Abstract
Humans will set foot on the Moon again soon. The lunar dust (LD) is potentially reactive and could pose an inhalation hazard to lunar explorers. We elucidated LD toxicity and investigated the toxicological impact of particle surface reactivity (SR) using three LDs, quartz, and TiO2. We first isolated the respirable-size-fraction of an Apollo-14 regolith and ground two coarser samples to produce fine LDs with increased SR. SR measurements of these five respirable-sized dusts, determined by their in-vitro ability to generate hydroxyl radicals (•OH), showed that ground LDs > unground LD ≥ TiO2 ≥ quartz. Rats were each intratracheally instilled with 0, 1, 2.5, or 7.5 mg of a test dust. Toxicity biomarkers and histopathology were assessed up to 13 weeks after the bolus instillation. All dusts caused dose-dependent-increases in pulmonary lesions and toxicity biomarkers. The three LDs, which possessed mineral compositions/properties similar to Arizona volcanic ash, were moderately toxic. Despite a 14-fold •OH difference among these three LDs, their toxicities were indistinguishable. Quartz produced the lowest •OH amount but showed the greatest toxicity. Our results showed no correlation between the toxicity of mineral dusts and their ability to generate free radicals. We also showed that the amounts of oxidants per neutrophil increased with doses, time and the cytotoxicity of the dusts in the lung, which supports our postulation that dust-elicited neutrophilia is the major persistent source of oxidative stress. These results and the discussion of the crucial roles of the short-lived, continuously replenished neutrophils in dust-induced pathogenesis are presented.
Introduction
Human return to the moon for research and exploration
The U.S. presidential space policy directives (NASA Citation2004, Space 2020) have directed NASA to send astronauts back to the Moon for long-duration exploration and research, and subsequently to use the Moon as a stepping-stone to Mars. The NASA Artemis program has charted the manned lunar exploration paths with the first human lunar return mission targeting on 2024. Other spacefaring countries are planning to set foot on the Moon for the first time. The European Space Agency (ESA) has announced plans to build a ‘Moon Village,’ an international lunar research station, by 2030. ESA is advocating ‘Moon 2020–2030’ as a new era of coordinated human and robotic missions to further explore the Moon, particularly on the far-side and unexplored regions (ESA Citation2015; Space News Citation2018). Employing 3 D-printing technology, ESA is proposing to use lunar soil in situ to build the infrastructure of a lunar base (ESA Citation2017; NASA Citation2018). A lunar soil structure could shield humans from solar and cosmic radiation. China is planning to launch the first manned lunar mission by around 2030 (Jones Citation2021). Due to constant solar particle bombardment, the lunar soil, particular on the far side where not reachable by Earth’s magnetic field, has been enriched with helium-3. This nonradioactive helium isotope could be mined and used as a fuel for thermonuclear fusion on Earth to generate safe and clean energy (Schmitt Citation2005; Bilder Citation2009). Mining, construction, and other activities on the Moon will subject the habitants to potential exposures to lunar dust (LD).
Reactive nature of lunar dust and extraterrestrial dusts
The Moon is constantly bombarded by high-speed micrometeoroids. When micrometeoroids (∼50 µm) hit surface rocks, they chip off the rocks to produce particles. When they strike surface regolith (soil), the impacts, which generate temperatures reaching >2000 °C, crush and melt surface particles. The molten particles weld surrounding grains together into glassy aggregates, which are fractured and pulverized to fine dust particles upon subsequent impacts. Cosmic and solar radiation further imparts electrochemical charges to lunar surface material (Taylor and Yang Citation2010). Over the course of four billion years of solar system history, these space-weathering processes have created a thick layer of lunar soil that contains a substantial amount of fine and potentially reactive particles on its surface.
In 2005, Japan’s Hayabusa spacecraft collected some fine dust grains from the asteroid Itokawa. The results of the Itokawa asteroid dust studies led the authors to conclude that the ‘majority of regolith surface particles suffered long-term thermal annealing and subsequent impact shock’ due to ‘constant bombardment by nm- to μm-scale particles’ (Nakamura et al. Citation2011, Citation2012). The mechanism of formation of potentially reactive fine celestial dusts, induced by high-speed micrometeoroid comminution of surface materials, appears to be common on dry and atmosphere-free celestial rocky bodies.
Assessment of the toxicity of lunar dust and the role of reactive oxygen species (ROS) on particle toxicity
As noted above, the Moon is covered by a thick layer of lunar soil that contains an abundance amount of reactive fine dust. To live and work on the Moon, human could be exposed intermittently to potentially reactive fine LD for prolonged periods that could be up to six months (Lam et al. Citation2013). Nonprofessional science articles proclaimed, ‘Moon dust is extremely toxic and poses health hazards for astronauts’ (Vandette Citation2018), and ‘Moon Dust Is Super Toxic to Human Cells’ (Specktor Citation2018). Both these articles were based on the test results of the same cell culture study conducted on samples of an Arizona volcanic ash designated as lunar simulants (Caston et al. Citation2018).
Vandette (Citation2018), in claiming that LD is highly toxic, stated that ‘researchers from Stony Brook University have discovered that compounds in lunar soil react with human cells and can generate extremely toxic hydroxyl radicals which are known to increase the risk of lung cancer.’ It is noteworthy that this Stony Brook’s cell culture study (Caston et al. Citation2018) was conducted on samples of an Arizona volcanic ash that were mined and designated as JSC-1A simulated lunar regolith (soil) (Gustafson Citation2004). These in vitro cell experiments were conducted on JSC-1A (particle size <1000 µm), JSC-1A-sieved (≤63 µm), and JSC-1A-ground (≤7 µm); the results showed that the ground dust (≤7 µm) possessed more ROS and was more toxic (cell death and DNA damage) than the unground dusts (≤63 µm and <1000 µm).
An early batch of this Arizona volcanic ash was mined and designated by NASA as JSC-1, which emulated an Apollo 14 lunar regolith (McKay et al. Citation1994) and was used for various lunar science/technology related tests. JSC-1A (which is considered as a replica/reproduction of JSC-1) were mined later from the same volcano location in Arizona (Gustafson Citation2004). In 2000, Lovelace Respiratory Research Institute (Albuquerque, NM) aerodynamically isolated the respirable-fraction (MMAD ≤5 um) from JSC-1 for our toxicity study. We reported that this simulated lunar respirable dust was much less toxic that quartz in the lung of mice 90 days after being instilled with these dusts (Lam, James, McCluskey et al. Citation2002; Lam, James, Latch et al. Citation2002), and in human lavage lung cell culture (Latch et al Citation2008).
The claims that ‘Moon dust is extremely toxic…’ and ‘Moon dust is super toxic …’ by the authors of popular science magazines was based on the findings that the ground lunar dust simulant contained surface ROS (Caston et al. Citation2018). Others have long proposed that particulate ROS plays a crucial role on the pathogenesis of dusts in the lungs (Donaldson et al. Citation1996). The toxicological impact or role of ROS generated or possessed by dust particles was the subject of the present investigation in conjunction with our NASA-funded studies to assess the toxicity of the authentic LD in the lung of exposed rodents. It is important to have solid data that could be used to assess the true toxicological risk of LD exposures to astronauts living on the Moon. Toxicity data are needed to establish LD exposure limits and to design dust mitigation systems on lunar landers and in lunar habitats to achieve safe exposure environments on the Moon (Lam et al. Citation2013). As stated above, the dust particles on lunar surface are potentially reactive. It is important that the role of surface ROS be critically examined to help understand the mechanism of dust-induced pathogenesis in the lung.
Impact of particle surface reactivity (or ROS) and iron on dust toxicity
Many pulmonary toxicological outcomes of dust exposures could be attributed to oxidative stress (OS) or ROS in the lungs (Mossman et al. Citation2007). Donaldson et al. (Citation1996) proposed that, ‘The ability of particles to generate free radicals at or near their surface, and thereby impose oxidant stress in key target cells, could be central to determining their pathogenicity.’ Fubini (Citation1998) made a similar proposal that ‘surface radicals and iron derived reactive oxygen species (ROS) are implicated in oxidative stress, considered to be the key event in the development of fibrosis and lung cancer.’
The hypothesis put forth by Donaldson et al. (Citation1996), Fubini (Citation1998) and his team (Horwell et al. Citation2003), that particles, particularly those containing iron, generate ROS on the particle surfaces leading to lung toxicity, still have many supporters (Lodovici and Bigagli Citation2011; Shen and Anastasio, Citation2012; Harrington, Schmidt et al. Citation2017). In-line with these proposals, Harrington, Schmidt et al. (Citation2017) postulated that, ‘[one] pathway to inflammation is particle-induced formation of ROS via reactions with surface defects and/or particle-mediated Fenton chemistry … dissolved molecular oxygen is reduced stepwise by dissolved ferrous iron, leading to the formation of superoxide, hydrogen peroxide, and hydroxyl radicals …’ Harrington, McCubbin et al. (Citation2017) subsequently conducted a study sponsored by NASA using ground meteorite dusts to test their postulation.
