ABSTRACT
In this study, we present an inner filter effect-based immunoassay (IFE-IA) for the detection of acetamiprid with a turn off–on model. In the IFE-IA, the monoclonal antibody (mAb) conjugated with upconversion nanoparticles (UCNPs) were set as a fluorescence source, and the antigen conjugated with gold nanoparticles (AuNPs) were set as the fluorescence switch off reagent, while acetamiprid acts as the fluorescence turn-on reagent. After optimization, the concentration of acetamiprid producing 50% saturation of the signal (SC50) was 0.04 µg/L, and the detection range (SC10–SC90) was 0.002–0.58 µg/L. The IFE-IA showed no cross-reactivity with the analogues of acetamiprid except for thiacloprid (36.4%). The average recoveries of the immunoassay for spiked samples were in the range of 75.1–104.7%. In addition, the assay showed good correlation with high-performance liquid chromatography in the detection of blind samples.
Introduction
Acetamiprid (N-[(6-chloro-3-pyridyl)methyl]-N’-cyano-N-methyl-acetamidine), a widely used neonicotinoid insecticide, has been registered in more than 120 countries, which works as nicotinic acetylcholine receptor agonist to interfere with the nervous system of insects to cause paralysis or death (Hladik et al., Citation2018; Matsuda et al., Citation2005). Due to the low toxicity to vertebrates and highly effective insecticidal activity, acetamiprid has become one of the best-selling insecticides around the world (Chara-Serna et al., Citation2019; Kumar et al., Citation2013). However, many studies have proved that acetamiprid has an adverse impact on non-target organisms including invertebrates and vertebrates, especially pollinators (Imamura et al., Citation2010; Sanchez-Bayo et al., Citation2016; Terayama et al., Citation2018). In recent years, some countries have prohibited the registration of acetamiprid, even France added acetamiprid and thiacloprid to the banned list (Biodiversity Act 2016). Besides, most countries have legislated the maximum residue limits (MRLs) for acetamiprid in foods, such as the MRLs in European Union range from 5 mg/kg in rucola to 0.01 mg/kg in the strawberries (Regulation (EC) No 396/2005). In China, the MRLs range from 5 mg/kg in the spinage to 0.01 mg/kg in the eggs according to GB 2763–2019. Therefore, it is necessary to detect acetamiprid residues in the environment and agricultural products.
Due to the advantages of sensitivity, simplicity, low-cost and high-throughput, immunoassays based on specific binding of antigens and antibodies have been increasingly used to detect pesticides, including acetamiprid (You et al., Citation2017). Wanatabe et al. established an indirect competitive enzyme-linked immunosorbent assay (ic-ELISA) using a monoclonal antibody (mAb), the 50% inhibitory concentrations (IC50) was 1.3 ng/mL (Wanatabe et al., Citation2001). Watanabe et al. reported a direct competitive enzyme-linked immunosorbent assay (dc-ELISA) for acetamiprid, the limit of detection (LOD) was 0.015 ng/mL (Watanabe et al., Citation2018). Fang et al. established a biotinylated indirect competitive ELISA (Bic-ELISA) based on a biotinylated anti-acetamiprid mAb for the detection of acetamiprid, the LOD was 0.17 ng/mL (Fang et al., Citation2019). Liu et al. developed an immunochromatographic strip (ICS) to detect acetamiprid in cucumber and apple samples, the cut-off limits were found to be 5 ng/mL (Liu et al., Citation2017). In these methods, ELISAs show high sensitivity and stability, but they have the shortcomings of needing washing steps, long incubation time and relying on laboratory conditions. The ICS is suitable for fast, simple and on-site detection, but its sensitivity is lower than the ELISAs. Therefore, it is urgent to establish sensitive immunoassays with simple operation and fast detection.
