidal peptide. Biotechnol Lett 2001; 23: 569–73
- Heavey PM, Rowland IR. The gut microflora of the developing infant: microbiology and metabolism. Microb Ecol Health Dis 1999; 11: 75–83
- Kleessen B, Bezirtzoglou E, Matto J. Culture-based knowledge on biodiversity, development and stability of human gastrointestinal microflora. Microb Ecol Health Dis 2000; 2: 53–63
- Kaye D. Effect of hyperbaric oxygen on Clostridia in vitro and in vivo. Proc Soc Exp Biol Med 1967; 124: 360–6
- Loesche WJ. Oxygen sensitivity of various anaerobic bacteria. Appl Microbiol 1969; 18: 723–7
- Straw TE. Bacteria of the rabbit gut and their role in the health of the rabbit. J Appl Rabbit Res 1989; 11: 142–50
- Guarner F, Malagelada JR. Gut flora in health and disease. Lancet 2003; 361: 512–19
- Kok RG, de Waal A, Schut F, Welling GW, Weenk G, Hellingwerf KJ. Specific detection and analysis of a probiotic Bifidobacterium strain in infant faeces. Appl Environ Microbiol 1996; 62: 3668–72
- Dewey DL, Boag JW. Modification of the oxygen effect when bacteria are given large pulses of radiation. Nature 1959; 183: 1450–1
- Tally FP, Stewart PR, Sutter VL, Rosenblatt JE. Oxygen tolerance of fresh clinical anaerobic bacteria. J Clin Microbiol 1975; 1: 161–4
- Schwarz R, Yayanos A, Colwell R. Metabolic activities of the intestinal microflora of a deep-sea invertebrate. Appl Environ Microbiol 1976; 31: 46–8
- Gillmore JD, Gordon FB. Effect of exposure to hyperoxic, hypobaric, and hyperbaric environments on concentrations of selected aerobic and anaerobic fecal flora of mice. Appl Microbiol 1975; 29: 358–67
- Mitsuoka T. The human gastrointestinal tract. The lactic acid bacteria, vol. 1. The lactic acid bacteria in health and disease, BJB Wood. Elsevier Applied Science, London 1992
- Ohno H, Tsunemine S, Isa Y, Shimakawa M, Yamamura H. Oral administration of Bifidobacterium bifidum g9-1 suppresses total and antigen specific immunoglobulin E production in mice. Biol Pharm Bull 2005; 28: 1462–6
- Parish WE. Necrotic enteritis in the fowl. II. Examination of the causal Clostridium welchii. J Comp Pathol 1961; 71: 394–404
- Rood JI, Cole ST. Molecular genetics and pathogenesis of Clostridium perfringens. Microbiol Rev 1991; 55: 621–48