Abstract
Natural killer (NK) cells play a key role in innate immune defense against infectious disease and cancer. A reduction of NK activity is likely to be associated with increased risk of these types of disease. In this study, we investigate the activation potential of kumquat pericarp acetone fraction (KP-AF) on NK cells. It is shown to significantly increase IFN-γ production and NK cytotoxic activity in human KHYG-1 NK cells. Moreover, oral administration of KP-AF significantly improves both suppressed plasma IFN-γ levels and NK cytotoxic activity per splenocyte in restraint-stressed mice. These results indicate that raw kumquat pericarp activates NK cells in vitro and in vivo. To identify the active constituents, we also examined IFN-γ production on KHYG-1 cells by the predicted active components. Only β-cryptoxanthin increased IFN-γ production, suggesting that NK cell activation effects of KP-AF may be caused by carotenoids such as β-cryptoxanthin.
Graphical abstract
Kumquat pericarp acetone fraction enhanced natural killer cell activity in human KHYG-1 natural killer cells and in restraint stress mice.
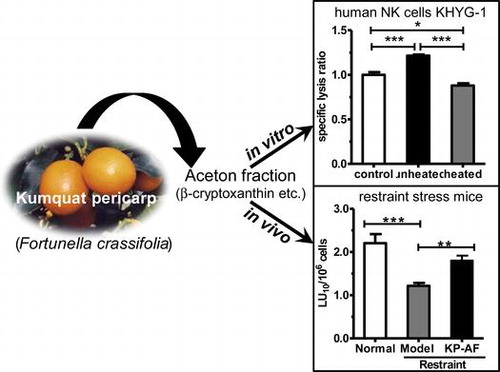
Natural killer (NK) cells play an important role in innate immune response, such as preventing growth of certain virally infected cells in the early stage of host defense, and elimination of tumor target cells.Citation1,2) NK cells are activated by both direct and indirect modulation of cytokines, such as interferon (IFN) and interleukins (ILs), to kill the target cells.Citation3) Decrease in NK activity is likely to be associated with increased cancer riskCitation4) and certain infectious disease outcomes,Citation5) therefore enhanced NK activity is beneficial for maintaining good health. Probiotics,Citation6) ginseng polysaccharide,Citation7) β-caroteneCitation8), and oligonolCitation9) have all been shown to enhance NK activity in human subjects. In vivo experiments with various foods (e.g. Lactobacillus acidophilusCitation10) and β-glucan from maitake mushroomsCitation11)) on viral infection and tumor-bearing mouse models have shown reduced disease risk by enhancing NK activity.
Psychological stress induces immune dysregulation, and negative effects are associated with the increased risk of developing diseases such as infectious diseases and cancer.Citation12−14) During stress, the hypothalamic–pituitary–adrenal axis regulates the systemic release of glucocorticoids and catecholamines which are known to suppress both innate and adaptive immune responses.Citation15,16) Glucocorticoids induce apoptosis in lymphocytesCitation17) and suppress NK cell activity and cytokine production, including IFN-γ, IL-6, and tumor necrosis factor-alpha.Citation18)
Kumquats (Fortunella spp.), a member of the Citrus genus, have long been cultivated in China, Japan, Taiwan, and Southeast Asia. As the peel is sweet and the fruit is sour, kumquats are usually eaten raw and whole, or only the peel is consumed. They are also used in the production of candied kumquats, marmalade, kumquat compote, liqueurs, and sauces. In addition to use in the food industry, kumquats have been used in folk medicine for treating sore throats and coughing. Kumquat fruit and peel have previously been reported to contain various nutrients and constituents such as ascorbic acid,Citation19) carotenoids,Citation19−21) essential oilsCitation22), and flavonoids.Citation19,21,23) Several studies on kumquats have provided evidence of their health-promoting effects and pharmacological activities, including antioxidant activity,Citation23−25) anti-metabolic disorder effects,Citation26) and antimicrobial activity.Citation27) However, to our knowledge, NK cell activation potential of kumquat (Fortunella crassifolia) pericarp has not been evaluated. Therefore, we investigated the effect of kumquat pericarp acetone fraction (KP-AF) on human KHYG-1 NK cells in vitro, and in vivo using a restraint stressed mouse model. We report here that eating kumquat can help maintain NK cell activity in a stressful environment.
Materials and methods
Materials
Acetone, n-hexane, ethanol, ethyl acetate (EtOAc), acetonitrile, tetrahydrofuran, methanol, RPMI 1640 medium with and without phenol red, penicillin–streptomycin solution, recombinant human interleukin-2, β-carotene, and concanavalin A were obtained from Wako Pure Chemical Industries, Ltd. (Osaka, Japan). Calcein-acetoxymethyl (calcein-AM) and HEPES were purchased from Dojindo (Kumamoto, Japan). Mineral oil light white was purchased from MP Biomedicals LLC (Illkirch, France). AssayMax Corticosterone enzyme-linked immunosorbent assay (ELISA) Kit was purchased from AssayPro (Charles, MO, USA). Lutein, zeaxanthin, and β-cryptoxanthin were obtained from Extrasynthese (ZI Lyon-Nord, Genay, France). d-Limonene was purchased from Accustandard Inc. (New Haven, CT, USA). Human and mouse IFN-γ ELISA Development Kit were purchased from PeproTech (Rocky Hill, NJ, USA).
Preparation of kumquat pericarp fraction (KPF)
Kumquats (Fortunella crassifolia) larger than 33 mm in diameter and over 16 brix were harvested in Miyazaki, Japan, 210 days after flowering. A lyophilized powder of kumquat pericarp (KP) was kindly supplied by Miyazaki JA Food Research & Development Inc. (Miyazaki, Japan). The fractionation scheme for KP is shown in Fig. . The lyophilized KP powder (30.1 g) was mixed with Na2CO3 (0.6 g) and 90 mL of cold acetone. The mixture was stirred for 3 min in an ice bath, filtered through filter paper (No 2; ADVANTEC, Tokyo, Japan), and the filtrate saved. The extraction and filtration was repeated four times using the residue from the first extraction. The filtrate was extracted twice with n-hexane/water (1:1.7) and the organic layer was dried (Na2SO4) and concentrated with a vacuum evaporator to give KP-AF (273 mg).