Homogeneous immunoassays, including fluorescence polarization (Ding et al., Citation2019), fluorescence resonance energy transfer (Hu et al., Citation2016), bioluminescence resonance energy transfer (Arts et al., Citation2016) and inner filter effect (IFE) (Chen et al., Citation2017), are popular on account of omitting separation steps and saving detection time. Among them, IFE is easy to achieve because it is a non-radiative energy conversion model in which the light of fluorescer is absorbed by the absorber (Yao et al., Citation2006). The IFE occurs when the absorption spectrum of the absorber overlaps with the emission spectrum of fluorescer (Fang et al., Citation2017). So, appropriate fluorescer–absorber pair is an important factor for the successful construction of IFE-based immunoassays (IFE-IA). Gold nanoparticles (AuNPs) are one of the effective absorbers with ultra-high extinction coefficients and wide energy bands, which can effectively absorb the fluorescence emitted by fluorescers (Wu et al., Citation2017; Yun et al., Citation2016). Upconversion nanoparticles (UCNPs) have been widely used in immunoassays due to their advantages of near-infrared excitation (typically 980 nm), large Stokes shifts, long fluorescence lifetimes, high resistance to photobleaching and low toxicity (Chen et al., Citation2020; Chen et al., Citation2021; Long et al., Citation2015). In recent years, the IFE-IAs based on the fluorescer–absorber pair of UCNPs-AuNPs have been successfully developed to detect pesticides, including imidaclothiz and imidacloprid (You et al., Citation2017; Si et al., Citation2018). The IFE-IAs mentioned above have the advantages of simple operation, high sensitivity and fast detection, and their detection signals were proportional to the concentration of the analyte (positive readout) because of a turn off–on model.
In this study, an anti-acetamiprid mAb was prepared to develop an IFE-IA for acetamiprid by using mAb-conjugated UCNPs as fluorescer and antigen-conjugated AuNPs as absorber. The sensitivity, specificity and accuracy of the IFE-IA were evaluated by standard curve, detection of acetamiprid analogues and spiked recovery, respectively. In addition, the IFE-IA was verified by high-performance liquid chromatography (HPLC) through the detection of blind samples.
Materials and methods
Reagents
Acetamiprid (99.7%) and its analogues, including thiacloprid (99.6%), imidacloprid (99.5%), clothianidin (99.4%), nitenpyram (99.1%) and thiamethoxam (99.6%), were provided by Dr. Ehrenstorfer GmbH (Augsburg, Germany). Imidaclothiz (97.82%) was obtained from Nantong Jiangshan Agrochemical and Chemicals Co., Ltd. (Nantong, China). Tetraethyl orthosilicate (TEOS, 98%), (3-aminopropyl)-triethoxysilane (APTES, 98%), tris (hydroxymethyl) aminemethane (99.9%), N-hydroxysuccinimide (NHS), N,N'-dicyclohexylcarbodiimide (DCC) and ammonium hydroxide solution (28% in H2O, ≥99.999% metals basis) were purchased from Aladdin Industrial Corporation (Shanghai, China). Freund's complete adjuvant, Freund's incomplete adjuvant, bovine serum albumin (BSA), ovalbumin (OVA) and 3,3’,5,5'-tetramethylbenzidine (TMB) were purchased from Sigma-Aldrich Chemical Co. (St. Louis, USA). 25% glutaraldehyde solution was purchased from Sinopharm Chemical Reagent Co., Ltd. (Shanghai, China). Polyoxyethylene sorbitan monolaurate (Tween-20) was provided by Solarbio (Beijing, China). Horseradish peroxidase (HRP)-labeled goat anti-mouse IgG was purchased from Boster (Wuhan, China). H2O2 (30%) was purchased from Shanghai Wokai Biotechnology Co., Ltd. (Shanghai, China). BLAB/c female mice were purchased from the Center of Comparative Medicine of Yangzhou University (Yangzhou, China). The mouse experiments were approved by the Department of Science and Technology of Jiangsu Province (license number: SYXK (SU) 2017-0007).
Preparation of artificial antigens and monoclonal antibodies
The synthesis of acetamiprid hapten was referred to Wanatabe et al. (Citation2001). The immunogen (hapten-BSA) and coating antigen (hapten-OVA) were prepared by conjugating hapten with BSA and OVA through the active ester method, respectively (Wanatabe et al., Citation2001). The hapten, carrier proteins and conjugates were scanned by an ultraviolet spectrophotometer (UV-2550, Shimadzu, Kyoto, Japan), and the coupling ratios of hapten and carrier proteins were estimated by the following formula: (ϵconjugate – ϵBSA or OVA)/ϵhapten (ϵ represents molar absorption coefficient).
