Abstract
Background
Risk assessment of individual tobacco smoke components is important for the purpose of prioritization or selecting chemicals for monitoring products. Smoking is characterized by a highly varying, intermittent exposure and the challenge is to choose the most appropriate dose metric.
Methods
Generally, average daily exposure estimates are used as dose metric, without considering temporal determinants. The applicability hereof is discussed in the context of choosing dose metrics for local respiratory tract effects and for systemic effects in a smoking scenario or for the use of e-cigarettes.
Results
Using average daily exposure estimates for the smoking scenario can lead to erroneous risk evaluations for several reasons. Inhaled peak air concentrations during a puff can be two to three orders of magnitude higher than the calculated average daily inhaled concentration, which may impact the assessment of both systemic and local health effects. A pragmatic risk assessment is proposed, based on the Margin of Exposure (MoE) approach. The choice of an appropriate dose metric, such as inhaled concentration, inhaled dose or absorbed dose, depends on the type of effect. Temporal characteristics should be considered in the final step of the MoE approach, as is illustrated by two cases, glycerol and benzene.
Conclusion
The choice of an appropriate dose metric and inclusion of temporal determinants are important aspects in the risk assessment of individual smoke components. The proposed MoE approach provides the opportunity to weigh smoking-related exposure characteristics during the final step of the risk evaluation.
Introduction
Cigarette smoke is a complex mixture composed of more than 5000 chemicals, which are mostly generated by the burning of tobacco, including tobacco product additives (Rodgman and Perfetti Citation2013). It has been estimated that over 100 hazardous chemicals are present in cigarette smoke (Talhout et al. Citation2011; FDA Citation2012), of which at least 100 toxicants have been linked to a range of serious diseases in smokers (Camacho et al. Citation2015). For this reason, cigarette smoking is one of the largest public health threats in the world estimated to result in six million deaths worldwide yearly (WHO Citation2016). Major health concerns resulting from cigarette smoke, in addition to cancer, include cardiovascular disease (CVD) (WHO Citation2012a) and chronic obstructive pulmonary disease (COPD) (WHO Citation2012b).
There are good reasons to study the impact of individual components of main stream smoke (MSS), including the identification of individual components that may have the highest impact on human health and that should be prioritized for risk reduction measures and the evaluation of the possible impact of lowering the concentration in tobacco smoke of these components (Pankow et al. Citation2007; Cunningham et al. Citation2011; Xie et al. Citation2012; Pack et al. Citation2018, Citation2019). Another purpose is the selection of components that can be monitored to compare different products, such as electronic cigarettes and heated tobacco products. For instance, Chen et al. (Citation2017) compared the health risks of smoking versus the use of e-cigarettes based on an evaluation of individual chemicals present in MSS or aerosols from e-cigarettes. The use of e-cigarettes is increasing (Al-Hamdani et al. Citation2020) and because the composition of the aerosol of e-cigarettes is less complex than that of MSS, the health effects resulting from the use of e-cigarettes can be addressed by evaluation of the health risks of individual components.
Smoking of tobacco cigarettes or the use of e-cigarettes are examples of a complex exposure scenario in which the temporal determinants of the exposure scenario, i.e. exposure duration and frequency, are of special importance. The exposure is a dynamic process with an exposure pattern that is intermittent in at least two ways. Intervals are present between cigarettes and between puffs during the smoking of a cigarette. The daily exposure therefore is a compilation of multiple peak exposures with irregular time intervals.
Traditional risk assessment is dictated by the fact that in most frameworks appropriate data on kinetics and mode-of-action are not available. An exception is within the framework of the development of pharmaceuticals where insight in human kinetics, mode-of-action and potential adverse side effects is a requirement. Therefore, risk assessments often have to be based on a direct comparison of an external human exposure estimate with an external exposure estimate that is related to no or a limited level of toxicity, i.e. the point of departure (PoD). For an adequate assessment of possible health effects in a specific exposure scenario, the hazard information (i.e. information on potential toxicity from which the PoD is derived) needs to be applicable to that exposure scenario. This means that the exposure characteristics (route, intensity, duration, frequency) of the PoD should resemble that of the human exposure scenario under evaluation as much as possible. For smoking or the use of e-cigarettes, this is especially challenging since hazard information is never obtained with exposure regimens resembling these scenarios. As a result, in risk assessment the exposure estimate in the smoking scenario is often simplified and expressed as an average daily exposure estimate for individual MSS components (Cunningham et al. Citation2011; Xie et al. Citation2012; Pack et al. Citation2019). The question is whether the average daily dose is the most appropriate dose metric for this purpose and how risk assessment of individual components in MSS or e-cigarette aerosols can be improved.
The present manuscript aims to discuss relevant aspects related to the choice of the dose metrics for human health risk assessment of inhalation exposure to individual components of MSS or e-cigarette aerosols and to propose a pragmatic approach forward for improvement of prioritization and risk assessment and subsequent risk management decisions. The discussion will focus on exposure to MSS components but the basics are also applicable to the use of e-cigarettes. It is noted that risk assessment of environmental exposure to second hand or third hand smoke is beyond the present scope. Further, as a first step, emphasis will be on gasses and vapors since the exposure assessment and subsequent risk assessment of particulate exposure, including the choice of the appropriate dose metric, is much more complex and subject of debate (Bos et al. Citation2019). Nevertheless, the proposed method may, in some cases, also be applied to aerosols as will be shown by the example of glycerol.
Exposure assessment in smoking
Several methods for the risk assessment and hazard and/or risk prioritization of components in cigarette smoke have been proposed (Fowles and Dybing Citation2003; Pankow et al. Citation2007; Burns et al. Citation2008; Cunningham et al. Citation2011; Xie et al. Citation2012; Lachenmeier and Rehm Citation2015). The analysis by Fowles and Dybing (Citation2003) was the first to provide a method for hazard prioritization of 158 known chemicals in cigarette smoke based on estimates of cancer and non-cancer risk indices. Fifty-two chemicals were identified as suspected or known carcinogen by IARC, and non-cancer reference values (based on respiratory effects and cardiovascular effects) were identified for an additional 17 chemicals. This analysis was the premise for the WHO TobReg selection for toxicants recommended for mandated lowering, including: 4-(methylnitrosoamino)-1-(3-pyridyl)-1-butanone (NNK), N’-nitrosonornicotine (NNN), acetaldehyde, formaldehyde, acrolein, benzene, benzo[a]pyrene (BaP), 1,3-butadiene (BD) and carbon monoxide (Burns et al. Citation2008).
Exposure to chemicals present in MSS is generally estimated as an average daily exposure, either deterministic (Pankow et al. Citation2007; Cunningham et al. Citation2011) or probabilistic (Xie et al. Citation2012), sometimes as average daily concentration but most often as daily inhaled or sometimes (implicitly) as absorbed dose.
Dose metrics in inhalation exposures
In inhalation exposures, an exposure scenario can be described by the exposure concentration, frequency and duration. However, our understanding of an exposure-response relationship in inhalation exposures is largely based on animal experiments in which only the concentration varies but where the exposure duration and frequency generally are kept constant. In these situations, an increase in exposure is interpreted as an increase in exposure concentration or inhaled daily dose without considering temporal determinants. In contrast, an increase in exposure in smoking rather results from an increase in the number of cigarettes smoked daily, i.e. an increase in frequency and thereby in total daily exposure duration. The question then arises whether an exposure-response relationship derived from an animal experiment (i.e. exposure concentration- or dose-response), is applicable to the smoking exposure scenario (rather described by an exposure duration-response relationship).
In order to adequately characterize human health risks for an actual exposure scenario, exposure-response estimates for toxicity should be applicable to that exposure scenario (Jarabek Citation1995). This is generally put into practice by transferring exposure to an average exposure for a comparable duration, often an average daily exposure concentration irrespective of the duration and frequency. However, the appropriateness of such an approach depends on the mode of action of the chemical and the dose metric that best describes the exposure-response relationship. These aspects usually are not taken into account when exposure is averaged out (Jarabek Citation1995).
Averaging exposure is based on the concept of total dose, i.e. on Haber’s law and holds that a given calculated product of exposure concentration and time will always yield the same biological response. Although this concept was originally based on acute inhalation exposures to war gasses (Haber Citation1924), it nowadays is applied as a basic toxicological law with a general applicability. However, the use of Haber’s law has introduced confusion in the use of concentration and dose in inhalation exposures (Atherley Citation1985) because it neglects the importance of dose rate on toxicity outcomes. Over the past decades, the importance to include appropriate temporal determinants in the interpretation of toxicity outcomes and in the choice of dose metric regularly has been stressed and the generality of Haber’s law has seriously been questioned (Atherley Citation1985; Jarabek Citation1995; Gaylor Citation2000; Bunce and Remillard Citation2003; Belkebir et al. Citation2011; Felter et al. Citation2011; Kuempel et al. Citation2015; Connell et al. Citation2016). For instance, Jarabek (Citation1995) stressed the importance of considering temporal determinants in risk assessment by showing that the impact of temporal determinants on a chemical’s kinetics may vary per chemical. It was shown by modeling that the exposure concentration was the determinant factor for the biotransformation rate of dichloromethane whereas for perchloroethylene, exposure duration appeared to be the more dominant factor.