The residue from the acetone extraction was extracted another three times with ethanol for 24 h at 40 °C. The ethanol extract was concentrated and the resultant solid (10.25 g) was redissolved in EtOAc to give the EtOAc-soluble solution and EtOAc-insoluble material KP-F8 (9.19 g). The EtOAc-soluble solution was acidified with 1 mol/L HCl to pH 3, then the aqueous layer (A1) was basified with 1 mol/L NaOH to pH 10 in the presence of EtOAc. The aqueous layer (A1-A) was lyophilized to give KP-F1 (6.8 g). The EtOAc layer (A1-E) was washed twice with water, dried with Na2SO4 and concentrated to give KP-F2 (3.3 mg). The EtOAc layer (E1) was basified with 1 mol/L NaOH to pH 10. The EtOAc extract (E1-E) was washed twice with water, dried with Na2SO4, and concentrated with a vacuum evaporator (KP-F4; 183 mg), while the aqueous layer (E1-A) was further acidified with 1 mol/L HCl to pH 3 in the presence of EtOAc. The aqueous layer (E1-AA) was lyophilized (KP-F3; 4.0 g), and the EtOAc layer (E1-AE) was shaken with 10% NaHCO3 until the aqueous layer reached pH 9. The EtOAc layer (E1-AE-E) was washed twice with water, dried with Na2SO4, and concentrated with a vacuum evaporator (KP-F7; 39 mg). The aqueous layer (E1-AE-A) was again acidified with 1 mol/L HCl to pH 3 in the presence of EtOAc. The EtOAc layer (E1-AE-AE) was washed twice with water, dried with Na2SO4, and concentrated with a vacuum evaporator (KP-F6; 79 mg). The aqueous layer (E1-AE-AA) was lyophilized (KP-F5; 5.0 g). For in vivo experiments, KP-AF (1.04 g) was obtained from the lyophilized powder (54.5 g) of KP.
Chemical assays
Carotenoids used were β-carotene, β-cryptoxanthin, lutein, and zeaxanthin, in agreement with previous kumquat studies.Citation19,21) The KP-AF used for the chemical assays was divided into two groups; an unheated group and a heated group (90 °C, 10 min). Each KP-AF sample was dissolved in acetone. The solutions were analyzed for β-carotene and β-cryptoxanthin after 100 times dilution with acetone, and for lutein and zeaxanthin after 10 times dilution with acetone. High-performance liquid chromatography analysis was performed using Shimadzu LC-10AD vp pumps (Shimadzu, Tokyo, Japan), a SPD-M10 Avp photodiode array detector (Shimadzu), and a Mightysil RP-18 GP C 18 column (250 × 4.6 mm i.d., 5 μm; Kanto Chemical Co., Inc., Tokyo, Japan). The analysis was carried out under isocratic conditions with a flow rate of 1.0 mL/min, and injection volume 10 μL. The mobile phase consisted of acetonitrile (solvent A)/ethanol (solvent B) (8/2, v/v) for β-carotene and β-cryptoxanthin, and tetrahydrofuran/methanol/water (40/30/25, v/v/v) for lutein and zeaxanthin. The column temperature was 50 °C and the absorbance was read at 450 nm. The standard curves were obtained in the concentration range of 0.25–5 μg/mL (β-carotene and β-cryptoxanthin) and the concentration range of 0.2–5 μg/mL (lutein and zeaxanthin).
Cells
Human NK cells KHYG-1 were obtained from the Japanese Collection of Research Bioresources (Osaka, Japan) and maintained in RPMI 1640 medium supplemented with 10% fetal calf serum, penicillin–streptomycin (100 U/mL penicillin and 100 μg/mL streptomycin), and 100 U/mL interleukin-2. The human chronic myeloid leukemia cells K562 and mouse lymphoma cells YAC-1 were cultured in RPMI 1640 medium supplemented with 10% fetal calf serum and penicillin–streptomycin (100 U/mL penicillin and 100 μg/mL streptomycin). All cells were maintained in a humidified atmosphere containing 5% CO2 at 37 °C.
Animals and treatment
Male 7-week-old C57BL/6 N mice were obtained from Japan SLC, Inc. (Shizuoka, Japan). The mice were acclimatized for a week prior to the experiment. All mice were housed under controlled conditions of temperature at 24 ± 1 °C and 12 h light/12 h dark cycle (lights on from 07:00 to 19:00), and fed with CRF-1 (Oriental Yeast Co. Ltd., Tokyo, Japan) and water ad libitum. Mice were treated according to the Animal Experiment Committee of Miyazaki University. The experimental protocol was approved by the ethics committee of Miyazaki University (permit number 2013-040).
The experimental mice were divided into three groups: normal, model control (restraint stress), and KP-AF (restraint stress + KP-AF administration). After acclimatization for 1 week, KP-AF was orally administered to the mice at a dose of 0.6 mg/kg body weight/day for 7 consecutive days. The normal and model control mice were orally administered with mineral oil light white as a vehicle control. On the 7th day of administration, the mice were subjected to restraint stress according to a previously described method with some modifications.Citation28) Briefly, the mice were first wetted with water and physically restrained for 15 h (19:00–10:00) in a restraint cage. The cage was a 50 mL polypropylene centrifuge tube with numerous air holes to increase ventilation, with half of the tube replaced by Mouse Net (BP98-NTM, Natsume Seisakusho, Tokyo, Japan). Normal mice were fed with food and water ad libitum for a whole day.