Five female BALB/c mice (6–8 weeks) were injected intraperitoneally with hapten-BSA to produce mAbs. The immunization and cell fusion were carried out according to Wang et al. (Citation2011). The positive well in the cell culture plates (Corning, New York, USA) was determined by ic-ELISA, and subcloned to obtain monoclonal hybridoma cells by the limiting dilution method (Nowinski et al., Citation1979). The monoclonal hybridoma cells were injected into the abdominal cavity of mice to get ascites. The mAb was prepared by the purification of ascites through a protein A column (GE Healthcare, Pittsburgh, USA).
Ic-ELISA protocol
Coating antigen was diluted to 0.0625 µg/mL by 0.05 M carbonate bicarbonate buffer (CBS) and added to 96-well microplates (100 µL/well, Costar, Massachusetts, USA) for overnight incubation at 4°C. The plates were blocked by 1% gelatin in PBS solution (200 µL/well) for 1.5 h at 37°C. Fifty microliters of mAb (0.25 µg/mL in phosphate-buffered saline buffer, PBS) and 50 µL of acetamiprid standard were added to the well for incubation of 1 h at 37°C. The diluted HRP-labeled goat anti-mouse IgG (1:20,000 in PBS) was added to the plates (100 µL/well) for incubating 1 h at 37°C. After each of these steps, the plates were washed five times with PBS containing 0.05% Tween 20 (PBST). The bound HRP-labeled goat anti-mouse IgG was detected by the addition of 100 µL of TMB/H2O2 substrate (0.4 mmol/L TMB, 3 mmol/L H2O2, 24.3 mmol/L citrate and 26.5 mmol/L Na2HPO4.12H2O in ultrapure water), and incubated 15 min at 37°C. After adding 50 µL of 2 M H2SO4, the absorbance at 450 nm was measured by a SpectraMax M5 microplate reader (Molecular Devices, San Francisco, USA).
Preparation of antigen-conjugated AuNPs and mAb-conjugated UCNPs
The AuNPs were systhesized according to the citric acid reduction method (Frens, Citation1973). Under boiling and stirring, 1% sodium citrate solution (1.7 mL) was quickly added to 100 mL of 0.01% chloroauric acid aqueous solution. When the solution turned wine red, continued to boil for another 5 min. Then, the AuNPs were cooled to room temperature and stored at 4°C. The salt precipitation experiment was used to determine the minimum amount of acetamiprid antigen for conjugating with AuNPs (Hua et al., Citation2010). The pH of AuNPs solution was adjusted to 8.0 by 0.2 mol/L K2CO3. Eighty microliters of 2 mg/mL acetamiprid antigen (hapten-OVA) was added to 5 mL AuNPs solution, and stirred for 1 h at room temperature. The AuNPs in the solution were blocked by adding 0.56 mL of 10% BSA in 0.01 mol/L borate buffer. After stirring 1 h at room temperature, the solution was centrifuged at 10,000 rpm for 10 min at 4°C. The sediment was washed 3 times with 1% BSA, and then dispersed in 5 mL borate buffer (containing 1% BSA and 3% sucrose) and stored at 4°C.
UCNPs of NaYF4:Yb,Er have been prepared by the hydrothermal synthesis method (Sun et al., Citation2007). The surfaces of the UCNPs were amino-functionalized to provide active chemical groups for conjugating mAbs according to the classic Stöber method (Stalder & Stöber, Citation1965). Anti-acetamiprid mAb was coupled with amino-functionalized UCNPs by the glutaraldehyde crosslinking method (Hun & Zhang, Citation2007; Wilhelm et al., Citation2013). Amino-functionalized UCNPs (10 mg) were dissolved in 2.5 mL borate buffer and ultrasonized for 15 min. And then, 50 mg sodium borohydride and 0.64 mL of 25% glutaraldehyde aqueous solution were added for mild shaking 1 h at room temperature in darkness. After centrifugation at 14000 rpm for 30 min at 4°C, the sediment was washed three times by borate buffer, and resuspended by 3 mL of borate buffer. The anti-acetamiprid mAb (0.5 mg) and sodium borohydride (50 mg) were added, and slowly shaked for 1 h at room temperature. The UCNPs were blocked by the addition of Tris (50 mg) with mild shaking for 1 h. The conjugate was separated by centrifugation and washed with borate buffer three times. Finally, the conjugate was dispersed in 5 mL borate buffer and stored at 4°C.