Belkebir et al. (Citation2011) evaluated animal experiments in which the toxicity outcome between intermittent and continuous exposures leading to the same inhaled total dose, was compared. They discussed four case studies with different types of effects (formaldehyde: nasal epithelium; carbon tetrachloride: hepatotoxicity; trichloroethylene: ototoxicity: benzene: hematotoxicity) for which appropriate experimental data were available. They concluded that Haber’s law does not have a general applicability for these four chemicals. It is therefore recommended to verify the applicability of Haber’s law case-by-case.
Jarabek (Citation1995) stated that concentration could be the appropriate dose metric for irritants, no matter the duration. ECHA. (Citation2012) also considers dose-related dose metrics not applicable for effects that are mainly concentration-driven, such as irritation. However, available animal experiments indicate that the exposure-response relationship for respiratory tract irritation might also be more complex. For instance, based on experiments with groups of rats exposed to formaldehyde up to 13 weeks (each group receiving a similar daily dose but different combinations of exposure duration and concentration), Wilmer et al. (Citation1987, Citation1989) concluded that exposure concentration rather than the total inhaled dose of formaldehyde determined the cytotoxicity on the nasal epithelium. In contrast, in a comparable experiment, Appelman et al. (Citation1986) did not observe differences in nasal toxicity in rats with different exposure regimens to acetaldehyde. Further, Arts et al. (Citation2004) exposed rats to furfural for either 3 or 6 hours per day for four weeks; groups of rats were exposed to various concentrations spaced by a factor of two. In rats exposed for 3 hours per day, the incidence and degree of severity of the histopathological changes in the nasal passage appeared to be less as compared to rats exposed for 6 hours to the same concentration but also as compared to rats exposed for 6 hours to half the concentration (i.e. to the same total dose). Thus exposure duration appeared to be an important factor for the induction of nasal passage lesions in rats by furfural. These examples with different outcomes show that further studies are needed to elucidate the exposure-response relationship for respiratory tract irritants.
Carcinogenicity is an important endpoint in smoking. In general, the cumulative dose is used as dose metric in human health risk assessment for carcinogenic effects. However, dose-rate (and thus time) may also be an important determinant for tumor induction. Based on an evaluation of the available literature, Verhagen et al. (Citation1994) concluded that a carcinogenic dose administered in a relatively short period of time showed a higher carcinogenic potency than when the same total dose was divided over a longer period of time and the use of a dose-rate correction factor was proposed. Felter et al. (Citation2011) proposed a framework for assessing health risks from less-than-lifetime exposures to carcinogens. In Appendix I of their publication, it was concluded that lifetime cumulative risk may not be the appropriate dose metric for MSS exposure. Duration of smoking, and especially recent exposures rather than the lifetime cumulative dose could be important determinants for lung cancer risk from smoking. It was stated that sharp drops in lung cancer risk upon quitting smoking argue against a uniform risk related to the cumulative number of cigarettes smoked over a lifetime. Also for benzene, an important tobacco smoke component, there were indications that concentration may be more critical for carcinogenesis than total cumulative exposure.
When increasing exposure by increasing the frequency, i.e. increasing the number of cigarettes per day, there is another aspect to consider. With increasing frequency, the time intervals between exposures (i.e. between cigarettes) become shorter. This will affect the onset and progression of health effects. For instance, the shorter the time interval between exposures (relative to the internal half-life of a chemical), the higher the likelihood of accumulation of the chemical in the body will be. Also, the degree or extent of repair may diminish when the between-exposure intervals will become smaller. Thus an increase of the number of smoking moments will have impact on the occurrence of adverse health effects for both kinetic as well as dynamic reasons (Doull and Rozman Citation2000; Rozman and Doull Citation2000, Citation2001). This clearly differs from the situation were an increase in exposure results from an increase in exposure concentration accompanied by constant exposure duration as is the case in the standard animal experiment.
Dose metric(s) in smoking
Awareness and knowledge of the role of exposure duration in inhalation exposures is especially of importance in smoking scenarios. To illustrate this, a smoking exposure scenario is compared with that for the worker (8-hour exposure per working day) and for the general population (continuous exposure of 24 hours per day), with the same total inhaled daily dose in each scenario. In this comparison, several default values are used but these can be substituted for actual measured data, if available; the default values for the smoking scenario are given in . Assuming that an amount of 10 mg of a fictitious chemical X is completely inhaled during the smoking of one cigarette, and that 20 cigarettes are smoked per day, the total inhaled dose equals 200 mg. Assuming 13 puffs per cigarette with a puff volume of 50 mL followed by inhalation of 500 mL (i.e. the tidal volume) of ambient air, it can be calculated that the average inhaled air concentration of chemical X per puff equals (10/13 mg/0.550 L =) 1.40 mg/L or 1400 mg/m3. During the smoking of one cigarette, the smoker inhales this concentration 13 times in 6 minutes (circa twice per minute). Assuming that in this scenario the 20 cigarettes are evenly divided over approximately 16 hours, a smoker is exposed to 13 peak exposures of 1400 mg/m3 during 6 min, every 48 minutes. Thus the 6-min exposures are separated by 42-min of non-exposure and finally, the 16-hour exposure period is followed by an 8-hour period of non-exposure, every day. For comparison, a worker would inhale the same total dose per day during an 8-hour exposure to a constant concentration of 20 mg/m3 (assuming an inhaled air volume of 10 m3 per 8 hours). Similarly, the corresponding exposure concentration for a member of the general population exposed for 24 hours would be 10 mg/m3 (assuming a total inhaled volume of 20 m3 per 24 hours). These calculations are summarized in and visualized in . Starting from the same total inhaled dose, the inhaled peak air concentrations of chemical X during a puff can be 70- and 140-fold higher than the average inhaled concentrations for a worker or a member of the general population, respectively. It is noted, that the average inhaled daily concentration is linearly related to the number of cigarettes smoked per day but that the height of the peak concentration is independent hereof. For instance, if in the given example the number of cigarettes is reduced from 20 to two cigarettes per day, the average daily concentration will also be decreased by a factor of ten. Since the inhaled peak concentrations will not change, it will then even be 1400-fold higher than the average daily exposure concentration, although the peak concentrations will occur less frequent and the total daily exposure duration is reduced from 2 hours to 12 min.
Figure 1. Comparison of the inhaled exposure concentrations of chemical X for different scenarios with the same total dose inhaled daily of 200 mg: smoking (20 cigarettes per day, 10 µg of chemical X/cigarette; a worker (8 hour exposure per day; inhalation rate of 10 m3 per 8 hour); a member of the general population (24 hour exposure per day; inhalation rate: 20 m3 per day). Only the first hour of exposure is shown. See text for additional details about the smoking scenario.
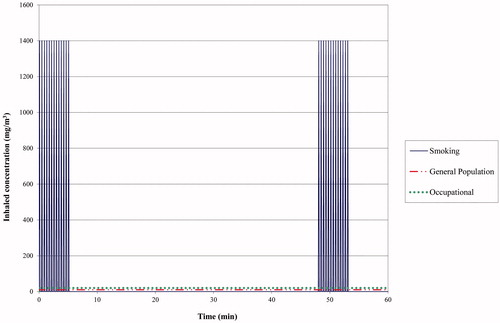
Table 1. Default parameter values for the exposure estimation during smoking.
Table 2. Inhaled concentrations for three scenarios (occupational, general population, smoking) starting from the same total inhaled dose of 200 mg of a fictive chemical X per day.
Ignoring the specific smoking exposure pattern and using the average inhaled daily dose of 200 mg as dose metric comes down to assuming that an average 24-hour exposure to 10 mg/m3 will induce similar toxicity as multiple peak exposures at regular intervals to a 140-fold higher concentration. The question then is whether it is reasonable to consider that such an assumption of equal toxicity independent of the dose-rate, is a general rule. On the contrary, it appears reasonable to assume that multiple pulses of high chemical exposure concentrations at regular intervals can have a more severe impact on the respiratory tract epithelium than a gradual exposure to an approximately two order of magnitude lower concentration. The more so, if the periods of non-exposure become shorter. The few available studies with varying exposure scenarios to respiratory tract irritants showed different results (Appelman et al. Citation1986; Wilmer et al. Citation1987, Citation1989; Arts et al. Citation2004) but differences in concentrations (at comparable total doses) were only a factor of two higher in the Wilmer et al. and Arts et al. studies and a factor of 5 in the Appelman et al. study.
Gasses and vapors are distributed throughout the airways when inhaled, whereas particle concentrations can be highly different depending on their deposition. Airway deposition is not dependent on the frequency of the exposure, but should be considered for risk assessment of local effects. Particles can be retained at the site of deposition, but this will depend on mucociliary clearance and particle dissolution. Further, the localization of deposition is dependent on the particle size and particle size distribution but also on factors such as airflow and anatomy of the respiratory tract. Currently, risk assessments often are based on comparison of external air concentrations, and deposition is not taken into account, even though we are aware of the differences in respiratory tract morphology between rodents and humans, and in the case of tobacco products exposure, also of the difference between inhalation via the nose for rodents and via the mouth for humans. Risk assessment of aerosols can be improved by consideration of deposition. However, the lack of adequate data on deposition of chemicals in the respiratory tract makes it not feasible yet to include this in a more general approach which is described here, but it is highly recommended for future improvements in chemical risk assessment of tobacco products.
