Abstract
Knowledge of platelet count and function is key to ensuring appropriate hemostatic management. We hypothesized that the novel, portable TEG®6s coagulation assessment system could evaluate the contribution of both platelet count and function to clot formation. Whole-blood samples with variable platelet counts were prepared from healthy volunteers. Platelet function was adjusted using seven concentrations of abciximab and evaluated by light transmission aggregometry (LTA) with TRAP agonist. Maximum amplitude (MA), reaction time (R) and activated clotting time (ACT) were assessed in citrated kaolin (CK), CK with heparinase (CKH), citrated RapidTEG® (CRT), and citrated functional fibrinogen (CFF) assays. Positive correlations were observed between platelet count and CK.MA, CKH.MA, and CRT.MA (p < .0001), and CK.R, CKH.R, and CRT.ACT (p < .05). Platelet count could be accurately quantified in the range 28–91 k/μL, 28–86 k/μL and 28–74 k/μL for CK.MA, CKH.MA, and CRT.MA, respectively. CK.MA, CKH.MA, and CRT.MA showed significant negative relationships with abciximab concentration (p < .001). Platelet function inhibition was detected by all three assays at >68% measured by LTA and quantified in the range 68.4–82% (CK), 69.4–88% (CKH), and 69.7–76% (CRT). This demonstrates the TEG®6s analyzer can accurately evaluate platelet count and function at the site-of-care.
Introduction
Platelets play an essential role in maintaining hemostasis by facilitating the activation of the coagulation cascade. In an uninjured vessel, platelets do not adhere to the vessel wall or aggregate; however, disruption to a vessel wall allows a procoagulant stimulus to come into contact with plasma, facilitating platelet activation, aggregation, and accumulation. This results in large-scale thrombin generation and leads to clot formation [Citation1].
Low platelet counts are associated with increased bleeding and mortality, while platelet dysfunction can occur even when the count is within the normal range [Citation2,Citation3]. This is supported by a study investigating platelet function which found that on admission, 45% of trauma patients had normal platelet counts, but impaired platelet function that was associated with an almost 10-fold increase in mortality [Citation3]. Platelet dysfunction appears integral to trauma-induced coagulopathy (TIC), although threshold criteria for triggering therapy are yet to be established [Citation4]. Surgery involving cardiopulmonary bypass (CPB) is another clinical scenario in which platelet dysfunction is associated with coagulopathic bleeding [Citation5]. During CPB, reversible downregulation of platelet surface glycoproteins and membrane receptors for vWF and fibrinogen occurs [Citation5,Citation6]. Therefore, across different clinical settings, it is important to assess platelet function as well as platelet count in patients with an established or potential need for coagulation therapy.
TEG® (Haemonetics Corporation, Braintree, MA, USA) has been used in clinical practice for decades to quantify fibrinolysis, coagulability, and the effects of antithrombotic and anticoagulant therapy [Citation7,Citation8]. TEG® hemostasis analyzers measure the viscoelastic properties of a clot from the enzymatic phase through to the fibrinolytic phase [Citation9]. Maximum amplitude (MA) represents the strength of the clot, as a result of platelet-fibrin interactions via the αIIbβ3 receptor, and the contribution of platelets to clot strength has been the focus of several studies [Citation10–Citation12]. Current conventional tests such as complete blood count (CBC) only measure platelet count; however, platelet function is also a critical component for the management and treatment of bleeding. Light transmission aggregometry (LTA) is a traditional platelet function test, but it is poorly standardized and unlikely to be used widely in clinical practice [Citation13]. Therefore, there is an unmet need for a device that can rapidly evaluate the contribution of both platelet count and function to clot formation at the site-of-care.
The TEG®6s system (Haemonetics, Braintree, MA) is a fully automated, novel, portable instrument, employing a four-channel cartridge system to assess patients’ coagulation status using the established TEG® assays. Compared with its predecessor, the TEG® 5000 analyzer (Haemonetics, Braintree, MA), TEG®6s enables a significant reduction in the volume of blood required for coagulation assessment and simplified assay procedures that reduce the time taken to obtain test results [Citation7,Citation9,Citation14]. The citrated multichannel (CM) cartridge results in platelet activation via thrombin generation pathways, and facilitates the simultaneous performance of the citrated kaolin (CK), citrated kaolin with heparinase (CKH), citrated rapidTEG® (CRT), and citrated functional fibrinogen (CFF) assays. The primary parameters affected by platelet function are the MA for CK, CKH, and CRT. The TEG® PlateletMapping® (PLM®) cartridge was later developed to detect the effect of antiplatelet therapy by allowing selective activation of the P2Y12 receptor by ADP or acetylsalicylic acid.
The primary aim of this study was to explore the independent contribution of platelet count and platelet function to the MA and R (reaction time) parameters in the traditional TEG assays contained in the TEG®6s CM cartridge.
Methods
Study Design
This study was conducted in accordance with Good Clinical Practice (GCP) guidelines and the ethical principles of the Declaration of Helsinki [Citation15]. Ethical approval, informed consent, and approval by the relevant authorities were obtained prior to the initiation of the study.
