Abstract
During exercise, blood levels of several hormones increase acutely. We hypothesized that consumption of a specific combination of amino acids (arginine, alanine, and phenylalanine; A-mix) may be involved in secretion of glucagon, and when combined with exercise may promote fat catabolism. Ten healthy male volunteers were randomized in a crossover study to ingest either A-mix (3 g/dose) or placebo (3 g of dextrin/dose). Thirty minutes after ingesting, each condition subsequently performed workload trials on a cycle ergometer at 50% of maximal oxygen consumption for 1 h. After oral intake of A-mix, the concentrations of plasma ketone bodies and adrenalin during and post-exercise were significantly increased. The area under the curve for glycerol and glucagon was significantly increased in the post-exercise by A-mix administration. These results suggest that pre-exercise ingestion of A-mix causes a shift of energy source from carbohydrate to fat combustion by increasing secretion of adrenalin and glucagon.
Here, Ueda et al. propose that pre-exercise ingestion of A-mix, Ala-Arg-Phe mixture, increased fat catabolism during and post-exercise.
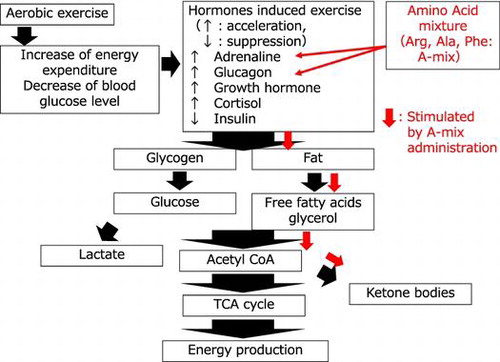
Obesity is a public health problem worldwide,Citation1) and a major risk factor for insulin resistance, type 2 diabetes, atherosclerosis, stroke, hypertension, and some types of cancer.Citation2) Obesity results from a chronic imbalance in energy metabolism. The most common strategies used for weight loss and weight control are calorie reduction and regular physical activity.Citation3)
Regular exercise is one important element in the approach to prevent obesity.Citation4) According to the exercise prescription recommended in the American College of Sports MedicineCitation5) guidelines, 45–60 min of exercise should be targeted to ensure sufficient energy expenditure in obese people. Exercise increases energy expenditure and insulin sensitivity in skeletal muscle,Citation6) and therefore regular exercise is fundamental for preventing obesity. Exercise is strongly encouraged and is likely to be most effective for weight control when combined with improved eating habits. In particular, the blood levels of several hormones such as catecholamines, glucagon, growth hormone, and cortisol are increased during exercise compared with rest periods.Citation7) We hypothesize that a combination of exercise and the factors that affect the secretion of some of these hormones may be effective for increasing fat catabolism and energy expenditure.
Recently, it was discovered that amino acids (AAs) are not only cell signaling molecules but also regulators of gene expression and the protein phosphorylation cascade. AAs are also key precursors for syntheses of hormones and low-molecular weight nitrogenous substances, with each having considerable biological importance.Citation8) It has been reported that ingestion or infusion of some AAs increases insulin or glucagon concentrations in the blood. For example, Nuttall et al.Citation9) showed ingestion of phenylalanine increased both insulin and glucagon concentrations. Intravenous administration of arginine and lysine has been shown to increase the circulating concentration of glucagon.Citation10) Alanine and glycine, but not valine, isoleucine, or leucine, have also been reported to stimulate glucagon secretion.Citation11–13) A specific combination of AAs may therefore stimulate the secretion of insulin/or glucagon. Glucagon is one of the key hormones implicated in fat catabolism during exercise.Citation14,15) Tan et al. reported that glucagon infusion acutely increased energy expenditure in humans.Citation16) We therefore hypothesize that a specific combination of AAs may acutely promote fat catabolism and energy expenditure during and after exercise.