As to systemic exposure, it can also be expected that differences in exposure regimen will lead to differences in internal dose metrics due to e.g. the possibility of saturation of kinetic processes (absorption, metabolism, excretion) which are important determinants of internal exposure and thus toxicity. This will also depend on chemical-specific characteristics. As mentioned, Jarabek (Citation1995) showed that the metabolic rate depends on the combination of exposure concentration and duration and that this dependency differs per chemical. It is therefore obvious that the different exposure scenarios in will result in different internal exposure patterns of the parent chemical but potentially also of its metabolites. If sufficient information is available, e.g. on biotransformation, PBPK-modeling can help to provide insight in the impact of these different scenarios on the internal exposures. Geraets et al. (Citation2018) simulated two different exposure scenarios with comparable total inhaled weekly doses for methyl tert-butyl ether (MBTE) and for 1,2-dichloroethane by PBPK-modeling, viz. a continuous exposure scenario (24 hours/day for 7 days per week) versus an intermittent scenario with a 6- or 7-hour exposure on weekdays only. The two scenarios showed large differences in venous blood concentrations over time. For instance, in the intermittent exposure scenario MTBE peak concentrations in venous blood were approximately twofold higher than that of its metabolite tert-butanol. In contrast, continuous exposure resulted in steady-state concentrations in venous blood of MTBE and tert-butanol, with tert-butanol concentrations being approximately twofold higher. Also, the ratio of the one-week AUCs of tert-butanol and MTBE in venous blood differed between the two scenarios, i.e. 1.4 versus 2.2 for the intermittent and the continuous exposure scenario, respectively.
The choice of an appropriate dose metric for the assessment of human health risks will depend on the mode of action of a chemical and its kinetics and dynamics. Therefore, to judge whether application of Haber’s law in an exposure scenario is justified, information about the mechanism of action is needed (Jarabek Citation1995; Belkebir et al. Citation2011). Among others, it should be verified whether the concentration - or time-effect relationship is linear (Belkebir et al. Citation2011). Comparison of the health risks of two chemicals may also require a comparison of different dose metrics, depending on the chemicals and their type of effect. For instance, the exposure concentration of a respiratory tract irritant may have to be compared with the systemically absorbed dose of a chemical inducing systemic toxicity. For the former the exposure concentration is considered to be more of importance whether for the latter also the exposure duration should be taken into account. To make it even more complex, one chemical can induce different toxic effects at different exposure levels to which different dose metrics may apply.
Lung cancer is the most prominent cancer type for smoking. For carcinogenic effects, the cumulative dose is usually applied as the dose metric in risk assessment. However, as stated in the previous section, the available data show that this assumption may not be generally applicable. Especially with inhalation exposures and lung cancer (i.e. tumors at the port of entry), it may be of importance to evaluate the impact of both the exposure concentration and the exposure duration separately and in combination to obtain an adequate insight in the exposure-response relationship. Insight in the mechanism of action is of high importance to adequately determine the appropriate dose metric and to relate the occurrence of health effects to the exposure.
Determination of the appropriate dose metric in smoking
An appropriate dose metric for risk assessment preferably reflects (internal) exposures at the target site. Considering the dynamic external exposure scenarios for smoking and for the use of e-cigarettes, internal dose metrics are presently not an option for individual components of MSS or of e-cigarette aerosols. The best way forward seems to use pragmatic dose metrics for risk assessment taking the different exposure determinants (intensity, duration and frequency) into account.
Dose metric for respiratory tract irritation
Because the concentration of an MSS component in inhaled air entering the respiratory tract varies continuously (see ), the determination of an appropriate dose metric for respiratory irritants is a real challenge. Although further investigations are needed to verify whether exposure concentration really is the most appropriate dose metric for the endpoint of respiratory tract irritation, it is the default assumption in regulatory frameworks (Jarabek Citation1995; Belkebir et al. Citation2011; ECHA Citation2012) and here taken as a pragmatic starting point. As a first choice, the best option will be to describe the smoking scenario in terms of exposure concentration, duration and frequency and to evaluate these exposure determinants in coherence as will be illustrated in the next section. This approach may help to identify the most appropriate hazard data (regarding the exposure regimen) and will provide insight in the uncertainties involved and thus in the kind of information that will help to refine the risk assessment, if needed.
The question then arising is how the exposure concentration should be defined and estimated since the mean concentration in MSS itself is not a good measure to compare directly with an exposure concentration derived from a toxicity experiment. The MSS inhaled during a puff is immediately diluted by a breath of ambient air before entering the respiratory tract. Following a puff, the concentration in the respiratory tract will (gradually) decrease over time until the next puff, among others because of deposition or absorption of the chemical and because of a number of breathing cycles of ambient air in between puffs. This fluctuation is nicely illustrated by Gordon et al. (Citation2002) who measured, among others, benzene in exhaled air during smoking. As a pragmatic approach, it is proposed to use a chemical’s concentration in the respiratory tract, taking into account dynamics of smoking and basic elements of respiration physiology. A balance is sought between adequacy (e.g. regarding smoking behavior and respiration physiology) and what is reasonably achievable depending on the data available.
The following parameters are used in the approach; default values for these parameters are presented in . The Tidal Volume (TV) is the volume of air that is inhaled or exhaled during breathing at rest. The volume of air that remains in the lungs at the end of the exhalation phase in rest is called the Functional Residual Capacity (FRC). Default values considered to be average values for an average adult human being are 500 mL for TV and 2 L for FRC. Further, during a breathing cycle, approximately 30% of an inhaled volume of air will not reach the alveoli where gas exchange takes place, i.e. the dead space volume. Default parameter values on human smoking behavior are obtained from Djordjevic et al. (Citation2000). After drawing a puff and one subsequent breathing-cycle, the interval until the next puff is set at 20 sec in smokers. Starting from a breathing-frequency at rest of 12 min−1, a 20-sec interval corresponds to four breathing cycles between puffs. Assuming thirteen puffs per cigarette, the time to smoke a cigarette lasts between 5 and 6 min.
Using the default values from , a first estimate of a chemical’s alveolar concentration can be estimated from its concentration in MSS (CMSS). The average CMSS per cigarette can be calculated by dividing the amount or dose of a component in the MSS of one cigarette (DMSS) by the total puff volume of one cigarette (i.e. 13 × 50 = 650 mL, see ). When taking a puff, a volume of approximately 50 mL of MSS with a calculated CMSS is inhaled. Following a puff, a volume of ambient air equal to the TV of 500 mL is assumed to be inhaled instantaneously, which means a total inhaled volume of 550 mL for a puff and a subsequent inhalation. The concentration of a chemical in MSS (CMSS) is thus diluted by a factor of 11 (550/50), and the exposure concentration in the extrathoracic (ET) and the tracheobronchial (TB) airways can be estimated as CMSS/11. Because of the dead space volume, only 70% of the inhaled volume of 550 mL (i.e. 385 mL) will reach the alveoli. This volume of 385 mL is mixed by diffusion with the volume of air present in the lungs (i.e. the FRC equaling approximately 2 L). Thus, the concentration of MSS components reaching the alveoli is diluted further by approximately a factor of maximally six (i.e. 385 mL mixed with 2 L). However, this process of diffusion will not be very fast and may not be completed before the next inhalation of ambient air. The component will then not be evenly distributed within the lungs and the concentration will vary. Therefore, instead of a dilution factor of six, a more conservative arbitrary factor of three was chosen for the estimation of a chemical’s initial alveolar concentration immediately following the first puff. Using this factor of three combined with the abovementioned dilution factor of 11, the initial alveolar concentration can be estimated to be a factor of 33 lower than CMSS.
It is acknowledged that several aspects are not taken into account in this approach, such as a possible retention in the ET and TB airways. However, it should be realized that the exposure estimate is for the purpose of risk assessment in which it is compared with a PoD (i.e. an (external) exposure estimate), obtained from either an epidemiological study or an animal toxicity experiment. In either case, it will be assumed that retention in the smoking scenario will be comparable to that in the exposure scenario underlying the PoD. When appropriate data are available to quantify a difference in retention, or in any other aspect, for these two exposure scenarios, the risk assessment can be further refined.
In , two fictitious scenarios are simulated to visualize the course of the alveolar concentration of MSS components during the smoking of one cigarette. One scenario assumes a rapid absorption into the systemic circulation (solid pink line). A second scenario whereby a low absorption of 15% is assumed, is depicted for comparison (dashed green line). For both scenarios, the alveolar concentration during smoking of one cigarette is depicted relative to the alveolar concentration immediately after the first puff (i.e. the initial alveolar concentration is set equal to 1). When breathing ambient air in between two puffs, 70% of the TV (i.e. 350 mL) will mix with the air present in the lung (i.e. 2000 mL) and a similar volume of 350 mL will subsequently be exhaled from the lung. Apart from absorption, the alveolar concentration of a chemical then will decrease by approximately 15% (i.e. equal to 100% × 350/(2000 + 350)) with each breathing cycle, assuming perfect mixing. For the solid pink curve (rapid absorption), it is assumed that, next to this 15% decrease, approximately an additional 80% of the amount present in the alveolar air is absorbed, leading to a negligible alveolar concentration just before a subsequent puff.