Dust activation by grinding of aged lunar dust for toxicity evaluation
Results from Vallyathan et al. (Citation1995) showed that freshly fractured silica (by jet-mill grinding) possessed a higher level of free radicals or ROS than the same ground silica that was aged for three months. Therefore, investigators of LD toxicity would typically grind dusts to increase their ROS, subsequently would obtain data from which toxicity of LD on the Moon could be inferred.
Any free radicals or ROS on dust particles at the lunar surface would not be likely to passivate or decay on the airless and waterless Moon. However, some of the lunar soil samples collected during the Apollo program might have become passivated over time due to exposure to trace amounts of air during 50 years of storage (i.e. any ROS originally present on the particles might have disappeared). Thus, grinding LD to ‘activate’ it (or to restore/recreate its surface ROS) would allow assessing its toxicity in a condition that is closer to that of in situ lunar dust. Taking a similar approach as Vallyathan et al. (Citation1995), the lunar geology team of our LD toxicity project ground two LD samples by jet-mill and by zirconia ball-mill to produce LDs with different levels of ROS. Starting with a pristine Apollo 14 lunar regolith (soil), the coarse fractions were aerodynamically removed; the fraction of respirable LD (unground LD or LD-ug) was collected after the fine dust passed through a cyclone particle fractionator. Two aliquots of a coarse dust fraction trapped inside the cyclone from this same parent Apollo 14 regolith were ground by a jet-mill or ball-mill; the respirable fractions of these ground dusts were also aerodynamically isolated and designated as LD-jm or LD-bm, respectively for our toxicity study. As presented in the Methods, all procedures (including all dust sample/aliquot weight measurements) were carried out under ultrapure nitrogen atmosphere in a NASA astromaterial hood/glovebox (Cooper et al. Citation2010; McKay et al. Citation2015).
Pulmonary toxicity studies of lunar dusts in rats
We conducted a study comparing the toxicity of these three respirable LDs with those of aged quartz (Min-U-Sil 5) and TiO2 in the lungs of rats exposed to these test dusts by intratracheal instillation (ITI). The three respirable LDs (LD-ug, LD-jm and LD-bm) used in our ITI study were of comparable size; they were all derived from the same parent Apollo-14 regolith and had the same mineral properties but differed 14-fold in the ROS contents. The ROS levels of the unground lunar dust (LD-ug), aged quartz, and TiO2 were similar, indicating that the levels of ROS of these three aged dusts were at their baseline state. It is noteworthy that Apollo 14 lunar dust contains 7.5% iron oxide with trace level of nanophase metallic iron (Lam et al. Citation2013; McKay et al. Citation2015), while TiO2 and quartz contain only trace levels of iron, if any.
The present study allows us to investigate: (1) the comparative toxicity of lunar dusts with quartz and TiO2 (the two toxicity-reference dusts); (2) the correlation between ROS and the toxicities of these five test dusts; (3) the contribution of particle surface ROS to the overall pulmonary toxicity of three lunar dusts with identical mineral composition but greatly different ROS levels; (4) the comparative toxicity of three unground/unaltered and aged dusts (LD-ug, quartz, and TiO2) with greatly different mineral composition, but comparable ROS contents; and (5) the impact of iron content on the dust toxicity.
Materials and methods
Laboratory animals
Fischer 344 male rats (∼8-10 weeks old at arrival) were purchased from Charles River (Raleigh, NC). The animals ordered for the lung lavage biomarkers study were housed in an AAALAC-approved animal facility at the National Institute for Occupational Safety and Health (NIOSH, Morgantown, WV). Rats ordered for the histopathology study were housed in an AAALAC-approved vivarium at NASA Johnson Space Center (JSC, Houston, TX, USA). Both facilities were set with a 12-h light/dark cycle. The animals lived in pairs in cages with HEPA-filtered air. They had free access to water and standard laboratory rodent chows. NASA and/or NIOSH Institutional Animal Care and Use Committees (IACUCs) approved these studies. The studies followed the IACUC guidelines and approved test protocols.
Stock lunar regolith sample
The respirable lunar dusts for the present investigation was isolated from a prestine lunar regolith sample (14003, 96), which was collected during the Apollo-14 mission. During the Apollo 14 mission, lunar regolith samples were collected from an area of the Moon in which the soils were comprised of mineral constituents from both major geological areas (highland and mare regions) in the Fra Mauro Formation near Cone Crater in the Imbrium Basin, located near the lunar equator. This regolith 14003, 96 sample is a good representatives of fine surface materials on the moon. Because of a good representation, Apollo-14 regolith has been served as a yardstick for deriving lunar soil simulants by NASA and other spacefaring countries. The regolith contains 1-2% fine dust (≤3 µm); amorphous silica (SiO2), aluminum oxide, calcium oxide, iron oxide, magnesium oxide, and titanium dioxide account for 47.9%, 20.4%, 12.1%, 7.5%, 6.8%, and 2.0% of the dust by weight, respectively (McKay et al. Citation1994, Citation2015). Lunar soils generally contain crystalline silica at 0-2%. This low level of crystalline silica in terrestrial dusts is not believed to contribute to the overall toxicity of the test dusts.
Respirable dust preparations and isolation from lunar soil/dust samples
Isolation of the respirable fraction of the pristine/unground lunar regolith sample − An Apollo-14 lunar regolith (∼200 g; stored in nitrogen since it was collected from the Moon) was allocated by NASA Astromaterials Branch for the present study. A fine (respirable) dust fraction (designated LD-ug) was aerodynamically separated from this regolith (McKay et al. Citation2015). The resulting yield of 2.7 g of respirable dust was consistent with previous studies showing that lunar regolith contains 1% to 2% respirable dust. The bulk sample was placed in an in-house-built fluidized bed, in which ultra-pure nitrogen percolated through it from the bottom (Cooper et al. Citation2010; McKay et al. Citation2015). The aerosol stream passed through a cyclone (specially manufactured for rodent studies, CH Technologies, Cherry Hill, NJ) allowing respirable dust to be collected on a Nuclepore filter. The particle size distribution of the collected dust was determined using a Microtrac particle-size analyzer (Montgomeryville, PA). The mass median diameter (MMD) of LD-ug was 2.1 (+0.6/-0.9) µm.
Grinding lunar dust samples and isolating respirable dust – Aliquots of the coarser dust that was captured in the cyclone (described above) were ground using a jet-mill or a zirconia-ball mill (see procedures below). A ground dust sample was then placed in a Vilnius dry dust generator (CH Technologies) that was connected to a tank of nitrogen. A cyclone was connected downstream of the dust generator. After the aerosol stream passed through the cyclone, the respirable dust was collected on a Telfon membrane filter. The particle size distributions were determined by the Microtrac. The MMD of the jet-milled lunar respirable dust (LD-jm) was 2.5 (+1.0/−0.7) µm; the MMD for the ball-milled lunar respirable dust (LD-bm) was 1.8 (+0.5/−0.9) µm (McKay et al. Citation2015).
Grinding lunar dust by ball-mill – A weighed amount (<1 g) of a coarse lunar dust sample and one zirconia ball (dia. 15 mm) were placed inside a 25-ml zirconia jar. The jar was then tightly sealed and placed inside a Retsch MM400 Mixer Mill. The lunar sample was ground for 90 minutes with the grinding/vibratinng frequency set at 30Hz. Respirable dust was then isolated from this ball-milled sample following the procedure discribed above.
Grinding lunar dust by jet-mill – A coarse lunar dust sample was placed in a hopper which fed the dust sample gradually into the grinding chamber of a small jet mill (Open Manifold Micronizer, Sturtevant Inc., Hanover, MA). A high-pressure jet (feed pressure 90 psi) of nitrogen gas was introduced into the chamber meeting the incoming dust and accelerating the lunar particles in a jet-like flow in the vertical loop path inside the chamber (grinder pressure 70 psi). The collision of the high-speed particles in the nitrogen stream resulted in particle breakage and comminution. The centrifuging force selectively allowed the fine dust particles to exit. The collected sample was then used for extracting the respirable dust following the procedure described above.
Preparative procedures associated with the LD, including sample grinding, particle size-separation, and sample/aliquot weighing, were carried out in an airtight and N2-filled hood/glove box at the NASA Astromaterials Laboratory by the lunar-dust geology team. For each animal instillation session, aliquots of respirable dust were weighed out in amounts sufficient for the instillation. All the LD samples were stored in pre-weighed vials. Each vial was then tightly sealed and double-bagged in plastic then heat-sealed while within the nitrogen environment. Lunar samples for the biomarker study were weighed out and also double-bagged at NASA-JSC one or two days before the samples were carried by the principal investigator to NIOSH (Morgantown, VA) where they were used for rat instillation within 5 days. The time between the addition of the vehicle (or air contact with the sample) to a LD sample and the last rat being instilled from this suspended LD sample was less than 2 hours. For each instillation experiment associated with the histopathology studies at Johnson Space Center Toxicology Laboratory, samples were weighed and allocated to the principal investigator one or two days before the LD was administered to the rats.
Quartz dust and TiO2
Quartz dust, a fine-sized crystalline silica (Min-U-Sil 5; MMD 1.6 µm), was obtained by NIOSH from U.S. Silica (Berkeley Springs, WV) many years ago. TiO2 (rutile pigment R-100, consisting of ∼99% TiO2, ∼1% alumina) was a product of DuPont Company (Newark, DE) and a gift from Dr. D. Warheit. The average primary particle size of this dust was about 0.4 µm. Both reference dusts were used without further treatment and were considered aged dusts.