Measurement of plasma corticosterone levels
After restraint, the mice were weighed and small blood samples were collected from the tail vein into a heparin-coated capillary tube (Drummond Scientific, Broomall, PA, USA). Plasma was isolated by centrifugation at 1500 × g for 5 min at 4 °C and the samples were stored at −80 °C until measurement. Plasma corticosterone levels were determined using a quantitative competitive sandwich enzyme immunoassay technique using an AssayMax Corticosterone ELISA Kit according to the manufacturer’s instructions.
Spleen lymphocytes preparation
The spleens were harvested, washed in RPMI 1640 medium with 10 mmol/L HEPES on ice, and mashed with a plunger on a 70 μm cell strainer (Greiner Bio-one, Frickenhausen, Germany). Collected splenocytes were centrifuged at 400 × g for 5 min and hemolyzed with a lysis buffer (0.75% NH4Cl, 17 mmol/L Tris-HCl, pH 7.65). The lymphocytes were then washed twice with cold RPMI 1640 medium containing 10 mmol/L HEPES, resuspended in cold RPMI 1640 medium without phenol red, and supplemented with 10% fetal calf serum. The cells were then filtered through a 40 μm cell strainer (Greiner Bio-one) and the total number of spleen lymphocytes was determined using a hemocytometer.
NK cell cytotoxicity assay
NK cytotoxic activity was measured using the calcein-AM-release assay, a fluorometric assay comparable to the traditional 51Cr-release assay.Citation29) Target K562 or YAC-1 cells (1 × 106 cells/mL) were labeled with 10 μg/mL calcein-AM for 1 h at 37 °C with gentle rotation and washed.
NK cell cytotoxicity assay in vitro was determined by the cytotoxicity of effector NK cells KHYG-1 against target cells K562. KHYG-1 cells (2 × 105 cells/mL) were treated with KPF, β-cryptoxanthin, or vehicle control (dimethyl sulfoxide). After 24 h, retrieved cells were then washed with cold phosphate buffered saline, resuspended in RPMI 1640 medium without phenol red, and supplemented with 1% bovine serum albumin. KHYG-1 cells (1 × 105 cells/well) and calcein AM-labeled K562 cells (1 × 104 cells/well) were co-cultured at an effector-to-target cell (E:T) ratio of 10:1 on 96-well U-bottomed plates, and incubated at 37 °C for 4 h. Maximum release was produced by incubation of only target cells in medium with 2% Triton X-100. Spontaneous release was produced by incubation of only target cells in medium. After incubation, the cultured mixtures in the 96-well plates were centrifuged at 260 × g for 10 min, and 100 μL of the supernatant was transferred to a new plate. The fluorescence was measured with a Spectramax Gemini XS Fluorescence Microplate Reader (Molecular Devices, Sunnyvale, CA, USA) (excitation filter 485 nm, emission filter 538 nm). The percentage of specific lysis was calculated as follows: Specific lysis (%) = [(experimental release − spontaneous release) / (maximum release − spontaneous release)] × 100.
NK cell cytotoxicity assay in vivo was determined by the cytotoxicity of mouse splenocytes against YAC-1 cells. Mouse splenocytes (effector cells) and calcein AM-labeled YAC-1 (target) cells were co-cultured at various E:T ratios (100:1, 50:1, 25:1 and 12.5:1) on 96-well U-bottomed plates, and incubated at 37 °C for 4 h. The percentage of specific lysis was calculated in the same manner as described above. Specific lysis values obtained from the calcein-AM-release assay were converted to lytic units. Calculation of lytic units (LU) has been defined as previously reported.Citation30) LU is defined as the number of cells required to cause 10% target cell lysis relative to 106 effector cells, as determined by the equation:
where is the mean of the logistically transformed specific lysis values, p is the reference lysis percentage used in defining the lytic unit (in this case, p = 10%),
= ln(p/100 – p), C is a constant set to 1, T is the number of target YAC-1 cells, and
is the geometric mean of the E:T ratio used in the assay. The NK activity per spleen (LU10/spleen) was calculated as follows:
Measurement of cytokine production
Human NK cell line KHYG-1 cells were inoculated into a 48-well microtiter plate at 6 × 104 cells/well and treated with KPF, experimental compounds (β-cryptoxanthin, β-carotene, zeaxanthin, or Lutein), or vehicle control (dimethyl sulfoxide). After 24 h of incubation, the culture medium was centrifuged at 180 × g for 5 min to remove the cells. IFN-γ levels in the supernatants were determined using a Human IFN-γ ELISA Development Kit according to the manufacturer’s instructions.
The levels of IFN-γ in mouse plasma were also measured by commercially available ELISA kits according to the manufacturer’s instructions.
Statistical analysis
The results are expressed as mean ± standard error (SE) or the mean ± standard deviation (SD). A one-way analysis of variance (ANOVA) and Dunnett’s post hoc test were used to evaluate the differences between the mean values of the results for the control and treated samples. Multiple comparisons were performed using one-way ANOVA followed by Tukey–Kramer as a post hoc test. Statistical differences between two groups were determined by Student’s t-test. A p-value of <0.05 was considered significant. Statistical analysis was performed using GraphPad Prism version 5.0 (GraphPad Software, San Diego, CA, USA).
Results and discussion
KP-AF activation of KHYG-1 NK cells
We screened pericarp extracts of three citruses, bitter orange (Citrus aurantium), hyuganatsu (Citrus tamurana), and kumquat, for 10 health-promoting effects using artificial neural network models by modifications of our previous report.Citation31) As a result of screening, all pericarp extracts were shown to activate NK cells, with KP extract having the strongest activity. Therefore, we separated various fractions from KP using pH-dependent extractions with various organic solvents (Fig. ). The effect of the different KP fractions on NK cell activation was determined by IFN-γ production using human NK cell line, KHYG-1. As shown in Fig. , the KP-AF showed the strongest NK cell activation effect as it exhibited higher relative IFN-γ production values than the other fractions (KP-F1–F8).