Figure 2. Simulations of alveolar air concentrations of a cigarette smoke component during the smoking of one cigarette in two scenarios, one with a substantial alveolar absorption (i.e. 80%) (pink curve) and one with low alveolar absorption (i.e. 15%) (green curve). Alveolar concentrations are expressed relative to the initial alveolar concentration after the first puff.
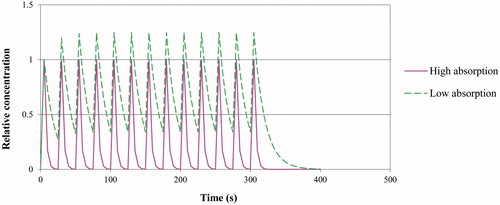
In the second scenario (low absorption of 15%), the alveolar concentration varies between approximately 0.34 and 1.25 times the initial alveolar concentration after the second puff. In this scenario, a chemical’s maximal alveolar concentration (Cmax) is estimated to be about 1.25 times higher than the initial alveolar concentration after the first puff. It is realized that, in general, it will rather be retention than absorption that will be experimentally estimated; for the present purpose it is assumed that the amount absorbed is approximately equal to the amount retained. Unless in case of a very low retention, the Cmax in the ET and TB airways can be estimated as CMSS/11 and in the alveoli as CMSS/33. Although Cmax can be chosen as a first worse-case approach, Cmax is only reached during very short, instantaneous moments and considered as a too worst case estimate (). Instead, 0.5×Cmax is proposed as a more realistic worst case estimate. Further, as a pragmatic first approach, the total exposure duration can be estimated as the sum of the durations of the individual exposures during one day. For instance, smoking 20 cigarettes then cumulates to an exposure duration of in total (20 × 6 min=) 120 min or 2 hours. The impact of disregarding the recovery periods in-between (including the possibility of non-daily smoking) can be considered in the final step of the risk assessment process (see section below on risk assessment).
Dose metric for systemic exposure
For risk assessment of concentration-driven systemic effects (such as depression of the central nervous system (CNS)), emphasis may be more on the exposure concentration than on the exposure duration although both should be taken into account. For dose-related systemic effects, the absorbed dose can be considered as dose metric, depending on the exposure scenario that underlies the hazard information. However, it should be realized that the larger the differences in concentration and duration between the smoking scenario and the exposure regimen underlying the hazard information, the larger the uncertainties will be in the final assessment. It cannot be assessed beforehand whether this will result in an under- or overestimation of risks involved. For instance, a high dose rate may result in saturation of a biotransformation pathway and the impact on health risks will depend on whether this pathway includes bioactivation or deactivation (see for instance the example of benzene hereafter).
Dose metric for carcinogenicity
For cancer endpoints, risk assessment is generally based on the assumption that tumor induction is a function of cumulative dose; the total dose determines the cancer risk irrespective of the duration of exposure, i.e. the dose rate. As aforementioned, there are reasons to doubt the general applicability of this concept (Bos et al. Citation2004; Felter et al. Citation2011). In inhalation exposures, considerations for temporal adjustments may especially be of importance for lung tumors, depending on the mechanism of action. Nevertheless, since an appropriate alternative is not available, the concept of cumulative dose might be applied to assess the cancer risk from exposure to carcinogenic chemicals for pragmatic reasons. As long as the exposure period is nearly lifetime, the concept of cumulative dose might give a reasonable estimate of the carcinogenic risk for individual smoke components. The inhaled dose can be used as dose metric for tumors of the respiratory tract and the absorbed dose for systemic occurring tumors. It is recommended to take the smoking exposure characteristics into consideration during the risk assessment process.
Risk assessment of MSS components
Considering the varying exposure determinants in smoking, the question remains how to assess risks from exposure to individual smoke components. Human limit values for inhalation exposures generally are derived for a fixed exposure scenario, e.g. continuous exposure for limit values for the general population (such as the WHO Air Quality Guidelines) or intermittent exposures of 8 hours per day for 5 days per week for workers (e.g. an occupational exposure limit). Direct comparison of an exposure estimate for smoking with a human limit value does not take into account factors such as the impact of differences in exposure duration and rate on the internal exposure (Atherley Citation1985; Jarabek Citation1995; Belkebir et al. Citation2011). Since no adequate human limit values are available for the smoking scenario, the Margin of Exposure (MoE) approach has been proposed and already used as an alternative approach for risk assessment of the smoking scenario (Cunningham et al. Citation2011; Xie et al. Citation2012).
An MoE is the ratio of a reference point for toxicity (the PoD) and an exposure estimate in humans (EFSA Citation2005). The PoD is often taken from an animal experiment and corresponds to an exposure that causes a low but measurable response and can be a benchmark dose or concentration (e.g. a BMDL10 or BMCL10) or a No-Observed-Adverse-Effect Level (NOAEL), but also a Lowest-Observed-Adverse-Effect level (LOAEL) in the absence of a NOAEL. Preferably, the PoD is expressed in units of the appropriate dose metric and derived from an exposure scenario that closely resembles the exposure scenario under evaluation. The MoE is evaluated whether the ratio is sufficiently large to conclude that the exposure is of low or no concern. Any difference in exposure characteristics between the human exposure scenario of interest and the exposure scenario underlying the PoD, can be weighed in the evaluation of the MoE. Therefore, this approach provides the flexibility to take the specific smoking exposure determinants and other factors into account and provides the opportunity to choose the most suitable PoD. It is therefore, considered to be a good approach for the assessment of health risks related to MSS components.
Basically, if differences in the two exposure scenarios compared are negligible and no adverse effects are observed at the PoD, only interspecies and inter-individual differences in susceptibility need to be taken into account in the evaluation of the MoE. Typically, an MoE of minimally a factor of 100 is then considered to be required for non-carcinogenic effects (i.e. a factor of 10 each for interspecies and intraspecies differences) to reach a conclusion of low concern, which means that adverse health effects are not to be expected. In case a PoD is derived from adequate human data, interspecies differences do not need to be accounted for and an MoE of 10 might be concluded sufficient.
If the exposure scenario from which the PoD is derived significantly differs from the regarding human exposure scenario, these differences need to be bridged by taking them into account in the evaluation whether an MoE is sufficiently large. For instance, if the PoD is derived from a 13-week animal experiment a larger MoE is warranted if human exposure is expected to be lifetime. On the other hand, a lower MoE may be considered sufficient if the PoD is obtained from a lifetime daily 6-hour exposure whereas human exposure via smoking is limited to for instance a total duration of only one hour per day. Further, if the PoD is a LOAEL, an additional margin might be considered necessary. By considering all factors involved in the risk assessment of a human exposure scenario, a minimum value for the MoE can be determined that is required to reach the conclusion that no adverse health effects are to be expected.
The complexity of the human exposure scenario of smoking is such that it is difficult to clearly quantify all differences with for instance, a daily 6-hour exposure in an animal experiment. This hampers the determination of a minimum value for the MoE. However, the question may be asked what the appropriate exposure pattern in an animal experiment should be to sufficiently mimic the human smoking behavior, considering the large inter-individual (and possibly also the intra-individual) variation in smoking habits (Gust et al. Citation1983; Djordjevic et al. Citation1995; Pauwels et al. Citation2020). Should the animal exposure scenario resemble that for the light, medium or heavy smoker? The best pragmatic way forward is to calculate the MoE, to list the factors to be accounted for by the MoE, to quantify these factors where possible and to judge the remaining not-quantifiable factors by expert judgment and to justify the evaluation in a narrative. One aspect to be accounted for is the fact that exposure is actually not continuous but in peaks with intermediate low (zero) alveolar concentrations (). This will not play a major role in the estimation of the total systemic dose, although the importance of dose rate should be kept in mind. Regarding the evaluation of local effects, it is important to keep in mind that a constant concentration is assumed during the smoking of a cigarette whereas in reality the concentration will fluctuate and recovery can take place in-between puffs and in-between cigarettes.
For the assessment of a minimal MoE value for carcinogenic endpoints, the approach recommended by the European Food Safety Authority (EFSA) can be applied (EFSA Citation2005). For risk assessment of chemicals that are both genotoxic and carcinogenic, EFSA stated that an MoE of 10,000 or higher would be of low concern from a public health perspective and can be considered low priority for risk management when it is based on a BMDL10 from a chronic animal study. This MoE of 10,000 is considered to account for factors such as species differences, human variability, uncertainties with regards to the nature of the carcinogenesis process, and the reference point on the dose-response curve. Additional uncertainties introduced by a reference point other than a BMDL10 can be accounted for in the evaluation of the MoE.
In the proposed approached, eight steps can be distinguished which are summarized in . Following these steps, the proposed approach will be illustrated by two examples, glycerol as example of a respiratory tract irritant and benzene as a systemic carcinogen. In both examples, a smoking scenario of daily consumption of 20 tobacco cigarettes per day is chosen (leading to an exposure duration of 120 min (step 1a in )) and the default values in are used. The exposure assessment can easily be adapted to scenarios with a higher or lesser frequency of smoking or with measured values substituted for the default values.