Earlier, we developed a mixture of 17 AAs that contained large amounts of arginine, alanine, and phenylalanine, and then conducted a double-blind, placebo-controlled, crossover study of this mixture. That study showed that maximum serum concentrations of glycerol and acetoacetic acid were significantly higher following ingestion of the AA mixture, whereas the area under the curve (AUC) for glucagon remained unchanged.Citation17) In order to acutely increase glucagon secretion, we recently developed a new mixture (A-mix) that contained 42% [mol/mol {m/m}] of phenylalanine, 38% [m/m] of alanine, and 20% [m/m] of arginine. This mixture increased the ratio of alanine and phenylalanine that are known to affect glucagon secretion. We conducted a randomized, double-blind, placebo-controlled crossover trial to investigate glucagon secretion induced by A-mix and fat catabolism during exercise. The aim of this study was to answer two questions: (a) Does acute oral administration of A-mix stimulate glucagon secretion?, and (b) if so, are fat catabolism and energy expenditure increased by this potentiation?
Methods
Subjects
Ten healthy young men who exercised regularly and belonged to a football team were recruited as study volunteers. The mean baseline characteristics of the participants were as follows: age, 21.1 ± 0.7 yr; height, 170.7 ± 4.9 cm; body mass, 65.6 ± 5.0 kg; body mass index (BMI), 22.5 ± 1.4 kg/m2; maximum oxygen uptake, 45.3 ± 4.4 mL/min/kg. All the study participants provided written informed consent prior to participation in the study. Each subject continued his usual diet and refrained from smoking. The trials were conducted in Japan from February 2015 to March 2015. All the participants underwent physical examinations and blood tests that included the following evaluations: platelet, white blood cell, and red blood cell counts, and the levels of hemoglobin, hematocrit, aspartate aminotransferase, alanine aminotransferase, lactate dehydrogenase, alkaline phosphatase, gamma-glutamyl transferase, total bilirubin, albumin, total protein, blood urea nitrogen, creatinine, uric acid, total cholesterol, high-density lipoprotein cholesterol, low-density lipoprotein cholesterol, triglycerides, glucose, sodium, potassium, and chloride. The study was approved by the Institutional Review Board of the Chiyoda Paramedical Care Clinic and the Meiji Institutional Review Board. The study was also performed in accordance with the ethical standards of the 1964 Declaration of Helsinki and its later amendments.
Experimental procedures
The study was conducted at the Chiyoda Paramedical Care Clinic. The subjects visited the clinic a total of three times. During the first visit, a baseline blood sample (10 mL) was collected and analyzed. Maximal oxygen uptake VO2max (mL/kg/min) and maximal heart rate (HR) (beats/min) were measured as described previously.Citation17) Following incremental exercise (15 W/min) on a cycle ergometer, the subjects continued cycling until exhaustion, defined as meeting two or more of the following criteria: (1) the subjects felt they could no longer continue (>19 on the Borg scale), (2) the point at which oxygen consumption plateaued, (3) ratio of respiratory exchange >1.1, and (4) HR ≥ 200 beats/min. The results of exhaustion testing were used to calculate the power output equivalent to 50% VO2max. The remaining two visits were separated by at least six days. Dietary intake was self-recorded by the subjects from their first until last visit. The subjects were instructed to refrain from binge eating, strenuous exercise, or drinking alcohol for 24 h prior to each trial and were also instructed to sleep more than eight hours the evening before each visit. On the days of the second and third visits, the subjects consumed the meals provided at least 6 h before each trial. All the meals had the same carbohydrate:fat:protein ratio (71:16:13) and contained 612 kcal. The subjects had no food or drink except water from the last meal to the start of each trial. Individual trials were performed at a similar time of the day for each subject (±3 h) to avoid any influence of circadian rhythm on the results.