Sample Collection
The study was conducted using blood samples from healthy donors (n = 65 samples each for platelet count analysis and platelet function analysis). For each donor, a draw of 3 mL of blood to a discard syringe followed by a draw of 60 mL blood drawn by venipuncture was performed, using three 20 mL syringes. The blood from the syringes was transferred into 4.5 mL vacutainer tubes coated with 3.2% Sodium citrate (Becton Dickinson, Franklin Lakes, New Jersey) within 1 min of blood draw. Thirteen samples were prepared from each donor: whole blood (n = 1), platelet-rich plasma (PRP) (n = 1), and whole blood with variable platelet levels (0–600 k/μL) (n = 11).
Platelet Count Variation
Platelet count was altered by harvesting platelet-poor plasma (PPP), platelet pellets, and packed red blood cells (RBCs) by centrifugation and mixing these constituents in appropriate ratios. A platelet-free sample comprised donor plasma (free from platelets) mixed with donor RBCs to match the original RBC count.
Constant coagulation factor levels and hematocrit ratios were maintained for each donor during sample preparation and for each platelet count. This was confirmed by measuring samples’ fibrinogen activity using the Clauss method. Platelet activation was controlled by using multiple centrifugation steps and apyrase for platelet harvesting. LTA with TRAP agonist was performed using PRP samples to confirm normal platelet function.
Platelet Function Variation
Abciximab is a humanized, hybrid, monoclonal antibody that binds to the αIIbβ3 receptor, thereby blocking fibrinogen binding and subsequent platelet aggregation. Whole-blood samples were investigated with seven concentrations of abciximab (range 1.9–20.0 μg/mL), in addition to a baseline sample and a negative control with saline. These concentrations only required the addition of small volumes of abciximab, enabling coagulation factor levels and platelet counts to be preserved. The inhibitory effects of abciximab were confirmed by LTA with TRAP agonist. Previous studies have used threshold values of 70–81% [Citation16,Citation17], and so we used a threshold value of 75% for determining the presence or absence of platelet inhibition (i.e., platelet inhibition deemed to be present if the LTA TRAP value was ≤75%).
Platelet counts of all samples analyzed by TEG®6s were assessed and confirmed by CBC (Complete Blood Count) (Medonic M-series Hematology analyzer) testing. Based on a recommendation from the Scientific and Standardization Committee of the International Society on Thrombosis and Hemostasis (SSC/ISTH), there were no adjustments of the platelet counts of PRP samples [Citation18].
Statistical Analysis
The relationships between TEG®6s parameters and platelet count or abciximab concentration were studied using generalized linear or nonlinear models. Various transformations for both dependent (TEG®6s parameters) and independent variables (platelet count and function) were considered in order to derive the optimal framework. The selection of final models was based on model quality metrics, including residual analysis and the Akaike information criterion (AIC).
A linear mixed-effects model was used to assess the significance of platelet count in explaining TEG®6s parameters. The platelet count was set as the fixed effect, and the donor was set as the random effect to account for the occurrence of inter-donor variation. Autoregressive process was used as the correlation model. Platelet count was log-transformed (log10) and fitted against the TEG®6s parameters for MA parameters. Platelet counts at the lower and upper bounds of the reference ranges for CK.MA, CKH.MA, and CRT.MA were also calculated. No variable transformations were used for R parameters. A binomial model-based approach was used to explore the ability of CK.MA, CKH.MA, and CRT.MA to detect low platelet counts (<150 k/µL), where the predicted probability of low platelets was calculated for a given CK.MA, CKH.MA, and CRT.MA value, and a predicted probability of >70% used to predict a low platelet count.
A mixed-effect, four-parameter logistic model was used to assess the significance of abciximab concentration with respect to the TEG®6s CK.MA, CKH.MA, and CRT.MA parameters. A linear mixed-effects model was used to assess the significance of abciximab concentration in explaining the TEG®6s CFF.MA. The abciximab concentration was set as the fixed effect, and the donor was set as the random effect to account for inter-donor variation. The values for LTA.TRAP in 3D plots were estimated using the modeling of LTA.TRAP versus Abciximab. The estimate represents the model-based average using all available data on donors. No variable transformations were employed.
Average differences in values for TEG® parameters over relevant intervals of platelet count and abciximab concentration were calculated with 95% bootstrap confidence intervals. Regression modeling was used to estimate the platelet count and abciximab concentration at the limits of the reference ranges for CK.MA, CKH.MA, and CRT.MA.
Results
Maximum Amplitude
Platelet Count Effects
TEG®6s parameters CK.MA, CKH.MA, and CRT.MA increased with increasing platelet count (). There was a significant relationship demonstrated between platelet count and CK.MA (β = 24.957, p < .0001), CKH.MA (β = 24.710, p < .0001), and CRT.MA (β = 25.478, p < .0001). No significant relationship was demonstrated between platelet count and CFF.MA.