Measurement of hydroxyl radicals
Hydroxyl radicals (•OH) are important ROS and are stable enough to be detected in an aqueous system. Terephthalate is a sensitive probe for •OH (Saran and Summer Citation1999; Wallace et al. Citation2009), though it has been suggested that other ROS may be detected, as well (Turci et al. Citation2015). The test dusts were assessed for chemical and oxidative reactivity by measuring the amount of •OH generated per unit weight of dust in the presence of terephthalate (Wallace et al. Citation2009). Each dust was tested in triplicate by adding an equal amount of dust to a terephthalate solution. The resultant fluorescence, which was proportional to the amount of •OH present, was spectrophotometrically determined. Briefly, disodium terephthalate was dissolved in phosphate-buffered saline to produce a final terephthalate concentration of 10 mM. A 10-mg aliquot of each test dust was added to 2.5 ml terephthalate solution. The mixtures were allowed to react for 30 min. The samples were then filtered using 0.22-μm syringe filters to remove mineral dust particles from the suspension. The fluorescence produced in each filtrate was measured with a Perkin-Elmer LS-50B fluorescence spectrometer, set at an excitation wavelength of 324 nm. For all samples, the fluorescence reading of blank terephthalate solution was subtracted from the filtrate signal, and the net signal was reported.
Intratracheal instillation of dusts
We conducted a pilot ITI study at NIOSH to find out the LD dose that would elicit mild pulmonary responses (neutrophilic inflammation). From these test results, we chose the target doses of 1, 2.5, and 7.5 mg/m3 for the core ITI study projecting the doses would produce no, mild, and moderate pulmonary toxicity.
Because mineral dusts, like TiO2 and quartz, generally are wettable, they are usually suspended in normal saline or buffered saline for ITI studies. However, lunar dust is hydrophobic. When suspended in water, dust particles agglomerate and precipitate rapidly. We found that 10% Survanta® (an artificial lung surfactant and therapeutic agent; Abbott Nutrition, Columbus, Ohio) in normal saline would reduce or prevent agglomeration of lunar dust particles. (It is noteworthy that NIOSH scientists have shown previously that Survanta (10%) produces no inflammatory or pathological effect in rat lungs). Therefore, in this core study, the five test dusts were each suspended in this dispersion medium and briefly (<10 sec) vortexed just before instillation of rats. Each rat was anesthetized with isoflurane and then instilled with 0.4 ml of this dispersion medium containing 0, 1, 2.5, or 7.5 mg of one of the five test dusts, or vehicle-only (control).
Collection of bronchoalveolar lavage fluid samples
To assess biomarkers of toxicity in the bronchoalveolar lavage fluid (BALF), rats were euthanized with an overdose of Sleepaway (containing pentobarbital; Fort Dodge Animal Health, Fort Dodge, IA]) at 1 or 4 wk after the dust instillation. Following NIOSH in-house protocols (Roberts et al. Citation2012), the lung was lavaged three times with 6 ml of phosphate-buffered saline. The first lavage was centrifuged, and its supernatant was used for measuring the acellular BALF biomarkers. The cell pellets of the first and subsequent lavages were combined and suspended in 1 ml of HEPES-buffered solution for assessment of cell numbers and differentials.
Assessment of BALF acellular components
Cell-free fractions of the BALFs were evaluated for biomarkers of toxicity. Albumin in BALF, a marker of air/blood barrier injury, was determined according to a Sigma Diagnostics method utilizing the reaction of albumin with bromocresol green. The reaction product was then measured with a spectrophotometer at 628 nm and quantified against known concentrations of bovine serum albumin. Lactate dehydrogenase (LDH) activity, a marker of cell damage, was determined by measuring the oxidation of lactate coupled to the reduction of NAD + at 340 nm. Measurements were performed with a COBAS® C111 analyzer (Roche Diagnostics, Indianapolis, IN). Concentrations of cytokines, markers of inflammation, were determined with enzyme-linked immunosorbent assay (ELISA) kits that were specifically designed to identify MCP-1, TNF-α, IL-1β, IL-6, and IL-10 (Biosource International, Camarillo, CA). The results of this colorimetric assay were obtained with a Spectramax 250-plate spectrophotometer using Softmax Pro 2.6 software (Molecular Devices Corp., Sunnyvale, CA). All BALF assays followed NIOSH standard protocols (Roberts et al. Citation2012).
Assessment of BAL cells
The total numbers of bronchoalveolar lavage (BAL) cells were counted using a Coulter Counter equipped with a Channelizer (model Zb, Coulter Electronics, Hialeah, FL). Cell differentials were performed by visually counting 300 cells after making Cytospin preparations on microscope slides (Shandon Cytospin II, Shandon Inc., Pittsburgh, PA, USA) and performing Wright-Giemsa staining (Hema-Tec 2000, Bayer Corp., Elkhart, IN, USA). Macrophages and neutrophils accounted for more than 99% of the cells counted. The number of macrophages and neutrophils was obtained by multiplying the total number of cells by the percentage of macrophages or neutrophils, respectively.
Lung collection and histopathological examination
For the histopathological study in rats, 4 or 13 weeks after instillation of the test dusts, each rat was euthanized with a lethal dose of Euthasol® (containing pentobarbital; Abbott, North Chicago, IL). The lung was then fixed in place by allowing neutral formalin (10%) to drip by gravity into the lungs for 10 min or longer through a catheter that was inserted into the trachea. The lungs were then isolated from the chest cavity and placed in the fixative. The left tracheobronchial and parathymic lymph nodes were removed and placed in a histology microcassette. All formalin-fixed tissues were processed and embedded in paraffin by the Department of Pathology and Laboratory Medicine (University of Texas Medical School, Houston), following standard histology tissue preparation procedures. The paraffin-embedded lungs were thin-sectioned coronally and mounted on glass microscope slides according to standard histopathological techniques. Tissue sections were stained with hematoxylin-eosin. For the 13-wk study, a second set of tissue sections were stained with trichrome blue for visualization of connective tissues. The slides were assessed by three pathologists who had extensive experience in pulmonary studies of particulates. The lesion scores for each rat were documented on a scoring sheet or directly into a formatted Excel data sheet; the scores of each rat and each group of rats were compiled and analyzed.
Statistics
Statistical analysis software (Stata 14.1, College Station, TX, USA) was used for analyses. The data were first tested for normality using the Shapiro-Wilk test and for homogeneity of variance (Bartlett’s test, and then Levine’s test), before testing for differences between means. If the data passed these tests, the means of the various treatment groups were tested for differences by one-way analysis of variance (ANOVA). If differences were detected, post hoc testing by the method of Bonferroni was used to identify pairs that differed significantly. If the data were not normally distributed or variance was nonhomogeneous, then the variable was transformed to a normal distribution using lnskew0 (Stata) or the nonparametric Kruskal–Wallis test was performed, and a modified Bonferroni test was used to identify pairs that differed significantly. Statistical significance was established when P < 0.05, in all cases except the modified Bonferroni testing. In that case, the threshold for statistical significance was set at 0.05 divided by the number of pairwise rank sum tests that had been performed after a Kruskal–Wallis
Test had indicated a statistically significant difference between means of the treatment groups ( and ).
Results
Lunar dust samples and toxicological reference dusts
The respirable-sized fraction of the LD dust (LD-ug) was isolated aerodynamically from a pristine Apollo 14 lunar regolith. Two aliquots of the coarser dust from the same starting material were ground by either jet-mill or ball-mill; respirable fractions of the ground dusts (LD-jm and LD-bm, respectively) were similarly isolated. The particle mass median diameters (MMD) for LD-ug, LD-jm, and LD-bm were 2.1, 2.5 and 1.8 µm, respectively. For comparison, quartz dust (Min-U-Sil 5) that was used as reference dust in the present study has a MMD of 1.6 µm. These five test dusts were coded by our NASA lunar geologists in our team as A, B, C, D, and E corresponding to LD-jm, TiO2, LD-ug, quartz, and LD-bm, respectively, for the blind and semi-blind studies.
Measurement of hydroxyl radicals of the test dusts
The hydroxyl radical (•OH) is a very important (if not the most important) ROS in many biological and toxicological processes (Knaapen et al. Citation2004; Cederbaum et al. Citation2017). Using terephthalate as a probe, •OH can be detected by a scavenging/fluorescence assay in liquid media (Wallace et al. Citation2009). We measured the •OH of two ground LDs (LD-jm & LD-bm), and one unground LD (LD-ug) together with aged quartz, and aged TiO2. The results showed that grinding did increase particle SR or ROS level; LD-jm and LD-bm produced, respectively, 1.9- and 14-fold more •OH/mg than LD-ug. Quartz generated the lowest level of •OH/mg dust ().
Table 1. Assessment of surface reactivity of test dusts by measured in vitro generation of hydroxyl free radicals.