Figure 3. Stepwise, pragmatic approach for the risk assessment of individual components in tobacco smoke and e-cigarette aerosols.
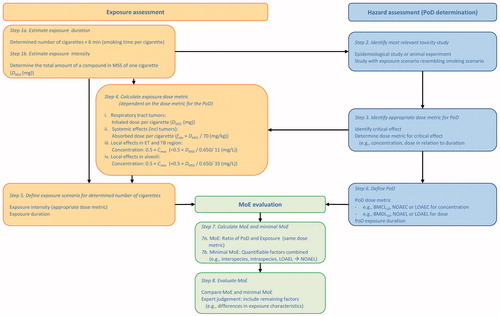
Example of glycerol
Exposure assessment
Glycerol is an important component of liquids for e-cigarettes and is also added to cigarettes as a humectant with a typical level in tobacco filler of 1.1%. Glycerol is on the European Commission’s list of priority additives (Pennings et al. Citation2019). The amount of glycerol present in the final product depends on the materials used in the manufacturing process and varies greatly per brand. Its presence is relatively high in comparison to other cigarette constituents. Recently, an average content of 7.8 mg glycerol/g tobacco was reported with a range of 1.1 to 23.7 mg/g (Pennings et al. Citation2019). Assuming a cigarette containing 700 mg tobacco, this corresponds to an average content of 5.5 mg glycerol/cigarette. The transfer rate of glycerol to MSS in filtered cigarettes has been reported to be 12% (Paschke et al. Citation2002), from which an average inhaled total dose (DMSS) of 0.66 mg per cigarette can be calculated (step 1 b). The appropriate dose metric for the PoD is concentration in the ET airways (steps 2 and 3, see below under PoD Selection). Starting from 13 puffs of 50 mL per cigarette (total volume of 0.65 L), an average concentration in MSS (CMSS) of (0.66/0.650=) 1.02 mg/L can be calculated. Then, as reasoned previously, the Cmax for the ET and TB airways is a factor of 11 lower, i.e. 0.092 mg/L or 92 mg/m3, and 0.5×Cmax equals 46 mg/m3 (step 4 iii). Hence, the exposure to glycerol is estimated to be to 46 mg/m3 for a total duration of 2 hours (step 5).
PoD selection
Pyrolysis of glycerol may also give rise to increased concentrations of aldehydes such as acrolein in MSS (Pennings et al. Citation2019); acrolein is much more toxic to the respiratory tract than glycerol (Feron et al. Citation1978). This formation of aldehydes is further left out of consideration, and should be assessed separately. The most appropriate study for PoD derivation for glycerol is the rat experiment by Renne et al. (Citation1992) (step 2). Main effects consisted of local irritation, i.e. squamous metaplasia of the epithelium lining of the epiglottis, observed when rats were exposed to 662 mg/m3, 6 hours per day, 5 days per week for 13 weeks, with no toxic effects observed at an exposure concentration of 165 mg/m3 (Renne et al. Citation1992) (step 3). No systemic effects were reported in this study or in a study with rats exposed to concentrations of up to 3910 mg/m3, 6 hours per day, 5 days per week for 14 days. The NOAEL of 165 mg/m3 for a 6-hour exposure was chosen as PoD for glycerol (step 6).
Risk assessment
The MoE for respiratory tract irritation calculated from the 6-hour PoD of 165 mg/m3 (step 6) and the 0.5×Cmax of 46 mg/m3 for the effects on the epiglottis (for a 2-hour exposure, step 5) is 3.6 (step 7a). For evaluation of this MoE, several factors need to be considered; basic factors include interspecies extrapolation (rat to humans), interindividual variability and temporal adjustments (i.e. less-than-lifetime exposure (13-week exposure versus (near) lifetime smoking and 6 hours/day for 5 days/week experimental exposure versus daily intermittent exposure to MSS with a total duration of 2 hours per day) (see for summary). To obtain an impression of what the minimal value of the MoE approximately should be (step 7 b), the default assessment factors as recommended by ECHA are applied to the PoD (ECHA Citation2012), i.e. local effects in the respiratory tract observed in a sub-chronic study. The following factors apply for the general population: 2.5 for interspecies differences for local effects on the respiratory tract, 10 for intraspecies differences in the general population, and 2 for sub-chronic to chronic exposure duration, leading to a total factor of 50. Further, the following remaining not-quantifiable factors need to be considered (step 8). For induction of respiratory tract effects the default in regulatory frameworks is that concentration is expected to be of more importance than the exposure duration; thus at least a longer daily recovery period is anticipated in the smoking scenario (although spread over the day). However, in contrast, in the animal experiment a 66-hour recovery period is present every weekend. Some time-related differences suggest that a smaller minimal MoE may be sufficient while others rather indicate that a higher value is required to reach a conclusion of no concern. Although it is difficult to weigh the impact of all these factors in the evaluation of the MoE in combination, it can be concluded that an MoE of 3.6 is so low that a risk of effects on the epiglottis due to glycerol is present when smoking a number of cigarettes per day.
Table 3. Summary table for MoE calculation and evaluation for glycerol.
For comparison, starting from the transfer of 0.66 mg glycerol into the MSS per cigarette and assuming a daily breathing volume of 20 m3, an average daily concentration of 0.66 mg/m3 can be calculated when 20 cigarettes are smoked per day. The MoE then would be (165/0.66=) 250 and a direct comparison with the minimal MoE value of 50 (as calculated in the preceding paragraph) would lead to a conclusion of no concern for glycerol-induced effects. If 2 cigarettes were smoked per day, the average daily concentration would be 0.066 mg/m3 and the MoE would be 2500.
Example of benzene
Exposure assessment
As mentioned in the introduction, benzene is one of the nine chemicals of the WHO TobReg selection for toxicants that are recommended for mandated lowering (Burns et al. Citation2008). Benzene is generated from the thermal decomposition of complex organic molecules such as complex sugars, amino acids, waxes, and cinnamic acid (Chortyk and Schlotzhauer Citation1973, Citation1975; O'Connor and Hurley Citation2008); a pulmonary absorption of approximately 50% benzene at relatively low levels of exposure is estimated for humans (Nomiyama and Nomiyama Citation1974; FDA Citation1999; HPA Citation2007; Health-Canada Citation2009). It is noted that by using pulmonary absorption rather than alveolar absorption, the dead space volume has been accounted for.
An average amount (DMSS) of 105 µg benzene per cigarette adjusted per mg nicotine was measured in MSS (step 1 b) (Counts et al. Citation2005; Burns et al. Citation2008), which is considered to be the inhaled dose per cigarette. Since for benzene leukemia is the cancer endpoint, the absorbed dose is the pragmatic dose metric of choice (see below under PoD Selection). From the DMSS, an absorbed dose per cigarette of 0.75 µg/kg body weight was calculated (assuming 50% pulmonary absorption and a 70 kg body weight) (step 4 ii). The daily absorbed dose for benzene (assuming a consumption of 20 cigarettes per day) is estimated to be 15 µg/kg or 0.015 mg/kg (step 5).
PoD selection
From the available epidemiological data, Health Canada derived a TC05 (the concentration in air for a 24-hour exposure that is associated with an additional cancer risk (incidence or mortality) of 5%) of 15 mg/m3 for the general population (CEPA Citation1993), which is selected as a PoD for benzene (steps 2 and 3, implicitly included in the evaluation by Health Canada (CEPA Citation1993)). Assuming a breathing volume of 20 m3 per 24 hour, a pulmonary absorption of 50% and an average body weight of 70 kg, the absorbed daily dose (24-hour exposure to 15 mg/m3) is estimated to be (15 (mg/m3) × 20 (m3/24 hour) × 0.5)/70 kg =) 2.1 mg/kg/day (step 6).
Risk assessment
For benzene an MoE of 140 (i.e. 2.1/0.015) can be calculated for carcinogenic effects based on average amounts of benzene in MSS (step 7a) (see for summary). For carcinogenicity, EFSA considered an MoE of 10,000 to be of low concern if the PoD is based on a BMDL10 obtained from an animal experiment (EFSA Citation2005). For benzene, the PoD is based on human information. Since in general a factor of 10 is applied for animal-to-human extrapolation (for the general population), an MoE of 1000 may in this case already be considered of low concern (step 7 b). In addition, the PoD is based on a TC05 instead of a BMDL10. Whether a TC05 is a more or a less conservative estimate than a BMDL10 depends on several factors, e.g. the uncertainties involved in both estimates. For pragmatic reasons the TC05 is considered to be approximately as conservative as a BMDL10 in the present example.
Table 4. Summary table for MoE calculation and evaluation for benzene.
