Abstract
Oyster reef, a key ecosystem component in many temperate and subtropical estuarine systems, has been greatly diminished by human influences and ongoing climatic changes. Changes in precipitation, oyster adult and larval distribution, benthic composition, hydrology, currents and/or inputs to the system such as pollutants or sediments complicate the identification of locations that may be suitable for oyster reef restoration. In such cases, development of a habitat suitability index model (HSI) can provide useful guidance on where restoration activities might be successful, and once complete, help to focus the area for restoration project siting consideration. HSI models can be constructed from a variety of data categories including biological requirements of the species to be restored, physical characteristics of the system, regulatory constraints, and socio-economic concerns. Here we describe the development of a geographic information system based HSI model to inform restoration of oyster reef in the Pensacola Bay System, Florida, USA that can be used as a template for oyster reef restoration in other Florida estuaries and beyond. We demonstrate that an oyster habitat HSI model can be relatively simple to construct, useful even with limited environmental data, improved with community stakeholder and resource user input, and easily adaptable.
Introduction
Many estuarine ecosystems have been substantially compromised by anthropogenic influences resulting in the loss of important ecosystem components as in the case with biogenic reef structures formed by the eastern oyster, Crassostrea virginica. Eastern oyster reefs were historically present in estuarine waters across the Western Atlantic from Canada to the Gulf of Mexico and south to Argentina. The reef structures formed by this species have been pushed toward functional extinction in most areas where they occur in United States waters including the Gulf of Mexico (GOM; Beck et al. Citation2011; Zu Ermgassen et al. Citation2012). Like many reef building oysters, C. virginica, provides many valuable ecosystem services including food, habitat for fish and invertebrates, shoreline protection (Coen, Luckenbach and Breitburg Citation1999; LaPeyre et al. Citation2014), water filtration (Grizzle, Greene, and Coen Citation2008), and nutrient cycling (Piehler and Smyth Citation2011; Beseres Pollack et al. Citation2013; Humphries et al. Citation2016; Lai, Irwin, and Zhang Citation2020a) in addition to supporting oyster related businesses and employment in fisheries, recreation, and tourism (Millennium Ecosystem Assessment Citation2005; Grabowski et al. Citation2012). The loss of oyster reef and the services it provides has a substantial adverse effect on the surrounding human communities that rely on and benefit from these services. Several studies have estimated the value of ecosystem services associated with natural and restored oyster reef (Grabowski et al. Citation2012; Kroeger Citation2012; Kroeger and Guannel Citation2014; Callihan et al. Citation2016; Lai, Irwin, and Zhang Citation2020b). Grabowski et al. (Citation2012) estimated an average annual net benefit of $10,325 per ha of reef for non-harvest benefits whereas Kroeger and Guannel (Citation2014) estimated annual net benefit from enhanced fisheries alone at two restored oyster reefs at $35,316 to $37,679 per ha in the Mobile Bay region of the GOM. Oyster reef losses have been attributed to and exacerbated by several anthropogenic issues including lack of sustainable management policies that address the needs of the habitat and fishery, unsustainable harvest, the mining of shell for construction fill, changes in water flow, poor water quality, siltation, disease and predation, sediment contamination and erosion from boat wakes (Grizzle, Adams, and Walters Citation2002) and storm events (USEPA (US Environmental Protection Agency) Citation2004, Seavey et al. Citation2011).
C. virginica reef (hereafter “oyster reef”), was historically a prominent feature in many Florida, USA estuaries. An account of Florida’s west coast estuaries from the late 1800s notes extensive and pervasive oyster reef once present in the region’s estuarine waters and how they were mostly gone within a 25-year period by the turn of the century (Smeltz Citation1898 as quoted in CHNEP 2012). One such estuary with extensive reduction of oyster reef is the 338 km2 Pensacola Bay System (PBS; ). The PBS is comprised of six main estuaries - Escambia, Pensacola, Blackwater, and East bays, Big Lagoon and Santa Rosa Sound - which are fed by the Escambia, Blackwater, East and Yellow rivers. The average depth of the system is 2.25 m in the northern portion and 6 m in the southern portion (USEPA (US Environmental Protection Agency) Citation2004). Significant tidal exchange occurs with both Big Lagoon and Santa Rosa Sound/Choctawhatchee Bay, and there is a connection to Choctawhatchee Bay to the east. The entire micro-tidal, low energy, river dominated system discharges primarily through a single narrow pass at the mouth of the PBS into the Gulf of Mexico (Northwest Florida Water Management District 2017). Average tidal range in the system is 0.5 m and, based on average river flow and tidal range, flushing time has been estimated at 21-34 days (Olinger et al. Citation1975). A nineteenth century map of the PBS depicts approximately 15,000 ha of oyster reef (USFC (US Fish Commission)) Citation1883; ) whereas a recent assessment of oyster habitat in Escambia, East and Blackwater bays (where most historic oyster reef was present) found only 91.5 ha (226.1 acres) of oyster reef and 30.2 ha (74.6 acres) of exposed shell (a substrate that can support live oysters) (Johnson Citation2021a Citation2021b). This approximates the extent of oyster reef reported in a US Environmental Protection Agency assessment of the environmental quality of the PBS (Lewis, Kirschenfeld, and Goodheart Citation2016).
Figure 1. The Pensacola Bay System (PBS; arrow on the inset map) as described for the HSI analysis. The study area is the PBS as designated by the area North of the thick black line on the main figure at the mouth of the system.
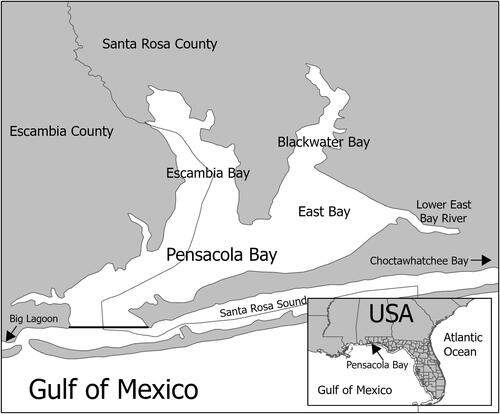
Figure 2. Detail from PBS from map dated 1883, United States Fish Commission. Mid-water clumps represent natural beds (i.e., oyster reef) and shaded areas indicate scattered natural beds.