Assessment of BALF acellular toxicological biomarkers
The cell-free fractions of BALFs were quantitatively assessed for albumin, cellular enzymes (such as lactate dehydrogenase [LDH]) and proinflammatory cytokines. These biomarkers are indicators for alveolar-capillary integrity, cellular damage, and inflammation, respectively. The values of these toxicological biomarkers increased with a dose-dependent trend in rats 1 and 4 weeks after the dust instillation (). Using a commercial cytokine kit for assessing TNF-α, IL-1β, IL-6, or IL-10 and MCP-1, only MCP-1 (macrophage chemoattractant protein-1) was detected in BALF (). For all measurable BALF biomarkers of toxicity, lungs of quartz exposure showed the greatest increases in values of these biomarkers indicating lung inflammation and injury. The overall pulmonary toxicity of dust exposures was quartz > LDs > TiO2; the toxic potencies of unground and ground LDs were similar.
Figure 1. Changes in acellular biomarkers of toxicity in BALFs from rats 1 and 4 wk after instillation with three lunar dusts, TiO2, and quartz (SiO2). Rats were instilled with 0 (Control), 1.0, 2.5, or 7.5 mg/rat of test dust. Figs. (A) Albumin, (B) LDH, and (C) Macrophage chemoattractant protein-1. Graph labels: LD-jm: jet-mill-ground lunar dust; LD-ug: unground lunar dust; LD-bm: ball-mill-ground lunar dust; lighter color bars: 1 wk; darker color bars: 4 wk. Each bar represents mean ± SD from 6 rats. Statistical analyses of differences between treatment groups are shown in and .
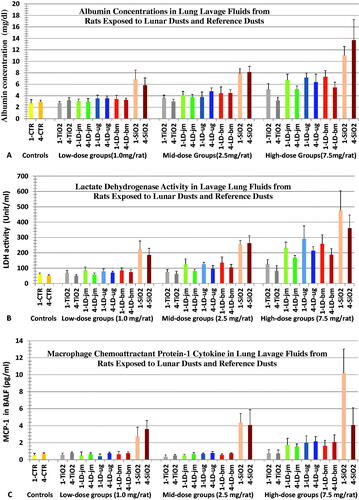
Figure 2. Changes in cell counts in BALFs from rats 1 and 4 wk after instillation with three lunar dusts, TiO2, and quartz (SiO2). Rats were instilled with 0 (Control), 1.0, 2.5, or 7.5 mg/rat of test dust. Figs., (A) total BAL cell counts, (B) macrophage counts, (C) neutrophil counts. Graph labels: LD-jm: jet-mill-ground LD; LD-ug: unground LD; LD-bm: ball-mill-ground LD; lighter color bars: 1 wk; darker color bars: 4 wk. Each bar represents mean ± SD from 6 rats. Statistic differences between treatments are shown in and .
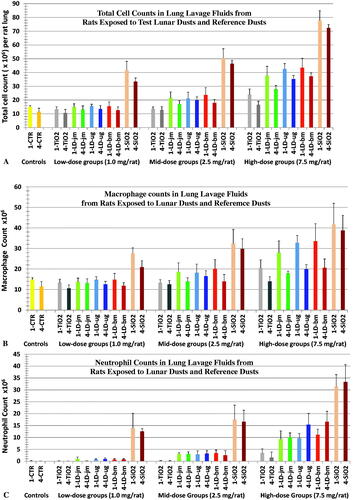
Assessment of BAL cells
The number of cells recovered in the BALF samples from rats 1 and 4 weeks after the dust instillation and their cell differentials were determined are shown in . Overall, neutrophil counts increased in a dose-dependent fashion in rats exposed to lunar dust or quartz. TiO2 was used in the present study as the negative (or low-toxicity) control; increased neutrophils were seen for only the high dose group. Results on neutrophil counts suggest that quartz was the most inflammatory dust, while TiO2 was the least inflammatory. In addition, the inflammatory potency of unground and ground LDs was similar. Neutrophil counts correlated with the histopathology.
Gross lesions in the lungs and lymph nodes (LNs) of dust-exposed rats
Lungs and the LNs (left tracheobronchial and parathymic LNs) were isolated from the rats 4 or 13 weeks after the bolus dust instillation. shows the representative gross lungs and LNs from the 13-wk rat groups treated with the 5 test dusts at the high dose. The lungs of the rats instilled with three LDs were similar (), some superficial nodules were visible; nodules in the lungs of quartz-treated were larger, the lungs appeared lumpy and rough (). Lungs of the TiO2-treated rats were unremarkable (). The LNs of quartz-treated rats were much bigger and firm, the left tracheobronchial LN is visible as a whitish nodule of the size of a peanut in front of the trachea and at center above the lung shown in . LNs of LD-exposed rats (Figure 3(D–F)) were much smaller than those isolated from quartz-treated rats (Figure 3(C)), but bigger than those from the TiO2-treated rats (Figure 3(B)); LNs of control animals were too small and could not be found, except from one animal ().
Figure 3. Lungs and lymph nodes (left tracheobronchial and parathymic lymph nodes). These tissues were isolated from rats of the high-dosed groups 13 wk after the dust instillation. Groups of 6 rats were each instilled with vehicle containing no dust (Controls, (A)), or 7.5 mg of TiO2 (B), quartz (C), LD-jm (Moon dust A), LD-ug (Moon Dust C) or LD-bm (Moon dust E). Lungs of TiO2-treated rats were unremarkable (B). The lungs of rats instilled with three LDs were similar (D–F), some superficial whitish nodules were visible. Nodules in the lungs of quartz-treated rats were larger; the lungs were lumpy and rough (C). The lymph nodes (LNs) of quartz-treated rats were much bigger and firm, the left tracheobronchial LN is visible as a translucent lump of the size a peanut front of the trachea and at the center above the lung in (C). LNs isolated from LD-exposed rats were smaller (grayish LD particles inside the LNs made the LNs appeared darker) (D–F); LNs of control animals were too small and could not be found, except from one animal (A).
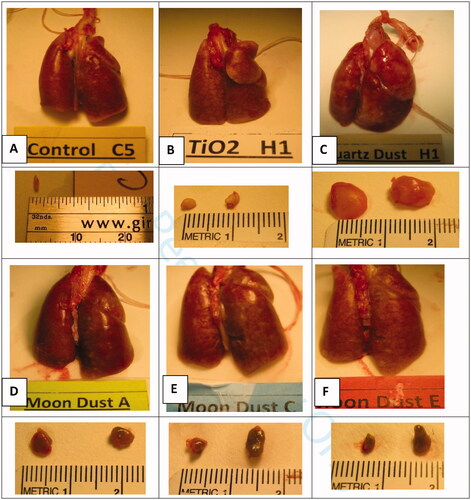
Microscopic lesions in the lungs and lymph nodes (LNs) of dust-exposed rats
The lung and the LN tissues of rats 4 and 13 weeks after dust treatment were microscopically examined by three pathologists. Representative images of lesions in rats treated with quartz, TiO2, and LD-ug are shown in . The three LDs produced comparable lesions at the same given ITI dose. only shows the slide images from rats treated with the unground/unaltered LD (LD-ug). In the LD-exposed rats, particles in the lungs were detected as individual particles or multifocal aggregates. The lung of the low-dose LD group had very few dust aggregates (Figure 4(B)); dust aggregates increased with the treatment dose (). Majority of these visible particles were found in macrophages. Neutrophils and lymphocytes were observed free in alveoli or around aggregates of particle-laden macrophages (PLMs) (). Granulomatous nodules containing closely packed, dense macrophages surrounded by inflammatory cells () were observed in the high-dose rats treated with any of the three LDs. Some thickening of alveolar septa was associated with the inflammatory response; very low-grade fibrosis was detected in or around granulomas in the majority of the high-dose LD groups. Perivascular lymphocytic infiltration (vasculitis) and peribronchiolar neutrophilic infiltration were also observed (). In LNs of the high-dose LD-ug rats, aggregates of PLMs in medullary sinuses probably led to granulomas and lymphoid hyperplasia (); mild to moderate lymphoid hyperplasia was present. The effects generally were milder in the mid-dose groups, and minimal in the low-dose group. The three LD preparations produced similar lung histopathology.
Figure 4. Lung and lymph node tissues 13 weeks after intratracheal instillation with unground lunar dust (LD-ug) and reference dusts (TiO2, and quartz). (A) Control rat: normal lung structure; (B) LD-ug, low dose: some particles in macrophages, no lesions; (C) LD-ug, mid dose: a gathering of dust particles, macrophages, and inflammatory cells in alveoli, some alveolar walls were thicker than those of more normal adjacent alveoli; (D) LD-ug, high dose (hd): a low-grade granuloma (microscopic nodule) consisting of PLMs, inflammatory cells, and thickened alveolar septa. (E) LD-ug, hd: an accumulation predominately composed of lymphocytes and particle-laden macrophages with some neutrophils visible around a blood vessel, characterized as vasculitis; (F) LD-ug, hd: low-grade granulomas (pink areas) containing fine LD grains mostly inside macrophages among lymphoid cells (in blue) in a mediastinal lymph node with no/minimal fibrosis, (G) TiO2, hd: showed TiO2 particles, minimal or no lesions; (H) Quartz, hd: Many large granulomas with moderate fibrosis, severe vasculitis; (I) Quartz, hd: A large structureless granuloma with diffuse and moderate fibrosis in a mediastinal lymph node (Magnifications: 10× to 50×.).