Figure 1. Relationship of platelet count with (a) CK.MA, (b) CKH.MA, and (c) CRT.MA. Reference ranges for the TEG® parameters are shown in green. Gray lines represent the point of statistical difference from the lower and upper bounds of the reference range; Brown line represents the point of saturation. Abbreviations: CK, citrated kaolin; CKH, citrated kaolin with heparin; CRT, citrated RapidTEG®; MA, maximum amplitude.
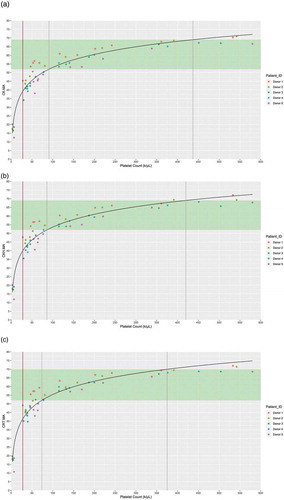
All MAs showed significant change over the ranges considered (p < .05). For the low platelet count range (0–150 k/μL), a mean change (mm) was 0.28, 0.28, and 0.29 for CK.MA, CKH.MA, and CRT.MA. For the higher platelet count range (150–450 k/μL), a mean change (mm) was 0.03, 0.04, and 0.04 for CK.MA, CKH.MA, and CRT.MA. Estimated platelet counts for the lower and upper bounds of the reference ranges for CK.MA, CKH.MA, and CRT.MA were 91 and 437 k/μL, 86 and 420 k/μL, and 74 and 375 platelet/μL, respectively.
Platelet count significantly differed from the reference range at 91 k/μL for CK.MA, 86 k/μL for CKH.MA and 74 k/μL for CRT.MA (shown by the lower gray line in ) corresponding to MA values of 52 for all three parameters. Platelet count can be accurately quantified in the range of 28–91 k/μL for CK.MA, 28–86 k/μL for CKH.MA and 28–74 k/μL for CRT.MA (range between the brown line to the lower gray line in ). For detecting low platelet count (<150 k/uL), the binomial model gave a sensitivity of 92% and specificity of 94% with CK.MA and CKH.MA variables, and sensitivity of 95%, specificity 94% with CRT.MA.
Platelet Function Effects
CK.MA, CKH.MA, and CRT.MA showed statistically significant relationships with the concentration of abciximab (p < .001; ). Increased concentrations of abciximab were associated with lower values for CK.MA, CKH.MA, and CRT.MA values, with negative Spearman correlations of ρ = −0.901, ρ = −0.900, and ρ = −0.877, respectively. The lowest abciximab concentrations to produce a statistically significant difference from the TEG® reference range in the value of CK.MA, CKH.MA, and CRT.MA was 3.2 μg/mL, 3.3 μg/mL, and 3.3 μg/mL, respectively (dashed black line on ), which corresponds to an MA value of 52 for all three parameters. No statistically significant relationship was observed between abciximab concentration and CFF.MA (β = −0.012, p = .24).
Figure 2. Relationship of abciximab concentration with (a) CK.MA, (b) CKH.MA, and (c) CRT.MA as correlated with LTA. The individual donor model is provided in colored dashed lines, with the overall model in black. Brown line represents the point of difference from baseline. Vertical dashed black line indicates the point of significant difference from the TEG® reference range.
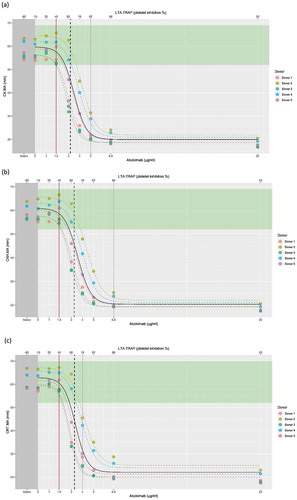
Platelet inhibition, as measured by LTA TRAP, increased with increasing abciximab concentration (). shows the model-based average using all available donor data. Inhibition of platelet function significantly differed from the reference range at 68.4%, 69.4%, and 69.7% inhibition (dashed black line on ), corresponding to MA values of 45.4, 45.7, and 44.2 for CK, CKH, and CRT assays, respectively. TEG®6s can therefore accurately quantify platelet function within a range of 68.4–82% for CK. 69.4–88% for CKH and 69.7–76% for CRT (range shown by the black-dashed line to gray line; ).
Reaction Time and Activated Clotting Time (ACT)
Platelet Count Effects
As shown in , statistically significant relationships were observed between platelet count and R-time in the CK and CKH assays (β = −0.002, p < .001; β = −0.002, p < .005), and ACT in the CRT assay (β = −0.019, p < .05). The lowest platelet count for which CK.R, CKH.R, and CRT.ACT showed significantly different values compared with a platelet count of zero was 7 k/μL. Despite the statistical associations, all three parameters showed only small differences in their values between low and higher platelet counts, suggesting that the relationships are not clinically meaningful.