Fig. 2. NK cell activation effects of KP fractions in KHYG-1 cells.
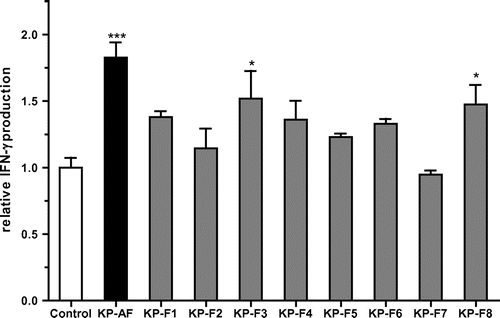
We next examined the thermal stability of KP-AF on NK cell activation. The KP-AF was divided into two groups, an unheated and a heated group (90 °C, 10 min). The effect of the unheated and heated KP-AF on NK cell activation was determined by IFN-γ production and NK cytotoxic activity using KHYG-1 cells. As shown in Fig. , unheated KP-AF treatment significantly increased IFN-γ production and NK cytotoxic activity in KHYG-1 cells. Interestingly, this increase was not observed after heat-treatment at 90 °C for 10 min (Fig. ). These results suggest that the reduction of activity in KP-AF may be due to the thermolability of active components.
Fig. 3. NK cell activation effects of KP-AF in KHYG-1 cells.
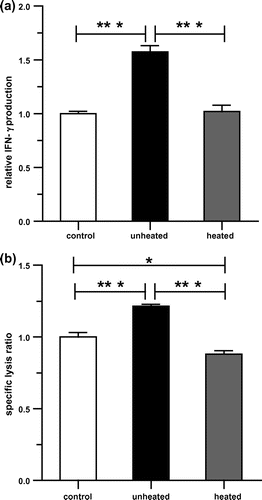
Carotenoids enhancement of IFN-γ production on KHYG-1 cells and determination of carotenoid levels
Kumquat has been reported to contain various constituents such as carotenoids,Citation19−21) essential oilCitation22), and polyphenols.Citation19,21,23) Among them, d-limonene,Citation32) which is one of the main components of essential oil, and the flavonoid chrysinCitation33) have been shown to possess NK cell activation effects in vitro or in vivo. The carotenoids β-cryptoxanthinCitation34) and citrus coumarin aurapteneCitation35) have also been reported to have immunomodulation effects in vitro and in vivo. Therefore, we examined IFN-γ production against KHYG-1 cells treated with these four compounds. The concentrations of the tested compounds were determined from previous reports.Citation32–35) As shown in Fig. , IFN-γ production was increased with auraptene and β-cryptoxanthin treatment of KHYG-1 cells. A similar result has been reported for the effect of IFN-γ production enhancement by auraptene on mouse splenocytes.Citation35) However, to our knowledge, IFN-γ production enhancement with β-cryptoxanthin has not yet been reported. Although chrysin and d-limonene were reported to activate NK activity,Citation32,33) no increase in IFN-γ production was observed in KHYG-1 cells (Fig. ). KP-AF is a dark red oil with a distinctive fragrant flavor, characteristic of kumquat.Citation22) We tested the effect of d-limonene on KHYG-1 cells, as it is the main essential oil constituent in kumquats, but found that it did not increase IFN-γ production. This demonstrates that KP-AF NK cell activation is not due to d-limonene. The dark red color of KP-AF is probably attributed to carotenoids, and IFN-γ production enhancement by the carotenoid β-cryptoxanthin was the highest among those tested (Fig. ). Kumquat contains β-cryptoxanthin as well as various other carotenoids such as β-carotene, lutein, and zeaxanthin, which have all been reported in previous kumquat studies.Citation19,21) Therefore, we examined the effects of these carotenoids and determined the quantities of them in KP-AF.
Fig. 4. Effect of IFN-γ production enhancement by carotenoids and other constituents on KHYG-1 cells.
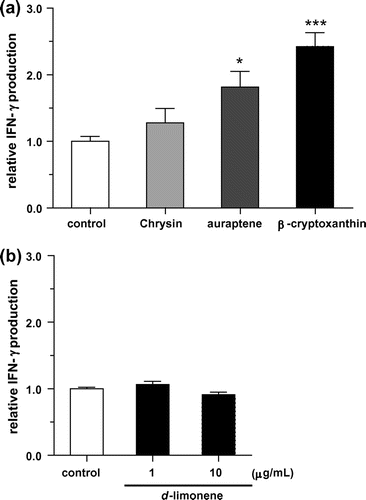
Of the four carotenoids, only β-cryptoxanthin (25 μg/mL) treatment significantly increased the IFN-γ production on KHYG-1 cells (Fig. (a)). Treatment with β-carotene (25 μg/mL) tended to increase the IFN-γ production, but not significantly. Lutein and zeaxanthin (oxygenated carotenoids and stereoisomers) did not enhance IFN-γ production. Although oral administration of β-carotene has been reported to enhance NK cytotoxic activity in elderly men,Citation8) the enhancement of IFN-γ production on KHYG-1 cells was weak, and β-cryptoxanthin showed a stronger effect (Fig. (a)). We also evaluated the effect of β-cryptoxanthin on NK cytotoxic activity. As shown in Fig. (b), β-cryptoxanthin (25 μg/mL) treatment significantly increased NK cytotoxic activity in KHYG-1 cells. These results show that β-cryptoxanthin may be one candidate active constituent. To our knowledge, there have been no previously published studies examining a single oral administration of β-cryptoxanthin to human subjects. In a clinical trial of viral hepatitis patients with cirrhosis, cumulative incidences of hepatocellular carcinoma development were compared among carotenoids mixture capsules treated group, carotenoids mixture capsules plus β-cryptoxanthin-enriched mandarin orange juice treated group, and control group.Citation36) The 2.5-year cumulative incidence in β-cryptoxanthin-enriched mandarin orange juice treated group was the lowest among three groups.Citation36) A cohort study also reported that a high serum β-cryptoxanthin concentration was significantly associated with reduced risk of lung cancer.Citation37) Although NK cytotoxic activity was not demonstrated in these studies, the reduced risk of these cancers may be related to NK cytotoxic activity. Therefore, oral administration of β-cryptoxanthin may also be expected to enhance NK cytotoxic activity in human.