IFE-IA protocol
For IFE-IA (), the mAb-UCNPs and antigen-AuNPs were set as fluorescence source and fluorescence switch-off reagent, respectively. In the absence of acetamiprid, the mAb-UCNPs were combined with antigen-AuNPs by the interaction of the mAb and antigen, the fluorescence was absorbed by AuNPs and a low-intensity fluorescence in the system was recorded. In the presence of acetamiprid, the intensity of fluorescence was higher because of the interaction of mAb and acetamiprid. Therefore, the concentration of acetamiprid can be detected by the change of fluorescence intensity. Specifically, acetamiprid standard solution or sample solution (250 μL) was mixed with 100 μL of antigen-conjugated AuNPs by vortex. Then, 40 μL of mAb-conjugated UCNPs and 110 μL of borate buffer containing 0.14 mol/L NaCl were added. The mixture was slowly shaken for 40 min at 37°C. The fluorescence intensity of the solution was measured by F-2700 fluorescence spectrophotometer (Hitachi, Tokyo, Japan) with a 980 nm NIR laser source (Changchun Laser Optoelectronics Technology Co., Ltd., Chuangchun, China) at 544 nm.
Optimization of IFE-IA
The experimental parameters, including the concentrations of mAb-conjugated UCNPs and antigen-conjugated AuNPs (from 0 to 1.6 nmol/L), organic solvent content (methanol content from 0 to 10%), pH value (from 5.4 to 10.4), ionic strength (NaCl concentration from 0.01 to 0.22 mol/L) and incubation time (from 10 to 60 min) were sequentially studied to determine the optimal conditions. After the optimization, the calibration curve of IFE-IA was plotted with the change values of fluorescence intensity at 544 nm (ΔI = I–I0, I represents the fluorescence intensity in the presence of acetamiprid; I0 is the fluorescence intensity in the absence of acetamiprid) as the lateral coordinates (y) and the concentration of acetamiprid as the longitudinal coordinates (x). The concentration of analyte producing 50% saturation of the signal (SC50), LOD and linear range (SC10 to SC90) were defined and calculated.
Cross-reactivity (CR)
Serial concentrations of the acetamiprid analogues (thiacloprid, imidacloprid, clothianidin, imidaclothiz, nitenpyram and thiamethoxam) were tested using the IFE-IA. The CR was estimated as follows: CR = [SC50 (acetamiprid) /SC50 (analogue)] × 100%.
Analysis of spiked samples
Soil was collected from a farm in Nanjing. Pear, wheat and cucumber were purchased from a Nanjing’s market. The soil was passed through a 2 mm sieve. The pear and cucumber were cut into small pieces and crushed to homogenate. The wheat was crushed to powder. All the samples were mixed and divided into three parts and stored in ziplock bags at –20°C. All of them were identified to be acetamiprid-free by HPLC (Saha et al., Citation2017; Watanabe et al., Citation2006). One milliliter standard solution of acetamiprid in methanol was added to 10.0 g samples in a 100-mL centrifuge tubes, the finally spiked concentrations of acetamiprid were 0.1, 1 and 10 μg/kg for soil, while 0.5, 5 and 25 μg/kg for pear, wheat and cucumber. Each spiked sample was set to three replicates.
The spiked samples (10.0 g) were mixed with 10 mL borate saline buffer (0.14 mol/L) containing 20% methanol in a 100-mL centrifuge tube. After vortexing for 3 min and sonication for 5 min, the samples were centrifugated at 4500 rpm for 5 min. The supernatant was collected into a 25-mL volumetric flask, and the sediment was extracted again. The collected supernatant was adjusted to 25 mL using the optimal buffer. The concentration of acetamiprid was detected by the IFE-IA after appropriate dilution with buffer.