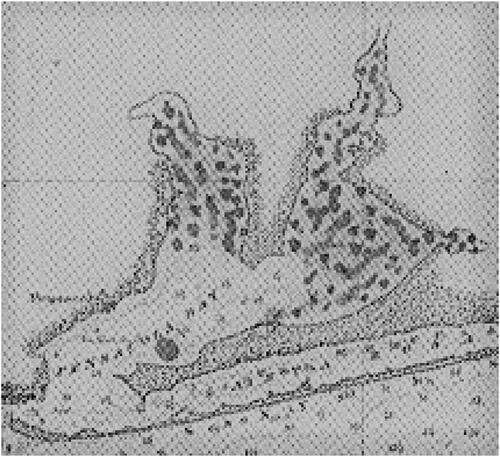
Figure 3. Mapped factors as scored for the HSI including the two informational factors (aquaculture and shellfish leases, and coastal maintained channels). These maps illustrate the scored factors prior to transforming into raster format.
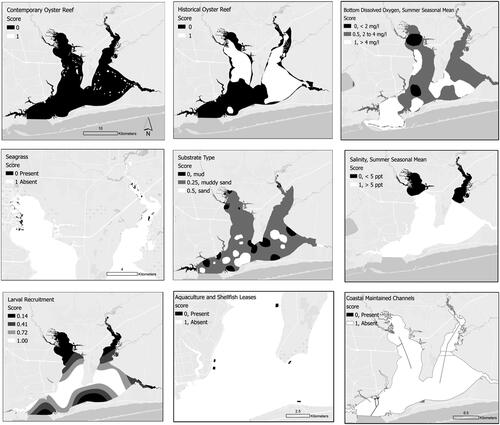
Given the magnitude of loss, human intervention is now recognized as necessary to restore this ecosystem in the PBS to recover lost ecosystem services (PPBEP (Pensacola & Perdido Bays Estuary Program) Citation2022). Restoring oyster reef ecosystems to large estuarine areas such as the PBS is a challenging and expensive task. It is important to assess historic changes to the estuarine system under consideration as well as current conditions to ascertain whether and where restoration may be possible. However, in many systems such as the PBS, studies characterizing the current condition of oyster reef and oyster populations as well as physical characteristics of the water column and bottom type are unavailable, dated or are lacking in resolution. To address the challenges of restoring oyster reef and its provided services to the PBS, a broad-based stakeholder engagement effort was initiated by The Nature Conservancy in the region in 2019. The objectives of the effort were to improve conditions for the commercial oyster harvest and increase restoration of oyster reef while also addressing the needs of the oyster aquaculture industry. Many state and local stakeholders participated in this effort as members of a Stakeholder Working Group (SWG), which included 29 local oyster fishers, oyster aquaculture farmers, academics, scientists, environmental, business, and community staff, and state and local government representatives. The outcome of this effort was development of the Oyster Fisheries and Habitat Management Plan for the Pensacola Bay System (PBS Oyster Plan; Birch et al. Citation2021). As part of plan development, which was completed in 2021, the SWG recognized the difficulty in identifying priority oyster reef restoration areas in the PBS and determined that spatially explicit information would facilitate identifying where best to pursue restoration activities for the fishery and for recovering ecosystem services. This recognition led to the development of the Oyster Reef Habitat Suitability Index Model (HSI) for the PBS.
Habitat suitability indices were first developed by the US Fish and Wildlife Service (USFWS) (Schamberger and Farmer Citation1978; USFWS Citation1981) and can be used as decision support tools to assist with the identification of areas to focus restoration efforts (Beseres Pollack et al. Citation2012; Puckett et al. Citation2018; Chowdhury et al. Citation2019; Smith et al. Citation2022; Theuerkauf and Lipcius Citation2016). HSIs have been extensively used for the eastern oyster throughout the US including estuaries in the Gulf of Mexico (La Peyre, Marshall, and Sable Citation2021). HSIs integrate spatial information important to the survival of a species or habitat under consideration, including biological, chemical, and/or physical characteristics. Socio-economic and regulatory information relevant to the cost, benefits and/or expediency of the restoration may also be included in an HSI (Puckett et al. Citation2018). While HSIs are often used to predict species presence, they can also be used to identify areas where restoration activities are likely to have the greatest success (Beseres Pollack et al. Citation2013, Puckett et al. Citation2018, Theuerkauf, Eggleston, and Puckett Citation2019). Factors to be included in an HSI are selected based on the specific purpose of restoration (e.g., harvest, oyster aquaculture, sportfishing, ecosystem restoration and/or water quality), as well as data relevancy, availability, and quality. While some HSIs have included larval oyster densities and movements, a recent meta-analysis and previous studies examining metapopulation dynamics recommend increased development and use of larval suitability and input/output components to better understand reef connectivity (Lipcius et al. Citation2015, La Peyre, Marshall, and Sable,Citation2021 Powers et al. Citation2023). Typically, HSIs combine the factors selected for inclusion into a ranking system with scores ranging from 0 to 1, with the highest values being most suitable for restoration (Cake Citation1983; Soniat and Brody Citation1988).
The objectives of this study were to (1) describe development of an estuary-specific decision support tool that streamlines the selection of oyster reef restoration sites to areas where they are most likely to persist even in systems with limited high-resolution data, (2) demonstrate the value of engaging oyster resource knowledgeable stakeholders and resource users in the HSI development process, and (3) illustrate how an HSI can be adapted as additional or higher resolution data become available. The purpose of this study is to facilitate and expedite the large-scale, estuary-specific oyster reef restoration that is currently underway to return valuable ecosystem services and oyster harvesting opportunities to the PBS. We hypothesize that applying estuary specific and adaptable HSIs will streamline decisions regarding distribution of finite restoration funds to priority locations, prevent years of misguided work, and capitalize on remaining larval oyster sources in estuaries where reefs with live oysters have been greatly diminished.