During the second and third visits, the subjects participated in the main experimental trials. After measurement of blood pressure and HR, blood samples were drawn from the antecubital vein. The subjects were randomized to ingest 50 mL of ordinary tap water and either a cellulose capsule containing 3 g of A-mix (Kyowa Hakko Bio Co, Ltd., Tokyo, Japan) as the active sample or a cellulose capsule containing 3 g of dextrin (Matsutani Chemical Industry Co., Ltd., Hyogo, Japan) as the placebo (designated 0 min). The treatments were subsequently switched during the crossover phase of the study. After sitting for 30 min (rest period), the subjects mounted a cycle ergometer and commenced cycling for 60 min at a constant power output equivalent to 50% VO2max (Exercise period). After exercising, the subjects rested for 60 min in the supine position (post-Exercise period). Blood samples were collected every 30 min during rest and post-exercise and every 15 min during exercise. HR was recorded and exhaled air samples collected throughout the rest, exercise, and post-exercise phases. Blood pressure and HR were recorded again at the end of the post-exercise period. The tests were conducted in a quiet environment in a controlled room at a temperature of 21 ± 2 °C and humidity of 45 ± 5%. The study design is summarized in Fig. .
Exhaled gas analysis
Exhaled oxygen and carbon dioxide concentrations were measured by the breath-by-breath method using a respiration metabolism monitor system (AE-310s, Minato Medical Science Co., Ltd., Osaka, Japan). Substrate oxidation rates were calculated from the respiratory exchange ratio (RER).Citation18) Substrate oxidation was calculated every 5 min based on the expiratory gas measurements. To calculate the amount of fat and carbohydrate oxidation, we used Péronnet and Massicotte’s method; 1.695 × VO2 – 1.701 × VCO2 for fat and 4.585 × VCOCitation2-3.226 × VCO2 for carbohydrate.Citation18)
Blood sampling
Whole blood collected in a vacutainer containing sodium fluoride and ethylenediaminetetraacetic acid (EDTA)-2Na was cooled to 4 °C for later analysis of glucose concentrations. Whole blood from a EDTA-2Na vacutainer with added aprotinin was centrifuged immediately at 1200 g for 10 min at 4 °C, and the plasma then separated and frozen immediately at −80 °C for later analysis of glucagon concentration. Whole blood from an EDTA-2Na vacutainer without added aprotinin was centrifuged immediately at 1200 g for 10 min at 4 °C, and the plasma then separated into two vials. One vial was cooled to 4 °C for later analysis of cortisol concentration, while the other vial was frozen immediately at −80 °C for later analysis of adrenalin and noradrenalin concentrations. Whole blood from a plain vacutainer was allowed to stand at room temperature for 20 min, and then centrifuged at 1200 g for 10 min at 4 °C, followed by separation of the serum into two vials. One vial was cooled to 4 °C for later analysis of FFA, growth hormone, and insulin concentrations, and the other vial frozen at −80 °C for later analysis of acetoacetic acid, 3-hydroxybutyrate, and glycerol concentrations. Whole blood from a regular syringe was transferred to a new vial containing 0.8 N HClO4 to remove protein and then centrifuged at 1200 g for 10 min at 4 °C. The deproteinized serum was frozen at −80 °C for later analysis of lactate concentration.
Glucagon concentrations were measured by a double-antibody radioimmunoassay (Glucagon RIA SML, Euro-Diagnostica AB, Malmö, Sweden) and insulin by a chemiluminescent immunoassay (Architect Insulin, Abbott Japan, Japan). Enzymatic methods were used to measure the levels of blood glucose (Iatoro LQ GLU, Unitica, Japan), acetoacetic acid, 3-hydroxybutyrate (Total ketone bodies Kainos, 3-HB Kainos, Kainos Co., Ltd., Japan), glycerol (Glycerol Colorimetric Assay Kit, Cayman Chemical, Ann Arbor, MI, USA), and lactate (Detamina-LA, Kyowa Medex Co., Ltd., Japan). Blood FFAs (NEFA-SS Eiken, Eiken Chemical Co., Ltd., Japan) were measured by the enzyme-ultraviolet (UV) method, and growth hormone (Access hGH, Beckman Coulter, Inc., USA) and cortisol (Access cortisol, Beckman Coulter, Inc., USA) concentrations by a chemiluminescent enzyme immunoassay. Adrenalin and noradrenalin were measured using high-performance liquid chromatography (HLC-725CAΠ, Tosoh Corporation, Tokyo, Japan). The assays used to measure glucagon, insulin, blood glucose, acetoacetic acid, 3-hydroxybutyrate, lactate, FFAs, growth hormone, adrenalin, and blood chemistry panels were performed at the LSI Medience Corporation (Tokyo, Japan), and the glycerol assay at IMUH Co., Ltd. (Tokyo, Japan).