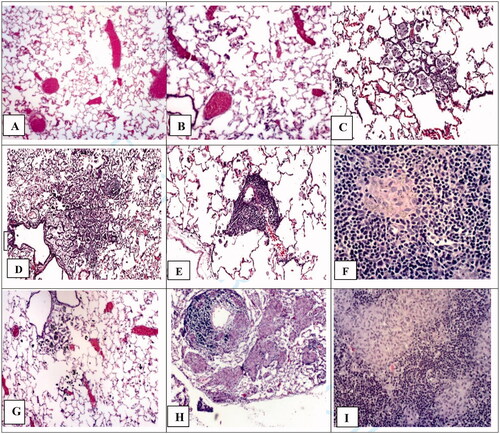
The lung of TiO2 exposed rats had aggregates of particles, but minimal lesions (). The toxicological response to instilled TiO2 was less than the response to LDs. In the quartz-exposed rats, alveolar septal thickening and fibrosis were most evident, and fibrotic lesions and granulomas were larger and most severe (). LNs from the high-dose quartz group consisted of confluent foci of PLMs in medullary sinuses. The foci were moderately fibrotic with marked accumulation of granulomas/nodules ().
The histopathology scores of lungs of the dust-treated rats were tabulated in and , which show that the lesions were dose-dependent in the lungs of rats exposed to quartz and LDs. It is noteworthy that the results of scores of fibrosis in lung interstitial area stained by typical hematoxylin and eosin histology dyes () and the results of the scores of the same tissue/area stained with trichrome blue dyes to visualize collagen/connective tissue for fibrosis detection () were very similar.
Figure 5. Histopathological responses of different tissues in the lungs of dust-instilled rats 13 weeks after instilled with 1.0 (L), 2.5 (M), or 7.5 (H) mg/rat of LD-jm (jet-mill ground), LD-ug (unground), LD-bm (ball-mill ground), TiO2, or quartz. Lesion scoring scale: 0 = none/normal, 1 = minimal, 2 = mild, 3= moderate, 4= marked, 5 = severe (two major effects in each pulmonary tissue area are shown in the figure). Each bar represents mean ± SD from 6 rats.
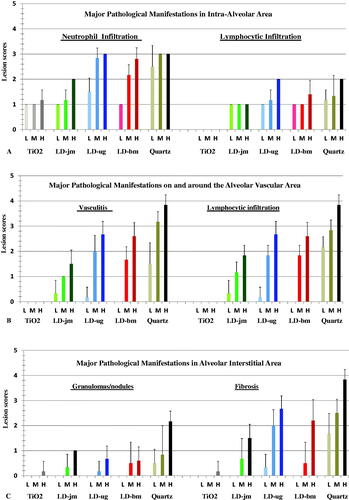
Figure 6. Histopathological responses in the lungs and lymph nodes of dust-instilled rats 13 weeks after instilled with 1.0 (L), 2.5 (M), or 7.5 (H) mg/rat of LD-jm (jet-mill ground), LD-ug (unground), LD-bm (ball-mill ground), TiO2, or quartz. Lesion scoring scale: 0 = none/normal, 1 = minimal, 2 = mild, 3= moderate, 4= marked, 5 = severe (two major effects in each pulmonary tissue area are shown in the figure). Each bar represents mean ± SD from 6 rats.
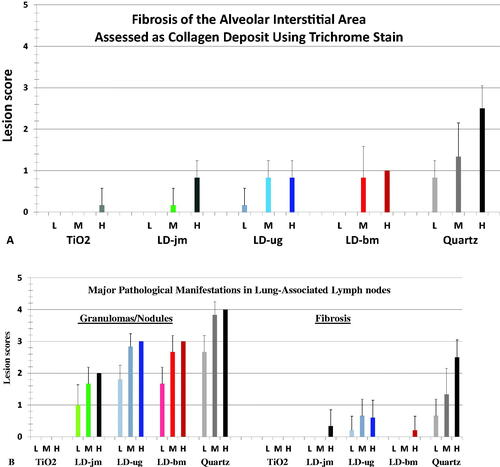
In general, the lesions in the lungs of the 13-wk groups were more focal and severe than those in the 4-wk groups. Overall, the pathologic potency of the dusts was quartz > LDs > TiO2; one of the pathologists concluded, ‘the inflammatory response to instilled quartz was noticeably greater than any other dust, while the response to titanium dioxide was less than any of the Moon dusts…. The inflammatory responses to the three Moon dusts were somewhat similar, with no clear difference in overall response.’
Discussion
Comparative toxicities of lunar dusts and reference dusts
As mentioned in the Introduction, the Moon has been bombarded by high-speed micrometeoroids for more than four billion years. The bombardment has pulverized much of the lunar top surface regolith yielding an abundance of potentially reactive fine dust. The lunar soil samples collected during the Apollo missions might have lost some or all of their surface reactivities because of the presence of trace amounts of air over the 50 years of storage. Therefore, the geologists in our LD toxicity study project first isolated the respirable-size fraction (LD-ug) from an Apollo-14 lunar soil, then ground two coarser samples to restore or increase their surface reactivity or ROS levels. We then toxicologically compared these ground LDs (LD-jm and LD-bm) with unground LD (LD-ug); TiO2 and quartz served as toxicological reference dusts. These five respirable-sized dusts were coded by the geology team as A, B, C, D, and E. The geologist team carried out all procedures including dust grinding, respirable-size dust isolation, and aliquot weighing of the LD samples (for toxicity studies) inside the N2-filled hood at the NASA Astromaterial Laboratory. The biomarker study team was blind to the codes of all five dusts; the pathologists were blind to the identity of the codes of the 3 LDs during the experiment studies.
All of these five respirable-sized dusts caused dose-dependent increases in pulmonary lesions and the magnitudes of BALF biomarkers of toxicity. The three Apollo-14 LD preparations were moderately toxic; they were all less toxic than quartz but more toxic than TiO2. The toxicity of these three LDs was comparable () indicating that grinding did not alter the toxicity of LDs, despite of 14-fold difference in ROS levels among these three LDs. It can be concluded that, for toxicity studies of extraterrestrial or meteorite dusts, grinding will help to obtain respirable-size dust and enhance the contents of particle surface ROS. However, the increase in particle surface ROS content will not contribute significantly to the dust toxicity in the lung (as shown in our present study) due to the fact that ROS are short-lived in biological media (like lung fluid, see discussion below).
NASA’s uses of the results of the present dust ITI study in rats
The LD dose-dependent pulmonary effects observed in this ITI study allowed us to use this information to calculate and choose the proper airborne LD exposure concentrations for the follow-up nose-only inhalation rat study (Lam et al. Citation2013). We then used the results of our LD inhalation study to estimate the permissible exposure limit (PEL) by traditional no-observable-adverse-effect-level approach (Lam et al. Citation2013), and by the bench-mark-dose (BMD) model (Scully et al. Citation2013). The results of the BALF biomarkers in our current comparative toxicity ITI study on these five test dusts allowed us to estimate a PEL for LD using OSHA PELs for TiO2 and quartz as references (James et al. Citation2014). All three estimated PELs are close to each other (James et al. Citation2013); the data were presented to NASA Headquarters for establishing an official NASA PEL on LD for lunar explorers.
No correlation between particles’ surface ROS and pulmonary toxicity in dust-exposed rats
It is well established that oxidative stress (OS) in the lung is the crucial determining factor in dust-induced pulmonary diseases. The issue of whether particles possessing higher levels of surface ROS are more toxic remains unsettled. Our 5-dust study allowed us to test the long-accepted hypothesis that particle-generated ROS have great impact on the overall toxicity of the dust (Donaldson et al. Citation1996). Grinding mineral dusts increases the surface free radicals or ROS of the particles. Vallyathan et al. (Citation1995) reported that a freshly jet-milled quartz dust (Qz-jm) generated •OH/mg at 1.3 times more than that produced by the same Qz-jm that aged for 2 months after grinding. Our results showed that, with respect to the dust’s ROS content (•OH/mg), the jet-milled LD (LD-jm) processed 1.9 times more than unground LD (LD-ug); the zirconia ball-milled LD (LD-bm [MMD: 1.8 µm]) processed 14 times more than LD-ug (MMD: 2.1 µm) (). These data illustrated that, despite the great differences in surface content of •OH varying by 14 fold, these three respirable LD preparations with the exact same mineral properties and of comparable particle size distributions exhibited comparable pulmonary toxicity (; ). LD-ug, quartz, and TiO2 were all unground and aged dusts, and possessed greatly different mineral properties but comparable levels of •OH (); they elicited great differences in lung toxicity. The overall results of BALF biomarkers of toxicity and pulmonary histopathology indicated that quartz, which generated the least amount of •OH/mg, was the most toxic; TiO2 was the least toxic. Therefore, our data show that the dusts’ intrinsic mineral properties or physicochemistry, rather than an ability to produce short-lived ROS (represented by and measured as •OH), appears to account for the differences in the relative toxicities of the tested dusts. These results are consistent with those of Vallyathan (Citation1994) who reported that quartz (aged) generated the least amount of •OH/mg in vitro and had the lowest iron content among 6 mineral dusts tested, but it was the most toxic.