Materials and methods
The PBS, excluding the Big Lagoon and Santa Rosa Sound, () comprises 338 km2 of potential oyster habitat. Although some early documentation suggests oysters may have once been present in these two excluded systems (USFC (US Fish Commission) Citation1883), our review of the literature revealed no documentation of oyster reef presence in recent decades. We chose an estuary-wide approach to evaluate locations for oyster growth, survival, and recruitment based on the present knowledge of the system and availability of spatial data. A GIS-based HSI was selected as a suitable approach for developing a PBS-wide decision support tool. The HSI developed for the PBS is similar to others developed for oysters (e.g., Barnes et al. Citation2007; Puckett et al. Citation2018; Theuerkauf, Eggleston, and Puckett Citation2019) and other living marine resources (Vinagre et al. Citation2006). As part of the PBS Oyster Plan development, existing biophysical, chemical and management data on PBS and oysters/oyster fishing were collated along with data important for oyster reef restoration and survival. Potential factors were then evaluated for 1) availability, quality, and resolution of the spatial data, 2) relevance of the biological, chemical, and physical factors that indicate areas with successful oyster recruitment, survival, and reef formation without harming other productive coastal ecosystems, and 3) whether the factors represented preexisting human uses that would conflict with oyster reef restoration.
Factors selected for inclusion in the HSI and their scoring
C. virginica reefs have been studied extensively which simplified the task of selecting the most important biological, chemical and physical parameters to include in the HSI. A challenge was finding parameter datasets that were available, relevant to the viability of the oyster reef ecosystem or of regulatory concern, and were of a sufficient quality and spatial resolution. The PBS, like many estuarine systems, is dynamic spatially and seasonally depending on several climatic, hydrologic and geomorphic factors, most notably precipitation in the watershed, winds and basin morphology, and hydrologic flow patterns into the system (Hagy and Murrell,Citation2007 Lewis,Citation2010 Livingston Citation2015). Twenty-two factors were identified as potentially important to the viability of oyster reef: aquaculture and shellfish leases, bathymetry, summer mean bottom dissolved oxygen (DO), chlorophyll a concentrations, coastal maintained channels, contemporary oyster reef (1995 – 2010; VanderKooy Citation2012), disease intensity and prevalence, historical oyster reef presence (USFC (US Fish Commission)), Citation1883), hydrology, oyster recruitment, managed areas, marsh migration areas, military zones, predation, regulatory restrictions, summer mean salinity, seagrass presence, sea level rise, shoreline type, substrate type, temperature, water quality (i.e., pollutants) (). From these 22 factors seven were selected as being important indicators of oyster recruitment and growth or represented a regulatory/permitting issue, and had sufficient, relatively recent spatial data available: contemporary oyster reef, summer mean bottom DO, historical oyster reef, oyster recruitment (as measured from oyster recruitment arrays placed around the PBS system in 2007 and 2008, Arnold et al. Citation2017), salinity, seagrass presence, and substrate type (). The rationales for including the selected factors can be found in associated supplemental information.
Table 1. The 22 biological, physical, chemical and management factors considered for HSI development. References for the datasets used in HSI scoring are provided for the factors included in the HSI whether as ranked factors or for informational purposes only.
Table 2. The seven biological, physical and chemical factors selected for inclusion in the HSI plus the two preexisting human use avoidance factors. The data scale and scoring methods are provided for the scored factors.
The seven factors selected for the HSI were scored from zero to one with zero representing unacceptable conditions and one representing optimal conditions for the factor in the PBS. Scoring was based on known tolerances and growing conditions of C. virginica based on literature review as described for each scored factor (). The spatial results of the scoring for each factor is illustrated in . In some cases, the factor scores did not encompass the entire range of values from zero to one, e.g. in the sediment type dataset as there were no occurrences of the optimal sediment/substrate type for oyster reef formation, i.e., hard bottom. Hardbottom is, however, captured by the included factor contemporary oyster reef. Each spatially explicit factor scored as identified in was transformed into a raster spanning the PBS project area (338 km2). Resolution of the data varied according to the data source (see ). Raster size (approximately 124 m2) was determined as fine enough for a range of stakeholder activities while streamlining computation time during the development of the model. The included factor, recruitment, required additional processing prior to converting into a raster. Oyster recruits per shell per day (RPS) over the June 2007 to July 2008 study period were obtained from Arnold et al. (Citation2017). Using these data, the mean RPS over the study period (June 2007 – July 2008) was calculated for each of the 18 sampled sites within the PBS. Next using GIS, a regularized spline interpolation routine was applied to estimate oyster recruitment distribution for all areas of the PBS, which included ‘barriers’ to prevent interpolating recruitment data across land masses. The resulting output was a smooth gradient of estimated oyster recruitment for the entire PBS that was then standardized to between 0.00 − 1.00 for use in the final HSM. GIS was then utilized to create a composite score for each study area raster cell by summing the individual factor scores and normalizing the summed individual factors scores to the maximum value to achieve a 0 to 1 (low to high) scale as follows:
(Factor 1 score + Factor 2 score + … + Factor 7 score)/Maximum Score = Composite Score
Here, “maximum score” is equal to the maximum score of the added factor scores for the PBS. Factors were added rather than multiplied to preserve each factor’s unique contribution to oyster habitat suitability regardless of whether any other factor scored a “zero” for a particular raster cell. In doing so we recognize that it may be possible to overcome some of the counter-indicated factors in areas with one or more “zero” scored factors. For example, it may be possible to place reef substrate in an area with no contemporary oyster reef and soft mud to a height that will allow oysters to thrive, especially in areas of historical reef presence. The composite map of HSI scores was then assembled for the entire PBS and a draft was presented to the SWG for evaluation and comment.
Factors not selected for inclusion in the HSI
Of the 13 factors considered but not included in the HSI () three were eliminated due to insufficient spatial data and/or data resolution (chlorophyl a, disease, hydrology and predation). Nine factors were excluded because they were not important as a primary driver of oyster reef viability in the PBS in the near-term or beyond (next 5 – 10 years) including altered hydrology, bathymetry, managed areas (in particular those with designated boundaries such as aquatic preserves and state parks, etc.), marsh migration areas, regulatory restrictions, sea level rise, shoreline type, temperature and water quality. One factor was absent from our study area (military restricted zones). Some of these excluded factors could become important for oyster reef survival in the PBS in the future depending on watershed and river management as well as the progression of climate change.