Statistical analysis. Data were expressed as mean ± standard deviation (SD) and were analyzed using Microsoft Excel (Microsoft Corp., Redmond, WA, USA). The time-related AUCs for blood concentrations of circulating hormones were calculated using the trapezium rule. Repeated measures two-factor analysis of variance (ANOVA, time-treatment) was used to examine differences between the biochemical parameters in the two trials. When the ANOVA showed significant effects or interactions between factors, Tukey’s post hoc test was used to detect significant differences between the two treatments. To specifically compare the AUCs for each hormone between treatments, we divided the experiment into three time phases (i.e., rest, exercise, and post-exercise). All variables were tested for normal distribution by the F-test using StatView-J 5.0 software (Abacus Concepts, Berkeley, CA, USA). The statistical significance of differences between the two trials was analyzed by a paired-sample t test using Microsoft Excel for normally distributed data and the Wilcoxon signed-rank test using StatView-J 5.0 software for data with a skewed distribution. Statistical significance was set at a p value < 0.05.
Results
Cardiorespiratory responses
The changes in HR, RER, carbohydrate oxidation, and fat oxidation are shown in Table . No differences in HR and carbohydrate oxidation were observed between the A-mix and placebo treatments during rest. During the rest period, RER was significantly lower with the A-mix than with placebo treatment (p = 0.038). Carbohydrate oxidation during exercise was significantly lower with the A-mix compared with that measured with placebo treatment (p = 0.041). No differences in fat oxidation were observed post-exercise between the A-mix treatment and placebo (p = 0.064). There was also no difference in total energy expenditure in subjects when they received either A-mix supplementation or placebo (598.3 ± 45.7 vs. 613.6 ± 70.0 kcal/150 min, respectively).
Table 1. Cardiorespiratory responses of participants during rest, exercise, and post-exercise after consuming the treatment.
Biochemical parameters
The biochemical parameters measured with the two treatments are summarized in Fig. and AUC for each condition during rest, exercise, recovery is summarized in Table . A two-factor ANOVA showed a significant treatment-time interaction for serum total ketone bodies concentration (treatment, p = 0.128; time, p < 0.0001; interaction, p = 0.0126), with Tukey’s post hoc test revealing significant differences between treatments at 75, 90, 120, and 150 min (p < 0.05). And post-exercise AUC for ketone bodies was significantly higher following supplementation with the A-mix compared with that measured with placebo. In the case of the concentrations of serum glycerol and blood glucose, a two-factor ANOVA revealed significant main effect of time, and no significant interaction. However, the post-exercise AUC for glycerol was significantly higher, and the post-exercise AUC for glucose was significantly lower following supplementation with the A-mix compared with that measured with placebo.
Fig. 2. Concentrations of biochemicals assessed during the experimental trials: glycerol (A), FFAs (B), total ketone bodies (C), glucose (D), and lactate (E).
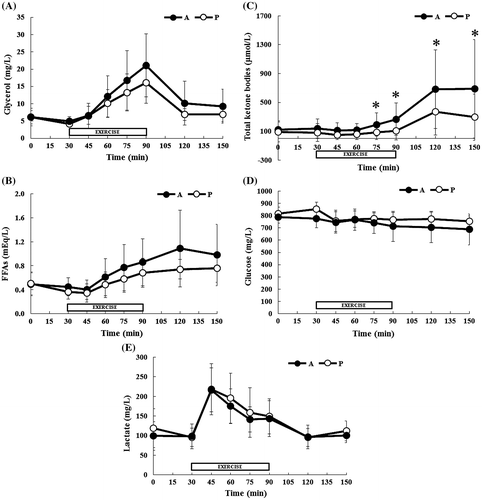
Table 2. Effects of amino acid mixture on the AUC for biochemical parameters.