Table 2. Statistical comparison of BALF toxicity biomarker induction by lunar dusts, quartz and TiO2.
Table 3. Statistical comparison of BALF inflammatory biomarker induction by three lunar dusts.
Mice exposed to meteorite dusts in a NASA-sponsored study by Harrington et al
It is well known that iron in the soluble form of ferrous ion (Fe++) can react with hydrogen peroxide to form •OH. Fubini (Citation1998) and Horwell et al. (Citation2003) postulated that iron on the dust particles, involving in Fenton reaction, produced ROS inside the lung resulting in toxicity. In-line with this proposal, Harrington, Schmidt et al. (Citation2017) of the geology group at Stone Brook University published a paper further advocating that ROS produced by particles, particularly those containing iron, are the major determinant of the toxicity. After joining NASA, Harrington conducted a study to test her postulation and the hypothesis that was put forth by Fubini (Citation1998) and Horwell et al. (Citation2003). Subsequently, Harrington, McCubbin et al. (Citation2017) conducted a mouse study on seven ground dusts to assess (1) the correlation of the dust surface ROS and pulmonary toxicity and, (2) the correlation of iron levels of the dusts and pulmonary toxicity. These respirable-sized test dusts were prepared (grinding under nitrogen) from six meteorites (from Mars, the Moon, and the Vesta asteroid) and a terrestrial basalt. This study was collaborated with the toxicology laboratory of NYU School of Medicine mainly to support NASA manned space missions to the Moon and Mars. The soluble iron contents and ROS generation in vitro (the authors termed as ‘geochemical reactivity’) of these ground dusts were measured. Pulmonary toxicity was assessed by measuring neutrophilic infiltration in the lung of mice intratracheally instilled with these dusts. The results showed that the terrestrial basalt, which was one of the two dusts that had the highest soluble iron and generated the highest ROS, produced the lowest pulmonary toxicity. The authors concluded that ‘The MORB [terrestrial basalt] demonstrated higher geochemical reactivity [i.e. soluble iron & ROS levels] than most of the meteorite samples but caused the lowest acute pulmonary inflammation (API)… there is no direct correlation between a particle’s ability to generate ROS acellularly and its ability to generate API.’ (Harrington, McCubbin et al. Citation2017). Harrington’s results and conclusion are consistent with those of our lunar dust study showing that surface ROS of the particles has no impact to the dust’s toxicity in the lung.
Particles’ ROS not correlated with the cytotoxicity and genotoxicity in cells exposed to lunar soil simulants in cultures
To help NASA understanding the toxicity of LD, Caston et al. (Citation2018) carried out a study on LD dust simulants, which were ground, to show ROS generated/possessed by particles is crucial to the toxicity. The results of this in vitro study led Caston et al. (Citation2018) to conclude that, ‘we present data showing the exposure to lunar soil simulants is cytotoxic and DNA damaging in both the neuronal and lung cell lines…. However, rather unexpectedly, the ability of the simulants to produce ROS in the surrounding solution was not correlated with the observed cytotoxic or genotoxic effects.’
Impact of dust’s iron content on its toxicity in the lung
It is noteworthy that NASA Martian exploration missions found that the soil samples at the three landing sites contained iron oxide at 16.5% or higher (Allen et al. 1998); The Apollo-14 LD dust used in our present study contains 7.5% iron oxide. One of the objectives of study by Harrington, McCubbin et al. (Citation2017) on the ground dusts of the Martian and lunar meteorites was to show a correlation between iron in the test dust samples and pulmonary toxicity. As mentioned above, the Harrington found no correlation. Our present study of LD (7.5% iron oxide and low level of nanophase metallic iron) and quartz (Min-U-sil 5; contains <0.1% iron (IARC Citation1997)) showing quartz is much more toxic than LDs suggesting that the toxicological role of iron is insignificant. Vallyathan (Citation1994) drew the similar conclusion that ROS and iron contents were not correlated to the toxicity of the tested dusts based on the study on six mineral dusts showing that quartz was the most toxic but contained the least amount of iron.
The results of a 4-week rat inhalation study of siderite (FeCO3: 12 and 38 mg/m3) and magnetite (Fe3O4: 22 and 66 mg/m3) with pulmonary toxicological assessments 3 months thereafter led Pauluhn and Wieman (Citation2011) to conclude that ‘the pulmonary effects were due to inflammation induced by dust overloading and did not correlate with iron content. The authors further concluded that ‘as far as evidence of oxidative stress existed, it appears to be causally related to the extent of particle-induced inflammation (PMNs) and not iron.’
The particles’ short-lived ROS could not account for the long-term pathogenesis of dust in the lung
Vallyathan et al. (Citation1988) reported that the half-life of ROS generated by freshly-milled quartz (Qz-jm) was ∼30 h in the air and only a few minutes when the dust was placed in phosphate-buffered saline. The half-life of particle-generated ROS would be expected to be even shorter in the presence of lung surfactant, antioxidants, and antioxidative enzymes. It is noteworthy that a single instillation of quartz (Min-U-Sil 5, not freshly ground) at 20 mg/rat resulted in lung cancer with a cumulative cancer incidence of 30/67 (controls: 1/75) when the animals were examined up to 22 months after the bolus instillation (Groth et al. Citation1986). Surface ROS of quartz would have no role in the observed chronic pathogenesis. Furthermore, particle-generated ROS also cannot explain why dusts in the lung cause severe lesions and/or cancer in rats but not in hamsters chronically exposed to same dust concentrations (Bermudez et al. Citation2002, Citation2004). Bermudez reported that, exposed to the same dust (TiO2), at the same concentration and for the same duration, a rat had many more alveolar neutrophils than a hamster had. Carter and Driscoll (Citation2001) reported that, when exposed to the same quartz dose, the content of oxidants (ROS and reactive nitrogen species) in a neutrophil in a rat was several times higher than that in a hamster.
The persistent presence of short-lived neutrophils from dust-induced inflammation provides a continuous source of ROS in the lung of exposed animals
Persistent oxidative stress is known to be the crucial factor leading to dust-induced lung diseases. The conclusion of Pauluhn and Wieman (Citation2011) that oxidative stress is attributed to neutrophilic inflammation is consistent with the observations of Morrow et al. (Citation1996) who pointed out that that particle-induced lung cancer was linked to persistent [neutrophilic] inflammation. Driscoll et al. (Citation1997) was the first group to show that oxidants from inflammatory cells in the lung, particularly neutrophils, were responsible for mutations in alveolar epithelium cells (AECs) in dust-exposed rats. In this study, Driscoll et al. reported dose-dependent and dust-dependent increases in mutation frequency (mf) in type II AECs obtained from rats 15 months after bolus instillation with these three test dusts (mf: Qz > CB > TiO2). Co-culture of bronchoalveolar lavage cells (BALCs) harvested from the lungs of these dust-treated rats with naïve RLE6TN cells ((Type II AECs) in vitro led to mutation in RLE6TN cells. Neutrophil-enriched BALCs (82% PMNs) were much more mutagenic than macrophage-enriched BALCs (85% macrophage). The presence of catalase in the co-culture prevented these mutations. Driscoll et al. attributed the increased mf in AECs chiefly to the oxidants from the BALCs, particularly the neutrophils. However, no increase in mf in rat lung RLE6TN cells was observed when these Type II AECs were incubated with quartz, carbon black, and TiO2.
The amounts of oxidants in neutrophils isolated from rats depending on the exposure dusts and concentrations
The data of Driscoll et al. (Citation1997), discussed in the preceding paragraph, showed that the dust- and dose-dependent increases of mf (i.e. mf: Qz > CB > TiO2) in Type II cells were caused by oxidants predominately from alveolar neutrophils of the dust exposed rats. It can be calculated from the data that oxidant level per neutrophil and the total oxidants of all the neutrophils depending on the cytotoxicity of the test dusts, that is Qz > CB > TiO2. In our 5-dust ITI study, we also observed dust-dependent and dose-dependent increases in number (η) of BAL neutrophils, oxidants per neutrophil [Ox]n, and total oxidants in all the BAL neutrophils (= Σ(Ox) = η × [Ox]n). In other words, (η): Qz > LD > TiO2; [Ox]n: Qz > LD > TiO2; and Σ(Ox): Qz > LD > TiO2. The oxidant levels for neutrophils [Ox]n and Σ(Ox) for the three LDs were comparable (Lam et al. In preparation). In our follow-up 4-week LD inhalation study with lung BAL cells (leukocytes) assessment on 1-d, 1, 4 and 13 wk after the LD exposure, we observed exposure concentration-dependent and postexposure time-dependent increases in oxidant level per neutrophil [Ox]n or total neutrophilic oxidants Σ(Ox). The Σ(Ox) correlated well with the lung histopathology (Lam et al. In preparation). It is well known that chronic exposures to dusts result in persistent neutrophilia in the lung. Neutrophils are recruited and activated (to increase oxidants) by cytokines that are released by the dust-afflicted alveolar epithelial cells and macrophages in the lungs of dust-exposed animals. Because neutrophils are short-lived (half-life a few hours), the dead ones are being replenished or replaced by incoming neutrophils. The unphagocytized apoptotic neutrophils would undergo secondary necrosis that would release their oxidants and proteolytic enzymes damaging local tissue (Robinson Citation1998; Zhang et al. Citation2002; Liu et al. Citation2017; Rydell-Törmänen et al. Citation2006; Silva et al. Citation2008). The persistent neutrophilic inflammation is the major source of continuous oxidative stress in the lung. In other words, the total neutrophilic oxidant (Σ(Ox) = η × [Ox]n) would reflect the extent of oxidative stress in the lung of a dust-exposed animal. This postulation is consistent with the findings of Pauluhn and Wieman (2011), as noted above that oxidative stress appeared to be causally related to the extent of particle-induced inflammation (PMNs). The crucial roles of neutrophils and neutrophil-derived oxidants in lung toxicity are described in detail in our review paper, which presents a general mechanism for the pathogenesis of particle-induced diseases (Lam et al. In preparation).