Model refinement and validation
The SWG was convened monthly over a year and a half to evaluate and review products related to PBS Oyster Plan development including the draft Oyster HSI. SWG suggestions were used to refine the HSI. One meeting was held to engage the SWG oyster fishers who had operated in the PBS for decades. Participants at this meeting were provided with large format printed maps of the PBS and asked to identify areas of reef where they had harvested oysters. The maps were later digitized and spatially compared with the HSI using GIS software as a means of validating the HSI with expert knowledge.
Model adaptation
In 2021, oyster reef mapping and condition assessment was conducted in the Santa Rosa County portion of the PBS (Johnson Citation2021b). This work entailed using side-scan sonar to map bottom conditions around known and suspected reef areas based on maps available through the Florida Fish and Wildlife Conservation Commission (FWC) as well as the entire lower portion of Blackwater Bay. Poling, a low-tech but effective method involving the assessment of bottom type hardness and composition by thrusting a pole onto and through the surface substrate, was also conducted concurrently with the side-scan sonar mapping to ground-truth sonar images and collect additional qualitative information on the bottom types encountered (e.g., whether buried shell substrate or hardbottom was present and approximate depth). This information was not available before the original Oyster HSI was completed. However, once available this new information was used to adapt the HSI by substituting the sediment type information originally used with the much higher resolution and more recently collected bottom type information.
Results
Habitat suitability index GIS processing
The resulting HSI raster is a grid of equal areas for all portions of the PBS with resulting suitability scores ranging from approximately 0.12 to 1.00 (). Using GIS, the model results were parsed into 5 classes using the natural break (Jenks) classification method (ESRI (Environmental Systems Research Institute and Inc.) Citation2023). Suitability scores associated with the highest oyster habitat suitability locations (HSI of 0.75 to 1.0) represent nearly 16% of the study area (). These highest suitability areas are primarily located in the central portion of East Bay and in southeastern portions of Escambia Bay (). High suitability areas (HSI of 0.64 to 0.74) represent approximately 27% of the study area and are located primarily in East and Escambia Bays with a portion also occurring in the lower East Bay River. Medium and low suitability areas (HSI of 0.54 to 0.64 and 0.41 to 0.54, respectively) each represent 20% of the study area and occur in much of Pensacola Bay. The lowest suitability areas (HSI of 0.12 to 0.41) about 9% of the study area and occur primarily in upper most reaches of Escambia and Blackwater bays. No one or two factors appear to drive the HSI results, but rather most suitable areas occur where the highest scores for three to four factors overlap ().
Model validation
Validation of the model using an independent source of qualitative information, i.e., the expert knowledge of area oyster fishers over recent decades, resulted in 87% agreement with the HSI areas with scores >0.50 (medium to high suitability range; ).
Model adaptation
Results of the bottom mapping conducted in 2021 were classified into the bottom types found in the PBS (i.e., soft mud/sand, buried shell, sand, moderately firm mud/sand, exposed shell and oyster reef structure and sand) and mapped using GIS software (Johnson Citation2021b; ). These results were incorporated into the existing HSI for the PBS to update the oyster HSI. The updated HSI results for BWB illustrate a finer detail of suitability for oyster reef restoration (). Whereas in the original application of the HSI all of the area was found to have a suitability of 0.54 or less, with utilization of the high-resolution mapping data, a majority of the BWB area was found to have and HSI score greater than 0.54 and a substantial area was found to have a suitability greater than 0.74. The task of adapting the model with new information required at most a couple of hours to substitute the updated bottom-type layer into the HSI using the raster calculator available in the GIS software.
Figure 7. Blackwater Bay (BWB) original HSI (far left), side-scan sonar bottom mapping (Middle), updated HSI using high resolution mapping (far right). The newly available side scan imagery was used to adapt the suitability information for this area. The highest suitability areas in BWB (those with buried shell bottom type) are now clearly discernable and may prove capable of supporting oyster reef with additional attention to substrate placement and type especially as climate change effects such as sea level rise progress. No exposed reef or oyster reef structure was found in BWB.
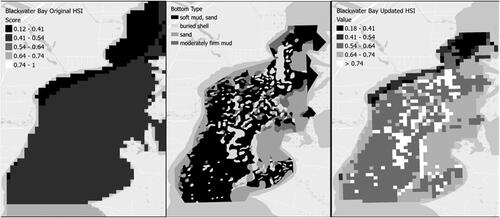
Discussion
HSIs are useful decision support tools for informing the likely best places to conduct ecosystem restoration by integrating relevant, objective, quantitative and spatially explicit information (Puckett et al. Citation2018; Roloff and Kernohan Citation1999). The value of the HSI results do however depend on validation with independent information (Chowdhury et al. Citation2019) and can be greatly improved with knowledgeable stakeholder input (Theuerkauf and Lipcius Citation2016; Puckett et al. Citation2018). In this study, we constructed and validated a spatially explicit HSI with seven factors relevant to oyster reef viability and restoration success using a simple and easily repeatable process. We also engaged ecosystem and oyster fisheries knowledgeable stakeholders to provide guidance and validation evidence.
The impact of nineteenth century exploitation, along with severe pollution in the 1960s and 1970s, extensive siltation driven by inappropriate road development, and management decisions based on the threat of contamination following the Deepwater Horizon oil spill, have reduced the PBS’s living oyster reef to less than 2% of its historic extent (based on a geospatial analysis of live oyster reef identified in Johnson,Citation2021a Citation2021b). It is therefore important to understand why oyster reef may no longer be present in the historical distribution areas. Such information can be used to focus restoration on those areas that have not been or are no longer being subject to stressors that would substantially impact viability. In the case of the PBS, it appears that the loss and burial of hard substrate in suitable locations that support reef building (e.g., existing or dead oyster reef) is the primary cause of loss as larval oysters require attachment to hard substrate to begin their sedentary lives (Collard,Citation1991a Citation1991b; Lewis, Kirschenfeld, and Goodheart Citation2016). For instance, the sedimentation of portions of the PBS from unsealed roads near tributaries has buried reef substrate in fine sediments up to 0.3 m deep in some areas (Johnson Citation2021b). Also contributing to the net loss of hard substrate has been direct removal of reef substrate through dredging, and extensive loss of live oysters through pollution spills and disease (WFRPC 2005; Radabaugh et al. Citation2019).