Circulating hormones
The concentration of circulating hormones during each treatment is summarized in Fig. and AUC for each condition during rest, exercise, recovery is summarized in Table . A two-factor ANOVA showed a significant treatment–time interaction for plasma adrenalin concentration (treatment, p = 0.220; time, p < 0.0001; interaction, p = 0.047), with Tukey’s post hoc test revealing significant differences between treatments at 60, 75, 90, 120, and 150 min (p < 0.05). However, the AUC for adrenalin did not significantly differ between the two treatments. In the cases of the plasma/serum concentrations of glucagon, insulin, noradrenalin, and cortisol, a two-factor ANOVA revealed significant main effect of time, and no significant interaction. However, the post-exercise AUC for glucagon was significantly higher following supplementation with the A-mix treatment compared with that measured with placebo (p = 0.042). Insulin, noradrenalin, growth hormone, and cortisol concentrations did not differ significantly between the two treatments throughout the experimental period.
Fig. 3. Concentrations of circulating hormones during the experimental trials: glucagon (A), insulin (B), adrenalin (C), noradrenalin (D), growth hormone (E), and cortisol (F).
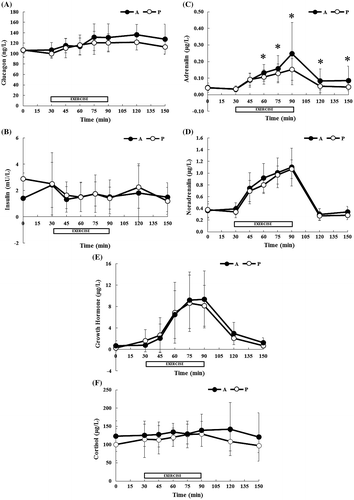
Table 3. Effects of amino acid mixture on the AUC for circulating hormones.
Discussion
This study in physically active, healthy young men investigated the acute effects of A-mix supplementation combined with exercise on glucagon secretion, fat catabolism, and energy expenditure. The study showed that compared with ingestion of placebo, ingestion of the A-mix supplement significantly increased the concentrations of glycerol (post-exercise) and ketone bodies (during exercise and post-exercise). The rate of lipolysis in adipose tissue can be estimated from the glycerol production since glycerol formed by lipolysis cannot be reutilized in this tissue due to low concentrations of α-glycerokinase.Citation19) In accordance with this lipolysis phenomena, the A-mix supplement significantly lowered carbohydrate oxidation during exercise, while fat oxidation tended to increase compared with that measured with placebo during the post-exercise period. These results suggested that ingestion of A-mix caused a shift of energy source from carbohydrate to fat combustion during and after exercise.
Previous reports suggested that several widely divergent effects of adrenalin and glucagon appear to be mediated by a common effector, adenosine 3′,5′-cyclic monophosphate.Citation20) The cyclic AMP cascade plays crucial roles in the activation of hormone-sensitive triacylglycerols lipase (HSL) and subsequent hydrolysis of triacylglycerols in adipose tissues.Citation21) Our study showed that the blood level of adrenalin during and post-exercise and the AUC for glucagon post-exercise were significantly higher after subjects received the A-mix treatment compared with administration of placebo. These results suggested that lipolysis via c-AMP-dependent phosphokinase activity and HSL activity induced by exercise may be increased by administration of A-mix combined with exercise compared with exercise alone. A further in vivo trial is necessary to investigate whether or not HSL is related to this cascade.