Disclaimer
The findings and conclusions in this report are those of the authors and do not necessarily represent the views of the NASA, NIOSH, or the University of Texas Medical Center at Houston.
Acronyms | ||
JSC | = | NASA Johnson Space Center |
NASA | = | National Aeronautics and Space Administration |
NIOSH | = | National Institute for Occupational Safety and Health |
OSHA | = | Occupational Safety and Health Administration |
AEC | = | Alveolar epithelium cell |
BALC | = | Bronchoalveolar lavage cell |
BALF | = | Bronchoalveolar lavage fluid |
BMD | = | Bench-mark-dose |
ITI | = | Intratracheal instillation |
JSC-1 | = | Simulated lunar regolith (an Arizona volcanic ash, designated by JSC Geology Team) |
JAC-1A | = | Simulated lunar regolith, replica of JSC-1 |
LD | = | Lunar dust |
LD-bm | = | Lunar dust, zirconia ball-milled, respirable size |
LD-jm | = | Lunar dust, jet-milled, respirable size |
LD-ug | = | Lunar dust, unground, respirable size |
MMD | = | Mass medium diameter |
MMAD | = | Mass medium aerodynamic diameter |
•OH | = | hydroxyl radicals |
PEL | = | Permissible exposure limit |
PMN | = | Polymorphonuclear leukocyte or Neutrophil |
Qz | = | Quartz |
Qz-jm | = | Quartz ground by jet mill |
ROS | = | Reactive oxygen species |
TiO2 | = | Titanium dioxide |
Acknowledgments
The present study and related toxicological studies were overseen and reviewed by the NASA-assembled Lunar Airborne Dust Toxicity Assessment Group (LADTAG) and the Non-Advocate Review Committee (NARC) consisting of national experts in toxicology, environmental science, and lunar geology. The authors thank the members of the LADTAG and NARC for their in-depth technical review, valuable advice, and research guidance on the lunar dust toxicity studies. The authors gratefully acknowledge the NASA Astromaterial Samples Curator for allocating an Apollo-14 lunar regolith sample, from which the NASA lunar geology team isolated the respirable lunar dusts for our animal toxicity studies. Technical assistance and contribution from S. Bassett, C. Gonzalez, and staff of the NIOSH Health Effects Laboratory Division (Morgantown, WV), NASA-JSC Clinical Laboratory, and Histology Laboratory of the University of Texas Medical Center (Houston) are also gratefully acknowledged. The authors also thank J. Krauhs, H. Garcia, and A. Romoser for editorial assistance and May-Ying Lam for graphic work. The senior author would like to dedicate this paper to Dr. Donald E. Gardner, who was a NASA-appointed member of the LADTAG. We specially thank Dr. Gardner for his valuable advice to these lunar dust toxicity studies. He helped guiding our conduct of these highly important and technical difficult lunar dust toxicity studies, particularly the lunar dust inhalation study (Lam et al., 2013), to successes. This was not his only great contribution to NASA; Dr. Gardner chaired, for many years, the National Research Council’s Subcommittee on Spacecraft Maximum Allowable Concentrations for helping NASA to establish exposure limits on spacecraft airborne and waterborne contaminants. In 2008, NASA awarded Dr. Gardner an Outstanding Public Service Award for his great contribution to the well-being and health of space crew. Gardner was the founder, and former and late Editor in Chief for Inhalation Toxicology. This senior author thanks him for publishing several our peer-reviewed articles in Inhalation Toxicology, and for the privilege as an invited peer-reviewer for other’s articles submitted to in this journal.
Disclosure statement
The authors have declared no financial conflicts of interest in conducting this lunar dust toxicity research or the presentation of the study results in the current manuscript.
Additional information
Funding
References
- Alien C, Morris RV., Jager KM, Golden, DC, Lindstrom DJ, Lindstrom MM, and Lockwood JP. 1998. JSC Mars-1: A Martian soil simulant. Space 98 Proceedings of the Conference of American Society of Civil Engineers; 26–30 April, Albuquerque, NM. Reston, VA: ASCE. p. 469–475.
- Bermudez E, Mangum JB, Asgharian B, Wong BA, Reverdy EE, Janszen DB, Hext PM, Warheit DB, Everitt JI. 2002. Long-term pulmonary responses of three laboratory rodent species to subchronic inhalation of pigmentary titanium dioxide particles. Toxicol Sci. 70(1):86–97.
- Bermudez E, Mangum JB, Wong BA, Asgharian B, Hext PM, Warheit DB, Everitt JI. 2004. Pulmonary responses of mice, rats, and hamsters to subchronic inhalation of ultrafine titanium dioxide particles. Toxicol Sci. 77(2):347–357.
- Bilder RB. 2009. A legal regime for the mining of helium-3 on the moon: U.S. policy options. Fordham. Int. Law J. 33:243–298.
- Carter JM, Driscoll KE. 2001. The role of inflammation, oxidative stress, and proliferation in silica-induced lung disease: a species comparison. J Environ Pathol Toxicol Oncol. 20(Suppl 1):12–43.
- Caston R, Luc K, Hendrix D, Hurowitz JA, Demple B. 2018. Assessing toxicity and nuclear and mitochondrial DNA damage caused by exposure of mammalian cells to lunar regolith simulants. Geohealth. 2 (4):139–148.
- Cederbaum AI. 2017. Cytochrome P450 and oxidative stress in the liver. in: liver pathophysiology therapies and antioxidants chapter 31, Ed.: P Muriel. New York, NY: Elsevier Inc. https://www.sciencedirect.com/science/article/pii/B978012804274800031X
- Cooper BL, McKay DS, Taylor LA, Kawamoto H, Riofrio LM, Gonzalez CP. 2010. Extracting respirable particles from lunar regolith for toxicology studies. In Proceedings of the 12th International Conference on Engineering, Science, Construction, and Operations in Challenging Environments. 12:66–73.
- Driscoll KE, Deyo LC, Carter JM, Howard BW, Hassenbein DG, Bertram TA. 1997. Effects of particle exposure and particle-elicited inflammatory cells on mutation in rat alveolar epithelial cells. Carcinogenesis. 18(2):423–430.
- Donaldson K, Beswick PH, Gilmour PS. 1996. Free radical activity associated with the surface of particles: a unifying factor in determining biological activity? Toxicol Lett. 88(1-3):293–298.
- ESA 2015. International symposium on moon 2020–2030, a new era of coordinated human and robotic exploration. European Space Research and Technology Centre of the European Space Agency (ESA/ESTEC) in the Netherlands. December 15–16. http://spaceflight.esa.int/humanrobotics/.
- ESA 2017. Lunar 3D printing. [Accessed June 2017]. http://m.esa.int/Highlights/Lunar_3D_printing
- Fubini B. 1998. Surface chemistry and quartz hazard. Ann Occup Hyg. 42(8):521–530.
- Groth DH, Stettler LE, Platek SF, Lal JB, Burg JR. 1986. Lung tumors in rats treated with quartz by intratracheal instillation. In: Goldsmith DF, Winn DM, Shy CM, eds. Silica, silicosis, and cancer: Controversy in occupational medicine. New York, NY: Praeger Publishers, p. 243–253. Cancer Research Monographs, Vol. 2.
- Gustafson R. 2004. JSC‐1A lunar regolith simulant availability and characterization. https://www.nasa.gov/sites/default/files/atoms/files/03_jsc-1a_lunar_regsimulant_update_bgustafson.pdf
- Harrington AD, Schmidt MP, Szema AM, Galdanes K, Tsirka SE, Gordon T, Schoonen MAA. 2017. The role of Iraqi dust in inducing lung injury in United States soldiers-An interdisciplinary study: Iraqi Dust and U. Geohealth. 1(5):237–246.
- Harrington AD, McCubbin FM, Kaur J, Smirnov A, Galdanes K, Schoonen MA, Chen LC, Tsirka SE, Gordon T. 2017. Acute meteorite dust exposure and pulmonary inflammation – implications for human space exploration. Lunar and Planetary Science XLVIII 2017. 2922. https://www.hou.usra.edu/meetings/lpsc2017/pdf/2922.pdf.
- Horwell CJ, Fenoglio I, Ragnarsdottir KV, Sparks RSJ, Fubini B. 2003. Surface reactivity of volcanic ash from the eruption of Soufrière Hills volcano, Montserrat, West Indies with implications for health hazards. Environ Res. 93(2):202–215.