The HSI constructed for the PBS helps restoration practitioners greatly reduce the amount of area to consider for restoration. For example, an HSI score > 0.64 reduces the area to be considered to 43% of the study area. If the focus is to be only in the highest suitable areas (an HSI score of 0.74 or greater), the area to be considered is reduced to 16%. While 16% of the study area is still a large area for consideration, representing approximately 61 km2, the costs associated with evaluating site specific parameter suitability such as bottom composition, DO and salinity regimes, and presence/absence of predators or diseased oysters will be a fraction of the cost to consider the entire PBS. HSI results should be viewed as an initial step in the site selection process and do not negate the need for field verification (Barnes and Mazzotti Citation2005).
Puckett et al. (Citation2018) identified four categories of potential factors for inclusion in an HSI, physical processes, biological processes, permitting, and logistical considerations. While the purpose of the HSI described in this report was to focus on the biological and physical factors affecting the viability of oysters/oyster reef, we included the locations of existing seagrass meadows as these are a regulatory consideration of importance. Two preexisting human use factors were also included in our HSI framework, existing aquaculture leases and shipping channels. These represent conflicting use areas that should be avoided. Logistical considerations were left to a future phase of restoration planning due to the potentially large number of other factors that would require evaluation by a larger group of stakeholders (i.e., government agency, engineering, and construction professionals).
While the biological and physical factors we selected for inclusion in the HSI were based on quantitative data, the resolution of the datasets were somewhat limited in terms of age and spatial/temporal resolution (e.g., seasonal averages or based on a single sampling year). Even so, we were able to validate the efficacy of the HSI with an independent source of information based on the expert knowledge of oyster fishers operating in the study area over recent decades and found 87% agreement. Our findings suggest that reliable HSIs can be constructed even where high resolution quantitative information is unavailable for the factors to be included and that the integration of knowledgeable stakeholders into the development process is a valuable addition to HSI development.
Four stages of HSI construction were discussed in Theuerkauf and Lipcius (Citation2016), development, calibration, verification, and validation. While each of the obligate steps was incorporated into this analysis, we did not follow the recommendation of multiplying the factor scores but rather added them. As a consequence, our HSI scores ranged from 0.13 to 1, and not the full range of 0 to 1. We took this approach as we did not view any of the attribute conditions we included as insurmountable but rather informational and to be considered during the permitting and design phase of the restoration work.
The engagement of the SWG members is continuing as the PPBEP seeks to implement the PBS Oyster Plan and further narrow the locations of areas to be restored. The incorporation of SWG members into development of the HSI ensured familiarity with the product, and also fostered acceptance to use it as a decision support tool. The engagement of stakeholders in the process of HSI development is likely to lead to improved success in implementation of the reef restoration plans (Theuerkauf, Eggleston, and Puckett Citation2019). The PPBEP is now combining the HSI results with additional socio-economic and regulatory information to further refine potential restoration sites based on reef purposes using community and oyster fisher preferences.
The use of GIS in the development of an HSI simplifies the process and facilitates updating when the HSI is used as a component of an adaptive management approach. The adaptation of the HSI for Blackwater Bay with the new high resolution side scan sonar data was simple and time efficient. Whereas our initial HSI developed for Blackwater Bay was fairly coarse based on the available data, the new bottom-type data allowed for finer detail of the suitability for oyster reef restoration in this area. Today, Blackwater Bay is generally less suitable for oyster reef restoration than in many areas of East and Escambia Bays due to bottom type, yet there are areas (e.g., areas where buried shell substrate is present) where restoration may be successful through design and placement of materials that will support oyster growth and persist through time.
The HSI could also be applied to forecast how future climate change scenarios such as a changing salinity regime in the PBS could affect areas suitable for oyster reef restoration. Sea level is predicted to rise by 0.44 m (1.44 ft) under an intermediate scenario by the year 2060 (Interagency Sea Level Rise Scenario Tool, Escambia County, and Florida Citation2023). With increased sea level rise, the seasonal salt wedge into PBS will penetrate further up the estuary. Areas suitable for oyster reef restoration may migrate up the estuary apace with this change in salt wedge extent. Other impacts of climate change such as changes in precipitation in the watershed could either ameliorate or exacerbate the changes in salt wedge extent. Strategic and regular monitoring of key oyster viability factors in the PBS is recommended to better anticipate environmental changes to suitable areas for oyster reefs in the present and future.
The key contributions of this study were (1) development of a relatively quick, simple and low-cost method for developing a reliable HSI where quantitative physical and biological data for the system may be lacking in timeliness or resolution, (2) illustrating the value of incorporating oyster fisher expert knowledge as an independent source of information to validate the HSI results and build their support for its use, and (3) demonstrating how the HSI developed can be relatively easily adapted as new and/or higher resolution data becomes available.
Florida is a state with at least 29 recognized estuaries (NHD (National Hydrography Dataset)) Citation2022). Many of these estuaries historically supported oyster reef (Radabaugh et al. Citation2019). In only a few of these areas is high resolution quantitative data available on the physical and biological attributes of importance to oyster reef survival. Utilizing the process described in this study can serve as a guide to develop HSIs for other estuaries where data is limited. While some quantitative information is needed to construct an HSI, restoration should not be delayed. Rather, additional quantitative information should be collected and incorporated as part of an adaptive approach to the restoration planning and implementation process over time.