Fig. 5. NK cell activation effects of carotenoids in KHYG-1 cells.
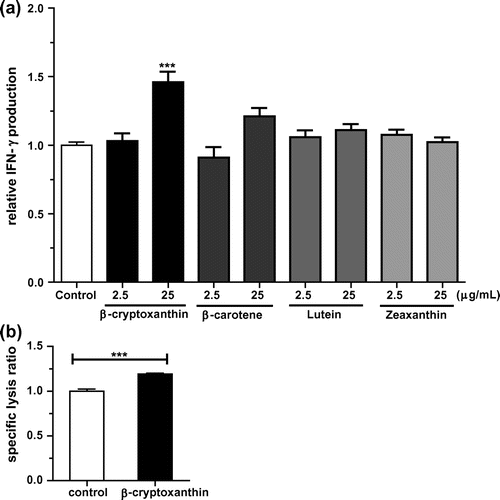
Carotenoids are generally known to be thermally labile,Citation38) so we quantified the amount of carotenoids (including β-cryptoxanthin, β-carotene, lutein, and zeaxanthin) in unheated and heated KP-AF. As shown in Table , the level of β-cryptoxanthin in KP-AF (98.2 ± 7.1 μg/g) was highest, followed by β-carotene (56.5 ± 10.1 μg/g), lutein (19.8 ± 14.1 μg/g), and zeaxanthin (10.1 ± 7.2 μg/g). β-Cryptoxanthin and β-carotene were the major carotenoids, whereas lutein and zeaxanthin were the minor carotenoids in KP-AF. A similar tendency was reported for kumquat fruit cultivated in Taiwan,Citation21) but the carotenoid content of kumquats cultivated in ItalyCitation19) was different from that of KP-AF. Such a difference will depend on various factors such as cultivar, harvesting area, and harvest timing. Carotenoid levels in heated KP-AF tended to be lower than that of unheated KP-AF. However, there were no statistically significant differences between the unheated and heated KP-AF in all four carotenoids tested. In addition, the percentage of each carotenoid per total carotenoids was maintained between unheated and heated KP-AF. These results show that the composition of carotenoids and the levels in KP-AF are unaffected by heat-treatment in at least these four carotenoids. A previous study has reported the influence of heating on carotenoids in vegetables and fruits. Heat-treatment (98 °C, 10 min) of some fruits and vegetables caused a decrease in the carotenoid levels, while levels in other fruits and vegetables were maintained.Citation39) The levels of carotenoids in KP-AF may not be easily influenced by heating. Unheated KP-AF significantly increased both IFN-γ production and NK cytotoxic activity in KHYG-1 cells, although this increase was not observed after heat treatment at 90 °C for 10 min (Fig. ). Carotenoids are degraded by oxidation by heat, light, oxygen, acid, transition metals, or interactions with radical species.Citation38) Therefore, reduction in NK activity in heated KP-AF may be caused by thermal oxidation of carotenoids, not by a quantitative change. It is also worth considering that KP-AF may not only enhance NK cell activity by carotenoids such as β-cryptoxanthin, but also by the interaction of carotenoids with other constituents.
Table 1. Carotenoids contents in unheated and heated KP-AF.
Effect of KP-AF on NK cell activation in restraint-stressed mice
We also investigated whether orally administered KP-AF enhances the NK cell activation of restraint-stressed mice. As shown in Table , restraint stress significantly reduces spleen weight, spleen index, and spleen lymphocyte number by about 31% (p < 0.001), 17% (p < 0.01), and 61% (p < 0.001), respectively. However, oral administration of KP-AF did not recover spleen weight, spleen index or spleen lymphocyte number in stressed mice. We then evaluated plasma corticosterone levels, as acute stress increases levels of glucocorticoids such as corticosterone. The plasma corticosterone level in restraint-stressed mice was approximately 4.7-fold higher than that in normal mice (Fig. (a)). However, the increase in the plasma corticosterone levels caused by restraint stress was not reduced by the orally administered KP-AF (Fig. (a)). These results suggest that oral administration of KP-AF does not suppress the increment in the plasma corticosterone level by restraint stress, resulting in reduction of spleen weight, spleen index, and spleen lymphocyte number. A previous report suggests that restraint stress markedly reduces spleen lymphocyte number and causes spleen atrophy owing to spleen lymphocyte apoptosis by stress-induced glucocorticoid overflow.Citation40) KP-AF was not involved in the protective effect of the reduction of spleen lymphocytes caused by restraint stress.
Table 2. Effect of KP-AF on spleen weight, spleen index, and spleen lymphocyte number in restraint-stressed mice.
Fig. 6. Effect of KP-AF on plasma corticosterone levels, IFN-γ level and NK cell cytotoxicity of restraint-stressed mice.
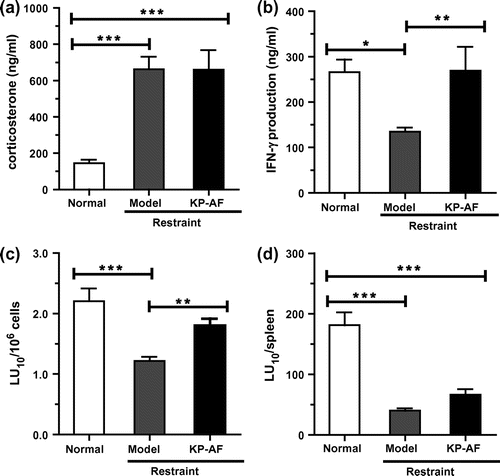
Moreover, effect of KP-AF on cytokine production was also examined in the plasma as shown in Fig. (b). The plasma IFN-γ levels in restraint-stressed mice were significantly reduced compared with those in normal mice. Oral administration of KP-AF significantly improved the suppressed plasma IFN-γ levels in stressed mice (Fig. (b), p < 0.01). This KP-AF enhancement of IFN-γ production shown in vivo is similar to in vitro experimental results using KHYG-1 cells.