- IARC 1997. Silica, some silicates, coal dust and para-aramid fibrils. IARC monographs on the evaluation of carcinogenic risks to humans, No. 68. International Agency for Research on Cancer; 1997. https://www.ncbi.nlm.nih.gov/books/NBK410038.
- James JT, Lam C-W, Santana PA, Scully RR. 2013. Estimate of safe human exposure levels for lunar dust based on comparative benchmark dose modeling. Inhal Toxicol. 25(5):243–256.
- James JT, Lam C-w, Scully RR, Meyers VE, McCoy JT. 2014. Lunar dust toxicity: final report (NASA On-line Report). https://humanresearchroadmap.nasa.gov/gaps/closureDocumentation/Lunar%20Dust%20Toxicity%20FINAL%20REPORT.pdf?rnd=0.157545329144944.
- Jones A. 2021. China displays crewed moon landing mission elements. SpaceNews. https://spacenews.com/china-displays-crewed-moon-landing-mission-elements
- Knaapen AM1, Borm PJ, Albrecht C, Schins RP. 2004. Inhaled particles and lung cancer. Part A: mechanisms. Int J Cancer. 109(6):799–809.
- Lam C-w, Driscoll KE, McClellan R, James JT, Zhang Y, Scully RR, Ryder V, Hunter R, McCluskey R, Zeidler-Erdely PC, Castranova V. (In preparation). Comparative Toxicities of Lunar Dusts and Terrestrial Dusts (Quartz & TiO2) and a Proposed Mechanism for the Particle-Induced Lung Diseases.
- Lam C-w, Scully RR, Zhang Y, Renne RA, Hunter RL, McCluskey RA, Chen BT, Castranova V, Driscoll KE, Gardner DE, et al. 2013. Toxicity of lunar dust assessed in inhalation-exposed rats. Inhal Toxicol. 25(12):661–678.
- Lam CW, James JT, McCluskey R, Cowper S, Balis J, Muro-Cacho C. 2002. Pulmonary toxicity of simulated lunar and Martian dusts in mice: I. Histopathology 7 and 90 days after intratracheal instillation. Inhal Toxicol. 14(9):901–916.
- Lam CW, James JT, Latch JN, Hamilton RF, Jr, Holian A. 2002. Pulmonary toxicity of simulated lunar and Martian dusts in mice: II. Biomarkers of acute responses after intratracheal instillation. Inhal Toxicol. 14(9):917–928.
- Latch JN, Hamilton RF, Jr, Holian A, James JT, Lam CW. 2008. Toxicity of lunar and martian dust simulants to alveolar macrophages isolated from human volunteers. Inhal Toxicol. 20(2):157–165.
- Liu J, Pang Z, Wang G, Guan X, Fang K, et al. 2017. Advanced role of neutrophils in common respiratory diseases. J Immunol Res:Article ID. 6710278:1–21.
- Lodovici M, Bigagli E. 2011. Oxidative Stress and Air Pollution Exposure. J. Toxicol. Article ID. 2011:1–9.
- McKay DS, Carter JL, Boles WW, Allen CC, Alton JH. 1994. JSC-1: a new lunar soil simulant. In: Engineering, construction and operations in space IV, Proceedings of Space ’94, sponsored by the Aerospace Division/ASCE, held February 26 – March 3, Albuquerque, NM.
- McKay DS, Cooper BL, Taylor LA, James JT, Thomas-Keprta K, Pieters CM, Wentworth SJ, Wallace WT, Lee TS. 2015. Physicochemical properties of respirable-size lunar dust. Acta Astronaut (UK). 107:163–176.
- Morrow PE, Haseman JK, Hobbs CH, Driscoll KE, Vu V, Oberdörster G. 1996. The maximum tolerated dose for inhalation bioassays: toxicity vs overload. Fundam Appl Toxicol. 29(2):155–167.
- Mossman BT, Borm PJ, Castranova V, Costa DL, Donaldson K, Kleeberger SR. 2007. Mechanisms of action of inhaled fibers, particles and nanoparticles in lung and cardiovascular diseases. Part Fibre Toxicol. 4:4–14.
- Nakamura T, Noguchi T, Tanaka M, Zolensky ME, Kimura M, Tsuchiyama A, Nakato A, Ogami T, Ishida H, Uesugi M, et al. 2011. Itokawa dust particles: a direct link between S-type asteroids and ordinary chondrites. Science. 333 (6046):1113–1116.
- Nakamura E, Makishima A, Moriguti T, Kobayashi K, Tanaka R, Kunihiro T, Tsujimori T, Sakaguchi C, Kitagawa H, Ota T, et al. 2012. Space environment of an asteroid preserved on micrograins returned by the Hayabusa spacecraft. Proc Natl Acad Sci USA. 109(11):E624–E629.
- NASA 2004, President Bush Delivers Remarks On U.S. Space Policy https://www.nasa.gov/pdf/54868main_bush_trans.pdf.
- NASA 2018. Building a lunar base with 3D printing. http://sservi.nasa.gov/articles/building-a-lunar-base-with-3d-printing/. (Accessed July 2018).
- Pauluhn J, Wieman M. 2011. Siderite (FeCO3) and magnetite (Fe3O4) overload-dependent pulmonary toxicity is determined by the poorly soluble particle not the iron content. Inhal Toxicol. 23(13):763–783.
- Roberts JR, Mercer RR, Chapman RS, Cohen GM, Bangsaruntip S, Schwegler-Berry D, et al. 2012. Pulmonary toxicity, distribution, and clearance of intratracheally instilled silicon nanowires in rats. J Nanomaterials 2012:398302.
- Robinson JP. 1998. Oxygen and nitrogen reactive metabolites and phagocytic cells. In: Robinson JP, Babcock GF, editor(s). Phagocyte function: a guide for research and clinical evaluation. New York (NY): Wiley-Liss. p. 217–252.
- Rydell-Törmänen K, Uller L, Erjefält JS. 2006. Direct evidence of secondary necrosis of neutrophils during intense lung inflammation. Eur Respir J. 28(2):268–274.
- Saran M, Summer KH. 1999. Assaying for hydroxyl radicals: Hydroxylated terephthalate is a superior fluorescence marker than hydroxylated benzoate. Free Radic Res. 31(5):429–436.
- Schmitt HH. 2005. Return to the moon: exploration, enterprise, and energy in the human settlement of space. New York: Springer.
- Scully RR, Lam CW, James JT. 2013. Estimating safe human exposure levels for lunar dust using benchmark dose modeling of data from inhalation studies in rats. Inhal Toxicol. 25(14):785–793.
- Shen H, Anastasio C. 2012. A comparison of hydroxyl radical and hydrogen peroxide generation in ambient particle extracts and laboratory metal solutions. Atmos Environ. 46:665–668.
- Silva MT, do Vale A, dos Santos NM. 2008. Secondary necrosis in multicellular animals: an outcome of apoptosis with pathogenic implications. Apoptosis. 13(4):463–482.
- Space News 2018. Urban planning for the Moon Village by Jeff Foust. Alexandria, Virginia: Space News. https://spacenews.com/urban-planning-for-the-moon-village/
- Space 2020. Trump signs executive order to support moon mining, tap asteroid resources by M. Wall. Space.com April 06, 2020. https://www.space.com/trump-moon-mining-space-resources-executive-order.html
- Specktor B. 2018. Moon dust is super toxic to human cells. Live science. https://www.livescience.com/62590-moon-dust-bad-lungs-brain.html.
- Taylor LA, Yang L. 2010. Important considerations for lunar soil simulants. Earth and Space 2010: engineering, science, construction, and operations in challenging environments.
- Turci F, Corazzari I, Alberto G, Martra G, Fubini B. 2015. Free-radical chemistry as a means to evaluate lunar dust health hazard in view of future missions to the moon. Astrobiology. 15(5):371–380.
- Vallyathan V. 1994. Generation of oxygen radicals by minerals and its correlation to cytotoxicity. Environ. Health Perspect. 102(10):111–115.
- Vallyathan V, Castranova V, Pack D, Leonard S, Shumaker J, Hubbs AF, Shoemaker DA, Ramsey DM, Pretty JR, McLaurin JL, et al. 1995. Freshly fractured quartz inhalation leads to enhanced lung injury and inflammation. Potential role of free radicals. Am J Respir Crit Care Med. 152(3):1003–1009.
- Vallyathan V, Shi XL, Dalal NS, Irr W, Castranova V. 1988. Generation of free radicals from freshly fractured silica dust. Potential role in acute silica-induced lung injury. Am Rev Respir Dis. 138(5):1213–1219.
- Vandette K. 2018. Moon dust is extremely toxic and poses health hazards for astronauts. https://www.earth.com/news/moon-dust-toxic-health/
- Wallace WT, Taylor LA, Liu Y, Cooper BL, McKAY DS, Chen B, Jeevarajan AS. 2009. Lunar dust and lunar simulant activation and monitoring. Meteorit & Planet Sci. 44(7):961–970.
- Zhang DD, Hartsky MA, Warheit DB. 2002. Time course of quartz and TiO(2) particle-induced pulmonary inflammation and neutrophil apoptotic responses in rats. Exp Lung Res. 28(8):641–670.