Supplemental Material
Download MS Word (15.1 KB)Acknowledgements
We thank Facilitated Solutions, LLC and all the Stakeholder Working Group members that were part of the process to develop the Oyster Fisheries and Habitat Management Plan for the Pensacola Bay System (Shelley Alexander, Jack Brown, Shawn Brown, Dr. Jane Caffrey, Will Dunaway, Beth Fugate, Pasco Gibson, Travis Gill, LD Henderson, Mark Jackson, Shelby Johnson, Chips Kirschenfeld, Matt Posner, Rick O’Connor, Donnie McMahon, Glen Miley, Josh Neese, Pete Nichols, Michael Norberg, Chris Phillips, Alan Pierce, Tommy Pugh, Phil Rollo, Portia Sapp, Kent Smith, Calvin Sullivan, Paul Thurman, Jim Trifilio, Chris Verlinde, and Christian Wagley, Keith Wilkins). We thank the Pensacola and Perdido Bays Estuary Program staff for utilizing the HSI results as part of the ongoing process to develop and implement estuary-scale oyster reef restoration in the Pensacola Bay System. We thank all the agencies and individuals that provided the data used in this study, Florida Department of Environmental Protection, Florida Fish and Wildlife Conservation Commission, US Environmental Protection Agency, National Oceanic and Atmospheric Administration and Marine Research Ecological Consulting. We thank Dr. Robert Brumbaugh for conceiving of the idea for developing an HSI as a useful addition to the PBS Oyster Plan development. We thank the funders of the oyster reef mapping and condition assessments: the Pensacola and Perdido Bays Estuary Program (Escambia County waters) and The Nature Conservancy (Santa Rosa County waters) and the reviewers of this manuscript for their helpful comments.
Disclosure statement
No potential conflict of interest was reported by the author(s).
References
- Arnold, W. S., S. D. Meyers, S. P. Geiger, M. E. Luther, D. Narváez, M. E. Frischer, and E. Hofmann. 2017. Applying a coupled biophysical model to predict larval dispersal and source/sink relationships in a depleted metapopulation of the eastern oyster, Crassostrea virginica. Journal of Shellfish Research 36 (1):101–18. doi: 10.2983/036.036.0112.
- Barnes, T. K., A. K. Volety, K. Chartier, F. J. Mazzotti, and L. Pearlstine. 2007. A habitat suitability index model for the eastern oyster Crassostrea virginica, a tool for restoration of the Caloosahatchee Estuary, Florida. Journal of Shellfish Research 26 (4):949–59. doi: 10.2983/0730-8000(2007)26[949:AHSIMF]2.0.CO;2.
- Barnes, T. K., and F. J. Mazzotti. 2005. Using conceptual models to select ecological indicators for monitoring restoration and management of estuarine ecosystems. In Estuarine Indicators, ed. S. A. Bortone, 493–502. Boca Raton, FL/USA: CRC Press.
- Beck, M. W., R. D. Brumbaugh, L. Airoldi, A. Carranza, L. Coen, C. Crawford, O. Defeo, G. J. Edgar, B. Hancock, M. C. Kay, et al. 2011. Oyster reefs at risk and recommendations for conservation, restoration and management. BioScience 61 (2):107–16. doi: 10.1525/bio.2011.61.2.5.
- Beseres Pollack, J., A. Cleveland, T. A. Palmer, A. S. Reisinger, and P. A. Montagna. 2012. A restoration suitability index model for the eastern oyster Crassostrea virginica in the Mission-Aransas Estuary, Texas, USA. PloS One 7 (7):e40839. doi: 10.1371/journal.pone.0040839.
- Beseres Pollack, J., D. Yoskowitz, H.-C. Kim, and P. A. Montagna. 2013. Role and value of nitrogen regulation provided by oysters Crassostrea virginica in the Mission-Aransas Estuary, Texas, USA. PloS One 8 (6):e65314. doi: 10.1371/journal.pone.0065314.
- Birch, A., R. Brumbaugh, B. DeAngelis, L. Geselbracht, A. Graves, J. Blair, and R. Jones. 2021. Oyster fisheries and habitat management plan for the Pensacola Bay System. Altamonte Springs, FL: The Nature Conservancy.
- Cake, C. W.Jr. 1983. Habitat suitability index models: Gulf of Mexico American oyster. Ocean Springs, MS: U.S. Department of the Interior, US Fish and Wildlife Service F\S/0BS-82/l0.57.
- Callihan, R., B. Depro, D. Lapidus, T. Sartwell, and C. Viator. 2016. Economic analysis of the costs and benefits of restoration and enhancement of shellfish habitat and oyster propagation in North Carolina. Research Triangle Park, NC: RTI International.
- Chowdhury, M. S. N., J. W. M. Wijsman, M. S. Hossain, T. Ysebaert, and A. C. Smaal. 2019. A verified habitat suitability model for the intertidal rock oyster, Saccostrea cucullate. PloS One 14 (6):e0217688. doi: 10.1371/journal.pone.0217688.
- Coen, L. D., M. W. Luckenbach, and D. L. Breitburg. 1999. The role of oyster reefs as essential fish habitat: A review of current knowledge and some new perspectives. In Fish habitat: Essential fish habitat and rehabilitation , Symposium 22, ed. L. R. Benaka, 438–54. Bethesda, MD: American Fisheries Society.
- Coen, L. D., R. D. Brumbaugh, D. Bushek, R. Grizzle, M. W. Luckenbach, M. H. Posey, S. P. Powers, and S. G. Tolley. 2007. Ecosystem services related to oyster restoration. Marine Ecology Progress Series 341:303–7. doi: 10.3354/meps341303.
- Collard, S. B. 1991a. Management options for the Pensacola Bay System: The potential value of seagrass transplanting and oyster bed refurbishment programs. Water Resources Special Report 91-4. Havana, FL: Northwest Florida Water Management District.
- Collard, S. B. 1991b. The Pensacola Bay system: Biological trends and current status. Water Resources Special Report 91-3. Havana, FL: Northwest Florida Water Management District.