Analysis of blind samples
Five pear samples spiked with unknown concentrations of acetamiprid were detected by the IFE-IA and HPLC simultaneously. The extraction and analysis of IFE-IA were the same as the analysis of spiked samples. For HPLC, 20.0 g samples were added into a triangle bottle and extracted with 50 mL acetonitrile by shaking at 200 rpm for 1 h. After suction filtration, the filtrate was mixed with 5 g NaCl and poured into a separating funnel. The organic phase was collected and the aqueous phase was extracted again by 30 mL acetonitrile. The organic phases were combined and dehydrated with anhydrous sodium sulfate. The residues in the organic phase were concentrated by a rotating evaporation and dissolved by 1 mL mobile phase (acetonitrile:water = 30:70, v/v), then filtered through 0.45 μm membrane. The concentration of acetamiprid was detected by Agilent 1260 HPLC with an ultraviolet–visible photometric detector and Eclipse pluse-C18 column (250 mm × 4.6 mm, 5 µm). The injection volume was 20 μL, the column temperature was 30 °C, the detection wavelength was 270 nm, and the flow rate was 0.8 mL/min.
Results and discussion
Identification of antigens and characterization of mAb
The hapten was determined by mass spectrometry (m/z = 293.18) and 1H NMR (400 MHz, DMSO) δ 12.34 (s, 1H), 8.38 (s, 1H), 7.56 (dd, J = 8.2, 2.2 Hz, 1H), 7.29 (dd, J = 8.2, 2.2 Hz, 1H), 4.67 (s, 2H), 3.30 (t, J = 6.9 Hz, 2H), 3.10 (s, 3H), 2.64 (t, J = 6.9 Hz, 2H), 2.41 (s, 3H). The results proved that the structure of hapten was correct. The ultraviolet absorption spectra of hapten, BSA and OVA, hapten-BSA (immunogen) and hapten-OVA (coating antigen) showed that the maximum ultraviolet absorption peaks of conjugates of hapten-BSA and hapten-OVA were deviated from the absorption peaks of BSA and OVA, which demonstrated that the antigens were prepared successfully. The coupling ratios of hapten-BSA and hapten-OVA were 25:1 and 12:1, respectively.
After cell fusion, the cell fusion rate was calculated to be 0.798‱ by dividing the number of hybridoma cell clusters by the number of SP2/0. Three positive wells in the cell culture plates were detected by the ic-ELISA, and subcloned to obtain three monoclonal hybridoma cells, named 2F11B12, 2F2B10 and 4D10B8. The 50% inhibitory concentrations (IC50) of the ic-ELISAs based on the purified mAbs of 2F11B12, 2F2B10 and 4D10B8 were 30, 8.5 and 0.15 µg/L, respectively. Compared with previous anti-acetamiprid mAbs produced by Wanatabe et al. (IC50 = 1.3 µg/L) and Liu et al. (IC50 = 1.76 µg/L), the sensitivity of the ic-ELISA based on mAb 4D10B8 was improved about 7–11 fold (Liu et al., Citation2019; Wanatabe et al., Citation2001).
Identification of UCNPs and AuNPs
The morphology of UCNPs and AuNPs was observed by transmission electron microscope (H-7650, Hitachi, Tokyo, Japan). As shown in (A, B), the UCNPs and AuNPs were spherical shape, and the diameters of UCNPs and AuNPs were about 90–110 and 20 nm, respectively. The complex of UCNP and AuNP was shown in (C). It was shown that UCNPs and AuNPs were tightly bound together through the interaction of antigen and antibody. The optical properties of UCNPs and AuNPs were shown in (D), the emission spectrum of UCNPs under the excitation of 980 nm had a significant overlap with the absorption spectrum of AuNPs.
Figure 2. TEM images of amino-modified NaYF4:Yb,Er UCNPs (A), AuNPs (B) and the complex of UCNPs and AuNPs (C). The emission spectrum of UCNPs at the excitation of 980 nm and the absorption spectrum of AuNPs (C). Effect of fluorescence quenching for mAb-conjugated UCNPs by different concentrations of antigen-coupled AuNPs (0, 0.2, 0.4, 0.6, 0.8, 1.0, 1.2, 1.4, 1.6 nmol/L) (D).