Further, a remaining factor to be accounted for is the difference in rate at which the chemical is estimated to enter the systemic circulation (step 8). In a smoking scenario of 20 cigarettes per day, the exposure is spread out over the day in 20 periods of approximately 6 minutes, as compared to continuous exposure for the PoD. The impact hereof on the interpretation of the MoE is difficult to assess. With cumulative dose as a pragmatic dose metric, the implicit assumption is that the rate of entrance into the systemic circulation is negligible. However, the Health Council of the Netherlands (HCN Citation2014) concluded that in experimental animals the absorption of benzene decreases with increasing exposure concentration. As mentioned previously, the inhaled concentration in smoking may be orders of magnitude higher than in a continuous exposure scenario as is assumed for the TC05 (see ). Further, the first step of metabolism of benzene is oxidation by CYP 2E1 which may also become saturated at relatively high exposures. Unfortunately, the data for benzene are insufficient to determine the difference in impact hereof on the benzene toxicity at high exposures of short duration as compared to lower concentrations for a longer duration.
Overall, starting from the cumulative dose and comparing the MoE of 140 with a minimally required MoE of 1000 (for a conclusion of ‘low concern’) a concern for a carcinogenic risk of exposure to benzene via smoking of 20 cigarettes per day is present.
It is noted, that in this example for benzene, using an average daily dose as dose metric would have provided the same MoE. However, by using the proposed approach a risk assessor will be aware of issues related to temporal characteristics, such as the dependency of absorption on exposure concentration for benzene, and take them into account in the evaluation of the MoE, if possible.
Discussion
Tobacco smoke is composed of over 5000 chemicals and is responsible for a high incidence of adverse health effects such as cancer, cardiovascular disease, chronic obstructive pulmonary disease, etc. (Talhout et al. Citation2011; WHO Citation2012a, Citation2012b; Rodgman and Perfetti Citation2013). There are good reasons to evaluate individual MSS components, for instance to prioritize for risk reduction measures or to assess the possible health impact of risk reduction strategies. For use of e-cigarettes, risk assessment of individual components such as flavoring compounds, can provide a valuable contribution to assess overall risks.
Exposure via MSS is a dynamic process where periods of continuously changing concentrations of smoke components in the respiratory tract alternate with periods of non-exposure. It is understandable that exposure estimates are simplified, also because the appropriate hazard data to perform an appropriate risk assessment of this complex scenario are lacking. PoDs are generally derived from animal experiments, with a standard exposure scenario of 6 hour/day for 5 days per week, or from epidemiological studies for which exposure scenarios may differ significantly from that for smoking. In several studies, (lifetime) average daily exposures were calculated in a similar way as for the general population in (Cunningham et al. Citation2011; Xie et al. Citation2012; Pack et al. Citation2019). However, shows an approximately two order of magnitude difference between the calculated inhaled concentration and an average daily concentration, and thus in the calculated MoE, when smoking 20 cigarettes per day. Cunningham et al. (Citation2011) did acknowledge that their exposure assessment needed further refinement.
Calculation of an average daily dose relies on the applicability of Haber’s law, but, as discussed, its applicability should be verified case-by-case. One should be aware of the assumptions and simplifications made and try to take them into account and to weigh them in the final step when the MoE is evaluated (step 8 in ). One important aspect is the choice of the most appropriate dose metric for a health effect under consideration. The use of average daily exposures as dose metric, either as average daily inhaled concentration or as an average daily dose, implicitly means that an exposure-response relationship refers to a dose-response relationship. However, in smoking an increase in exposure predominantly comes from an increase in the number of cigarettes and hence the exposure-response relationship rather refers to the relationship between exposure duration and response. It is therefore, recommended to pay more attention to the role of both concentration and duration in inhalation exposures. This requires insight in the mode of action of a chemical, but this information is generally not available. Until such data become available, the best way forward is to account for the characteristics of the smoking scenario as good as possible in a pragmatic way.
Many chemicals present in MSS or in the aerosols of e-cigarettes induce irritation of the respiratory tract. Although the available animal data point that exposure duration may play a role, it is generally accepted in the context of risk assessment that the exposure concentration plays a more dominant role. For these kind of effects, the calculation of a daily average concentration might not provide the appropriate picture of the situation. shows an approximately two order of magnitude difference between the calculated inhaled concentration and an average daily concentration, and thus in the calculated MoE, when smoking 20 cigarettes per day. As illustrated by the example of glycerol, the use of an average human exposure estimate can lead to an underestimation of human health risks. It also showed that the lower the number of cigarettes smoked, the lower the average exposure concentration will be, although the calculated inhaled concentration remains the same. Hence, the lower the number of cigarettes smoked, the greater the difference between the calculated inhaled concentration and a daily average inhaled concentration. Also for systemic exposures, one should be aware of the possible impact of dose rate on the toxicity outcome, as was indicated by the example of benzene. Depending on the dose rate, the internal exposure pattern may vary largely (Jarabek Citation1995; Geraets et al. Citation2018) which will affect the extent of retention and absorption, metabolism and toxicity outcome (Belkebir et al. Citation2011). In addition, in intermittent exposure, as is the case in smoking and also in the use of e-cigarettes, the length and frequency of recovery periods is also an important factor to be considered, both for respiratory tract effects as well as for systemic effects. Unfortunately, adequate data to take these factors into account in a quantitative way are generally lacking.
A pragmatic method is proposed to better address both exposure concentration and exposure duration in the risk assessment of exposure to smoke components (see ). Respiratory exposure is characterized by concentration, duration and frequency. The concentration in the different parts of the respiratory tract is estimated in a pragmatic way, taking basic dynamics of smoking and basic elements of the respiration physiology into account. With this, a balance is sought between adequacy and pragmatism. The exposure duration is estimated from the number of cigarettes smoked per day and the duration of smoking one cigarette (default of 6 min); e.g. an exposure duration of 2 hours is used for risk assessment of smoking 20 cigarettes per day. The frequency of smoking (the number of cigarettes per day with intermediate periods of non-exposure) is difficult to quantify and is considered in the final evaluation step of the risk assessment. It is noted that frequency can only be adequately taken into account if appropriate data on toxicokinetics (e.g. half-life of toxic agent) and toxicodynamics (e.g. rate of repair) are available.
For systemic exposures, it is preferable to start from the combination of exposure concentration and duration in the smoking scenario and to search for hazard information that fits that scenario. In that case, dose rate can be accounted for. Internal exposure can be estimated with PBPK-modeling which can include the impact of temporal characteristics. Kinetic processes may also depend on the dose rate and this can be made more explicit by PBPK-modeling (Jarabek Citation1995). However, if the internal exposure cannot be estimated, the inhaled or absorbed dose may be used as dose metric as a pragmatic choice but one should remain aware that these dose metrics may not be appropriate if dose rate is ignored, especially for smoking and vaping exposure scenarios. Also the measurement of adequate biomarkers might be of aid to understand the relationship between external and internal exposures in a smoking scenario.
The MoE approach is proposed for risk assessment of the smoking scenario since it is a pragmatic and flexible approach. It provides the opportunity to choose a PoD that is best suitable for the smoking exposure scenario under consideration and to weigh uncertain factors in a narrative in a final evaluation step of the risk assessment process. Preferably, the exposure regimes of both the PoD and the exposure assessment should be comparable. Therefore, starting from the exposure estimate expressed as a combination of concentration and duration, hazard information should be sought that resembles these exposure characteristics as closely as possible. It is noted, that the best study to provide a PoD for smoking does not have to be the study that served as basis for the derivation of a human health-based guidance value such as an RfC or an OEL for environmental or occupational inhalation exposure.
For respiratory tract irritation, the MoE is calculated as the ratio of the exposure concentration in the toxicity experiment and an estimate of the human exposure concentration in the respiratory tract; the difference in duration can be taken into account and weighed in the evaluation step of the MoE. As a first step, 0.5×Cmax is suggested as concentration estimate for smoking. In the evaluation of the MoE, it should be verified what the MoE minimally should be to reach a conclusion of low or no concern, taking into account extrapolation steps such as inter- and intraspecies differences, differences in exposure characteristics (e.g. hours per day, days per week, number of weeks or years relative to lifetime) and other remaining uncertainties.
If for an individual smoke component a risk cannot be excluded with the proposed approach, it is recommended to identify the most important data gaps and to verify what data is required to refine the risk assessment, e.g. by generating chemical- or exposure-related information to substitute default values for actual data. Before starting to generate data, it can be verified whether refinement is likely to change the conclusion of concern.
It is noted that this approach can also be applied to groups or classes of chemicals that share the same hazard endpoint, such as aldehydes, glycols or nitrosamines. If within a group or class of chemicals a reference compound can be identified and relative potency factors can be estimated to transform the toxic potential of each chemical in that of an equipotent exposure of the reference compound, the exposure to the individual chemicals can be added up and considered as a (hypothetical) exposure to the single reference compound (Slob et al. Citation2020). The proposed approach then can be applied to the single reference compound.
The MoE approach has been used for prioritization of individual MSS components by ranking their MoE values (Cunningham et al. Citation2011; Xie et al. Citation2012). It should however be realized that a direct and straightforward comparison of MoE values is not appropriate. After all, a specific MoE value may be sufficient to conclude no concern in one situation but that same value may be insufficient in another situation, depending on the number and type of extrapolation steps and uncertainties involved. For instance, in the benzene example, an MoE of 140 was considered insufficient to reach a conclusion of no concern. In contrast, a minimal MoE value of 50 was estimated for glycerol and thus an MoE value of 140 for glycerol would have led to a conclusion of no concern. If MoE values are used for risk comparison between chemicals (e.g. for the purpose of prioritization), a calculated MoE for a specific compound should therefore, always be interpreted relative to its minimal MoE value required.