We observed a significant increase not only in the blood levels of glycerol, but also in total ketone bodies in the A-mix group compared with the placebo group. The increase in blood ketone body levels suggested increased hepatic ketogenesis. These results also suggested that the A-mix increased utilization of fat as a substrate. Abe et al. reported that serum free fatty acid and blood ketone body concentrations were increased in swimming mice following p.o. administration of a Vespa Amino Acid Mixture (VAAM) compared to those that received a casein amino acid mixture.Citation22) Sasai et al. also reported that regular exercise combined with ingestion of VAAM appeared to slightly increase aerobic fitness and decrease intra-abdominal fat in sedentary older women.Citation23) These results suggest that continuous administration of A-mix combined with exercise may have beneficial effects on fat metabolism if administrated continuously over a long term with regular exercise. Future studies such as long-term prospective studies are necessary to determine whether this acute response induced by administration of A-mix is sustained if the supplement is ingested for several months.
Several reports have suggested that increasing blood glucagon levels may accelerate whole body energy expenditure. For example, Tan et al. reported that glucagon infusion acutely increased energy expenditure in humans,Citation16) while isolated brown fat cells from rats have been shown to respond thermogenically to glucagon.Citation24) There is also evidence that glucagon administration to rodents increases BAT mass and activity.Citation25) In our study, although glucagon secretion was significantly higher with A-mix ingestion compared with placebo, total energy expenditure and energy expenditure after exercise were not different. However, fat catabolism induced by exercise continues for one hour after exercise. A further study is necessary to measure daily energy consumption using a metabolic chamber.
The balance of several hormones including glucagon, insulin, catecholamine, growth hormone, and cortisol reportedly plays a critical role in fat catabolism, both at rest and during exercise.Citation14,15) We found that ingestion of the A-mix caused a significant increase in catecholamine concentration, especially adrenalin, during and after exercise. Catecholamine is synthesized from tyrosine in the human body. Phenylalanine, a tyrosine precursor, is a substrate for tyrosine hydroxylase, the enzyme that catalyzes the rate-limiting step in catecholamine synthesis.Citation26) Several reports have suggested that a mixture of AAs lacking tyrosine and phenylalanine rapidly depletes blood tyrosine and phenylalanine concentrations in both rodents and humans,Citation27–29) and reduces brain tyrosine concentration in rats.Citation30) Several reports have also shown that administration of a mixture of AAs lacking tyrosine and phenylalanine lowers the ratio of plasma catecholamine precursors to other competing AAs.Citation27,28,30,31) The current data therefore imply that ingestion of the A-mix, especially the phenylalanine content, plays an important role in increasing the concentration of adrenalin in blood.
Several AAs when combined with resistance exercise are known to accelerate fat oxidation by increasing the levels of growth hormone and insulin-like growth factor-1.Citation32,33) In particular, ingestion of 1.5 g of arginine stimulated secretion of growth hormone.Citation34) However, in the current study, growth hormone levels remained changed indicating that the A-mix stimulates fat catabolism via a growth hormone-independent pathway. Isidori et al.Citation35) reported that oral ingestion of a mixture of arginine and lysine stimulated secretion of insulin and growth hormone, whereas ingestion of either AA alone did not elicit the same effect. We therefore speculate that stimulation of growth hormone by arginine may be affected by the composition of the AAs administered.
In conclusion, pre-exercise ingestion of the A-mix supplement significantly accelerated secretion of adrenalin during a period of exercise and secretion of glucagon during the post-exercise phase. Furthermore, lipolysis and fat catabolism—especially hepatic ketogenesis—during and after exercise increased significantly, indicating a shift toward fat catabolism. Taken together, these results indicate that ingestion of a combination of specific AAs efficiently stimulates fat catabolism during exercise. A major limitation of this study was that we only investigated 10 young men. Future clinical trials are necessary in humans with obesity, especially in older adults.
Author Contributions
K. Ueda, C. Sanbongi, S. Takai, S. Ikegami, and S. Fujita designed the study. K. Ueda wrote the manuscript, and S. Fujita supervised manuscript preparation. All authors reviewed and approved the final manuscript.
Disclosure statement
K. Ueda, C. Sanbongi, S. Takai, and S. Ikegami are employees of Meiji Co., Ltd.
Funding
This work was supported by a Meiji Co., Ltd.