We also evaluated the effects of KP-AF on NK cytotoxic activity in the spleen using a calcein-AM-release assay. As shown in Fig. (c) and (d), restraint stress significantly reduces both NK cytotoxic activity per splenocyte (LU10/106 cells) and NK cytotoxic activity per spleen (LU10/spleen) compared with normal mice. Oral administration of KP-AF significantly improves the suppressed NK cytotoxic activity per splenocyte (LU10/106 cells) in stressed mice (Fig. (c); p<0.01), and so the NK cell activation effects of KP-AF in vitro were confirmed by these in vivo experiments. Although oral administration of KP-AF tends to improve the suppressed NK cytotoxic activity per spleen (LU10/spleen), this is not significant (restraint vs. KP-AF; Fig. (d)). This result means that KP-AF is not involved in the protective effect on the reduction of splenocytes by stress-induced corticosterone. In contrast to our study, recent in vivo studies in restraint stressed mice have shown that carnosine (β-alanyl-L-histidine) can maintain spleen lymphocyte numbers to prevent immunocompromise caused by restraint stress, although carnosine administration had no obvious effect on NK cytotoxic activity per splenocyte.Citation40) The major food source of carnosine is foods containing muscle tissue, such as chicken and beef. Kumquat and carnosine eaten at the same time could prevent most immunocompromise caused by stress.
Among the tested compounds, only β-cryptoxanthin showed enhancement of IFN-γ production in KHYG-1 cells. β-cryptoxanthin was also enhanced KHYG-1 cytotoxic activity. These suggest that the NK cell activation effect of KP-AF may be caused by carotenoids such as β-cryptoxanthin. Reactive oxygen species contribute to NK cell dysfunction such as loss of numbers and activities.Citation41) Carotenoids from kumquats have been shown to possess antioxidant activity.Citation23) In restraint-stressed mice, apple polyphenols extractCitation42) and astaxanthinCitation43) elevated immune functions of stressed animals because of antioxidant activities directly or indirectly in immunocytes. Oral administration of KP-AF may also enhance NK cell activation of restraint stressed mice because of antioxidant activities of carotenoids including β-cryptoxanthin. However, the bioactivity of the antioxidant may not be solely due to the anti-oxidative capacity. For example, epigallocatechin-3-gallate (a polyphenol found in green tea) has been reported to exert various bioactivities such as anti-cancer activity,Citation44) anti-allergic activity,Citation45) and anti-inflammatory activityCitation46) through the 67-kDa laminin receptor. The active component in KP-AF may also enhance NK activity through another cell surface receptor.
In conclusion, this is the first study to show NK cell activation effects of KP-AF from kumquats in vitro and in vivo. KP-AF treatment significantly increased both IFN-γ production and NK cytotoxic activity in human KHYG-1 NK cells. Moreover, we examined whether orally administered KP-AF could also enhance the NK cell activation of restraint stressed mice. Oral administration of KP-AF significantly improved both suppressed plasma IFN-γ levels and NK cytotoxic activity per splenocyte in stressed mice. However, the increased IFN-γ production in KHYG-1 cells was not observed after heat-treatment at 90 °C for 10 min (Fig. ). The reduction in KP-AF activity may be caused by the thermolability of its active components. The heat-processed kumquat was not expected to have the same level of activation of NK activity as the unheated sample. However, the thermolability of the active components may not be important for kumquats because they are also eaten raw (whole fruit or pericarp). Recently, kumquat consumption by eating raw is increasing. Given the benefits of eating raw, we should draw additional attention to functionality of thermolabile compounds for utilizing advantage of raw diet. Further studies of KP-AF are needed to identify the active components and to evaluate the molecular mechanism responsible for the NK cell activation effect.
Acknowledgments
We thank Dr Noboru Murakami (Department of Veterinary Physiology, Faculty of Agriculture, University of Miyazaki) for his expertise in animal experiments and for providing the necessary facilities to perform this experiment. We thank Dr Takashi Ando and Ms. Miwa Satoh (Miyazaki Agricultural Research Institute) for analysis of carotenoids.
Disclosure statement
No potential conflict of interest was reported by the authors.
References
- Biron CA, Brossay L. NK cells and NKT cells in innate defense against viral infections. Curr. Opin. Immunol. 2001;13:458–464.10.1016/S0952-7915(00)00241-7
- Levy EM, Roberti MP, Mordoh J. Natural killer cells in human cancer: from biological functions to clinical applications. J. Biomed. Biotechnol. 2011;2011:676198.
- Biron CA, Nguyen KB, Pien GC, Cousens LP, Salazar-Mather TP. Natural killer cells in antiviral defense: function and regulation by innate cytokines. Annu. Rev. Immunol. 1999;17:189–220.10.1146/annurev.immunol.17.1.189
- Imai K, Matsuyama S, Miyake S, Suga K, Nakachi K. Natural cytotoxic activity of peripheral-blood lymphocytes and cancer incidence: an 11-year follow-up study of a general population. Lancet. 2000;356:1795–1799.10.1016/S0140-6736(00)03231-1
- Evans DL, Ten Have TR, Douglas SD, Gettes DR, Morrison M, Chiappini MS, Brinker-Spence P, Job C, Mercer DE, Wang YL, Cruess D, Dube B, Dalen EA, Brown T, Bauer R, Petitto JM. Association of depression with viral load, CD8 T lymphocytes, and natural killer cells in women with HIV infection. Am. J. Psychiatry. 2002;159:1752–1759.10.1176/appi.ajp.159.10.1752
- Shida K, Nomoto K. Probiotics as efficient immunopotentiators: translational role in cancer prevention. Indian J. Med. Res. 2013;138:808–814.