- ESRI (Environmental Systems Research Institute, Inc.). 2023. Jenks Natural Breaks Classification. Site visited on October 27, 2023. https://wiki.gis.com/wiki/index.php/Jenks_Natural_Breaks_Classification
- FDACS (Florida Department of Agriculture and Consumer Services). 2020. Aquaculture lease parcel availability maps. Accessed November 22, 2022. https://www.fdacs.gov/Agriculture-Industry/Aquaculture/Aquaculture-Submerged-Land-Leasing/Aquaculture-Lease-Parcel-Availability-Maps
- FWC (Florida Fish and Wildlife Conservation Commission). 2019. Oyster habitats of Florida. Accessed July 7, 2022. http://geodata.myfwc.com/datasets/a78160f5acaf4439b49f9fbef4c100ac_5
- FWC (Florida Fish and Wildlife Conservation Commission). 2020. Contemporary oyster beds. Accessed November July 7, 2022. https://geodata.myfwc.com/datasets/myfwc::oyster-beds-in-florida/about
- FWC (Florida Fish and Wildlife Conservation Commission). 2021. Seagrass habitat in Florida. Accessed July 7, 2022. https://geodata.myfwc.com/datasets/myfwc:seagrass-habitat-in-florida/about
- Grabowski, J. H., R. D. Brumbaugh, R. F. Conrad, A. G. Keeler, J. J. Opaluch, C. H. Peterson, M. F. Piehler, S. P. Powers, and A. R. Smyth. 2012. Economic valuation of ecosystem services provided by oyster reefs. BioScience 62 (10):900–9. doi: 10.1525/bio.2012.62.10.10.
- Grizzle, R. E., J. R. Adams, and L. J. Walters. 2002. Historical changes in intertidal oyster Crassostrea virginica in a Florida lagoon potentially related to boating activities. Journal of Shellfish Research 21 (2):749–56.
- Grizzle, R., J. Greene, and L. D. Coen. 2008. Seston removal by natural and constructed intertidal Eastern Oyster Crassostrea virginica Reefs: A comparison with previous laboratory studies, and the value of in situ methods. Estuaries and Coasts 31 (6):1208–20. doi: 10.1007/s12237-008-9098-8.
- Hagy, J. D., and M. C. Murrell. 2007. Susceptibility of a northern Gulf of Mexico estuary to hypoxia: An analysis using box models. Estuarine, Coastal and Shelf Science 74 (1-2):239–53. doi: 10.1016/j.ecss.2007.04.013.
- Humphries, A. T., S. G. Ayvazian, J. C. Carey, B. T. Hancock, S. Grabbert, D. Cobb, C. J. Strobel, and R. W. Fulweiler. 2016. Directly measured denitrification reveals oyster aquaculture and restored oyster reefs remove nitrogen at comparable high rates. Frontiers in Marine Science 3:74. doi: 10.3389/fmars.2016.00074.
- Interagency Sea Level Rise Scenario Tool, Escambia County, Florida. 2023. Accessed November 4, https://sealevel.nasa.gov/task-force-scenario-tool
- Johnson, G. 2021a. Escambia and Pensacola bays subtidal oyster reef mapping and assessment. Marrero, LA: MREC Environmental.
- Johnson, G. 2021b. Pensacola East and Blackwater bays subtidal and intertidal oyster reef mapping and assessment. Marrero, LA: MREC Environmental.
- Jones, W. K., J. H. Cason, and R. Bjorklund. 1992. A literature-based review of the physical, sedimentary, and water quality aspects of the Pensacola Bay system. Water Resources Special Report 90-3. Havana, FL: Northwest Florida Water Management District.
- Kroeger, T. 2012. Dollars and sense: Economic benefits and impacts from two oyster reef restoration projects in the northern Gulf of Mexico. Arlington, VA: The Nature Conservancy.
- Kroeger, T., and G. Guannel. 2014. Fishery enhancement and coastal protection services provided by two restored Gulf of Mexico oyster reefs. In Valuing ecosystem services-methodological issues and case studies, ed. K. Ninan, 334–58. Cheltenham, England: Edward Elgar.
- La Peyre, M. K., A. T. Humphries, S. M. Casas, and J. F. La Peyre. 2014. Temporal variation in development of ecosystem services from oyster reef restoration. Ecological Engineering 63:34–44. doi: 10.1016/j.ecoleng.2013.12.001.
- La Peyre, M. K., D. A. Marshall, and S. E. Sable. 2021. Oyster model inventory: Identifying critical data and modeling approaches to support restoration of oyster reefs in coastal U.S. Gulf of Mexico waters: U.S. Geological Survey, Open-File Report 2021–1063, Reston, VA.
- Lai, Q. T., E. R. Irwin, and Y. Zhang. 2020a. Estimating nitrogen removal services of eastern oyster Crassostrea virginica in Mobile Bay, Alabama. Ecological Indicators 117:106541. doi: 10.1016/j.ecolind.2020.106541.
- Lai, Q. T., E. R. Irwin, and Y. Zhang. 2020b. Quantifying harvestable fish and crustacean production and associated economic values provided by oyster reefs. Ocean & Coastal Management 187:105104. doi: 10.1016/j.ocecoaman.2020.105104.
- Lewis, F. G. 2010. East Bay/Blackwater Bay/Lower Yellow River preliminary baseline resource characterization with a discussion of flow-dependent habitats and species. Water Resources Special Report 2010-2. Havana, FL: Northwest Florida Water Management District.
- Lewis, M., J. T. Kirschenfeld, and T. Goodheart. 2016. Environmental quality of the Pensacola Bay System: Retrospective review for future resource management and rehabilitation. EPA/600/R-16/169. Gulf Breeze, FL: US Environmental Protection Agency.
- Lipcius, R. N., R. P. Burke, D. N. McCulloch, S. J. Schreiber, D. M. Schulte, R. D. Seitz, and J. Shen. 2015. Overcoming restoration paradigms: Value of the historical record and metapopulation dynamics in native oyster restoration. Frontiers in Marine Science 2:65. doi: 10.3389/fmars.2015.00065.
- Livingston, R. J. 2015. Climate change and coastal ecosystems: Long-term effects of climate and nutrient loading on trophic organization. Boca Raton, FL: CRC Press.
- Millennium Ecosystem Assessment. 2005. Ecosystems and human well-being: Synthesis. Washington, DC: Island Press.
- NHD (National Hydrography Dataset). 2022. Accessed September 22, 2022. https://www.usgs.gov/national-hydrography/national-hydrography-dataset
- OCS (Office of Coast Survey, National Marine Fisheries Service). 2015. Coastal maintained channels in US waters. Accessed July 7, 2022. https://www.fisheries.noaa.gov/inport/item/39972.