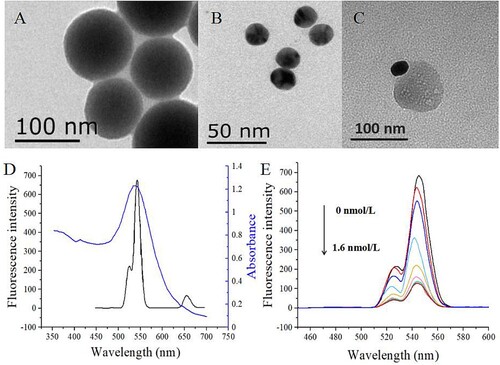
Optimal conditions of IFE-IA
The concentration of mAb-conjugated UCNPs was 0.2 mg/mL, because the fluorescence intensity was close to 1000 and the concentration was relatively low. When the concentration of antigen-conjugated AuNPs reached 1.2 nmol/L, the fluorescence intensity of IFE-IA without analyte was reduced to the minimum (E). After optimization, the optimal concentration of methanol, pH value, Na+ ionic strength and incubation time were 2.5%, 8.2, 0.15 mol/L and 40 min, respectively.
Sensitivity
Under the optimal conditions, the fluorescence intensity of IFE-IA under serial concentrations of acetamiprid is shown in (A). The calibration curve between the change values of fluorescence intensity and the logarithm of concentrations of acetamiprid was established, and the linear regression equation was y = 184.67x + 525.37 (R2 = 0.99). The SC50, LOD and linear range were 0.04, 0.002 µg/L and 0.002–0.58 µg/L, respectively (B).
Figure 3. The changes of fluorescence intensity with different concentrations of acetamiprid (A) and the calibration curve of the IFE-IA for acetamiprid (B).
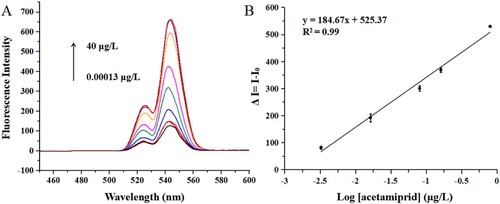
The IFE-IA was compared with the previous immunoassays for acetamiprid (). The sensitivity of the IFE method established in this study was improved 1.6–85 times than the previous methods, including the ic-ELISA (Wanatabe et al., Citation2001), dc-ELISA (Watanabe et al., Citation2018), Bic-ELISA (Fang et al., Citation2019), ICS (Liu et al., Citation2017), quantum dots-based immunochromatographic strip (QD-ICS) (Liu et al., Citation2019) and immunosensor (Xu et al., Citation2018). The developed IFE-IA has the highest sensitivity because of the high sensitivity of the produced mAb (IC50 = 0.15 µg/L in ic-ELISA) and the superiority of UCNPs. Besides, the IFE-IA has fewer detection steps and shorter detection time than the immunoassays except for ICS, because the IFE-IA is a homogeneous immunoassay. In summary, the IFE-IA is a simple, sensitive and fast method for the analysis of acetamiprid.
Table 1. The comparison of different immunoassays for acetamiprid.
Table 2. Cross-reactivity of acetamiprid toward its analogues.
Specificity
The CRs of IFE-IA for the analogues of acetamiprid were calculated (). The IFE-IA showed no CR with the analogues (<0.04%) except for thiacloprid (36.4%), because acetamiprid and thiacloprid have the common functional group of =N-CN. Besides, the result of this study is consistent with the reported immunoassays, which had CR with thiacloprid (Fang et al., Citation2019; Liu et al., Citation2019; Wanatabe et al., Citation2001; Watanabe et al., Citation2018).
Table 3. Average recoveries of samples spiked with acetamiprid.
Accuracy
The matrix interference of the samples was eliminated by dilution with buffer. A final 20-fold dilution of soil, 40-fold dilution of pear, wheat and cucumber could remove the matrix interference, because the standard curves prepared by the diluted extract were similar with that prepared by the buffer. The average recoveries of the IFE-IA for the spiked samples were 75.1−104.7% with relative standard deviations (RSDs) of 1.4–10.1% under the dilution conditions mentioned above (), which meet the standard of the International Union of Pure and Applied Chemistry (IUPAC, 70%−120% with RSD ≤ 20%). In addition, some spiked concentrations of acetamiprid in this study were lower than the lowest MRL of acetamiprid in foods in China (10 µg/kg, GB 2763-2019). Therefore, the IFE-IA holds a great promise for the detection of acetamiprid in foods.