Figure 3. Linear mixed-effects model of (a) CK.R, (b) CKH.R, and (c) CRT.ACT in relation to the platelet count (k/μL), with the donor as a random effect. Individual donor model is provided in colored dashed lines, with the overall model in black. Reference range limits are shown in green. Abbreviations: ACT, activated clotting time; CK, citrated kaolin; CKH, citrated kaolin with heparinase; CRT, citrated RapidTEG®; R, reaction time.
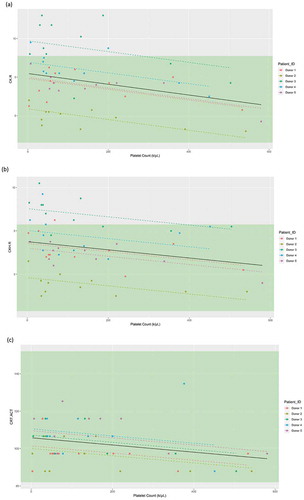
Platelet Function Effects
Contrary to platelet count, abciximab had no statistically significant effect on CK.R (β = −0.02, p = .14), CKH.R (β = −0.005, p = .64), or CRT.ACT (β = −0.13, p = .58) (). The lowest abciximab concentration to show a statistically significant effect on CRT.ACT versus a platelet count of zero was 5 μg/mL. For CK.R and CKH.R, no abciximab concentration produced a statistically significantly different value versus zero.
Figure 4. Linear mixed-effects model of (a) CK.R, (b) CKH.R, and (c) CRT.ACT in relation to the abciximab concentration with the donor as the random effect. The individual donor model is provided in colored dashed lines, with the overall model in black. Parameter reference range limits are shown in green. Abbreviations: CK, citrated kaolin; CKH, citrated kaolin with heparinase; CRT, citrated RapidTEG®; MA, maximum amplitude.
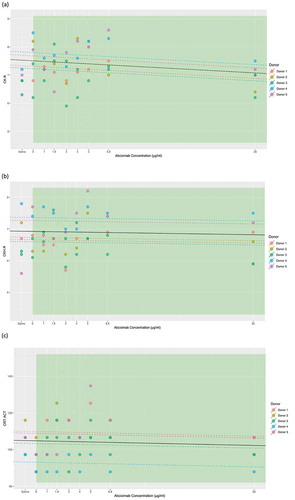
Discussion
Thromboelastography is an established method that has been used for decades as a diagnostic tool in several therapeutic areas. The portable, new-generation TEG analyzer has been recently introduced which provides a holistic overview of the coagulation status of a patient allowing health-care providers to identify a problem and provide targeted, goal-directed therapy at the site-of-care [Citation7,Citation9,Citation19]. This was the first study to investigate the impact of both platelet count and platelet function on coagulation parameters measured using the new TEG®6s device. The study shows that TEG is able to detect platelet count below the reference range at 91 k/μL for CK.MA, 86 k/μL for CKH.MA and 74 k/μL for CRT.MA, and quantify platelet levels within a range. Furthermore, using LTA TRAP as a confirmatory assay, we have demonstrated that the TEG®6s analyzer can accurately detect platelet function inhibition, which may allow earlier and more directed therapy.
Platelets are integral to coagulation, and an understanding of the patient’s platelet count and function is important in ensuring that hemostatic therapy is optimized. Conventional coagulation tests such as prothrombin time (PT), and activated partial thromboplastin time (aPTT) are plasma based and, therefore, insensitive to changes in platelet count or function [Citation1]. Other coagulation tests, such as CBC, provide a platelet count without assessing the contribution that platelets make to the clotting process (i.e., platelet function). TEG®6s assays are performed using whole blood and analyzing the interaction of all blood components provides comprehensive insight into the patient’s coagulation status. Comparisons to other whole-blood platelet function tests Multiplate® and VerifyNow® show TEG®6s is able to distinguish between healthy blood spiked with different concentrations of platelet inhibitor, with TEG®6s showing the best score for internal distribution of disagreement (5.0% for TEG®6s, 8.3% for VerifyNow® and 13.3% for Multiplate®) and lowest variability (5.1% for TEG®6s, 17.7% for VerifyNow® and 14.1% for Multiplate®) [Citation20]. The TEG®6s is also smaller, more portable, and less sensitive to vibrations than previous models [Citation9,Citation21].
Relationships between platelet count or function and TEG® parameters have been studied using previous thromboelastography devices. In one such study, performed using PRP or PPP with CaCl2 as the coagulation activator, both MA and the coagulation time (K-time) increased linearly with log-transformed platelet count, with platelet counts below 66 k/μL associated with dysfunctional clotting [Citation22]. A subsequent study was performed using whole blood and, as in the earlier study, linear relationships were observed between log-transformed platelet count and either MA or K-time [Citation23]. No significant relationship was found between R-time and platelet count in either of these studies. The limitations of these studies were addressed in the current study, to provide further evidence of the ability of TEG® to analyze the hemostatic function of platelets.