- Cho YJ, Son HJ, Kim KS. A 14-week randomized, placebo-controlled, double-blind clinical trial to evaluate the efficacy and safety of ginseng polysaccharide (Y-75). J. Transl. Med. 2014;12:283.10.1186/s12967-014-0283-1
- Santos MS, Gaziano JM, Leka LS, Beharka AA, Hennekens CH, Meydani SN. Beta-carotene-induced enhancement of natural killer cell activity in elderly men: an investigation of the role of cytokines. Am. J. Clin. Nutr. 1998;68:164–170.
- Lee JB, Shin YO. Oligonol supplementation affects leukocyte and immune cell counts after heat loading in humans. Nutrients. 2014;6:2466–2477.10.3390/nu6062466
- Goto H, Sagitani A, Ashida N, Kato S, Hirota T, Shinoda T, Yamamoto N. Anti-influenza virus effects of both live and non-live Lactobacillus acidophilus L-92 accompanied by the activation of innate immunity. Br. J. Nutr. 2013;110:1810–1818.10.1017/S0007114513001104
- Masuda Y, Murata Y, Hayashi M, Nanba H. Inhibitory effect of MD-fraction on tumor metastasis: involvement of NK cell activation and suppression of intercellular adhesion molecule (ICAM)-1 expression in lung vascular endothelial cells. Biol. Pharm. Bull. 2008;31:1104–1108.10.1248/bpb.31.1104
- Powell ND, Tarr AJ, Sheridan JF. Psychosocial stress and inflammation in cancer. Brain Behav. Immun. 2013;30(Suppl):S41–S47.10.1016/j.bbi.2012.06.015
- Glaser R, Kiecolt-Glaser JK. Science and society: stress-induced immune dysfunction: implications for health. Nat. Rev. Immunol. 2005;5:243–251.10.1038/nri1571
- Segerstrom SC, Miller GE. Psychological stress and the human immune system: a meta-analytic study of 30 years of inquiry. Psychol. Bull. 2004;130:601–630.10.1037/0033-2909.130.4.601
- Franchimont D, Galon J, Gadina M, Visconti R, Zhou Y, Aringer M, Frucht DM, Chrousos GP, O’Shea JJ. Inhibition of Th1 immune response by glucocorticoids: dexamethasone selectively inhibits IL-12-induced Stat4 phosphorylation in T lymphocytes. J. Immunol. 2000;164:1768–1774.10.4049/jimmunol.164.4.1768
- Inagaki N, Miura T, Nakajima T, Yoshida K, Nagai H, Koda A. Studies on the anti-allergic mechanism of glucocorticoids in mice. J. Pharmacobiodyn. 1992;15:581–587.10.1248/bpb1978.15.581
- Planey SL, Litwack G. Glucocorticoid-induced apoptosis in lymphocytes. Biochem. Biophys. Res. Commun. 2000;279:307–312.10.1006/bbrc.2000.3922
- Krukowski K, Eddy J, Kosik KL, Konley T, Janusek LW, Mathews HL. Glucocorticoid dysregulation of natural killer cell function through epigenetic modification. Brain Behav. Immun. 2011;25:239–249.10.1016/j.bbi.2010.07.244
- Schirra M, Palma A, D’Aquino S, Angioni A, Minello EV, Melis M, Cabras P. Influence of postharvest hot water treatment on nutritional and functional properties of kumquat (Fortunella japonica Lour. swingle Cv. Ovale) fruit. J. Agric. Food. Chem. 2008;56:455–460.10.1021/jf0714160
- Agócs A, Nagy V, Szabó Z, Márk L, Ohmacht R, Deli J. Comparative study on the carotenoid composition of the peel and the pulp of different citrus species. Innovative. Food Sci. Emerg. Technol. 2007;8:390–394.10.1016/j.ifset.2007.03.012
- Wang YC, Chuang YC, Ku YH. Quantitation of bioactive compounds in citrus fruits cultivated in Taiwan. Food Chem. 2007;102:1163–1171.10.1016/j.foodchem.2006.06.057
- Peng LW, Sheu MJ, Lin LY, Wu CT, Chiang HM, Lin WH, Lee MC, Chen HC. Effect of heat treatments on the essential oils of kumquat (Fortunella margarita Swingle). Food Chem. 2013;136:532–537.10.1016/j.foodchem.2012.08.014
- Jayaprakasha GK, Chidambara Murthy KN, Etlinger M, Mantur SM, Patil BS. Radical scavenging capacities and inhibition of human prostate (LNCaP) cell proliferation by Fortunella margarita. Food Chem. 2012;131:184–191.10.1016/j.foodchem.2011.08.058
- Sadek ES, Makris DP, Kefalas P. Polyphenolic composition and antioxidant characteristics of kumquat (Fortunella margarita) peel fractions. Plant Foods Hum. Nutr. 2009;64:297–302.10.1007/s11130-009-0140-1
- Barreca D, Bellocco E, Caristi C, Leuzzi U, Gattuso G. Kumquat (Fortunella japonica Swingle) juice: flavonoid distribution and antioxidant properties. Food Res. Int. 2011;44:2190–2197.10.1016/j.foodres.2010.11.031
- Tan S, Li M, Ding X, Fan S, Guo L, Gu M, Zhang Y, Feng L, Jiang D, Li Y, Xi W, Huang C, Zhou Z. Effects of fortunella margarita fruit extract on metabolic disorders in high-fat diet-induced obese C57BL/6 mice. PLoS One. 2014;9:e93510.10.1371/journal.pone.0093510
- Wang YW, Zeng WC, Xu PY, Lan YJ, Zhu RX, Zhong K, Huang YN, Gao H. Chemical composition and antimicrobial activity of the essential oil of kumquat (Fortunella crassifolia swingle) peel. Int. J. Mol. Sci. 2012;13:3382–3393.10.3390/ijms13033382
- Iwakabe K, Shimada M, Ohta A, Yahata T, Ohmi Y, Habu S, Nishimura T. The restraint stress drives a shift in Th1/Th2 balance toward Th2-dominant immunity in mice. Immunol. Lett. 1998;62:39–43.10.1016/S0165-2478(98)00021-2
- Neri S, Mariani E, Meneghetti A, Cattini L, Facchini A. Calcein-acetyoxymethyl cytotoxicity assay: standardization of a method allowing additional analyses on recovered effector cells and supernatants. Clin. Diagn. Lab. Immunol. 2001;8:1131–1135.