Hence, prioritization of tobacco smoke components just based on a comparison of absolute MoE values is not appropriate without taking into account what the minimally required value for the MoE should be for the individual chemicals that are compared. The minimal MoE value that is required in a specific situation depends on the differences in (exposure) characteristics between the human exposure situation under consideration and that of the study providing the PoD. When the PoD is based on an animal experiment, such as a BMDL10, a minimal value of 10,000 is considered of low concern for carcinogenic endpoints (EFSA Citation2005) whereas for non-carcinogenic endpoints a factor of 100 (consisting of a factor of 10 each for interspecies and intraspecies differences) can be considered to be sufficient if the PoD is a NOAEL for chronic exposure. Further, since these minimally required values include extrapolation of the animal data to the human population a lower value of the MoE might be considered adequate if the PoD is based on human data. On the other hand, if a PoD obtained from an animal experiment is a LOAEL and/or obtained from, for instance, a 90-day experiment, a larger MoE value is warranted to reach a conclusion of no concern. In addition, if the total daily exposure duration in a smoking scenario is significantly shorter than that for the PoD, a smaller MoE may be acceptable. Therefore, whether a specific MoE value is sufficient depends on the situation.
It is obvious that in general a reduction in exposure to a chemical will reduce the risk induced by that chemical. However, a quantitative estimation of the reduction in health risk given a specific increase in an MoE is not so easy. A reduction in exposure of, for instance, a factor of 5 will lead to a fivefold higher MoE but this does not necessarily mean that the health risk is decreased by that same factor. This will depend on several factors, among others on the slope of the exposure-response relationship. However, following the proposed approach it can be estimated what the reduction in exposure approximately need to be to reach a conclusion of no or a low concern. It is further noted that for systemic carcinogenicity, a linear relationship between dose and carcinogenic risk is generally assumed (concept of cumulative dose); a reduction in carcinogenic risk then is directly proportional to the reduction in exposure (i.e. dose). However, as discussed in a previous section, it should be kept in mind that in general, no scientific data are available underlying this assumption. In the case of benzene, for instance, it was brought forward that the rate of absorption and biotransformation changed with exposure level causing a non-linear exposure-response relationship.
In the proposed approach, including the examples, default values are used. It is recommended to replace these default values by chemical-specific data, whenever available. It is also recognized that large interindividual as well as intra-individual differences in smoking habits and topography do exist (Gust et al. Citation1983; Djordjevic et al. Citation1995; Pauwels et al. Citation2020). In addition, it will be worthwhile to verify the effect of interindividual and intraindividual variation in smoking or vaping habits on the exposure estimates and thereby on possible health risks. These differences may be subject of future research and may best be addressed by means of a probabilistic approach.
In summary, despite the fact that several anatomical and physiological aspects could not be taken into account, the pragmatic approach as proposed in , is believed to better address the characteristics of exposure from smoking or from the use of e-cigarettes, especially through the considerations in steps 3 and 4. This proposal is considered as a first step and it is recommended to further improve it by taking additional aspects, including anatomical and physiological aspects, into account. For instance, it is noted that especially step 4 (iii and iv, but probably also i), and thereby steps 7 and 8, can be improved by appropriate information on deposited dose (dose and location). At present, this is not possible and will require generation of specific data that are not obtained by default. Despite the absence of data, the approach still has the advantage to become aware of parameters that might be of importance for the assessment and to consider their possible impact on the outcome of the risk assessment process. Further, it helps to identify the most appropriate PoD obtained with an exposure scenario that is closest to the smoking scenario (steps 1 and 2), it supports the identification of the most appropriate dose metric (steps 3 and 4) and it helps to identify the assessment factors needed to bridge differences between the exposure characteristics in the smoking scenario and that of the PoD (steps 7 and 8). Therefore, a transparent description of the parameters involved and how they affect (in case of quantifiable parameters) or might affect (for remaining parameters) the risk evaluation will make the uncertainties in the assessment transparent and will help to identify possibilities for refinement.
Acknowledgements
Dr. T.G. Vermeire and Dr. Y.C.M. Staal from the National Institute for Public Health and the Environment are gratefully acknowledged for critical reading of the manuscript and their valuable comments for improvement.
Disclosure statement
All authors are employees of the National Institute for Public Health and the Environment (RIVM) in the Netherlands and declare no conflict of interest. The authors also have sole responsibility for the writing and content of the paper.
Additional information
Funding
References
- Al-Hamdani M, Hopkins DB, Park T. 2020. Vaping among youth and young adults: a “red alert” state. J Public Health Policy. 41(1):63–69.
- Appelman LM, Woutersen RA, Feron VJ, Hooftman RN, Notten WR. 1986. Effect of variable versus fixed exposure levels on the toxicity of acetaldehyde in rats. J Appl Toxicol. 6(5):331–336.
- Arts JH, Muijser H, Appel MJ, Kuper CF, Bessems JG, Woutersen RA. 2004. Subacute (28-day) toxicity of furfural in Fischer 344 rats: a comparison of the oral and inhalation route. Food Chem Toxicol. 42(9):1389–1399.
- Atherley G. 1985. A critical review of time-weighted average as an index of exposure and dose, and of its key elements. Am Ind Hyg Assoc J. 46(9):481–487.
- Belkebir E, Rousselle C, Duboudin C, Bodin L, Bonvallot N. 2011. Haber's rule duration adjustments should not be used systematically for risk assessment in public health decision-making. Toxicol Lett. 204(2-3):148–155.
- Bos PMJ, Baars BJ, Van Raaij MT. 2004. Risk assessment of peak exposure to genotoxic carcinogens: a pragmatic approach. Toxicol Lett. 151(1):43–50.
- Bos PMJ, Gosens I, Geraets L, Delmaar C, Cassee FR. 2019. Pulmonary toxicity in rats following inhalation exposure to poorly soluble particles: the issue of impaired clearance and the relevance for human health hazard and risk assessment. Regul Toxicol Pharmacol. 109:104498.
- Bunce NJ, Remillard RBJ. 2003. Haber's Rule: The Search for Quantitative Relationships in Toxicology. Hum Ecol Risk Assessment. 9(4):973–985.
- Burns DM, Dybing E, Gray N, Hecht S, Anderson C, Sanner T, O'Connor R, Djordjevic M, Dresler C, Hainaut P, et al. 2008. Mandated lowering of toxicants in cigarette smoke: a description of the World Health Organization TobReg proposal. Tob Control. 17(2):132–141.
- Camacho OM, Eldridge A, Proctor CJ, McAdam K. 2015. Empirical characterisation of ranges of mainstream smoke toxicant yields from contemporary cigarette products using quantile regression methodology. Regul Toxicol Pharmacol. 72(3):458–472.
- CEPA. 1993. Canadian Environmental Protection Act (CEPA) Priority Substances List Assessment Report: Benzene. Available from: http://www.hc-sc.gc.ca/ewh-semt/alt_formats/hecs-sesc/pdf/pubs/contaminants/psl1-lsp1/benzene/benzene-eng.pdf.
- Chen J, Bullen C, Dirks K. 2017. A comparative health risk assessment of electronic cigarettes and conventional cigarettes. IJERPH. 14(4):382.
- Chortyk OT, Schlotzhauer WS. 1973. Studies on the pyrogenesis of tobacco smoke constituents (a review). Beitr Zur Tobackforsch. 7:165–178.
- Chortyk OT, Schlotzhauer WS. 1975. Comparison of pyrolytic products from flue-cured tobacco leaf and a reconstituted tobacco sheet. Beitr Zur Tobackforsch. 8:84–88.
- Connell DW, Yu QJ, Verma V. 2016. Influence of exposure time on toxicity - An overview. Toxicology. 355-356:49–53.
- Counts ME, Morton MJ, Laffoon SW, Cox RH, Lipowicz PJ. 2005. Smoke composition and predicting relationships for international commercial cigarettes smoked with three machine-smoking conditions. Regul Toxicol Pharmacol. 41(3):185–227.
- Cunningham FH, Fiebelkorn S, Johnson M, Meredith C. 2011. A novel application of the Margin of Exposure approach: segregation of tobacco smoke toxicants. Food Chem Toxicol. 49(11):2921–2933.
- Djordjevic MV, Fan J, Ferguson S, Hoffmann D. 1995. Self-regulation of smoking intensity. Smoke yields of the low-nicotine, low-'tar' cigarettes. Carcinogenesis. 16(9):2015–2021.
- Djordjevic MV, Stellman SD, Zang E. 2000. Doses of nicotine and lung carcinogens delivered to cigarette smokers. J Natl Cancer Inst. 92(2):106–111.
- Doull J, Rozman KK. 2000. Using Haber's law to define the margin of exposure. Toxicology. 149(1):1–2.