TEG®-guided algorithms are used for platelet management in a variety of clinical settings. Compared with conventional coagulation assay protocols, a TEG®-guided approach can improve morbidity and mortality rates and decrease blood product use in both trauma [Citation24–Citation26] and cardiac surgery [Citation27–Citation29]. Accurate and rapid analysis of platelet count and function can help ensure appropriate management of hemostasis, decreasing the risks of bleeding and thromboembolic events. The relationships described in this study between platelet count or platelet function and MA parameters show that the TEG®6s analyzer can provide the required level of guidance. Not only can the TEG®6s CM cartridge tests detect low platelet levels, they are also able to quantify both count and function within the reported ranges, giving a holistic overview of a patient’s coagulation status and potentially allowing for earlier or more direct therapy.
We observed strong positive relationships between platelet count and CK.MA, CKH.MA, and CRT.MA, showing that stronger clots were formed at higher platelet counts. The high sensitivity of the TEG®6s assays was reflected by the association of platelet counts below 74–92 k/μL with sub-normal range values that significantly differed from the reference range for CK.MA (91 k/μL), CKH.MA (86 k/μL) and CRT.MA (74 k/μL). In addition to being able to identify platelet counts below the reference range, platelet count could be accurately quantified with high sensitivity in the range of 28–91 k/μL for CK.MA, 28–86 k/μL for CKH.MA and 28–74 k/μL for CRT.MA. Below these ranges, the TEG®6s can detect a low platelet count but cannot accurately quantify it.
A significant inverse relationship was seen between platelet function, as determined by abciximab-modulated aggregation, and CK.MA, CKH.MA, and CRT.MA. Small changes in these parameters were observed at low levels of abciximab, with marked decreases when a threshold abciximab concentration was reached and small changes at higher abciximab concentrations reflecting response saturation. Furthermore, using LTA TRAP as a confirmatory assay, we have demonstrated that the TEG®6s analyzer can accurately detect platelet function inhibition at levels >68%, below the established cutoff value, and quantify platelet function within a range of 68.4–82% for CK. 69.4–88% for CKH and 69.7–76% for CRT. Below this range, TEG®6s can detect that platelet function is inhibited, as correlated with LTA, but cannot accurately quantify it. This suggests that TEG® is more sensitive than LTA, with TEG® parameters showing more gradual changes with increasing levels of abciximab.
The small but statistically significant reductions in reaction time parameters (CK.R, CKH.R, and CRT.ACT) with increasing platelet counts are consistent with the cell-based model of hemostasis [Citation1]. Accelerated clot formation at higher platelet concentrations may be due to the increased number of phospholipid membranes providing a substrate for tenase and prothrombinase complexes [Citation1]. During the propagation phase of the hemostatic process, tenase and prothrombinase complexes are assembled on the platelet surface, and large-scale thrombin generation takes place. A lower platelet count decreases the speed of this process. Unlike platelet count, changes in platelet function did not affect reaction time parameters in our study. This may be explained by the fact that provision of surfaces for the hemostatic process by platelets is independent of their function. Alternatively, it is possible that abciximab, through steric hindrance and conformational changes, prevents binding to the αIIbβ3 receptor, blocking secondary platelet activation, but not the initial platelet activation. This would mean that platelets continue to be activated and generate thrombin, leading to a feedback loop increasing the number of available platelets. When the effects of both MA and reaction time parameters are combined, the TEG®6s analyzer can be used to accurately monitor functional platelets at any count.
The main limitation of this study was that it focused on clotting via thrombin generation, and only examined one platelet function activator. The study focused on inhibition of αIIbβ3 and was not set up to explore other important aspects of clotting which may have affected the platelet function (such as the role of GP1B, release of granule contents and externalized phospholipids). The effects of platelet number and function were studied separately, and the effect of both platelet number and function varying simultaneously was not examined by the study. In this study, the experiment was not set up to manipulate both platelet count and platelet function. Specific, independent measurements of platelet count and platelet function were not performed, meaning that the relative contributions of these two elements to the TEG® parameters could not be determined. Furthermore, the role of flow and sheer could not be investigated in this in vitro study. While clotting via thrombin generation pathways can be valuable for interventional cardiology [Citation30] and stroke [Citation31], it does not fully address platelet activation via ADP or clinical scenarios with the use of dual antiplatelet therapy [Citation32,Citation33].
In these clinical settings, TEG® PLM® should be used to quantify platelet function, including the contribution of ADP and thromboxane A2 receptors to clot formation. Results obtained using the PLM® cartridge have been shown to have negative predictive value for bleeding and thrombotic risks in patients undergoing cardiac surgery [Citation34,Citation35], non-cardiac surgery [Citation36], and percutaneous coronary intervention [Citation10,Citation30]. Additionally, in surgical patients, compared with no preoperative platelet function testing, the use of the PLM® cartridge was shown to significantly reduce blood product utilization [Citation34,Citation37], and benefits have been shown in guiding treatment for patients with trauma [Citation38]. This was an in vitro experiment, and so the manipulations needed to create the artificial conditions may have contributed to platelet activation or had other unintended effects; however, platelet blocker was utilized to minimize these effects. The participants in our current study were healthy volunteers, and studies are needed in patients with coagulopathy; however, our study serves as a proof-of-concept for the measurement of both platelet count and function using the TEG®6s. Due to these limitations, no conclusions can be drawn from this study on the impact of the results on clinical hemostasis. Future studies may be designed using this analyzer with multiple cofounders varied individually within the same population, and larger trials may explore use in surgical situations to evaluate the clinical benefit of TEG®6s guided platelet transfusions versus those guided by platelet count alone. It would also be interesting to explore how the TEG parameters react to bleeding management strategies such as pooled platelets or transfusions, and whether TEG is able to detect recovery of platelet function following these strategies.