- Bryant J, Day R, Whiteside TL, Herberman RB. Calculation of lytic units for the expression of cell-mediated cytotoxicity. J. Immunol. Methods. 1992;146:91–103.10.1016/0022-1759(92)90052-U
- Nagahama K, Eto N, Yamamori K, Nishiyama K, Sakakibara Y, Iwata T, Uchida A, Yoshihara I, Suiko M. Efficient approach for simultaneous estimation of multiple health-promoting effects of foods. J. Agric. Food. Chem. 2011;59:8575–8588.10.1021/jf201836g
- Li Q, Nakadai A, Matsushima H, Miyazaki Y, Krensky AM, Kawada T, Morimoto K. Phytoncides (wood essential oils) induce human natural killer cell activity. Immunopharmacol. Immunotoxicol. 2006;28:319–333.10.1080/08923970600809439
- Lin CC, Yu CS, Yang JS, Lu CC, Chiang JH, Lin JP, Kuo CL, Chung JG. Chrysin, a natural and biologically active flavonoid, influences a murine leukemia model in vivo through enhancing populations of T-and B-cells, and promoting macrophage phagocytosis and NK cell cytotoxicity. In Vivo. 2012;26:665–670.
- Nishi K, Muranaka A, Nishimoto S, Kadota A, Sugahara T. Immunostimulatory effect of β-cryptoxanthin in vitro and in vivo. J. Funct. Foods. 2012;4:618–625.10.1016/j.jff.2012.04.001
- Nishimoto S, Muranaka A, Nishi K, Kadota A, Sugahara T. Immunomodulatory effects of citrus fruit auraptene in vitro and in vivo. J. Funct. Foods. 2012;4:883–890.10.1016/j.jff.2012.06.005
- Nishino H, Murakoshi M, Tokuda H, Satomi Y. Cancer prevention by carotenoids. Arch. Biochem. Biophys. 2009;483:165–168.10.1016/j.abb.2008.09.011
- Mannisto S, Smith-Warner SA, Spiegelman D, Albanes D, Anderson K, van den Brandt PA, Cerhan JR, Colditz G, Feskanich D, Freudenheim JL, Giovannucci E, Goldbohm RA, Graham S, Miller AB, Rohan TE, Virtamo J, Willett WC, Hunter DJ. Dietary carotenoids and risk of lung cancer in a pooled analysis of seven cohort studies. Cancer Epidemiol. Biomarkers Prev. 2004;13:40–48.10.1158/1055-9965.EPI-038-3
- Boon CS, McClements DJ, Weiss J, Decker EA. Factors influencing the chemical stability of carotenoids in foods. Crit. Rev. Food Sci. Nutr. 2010;50:515–532.10.1080/10408390802565889
- Leong SY, Oey I. Effects of processing on anthocyanins, carotenoids and vitamin C in summer fruits and vegetables. Food Chem. 2012;133:1577–1587.10.1016/j.foodchem.2012.02.052
- Li YF, He RR, Tsoi B, Li XD, Li WX, Abe K, Kurihara H. Anti-stress effects of carnosine on restraint-evoked immunocompromise in mice through spleen lymphocyte number maintenance. PLoS One. 2012;7:e33190.10.1371/journal.pone.0033190
- Mellqvist UH, Hansson M, Brune M, Dahlgren C, Hermodsson S, Hellstrand K. Natural killer cell dysfunction and apoptosis induced by chronic myelogenous leukemia cells: role of reactive oxygen species and regulation by histamine. Blood. 2000;96:1961–1968.
- He RR, Wang M, Wang CZ, Chen BT, Lu CN, Yao XS, Chen JX, Kurihara H. Protective effect of apple polyphenols against stress-provoked influenza viral infection in restraint mice. J. Agric. Food. Chem. 2011;59:3730–3737.10.1021/jf104982y
- Kurihara H, Koda H, Asami S, Kiso Y, Tanaka T. Contribution of the antioxidative property of astaxanthin to its protective effect on the promotion of cancer metastasis in mice treated with restraint stress. Life Sci. 2002;70:2509–2520.10.1016/S0024-3205(02)01522-9
- Tachibana H, Koga K, Fujimura Y, Yamada K. A receptor for green tea polyphenol EGCG. Nat. Struct. Mol. Biol. 2004;11:380–381.10.1038/nsmb743
- Fujimura Y, Umeda D, Yamada K, Tachibana H. The impact of the 67 kDa laminin receptor on both cell-surface binding and anti-allergic action of tea catechins. Arch. Biochem. Biophys. 2008;476:133–138.10.1016/j.abb.2008.03.002
- Hong Byun E, Fujimura Y, Yamada K, Tachibana H. TLR4 signaling inhibitory pathway induced by green tea polyphenol epigallocatechin-3-gallate through 67-kDa laminin receptor. J. Immunol. 2010;185:33–45.10.4049/jimmunol.0903742