Acknowledgments
The authors thank all the volunteers for taking part in these studies, and thank Dr Shukuko Ebihara and the staff of CPCC Co., Ltd. for data collection.
References
- Batsis JA, Nieto-Martinez RE, Lopez-Jimenez F. Metabolic syndrome: from global epidemiology to individualized medicine. Clin Pharmacol Ther. 2007;82:509–524.10.1038/sj.clpt.6100355
- Zou C, Shao J. Role of adipocytokines in obesity-associated insulin resistance. J Nutr Biochem. 2008;19:277–286.10.1016/j.jnutbio.2007.06.006
- National Institutes of Health. Office of medical applications of research: methods for voluntary weight loss and control, NIH Technology Assessment Conference Statement. Ann Intern Med. 1993;119(7):641–770.
- Jakicic JM. Exercise in the treatment of obesity. Endocrinol Metab Clin North Am. 2003;32:967–980.10.1016/S0889-8529(03)00075-6
- American College of Sports Medicine. Clinical exercise testing. In: Whaley MH editor. ACSM’s guidelines for exercise testing and prescription 7th ed. Philadelphia (PA): Lippincott Williams & Wilkins; 2006. p. 93–114.
- Henriksen EJ. Invited review: effects of acute exercise and exercise training on insulin resistance. J Appl Physiol. 2002;93:788–796.10.1152/japplphysiol.01219.2001
- Richter EA, Kiens B, Mizuno M, et al. Insulin action in human thighs after one-legged immobilization. J Appl Physiol. 1989;67(1):19–23.
- Guoyao Wu. Amino acids: metabolism, functions, and nutrition. Amino Acids. 2009;37:1–17.
- Nuttall FQ, Schweim KJ, Gannon MC. Effect of orally administered phenylalanine with and without glucose on insulin, glucagon and glucose concentrations. Horm Metab Res. 2006;38:518–523.10.1055/s-2006-949523
- Gerich JE, Charles MA, Grodsky GM. Regulation of pancreatic insulin and glucagon secretion. Annu Rev Physiol. 1976;38:353–388.10.1146/annurev.ph.38.030176.002033
- Gannon MC, Nuttall JA, Nuttall FQ. The metabolic response to ingested glycine. Am J Clin Nutr. 2002;76:1302–1307.
- Malaisse-Lagae F, Welsh M, Lebrun P, et al. The stimulus-secretion coupling of amino acid-induced insulin release: secretory and oxidative response of pancreatic islets to L-asparagine. Diabetes. 1984;33:464–469.10.2337/diab.33.5.464
- Müller WA, Aoki TT, Cahill GFJ. Effect of alanine and glycine on glucagon secretion in postabsorptive and fasting obese man. J Clin Endocrinol Metab. 1975;40:418–425.10.1210/jcem-40-3-418
- Wasserman DH, Spalding JA, Lacy DB, et al. Importance of intrahepatic mechanisms to gluconeogenesis from alanine during exercise and recovery. Am J Physiol. 1989;257:E108–E117.
- Wolfe RR, Nadel ER, Shaw JHF, et al. Role of changes in insulin and glucagon in glucose homeostasis in exercise. J Clin Invest. 1986;77:900–907.10.1172/JCI112388
- Tan TM, Field BC, McCullough KA, et al. Coadministration of glucagon-like peptide-1 during glucagon infusion in humans results in increased energy expenditure and amelioration of hyperglycemia. Diabetes. 2013;62:1131–1138.10.2337/db12-0797
- Ueda K, Nakamura Y, Yamaguchi M, et al. Amino acid mixture enriched with arginine, alanine, and phenylalanine stimulates fat metabolism during exercise. Int J Sport Nutr Exercise Metab. 2016;26:46–54.10.1123/ijsnem.2015-0137
- Peronnet F, Massicotte D. Table of nonprotein resspiratory quotient: an update. Can J Sport Sci. 1991;16:23–29.