TEG®6s CM cartridge assay parameters are sensitive to the contributions of platelet count and platelet function to clot formation and strength. This is the first study to show that TEG®6s can detect platelet function inhibition correlated with LTA, as determined by the points at which TEG values differ from the reference range. Furthermore, not only can the TEG®6s detect either low platelet count or low platelet function, it can also quantify platelet count and platelet function inhibition within the reported ranges which may allow earlier, more direct targeted medical intervention. Thus, we can accept the hypothesis that, contrary to conventional coagulation tests, the TEG®6s analyzer can be used to evaluate the net effect of both platelet count and function. By ensuring appropriate hemostatic therapy, accurate monitoring of platelet count and function could potentially lead to decreased morbidity and mortality, as well as lower, more effective use of allogeneic blood products. This study provides further evidence to support the use of the portable TEG®6s analyzer to diagnose and monitor the hemostatic status of patients across a range of clinical settings at the site-of-care.
Co-authors contribution
JD and CL contributed equally to the study design, data analysis, data interpretation, and manuscript review. CL was the main contributor to the initial experimental design and data analysis, while JD was the main contributor to the later data analysis, interpretation and manuscript development. KB and PG contributed to the data interpretation and manuscript review. JH and HA contributed to the data analysis and manuscript review. All authors approved the final manuscript for publication in Platelets. The corresponding author confirms he had full access to the data in the study and has final responsibility for the decision to submit for publication.
Declaration of interest
JD, CL, JH, and HA are or were employees of Haemonetics Corporation at the time of the study. This study was supported by Haemonetics Corporation (Braintree, Massachusetts). The authors have no other relevant financial interest in the products or companies described in this article. PG received honoraria for lectures, consultations, including service on speakers’ bureaus from Bayer, Merck, Janssen, Medicure, and World Medical; receiving grants from the National Institutes of Health, Janssen, Merck, Bayer, Haemonetics, Instrumentation Labs, and Amgen. PG is holding stock or stock options in Merck, Medtronic, and Pfizer; and holding patents in the area of personalized antiplatelet therapy and interventional cardiology.
Acknowledgements
The authors thank Meridian HealthComms, Plumley, UK for providing medical writing support, which was funded by Haemonetics SA, Signy, Switzerland in accordance with Good Publication Practice (GPP3). The authors also thank ClinStatDevice LLC, Lexington, MA, USA for providing biostatistics support, which was funded by Haemonetics Corp., Braintree, MA, USA. Finally, the authors would like to thank Elmar R. Burchardt MD, PhD for his comments on this work.
Additional information
Funding
References
- Hoffman M, Monroe DM 3rd. A cell-based model of hemostasis. Thromb Haemost 2001;85:958–965. doi:10.1055/s-0037-1615947.
- Brown LM, Call MS, Knudson MM, Cohen MJ, Group TO. A normal platelet count may not be enough: the impact of admission platelet count on mortality and transfusion in severely injured trauma patients. J Trauma 2011;71:S337. doi:10.1097/TA.0b013e318227f67c.
- Kutcher ME, Redick BJ, McCreery RC, Crane IM, Greenberg MD, Cachola LM, Nelson MF, Cohen MJ. Characterization of platelet dysfunction after trauma. J Trauma Acute Care Surg 2012;73:13. doi:10.1097/TA.0b013e318256deab.
- Chang R, Cardenas JC, Wade CE, Holcomb JB. Advances in the understanding of trauma-induced coagulopathy. Blood 2016;128:1043–1049. doi:10.1182/blood-2016-01-636423.
- Varghese S, Unni M, Mukundan N, Ramji RL. Platelet functions in cardiopulmonary bypass surgery. Med J Armed Forces India 2005;61:316–321. doi:10.1016/S0377-1237(05)80053-X.
- Rinder CS, Mathew JP, Rinder HM, Bonan J, Ault KA, Smith BR. Modulation of platelet surface adhesion receptors during cardiopulmonary bypass. Anesthesiology 1991;75:563–570. doi:10.1097/00000542-199110000-00004.
- Hartmann J, Mason D, Achneck H. Thromboelastography (TEG) point-of-care diagnostic for hemostasis management. Point Care 2018;17:15–22.
- Ramiz S, Hartmann J. Clinical utility of viscoelastic testing (TEG and ROTEM analyzers) in the management of old and new therapies for hemophilia. Am J Hematol 2019;94:249–256. doi:10.1002/ajh.25319.