- Olinger, L. W., R. G. Rogers, P. L. Fore, R. L. Todd, B. L. Mullins, F. T. Bisterfeld, and L. A. Wise. 1975. Environmental and recovery studies of Escambia Bay and the Pensacola Bay System, Florida. EPA 904/9-76-016. Atlanta, GA: US Environmental Protection Agency.
- Orlando, S. P., Jr., L. P. Roaza, G. H. Ward, and C. J. Klein. 1993. Salinity characteristics of Gulf of Mexico estuaries. Silver Springs, MD: National Oceanic and Atmospheric Administration, Office of Ocean Resources Conservation and Assessment.
- Paraso, M. C., S. E. Ford, E. N. Powell, E. E. Hofmann, and J. M. Klinck. 1999. Modeling the MSX Parasite in eastern oyster, Crassostrea virginica, populations. II. Salinity effects. Norfolk, VA: CCPO Publications.
- Piehler, M. F., and A. R. Smyth. 2011. Habitat-specific distinctions in estuarine denitrification affect both ecosystem function and services. Ecosphere 2 (1):art12. doi: 10.1890/ES10-00082.1.
- Powers, S. P., H. Roman, J. Meixner, D. Wirasaet, S. Brus, G. Fricano, and J. Westerink. 2023. Establishing connectivity patters of eastern oysters, Crassostrea virginica, on regional oceanographic scales. Ecosphere 14 (1):e4337. doi: 10.1002/ecs2.4337.
- PPBEP (Pensacola & Perdido Bays Estuary Program). 2022. Comprehensive conservation and management plan: A prescription for healthy bays. Pensacola, FL: Pensacola & Perdido Bays Estuary Program.
- Puckett, B. J., S. J. Theuerkauf, D. B. Eggleston, R. Guajardo, C. Hardy, J. Gao, and R. A. Luettich. 2018. Integrating larval dispersal, permitting, and logistical factors within a validated habitat suitability index for oyster restoration. Frontiers in Marine Science 5:76. doi: 10.3389/fmars.2018.00076.
- Radabaugh, K. R., L. D. Coen, S. P. Geiger, and R. P. Moyer. 2019. Introduction. In Oyster integrated mapping and monitoring program report for the State of Florida, eds. K. R. Radabaugh, S. P. Geiger, and R. P. Moyer, 1–23. St. Petersburg, FL: Fish and Wildlife Research Institute, Florida Fish and Wildlife Conservation Commission.
- Roloff, G. J., and B. J. Kernohan. 1999. Evaluating the reliability of habitat suitability index models. Wildlife Society Bulletin 27:973–85.
- Schamberger, M., and A. H. Farmer. 1978. The habitat evaluation procedures: Their application in project planning and impact evaluation. In Transactions of the forty-third North American Wildlife and Natural Resources Conference. Washington, DC. Wildlife Management Institute.
- Seavey, J. R., W. E. Pine, III, P. Frederick, L. Sturmer, and M. Berrigan. 2011. Decadal changes in oyster reefs in the Big Bend of Florida’s Gulf Coast. Ecosphere 2 (10):art114. doi: 10.1890/ES11-00205.1.
- Smeltz, H. A. 1898. The oyster-bars of the west coast of Florida: Their depletion and restoration. Bulletin of the US Fish Commission 17:305–8.
- Smith, R. S., S. Hogan, K. N. Tedford, B. Lusk, M. A. Reidenbach, and M. C. N. Castorani. 2022. Long-term data reveal greater intertidal oyster biomass in predicted suitable habitat. Marine Ecology Progress Series 683:221–6. doi: 10.3354/meps13949.
- Soniat, T. M., and M. S. Brody. 1988. Field verification of a habitat suitability model for the American oyster. Estuaries 11 (2):87–95. doi: 10.2307/1351995.
- Theuerkauf, S. J., and R. N. Lipcius. 2016. Quantitative validation of a habitat suitability index for oyster restoration. Frontiers in Marine Science 3:64. doi: 10.3389/fmars.2016.00064.
- Theuerkauf, S. J., D. B. Eggleston, and B. J. Puckett. 2019. Integrating ecosystem services considerations within a GIS-based habitat suitability index for oyster restoration. PloS One 14 (1):e0210936. doi: 10.1371/journal.pone.0210936.
- USEPA (US Environmental Protection Agency). 2004. The ecological condition of the Pensacola Bay System, northwest Florida (1994 - 2001). Report 620-R-05-002. Gulf Breeze, FL: USEPA.
- USFC (US Fish Commission). 1883. General chart of the coast, No. XIII, Gulf Coast from Cape San Blas to Mississippi Passes. Compiled by Lt. Francis Winslow. Washington, DC: US Navy.
- USFWS (US Fish and Wildlife Service). 1981. Standards for the development of habitat suitability index models. Washington, DC: USFWS.
- VanderKooy S, editor. 2012. The oyster fishery of the Gulf of Mexico, United States: A regional management plan–2012 revision. Publication No. 202. Ocean Springs, MS: Gulf States Marine Fisheries Commission. Available from http://www.gsmfc.org/publications/GSMFC%20Number%20202.pdf.
- Vinagre, C., V. Fonseca, H. Cabral, and M. J. Costa. 2006. Habitat suitability index models for the juvenile soles, Solea solea and Solea senegalensis, in the Tagus estuary: Defining variables for species management. Fisheries Research 82 (1-3):140–9. doi: 10.1016/j.fishres.2006.07.011.
- WFRPC (West Florida Regional Planning Council), Bay Area Research Council, National Oceanic and Atmospheric Administration, U.S. Environmental Protection Agency. 2005. The Pensacola Bay watershed management plan, an integrated action plan. Pensacola, FL: Cooperative publication of U.S. Environmental Protection Agency, Bay Area Research Council, National Oceanic and Atmospheric Administration, the West Florida Regional Planning Council.
- Zu Ermgassen, P. S. E., M. D. Spalding, B. Blake, L. D. Coen, B. Dumbauld, S. Geiger, J. H. Grabowski, R. Grizzle, M. Luckenbach, K. McGraw, et al. 2012. Historical ecology with real numbers: Past and present extent and biomass of an imperiled estuarine habitat. Proceedings of the Royal Society B: Biological Sciences 279 (1742):3393–400. doi: 10.1098/rspb.2012.0313.