- Koschinsky T, Gries FA. Glycerol kinase and lipolysis in human adipose tissue in relation to relative body weight. Hoppe Seylers Z Physiol Chem. 1971;352(1):430–432.10.1515/bchm2.1971.352.1.430
- Blecher M, Merlino NS, Ro’Ane JT, et al. Independence of the effects of epinephrine, glucagon, and adrenocorticotropin on glucose utilization from those on lipolysis in isolated rat adipose cells. J Biol Chem. 1969;244(13):3423–3429.
- Bahr R, Hansson P, Sejersted OM. Triglyceride/fatty acid cycling is increased after exercise. Metabolism. 1990;39(9):993–999.10.1016/0026-0495(90)90313-2
- Abe T, Inamori M, Iida K, et al. The activation of fatty acid metabolism by Vespa amino acid mixture (VAAM) and related nutrients during endurance exercise in mice. Adv Exercise Sports Physiol. 1997;3:35–44.
- Sasai H, Matsuo T, Fujita M, et al. Effects of regular exercise combined with ingestion of vespa amino acid mixture on aerobic fitness and cardiovascular disease risk factors in sedentary older women: a preliminary study. Geriatr Gerontol Int. 2011;1:24–31.10.1111/ggi.2011.11.issue-1
- Howland RJ, Bond KD. Modulation by insulin and glucagon of noradrenaline-induced activation of isolated brown adipocytes from the rat. Eur J Biochem. 1987;169:155–166.10.1111/ejb.1987.169.issue-1
- Billington CJ, Bartness TJ, Briggs J, et al. Glucagon stimulation of brown adipose tissue growth and thermogenesis. Am J Physiol. 1987;252(1 Pt 2):R160–R165.
- Fernstrom JD, Fernstrom MH. Tyrosine, phenylalanine, and catecholamine synthesis and function in the brain. J Nutr. 2007;137:1539S–48S.
- Gijsman HJ, Scarnà A, Harmer CJ, et al. A dose-finding study on the effects of branch chain amino acids on surrogate markers of brain dopamine function. Psychopharmacology. 2002;160:192–197.10.1007/s00213-001-0970-5
- McTavish SF, McPherson MH, Harmer CJ, et al. Antidopaminergic effects of dietary tyrosine depletion in healthy subjects and patients with manic illness. Br J Psychiatry. 2001;179:356–360.10.1192/bjp.179.4.356
- Moja EA, Lucini V, Benedetti F, et al. Decrease in plasma phenylalanine and tyrosine after phenylalanine-tyrosine free amino acid solutions in man. Life Sci. 1996;28:58(26):2389–2395.10.1016/0024-3205(96)00242-1
- McTavish SF, Cowen PJ, Sharp T. Effect of a tyrosine-free amino acid mixture on regional brain catecholamine synthesis and release. Psychopharmacology. 1999a;141:182–188.10.1007/s002130050823
- McTavish SF, McPherson MH, Sharp T, et al. Attenuation of some subjective effects of amphetamine following tyrosine depletion. J Psychopharmacol. 1999b;13:144–147.10.1177/026988119901300205
- Elam RP. Morphological changes in adult males from resistance exercise and amino acid supplementation. J Sports Med Phys Fitness. 1988;28(1):35–39.
- Zajac A, Poprzecki S, Zebrowska A, et al. Arginine and ornithine supplementation increases growth hormone and insulin-like growth factor-1 serum levels after heavy-resistance exercise in strength-trained athletes. J Strength Cond Res. 2010;24(4):1082–1090.10.1519/JSC.0b013e3181d321ff
- Suminski RR, Robertson RJ, Goss FL, et al. Acute effect of amino acid ingestion and resistance exercise on plasma growth hormone concentration in young men. Int J Sport Nutr. 1997;7:48–60.10.1123/ijsn.7.1.48
- Isidori A, Monaco AL, Cappa M. A study of growth hormone release in man after oral administration of amino acids. Curr Med Res Opin. 1981;7:475–481.10.1185/03007998109114287