- Gurbel PA, Bliden KP, Tantry US, Monroe AL, Muresan AA, Brunner NE, Lopez-Espina CG, Delmenico PR, Cohen E, Raviv G. First report of the point-of-care TEG: a technical validation study of the TEG-6S system. Platelets 2016;27:642–649. doi:10.3109/09537104.2016.1153617.
- Gurbel PA, Bliden KP, Navickas IA, Mahla E, Dichiara J, Suarez TA, Antonino MJ, Tantry US, Cohen E. Adenosine diphosphate-induced platelet-fibrin clot strength: a new thrombelastographic indicator of long-term poststenting ischemic events. Am Heart J 2010;160:346–354. doi:10.1016/j.ahj.2010.05.034.
- Mousa SA, Khurana S, Forsythe MS. Comparative in vitro efficacy of different platelet glycoprotein IIb/IIIa antagonists on platelet-mediated clot strength induced by tissue factor with use of thromboelastography: differentiation among glycoprotein IIb/IIIa antagonists. Arterioscler Thromb Vasc Biol 2000;20:1162–1167. doi:10.1161/01.ATV.20.4.1162.
- Plotkin AJ, Wade CE, Jenkins DH, Smith KA, Noe JC, Park MS, Perkins JG, Holcomb JB. A reduction in clot formation rate and strength assessed by thrombelastography is indicative of transfusion requirements in patients with penetrating injuries. J Trauma Acute Care Surg 2008;64:S64–S68. doi:10.1097/TA.0b013e318160772d.
- Paniccia R, Priora R, Liotta AA, Abbate R. Platelet function tests: a comparative review. Vasc Health Risk Manag 2015;11:133. doi:10.2147/VHRM.
- Dias JD, Haney EI, Mathew BA, Lopez-Espina CG, Orr AW, Popovsky MA. New-generation thromboelastography: comprehensive evaluation of citrated and heparinized blood sample storage effect on clot-forming variables. Arch Pathol Lab Med 2017;141:569–577. doi:10.5858/arpa.2016-0088-OA.
- Association WM. World medical association declaration of Helsinki: ethical principles for medical research involving human subjects. JAMA 2013;310:2191. doi:10.1001/jama.2013.281053.
- Platton S, McCormick A, Bukht M, Gurney D, Holding I, Moore GW. A multicenter study to evaluate automated platelet aggregometry on Sysmex CS-series coagulation analyzers-preliminary findings. Res Pract Thromb Haemost 2018;2:778–789. Epub 2018/ 10/24. doi:10.1002/rth2.12140.
- Reece MJ, Klein AA, Salviz EA, Hastings A, Ashworth A, Freeman C, Luddington RJ, Nair S, Besser MW. Near-patient platelet function testing in patients undergoing coronary artery surgery: a pilot study. Anaesthesia 2011;66:97–103. Epub 2011/ 01/25. doi:10.1111/anae.2011.66.issue-2.
- Cattaneo M, Cerletti C, Harrison P, Hayward CP, Kenny D, Nugent D, Nurden P, Rao AK, Schmaier AH, Watson SP, et al. Recommendations for the standardization of light transmission aggregometry: a consensus of the working party from the platelet physiology subcommittee of SSC/ISTH. J Thromb Haemost 2013;11:1183–1189. doi:10.1111/jth.2013.11.issue-6.
- Dias JD, Sauaia A, Achneck HE, Hartmann J, Moore EE. Thromboelastography-guided therapy improves patient blood management and certain clinical outcomes in elective cardiac and liver surgery and emergency resuscitation: a systematic review and analysis. J Thromb Haemost 2019;17:984–994. Epub 2019/ 04/05. doi:10.1111/jth.2019.17.issue-6.
- Dias JD, Pottgiesser T, Hartmann J, Duerschmied D, Bode C, Achneck HE. Comparison of three common whole blood platelet function tests for in vitro P2Y12 induced platelet inhibition. J Thromb Thrombolysis 2019. doi:10.1007/s11239-019-01971-1.
- Neal MD, Moore EE, Walsh M, Thomas S, Callcut RA, Kornblith LZ, Schreiber M, Ekeh AP, Singer AJ, Lottenberg L, et al. A comparison between the TEG® 6s and TEG® 5000 analyzers to assess coagulation in trauma patients. J Trauma Acute Care Surg 9000. Published Online Ahead of Print. doi:10.1097/TA.0000000000002545.
- Oshita K, Az-ma T, Osawa Y, Yuge O. Quantitative measurement of thromboelastography as a function of platelet count. Anesth Analg 1999;89:296–299. doi:10.1097/00000539-199908000-00006.
- Bowbrick VA, Mikhailidis DP, Stansby G. Influence of platelet count and activity on thromboelastography parameters. Platelets 2003;14:219–224. doi:10.1080/0953710031000118849.
- Gonzalez E, Moore EE, Moore HB, Chapman MP, Chin TL, Ghasabyan A, Wohlauer MV, Barnett CC, Bensard DD, Biffl WL, et al. Goal-directed hemostatic resuscitation of trauma-induced coagulopathy: A pragmatic randomized clinical trial comparing a viscoelastic assay to conventional coagulation assays. Ann Surg 2016;263:1051–1059. doi:10.1097/SLA.0000000000001608.