- ECHA. 2012. European Chemical Agency (ECHA). Guidance on the information requirements and chemical safety assessment. Chapter R.8: Characterisation of dose [concentration]-response for human health. Available from: http://echa.europa.eu/documents/10162/3632/information_requirements_r8_en.pdf
- EFSA. 2005. European Food Safety Authority (EFSA) Opinion of the Scientific Committee on a request from EFSA related to a harmonised approach for risk assessment of substances which are both genotoxic and carcinogenic. (Request No. EFSA-Q-2004-2020). Adopted on 2018 October 2005. Available from: http://www.efsa.europa.eu/en/efsajournal/doc/2282.pdf
- FDA. 1999. US Food and Drug Administration (FDA): Benzene Support Documents: Response to the peer review comments. II. Extrapolation of the Benzene Inhalation Unit Risk Estimate to the Oral Route of Exposure (EPA/NCEA-W-0517, July 1999) http://www.epa.gov/iris/supdocs/benzpr2.html
- FDA. 2012. US Food and Drug Administration (FDA): Harmfull and potentially harmful constituents in tobacco products and tobacco smoke; established list. Available from: http://www.fda.gov/downloads/TobaccoProducts/GuidanceComplianceRegulatoryInformation/UCM297981.pdf
- Felter SP, Conolly RB, Bercu JP, Bolger PM, Boobis AR, Bos PMJ, Carthew P, Doerrer NG, Goodman JI, Harrouk WA, et al. 2011. A proposed framework for assessing risk from less-than-lifetime exposures to carcinogens. Crit Rev Toxicol. 41(6):507–544.
- Feron VJ, Kruysse A, Til HP, Immel HR. 1978. Repeated exposure to acrolein vapour: subacute studies in hamsters, rats and rabbits. Toxicology. 9(1-2):47–57.
- Fowles J, Dybing E. 2003. Application of toxicological risk assessment principles to the chemical constituents of cigarette smoke. Tob Control. 12(4):424–430.
- Gaylor DW. 2000. The use of Haber's law in standard setting and risk assessment. Toxicology. 149(1):17–19.
- Geraets L, Zeilmaker MJ, Bos PMJ. 2018. The importance of inclusion of kinetic information in the extrapolation of high-to-low concentrations for human limit setting. Toxicol Lett. 282:81–92.
- Gordon SM, Wallace LA, Brinkman MC, Callahan PJ, Kenny DV. 2002. Volatile organic compounds as breath biomarkers for active and passive smoking. Environ Health Perspect. 110(7):689–698.
- Gust SW, Pickens RW, Pechacek TF. 1983. Relation of puff volume to other topographical measures of smoking. Addict Behav. 8(2):115–119.
- Haber F. 1924. Zur Geschichte des Gaskrieges. In: Fünf Vorträge aus den Jahren 1920–1923. Berlin, Heidelberg: Springer. p. 76–92. https://doi.org/10.1007/978-3-662-33987-9_5
- HCN. 2014. The Health Council of The Netherlands (HCN). Benzene. Health-based recommended occupational exposure limits. Available from: https://www.healthcouncil.nl/documents/advisory-reports/2014/02/21/benzene-health-based-recommended-occupational-exposure-limit.
- Health-Canada. 2009. Guidelines for Canadian Drinking Water Quality Guideline Technical Document Benzene. http://www.hc-sc.gc.ca/ewh-semt/alt_formats/hecs-sesc/pdf/pubs/water-eau/benzene/benzene-eng.pdf.
- HPA. 2007. Health Protection Agency (HPA) in the United Kingdom. Benzene Toxicological Review. http://www.hpa.org.uk/webc/hpawebfile/hpaweb_c/1194947391801.
- Jarabek AM. 1995. Consideration of temporal toxicity challenges current default assumptions. Inhalation Toxicol. 7(6):927–946.
- Kuempel ED, Sweeney LM, Morris JB, Jarabek AM. 2015. Advances in inhalation dosimetry models and methods for occupational risk assessment and exposure limit derivation. Journal of Occupational and Environmental Hygiene. 12(sup1):S18–S40.
- Lachenmeier DW, Rehm J. 2015. Comparative risk assessment of alcohol, tobacco, cannabis and other illicit drugs using the margin of exposure approach. Sci Rep. 5(1):8126.
- Nomiyama K, Nomiyama H. 1974. Respiratory elimination of organic solvents in man. Benzene, toluene, n-hexane, trichloroethylene, acetone, ethyl acetate and ethyl alcohol. Int Arch Arbeitsmed. 32(1):85–91.
- O'Connor RJ, Hurley PJ. 2008. Existing technologies to reduce specific toxicant emissions in cigarette smoke. Tob Control. 17(Suppl 1):i39–48.
- Pack EC, Jang DY, Kim HS, Lee SH, Kim HY, Song SH, Cho HS, Kwon KH, Park KH, Lim KM, et al. 2018. Mixture risk assessment of selected mainstream cigarette smoke constituents generated from low-yield cigarettes in South Korean smokers. Regul Toxicol Pharmacol. 94:152–162.
- Pack EC, Kim HS, Jang DY, Koo YJ, Yu HH, Lee SH, Lim KM, Choi DW. 2019. Risk assessment of toxicants on WHO TobReg priority list in mainstream cigarette smoke using human-smoked yields of Korean smokers. Environ Res. 169:206–219.
- Pankow JF, Watanabe KH, Toccalino PL, Luo W, Austin DF. 2007. Calculated cancer risks for conventional and “potentially reduced exposure product” cigarettes. Cancer Epidemiol Biomarkers Prev. 16(3):584–592.
- Paschke T, Scherer G, Heller WD. 2002. Effects of ingredients on cigarette smoke composition and biological activity: a literature overview. Contributions Tobacco Res. 20(3):107–244.
- Pauwels CGGM, Boots AW, Visser WF, Pennings JLA, Talhout R, Schooten FV, Opperhuizen A. 2020. Characteristic human individual puffing profiles can generate more tnco than iso and health canada regimes on smoking machine when the same brand is smoked. IJERPH. 17(9):3225.
- Pennings JLA, Cremers JWJM, Becker MJA, Klerx WNM, Talhout R. 2019. Aldehyde and VOC yields in commercial cigarette mainstream smoke are mutually related and depend on the sugar and humectant content in tobacco. Nicotine Tob Res. 22:1748–1756.
- Renne RA, Wehner AP, Greenspan BJ, Deford HS, Ragan HA, Westerberg RB, Buschbom RL, Burger GT, Hayes AW, Suber RL, et al. 1992. 2-Week and 13-Week Inhalation Studies of Aerosolized Glycerol in Rats. Inhal Toxicol. 4(2):95–111.
- Rodgman A, Perfetti TA. 2013. The chemical components of tobacco and tobacco smoke. Second edition. Boca Raton, FL: CRC Press. p. 33487–32742.
- Rozman KK, Doull J. 2000. Dose and time as variables of toxicity. Toxicology. 144(1-3):169–178.
- Rozman KK, Doull J. 2001. The role of time as a quantifiable variable of toxicity and the experimental conditions whn Haber's c x t product can be observed: implications for therapeutics. J Pharmacol Exp Ther. 296(3):663–668.
- Slob W, Soeteman-Hernández L, Bil W, Staal YCM, Stephens WE, Talhout R. 2020. A Method for Comparing the Impact on Carcinogenicity of Tobacco Products: A Case Study on Heated Tobacco Versus Cigarettes. Risk Anal. 40(7):1355–1366.
- Snyder WS, Cook MJ, Nasset ES, Karhausen LR, Parry Howells G, Tipton IH. 1974. Report of the task group on reference man. International commission on radiological protection, No. 23. Pergamon Press. p 344.
- Talhout R, Schulz T, Florek E, Van Benthem J, Wester P, Opperhuizen A. 2011. Hazardous compounds in tobacco smoke. Int J Environ Res Public Health. 8(2):613–628.
- Verhagen H, Feron VJ, Van Vliet PW. 1994. Risk assessment of peak exposure to genotoxic carcinogens. The Hague: Health Council of The Netherlands.(Publication no. A94/04).
- WHO. 2012a. World Health Organization (WHO) (Cardiovascular Diseases (CVD) Fact Sheet No. 317). http://www.who.int/mediacentre/factsheets/fs317/en/
- WHO. 2012b. World Health Organization (WHO) (Chronic Obstructive Pulmonary Disease (COPD) Fact Sheet No. 315). http://www.who.int/mediacentre/factsheets/fs315/en/
- WHO. 2016. World Health Organization (Tobacco Fact Sheet No. 339, May 2012). http://www.who.int/mediacentre/factsheets/fs339/en/
- Wilmer JW, Woutersen RA, Appelman LM, Leeman WR, Feron VJ. 1987. Subacute (4-week) inhalation toxicity study of formaldehyde in male rats: 8-hour intermittent versus 8-hour continuous exposures. J Appl Toxicol. 7(1):15–16.
- Wilmer JW, Woutersen RA, Appelman LM, Leeman WR, Feron VJ. 1989. Subchronic (13-week) inhalation toxicity study of formaldehyde in male rats: 8-hour intermittent versus 8-hour continuous exposures. Toxicol Lett. 47(3):287–293.
- Xie J, Marano KM, Wilson CL, Liu H, Gan H, Xie F, Naufal ZS. 2012. A probabilistic risk assessment approach used to prioritize chemical constituents in mainstream smoke of cigarettes sold in China. Regul Toxicol Pharmacol. 62(2):355–362.