- Johansson PI, Sorensen AM, Larsen CF, Windelov NA, Stensballe J, Perner A, Rasmussen LS, Ostrowski SR. Low hemorrhage-related mortality in trauma patients in a Level I trauma center employing transfusion packages and early thromboelastography-directed hemostatic resuscitation with plasma and platelets. Transfusion 2013;53:3088–3099. doi:10.1111/trf.2013.53.issue-12.
- Stensballe J, Ostrowski SR, Johansson PI. Viscoelastic guidance of resuscitation. Curr Opin Anaesthesiol 2014;27:212–218. doi:10.1097/ACO.0000000000000051.
- Ak K, Isbir CS, Tetik S, Atalan N, Tekeli A, Aljodi M, Civelek A, Arsan S. Thromboelastography-based transfusion algorithm reduces blood product use after elective CABG: a prospective randomized study. J Card Surg 2009;24:404–410. doi:10.1111/jcs.2009.24.issue-4.
- Royston D, von Kier S. Reduced haemostatic factor transfusion using heparinase-modified thrombelastography during cardiopulmonary bypass. Br J Anaesth 2001;86:575–578. doi:10.1093/bja/86.4.575.
- Shore-Lesserson L, Manspeizer HE, DePerio M, Francis S, Vela-Cantos F, Ergin MA. Thromboelastography-guided transfusion algorithm reduces transfusions in complex cardiac surgery. Anesth Analg 1999;88:312–319. doi:10.1097/00000539-199902000-00016.
- Gurbel PA, Bliden KP, Guyer K, Cho PW, Zaman KA, Kreutz RP, Bassi AK, Tantry US. Platelet reactivity in patients and recurrent events post-stenting: results of the PREPARE POST-STENTING Study. J Am Coll Cardiol 2005;46:1820–1826. doi:10.1016/j.jacc.2005.07.041.
- Elliott A, Wetzel J, Roper T, Pivalizza E, McCarthy J, Wallace C, Hess MJ, Peng H, Rahbar MH, Sangha N, et al. Thromboelastography in patients with acute ischemic stroke. Int J Stroke 2015;10:194–201. doi:10.1111/j.1747-4949.2012.00919.x.
- Castellino FJ, Chapman MP, Donahue DL, Thomas S, Moore EE, Wohlauer MV, Fritz B, Yount R, Ploplis V, Davis P, et al. Traumatic brain injury causes platelet adenosine diphosphate and arachidonic acid receptor inhibition independent of hemorrhagic shock in humans and rats. J Trauma Acute Care Surg 2014;76:1169–1176.
- Gurbel PA, Bliden KP, Navickas IA, Mahla E, Dichiara J, Suarez TA, Antonino MJ, Tantry US, Cohen E. Adenosine diphosphate–induced platelet-fibrin clot strength: A new thrombelastographic indicator of long-term poststenting ischemic events. Am Heart J 2010;160:346–354. doi:10.1016/j.ahj.2010.05.034.
- Mahla E, Suarez TA, Bliden KP, Rehak P, Metzler H, Sequeira AJ, Cho P, Sell J, Fan J, Antonino MJ, et al. Platelet function measurement-based strategy to reduce bleeding and waiting time in clopidogrel-treated patients undergoing coronary artery bypass graft surgery: the timing based on platelet function strategy to reduce clopidogrel-associated bleeding related to CABG (TARGET-CABG) study. Circ Cardiovasc Interventions 2012;5:261–269. doi:10.1161/CIRCINTERVENTIONS.111.967208.
- Sivapalan P, Back AC, Ostrowski SR, Ravn HB, Johansson PI. Transfusion requirements in elective cardiopulmonary bypass surgery patients: predictive value of Multiplate and Thromboelastography (TEG) platelet mapping assay. Scand J Clin Lab Invest 2017;77:345–351. doi:10.1080/00365513.2017.1325000.
- Kasivisvanathan R, Abbassi-Ghadi N, Kumar S, Mackenzie H, Thompson K, James K, Mallett SV. Risk of bleeding and adverse outcomes predicted by thromboelastography platelet mapping in patients taking clopidogrel within 7 days of non-cardiac surgery. Br J Surg 2014;101:1383–1390. doi:10.1002/bjs.2014.101.issue-11.
- Agarwal S, Johnson RI, Shaw M. Preoperative point-of-care platelet function testing in cardiac surgery. J Cardiothorac Vasc Anesth 2015;29:333–341. doi:10.1053/j.jvca.2014.06.025.
- Tapia NM, Chang A, Norman M, Welsh F, Scott B, Wall MJ Jr., Mattox KL, Suliburk J. TEG-guided resuscitation is superior to standardized MTP resuscitation in massively transfused penetrating trauma patients. J Trauma Acute Care Surg 2013;74:378–385. discussion Epub 2013/ 01/29. doi:10.1097/TA.0b013e31827e20e0.