Validation with HPLC
The validation was completed by comparing the results of IFE-IA and HPLC for the determination of five pear samples. The concentrations of acetamiprid detected by IFE-IA were in the range of 1.8–24.3 µg/kg, and the HPLC were in the range of 2.1–21.3 µg/kg. The results of IFE-IA and HPLC were not significantly different, because the P-value calculated by calculating Student’s t-test (0.92) was more than 0.05. As shown in , the correlation curve was established by plotting the results of HPLC versus the results of IFE-IA. The correlation equation was y = 1.1289x−1.0139 (R2 = 0.98), its slope is close to 1. These results indicate that the IFE-IA is the reliability and accuracy for the analysis of acetamiprid.
Conclusion
In this study, an anti-acetamiprid mAb was prepared to develop an IFE-IA for acetamiprid by using mAb-conjugated UCNPs as fluorescer and antigen-conjugated AuNPs as absorber. The mAb showed the IC50 of 0.15 µg/L in the ic-ELISA, which is better than the reported antibodies. After the optimization, the SC50, LOD and linear range of the IFE-IA were 0.04, 0.002 and 0.002−0.58 µg/L, respectively. Compared with the previous immunoassays for acetamiprid, the sensitivity of the IFE-IA was improved 1.6−85 fold. In addition, the IFE-IA showed the advantages of faster detection (40 min), simpler operation (1 step) and positive readout. The specificity, accuracy and reliability of the IFE-IA were satisfactory for the quantitative detection of residual acetamiprid. In summary, the IFE-IA is a sensitive, rapid, and simple method to detect acetamiprid in environmental and agricultural samples.
Disclosure statement
No potential conflict of interest was reported by the author(s).
Additional information
Funding
References
- Arts, R., Hartog, I. D., Zijlema, S., Thijssen, V., Stan, V., & Merkx, M. (2016). Detection of antibodies in blood plasma using bioluminescent sensor proteins and a smartphone. Analytical Chemistry, 88(8), 4525–4532. https://doi.org/10.1021/acs.analchem.6b00534
- Chara-Serna, A.M., Epele, L.B., Morrissey, C.A., & Richardson, J.S. (2019). Nutrients and sediment modify the impacts of a neonicotinoid insecticide on freshwater community structure and ecosystem functioning. Science of the Total Environment, 692, 1291–1303.
- Chen, F., Lu, Q. J., Huang, L. N., Liu, B. W., Liu, M. L., Zhang, Y. Y., & Liu, J. W. (2021). DNA triplex and quadruplex assembled nanosensors for correlating K+ and pH in lysosomes. Angewandte Chemie-International Edition, 60(10), 5453–5458. https://doi.org/10.1002/anie.202013302
- Chen, H. Y., Pang, X., Ni, Z., Liu, M., Zhang, Y., & Yao, S. (2020). Upconversion nanoparticles with bright red luminescence for highly sensitive quantifying alkaline phosphatase activity based on target-triggered fusing reaction. Analytica Chimica Acta, 1095, 146–153. https://doi.org/10.1016/j.aca.2019.10.014
- Chen, S., Yu, Y. L., & Wang, J. H. (2017). Inner filter effect-based fluorescent sensing systems: A review. Analytica Chimica Acta, 999, 13–26. https://doi.org/10.1016/j.aca.2017.10.026
- Ding, Y., Chen, H., Yang, Q., Feng, L., Hua, X. D., & Wang, M. H. (2019). A fluorescence polarization immunoassay for detection of thiacloprid in environmental and agricultural samples. RSC Advances, 9(63), 36825–36830. https://doi.org/10.1039/C9RA04776J
- Fang, A. J., Chen, H., Li, H., Liu, M., Zhang, Y., & Yao, S. (2017). Glutathione regulation-based dual-functional upconversion sensing-platform for acetylcholinesterase activity and cadmium ions. Biosensors & Bioelectronics, 87, 545–551. https://doi.org/10.1016/j.bios.2016.08.111
- Fang, Q., Zu, Q., Hua, X. D., Lv, P., Lin, W. W., Zhou, D. H., Xu, Z. H., Fan, J. R., Li, X. H., & Cao, H. Q. (2019). Quantitative determination of acetamiprid in pollen based on a sensitive enzyme-linked immunosorbent assay. Molecules, 24(7), 1265. https://doi.org/10.3390/molecules24071265
- Frens, G. (1973). Preparation of gold dispersions of varying particle size: Controlled nucleation for the regulation of the particle size in monodisperse gold suspensions. Nature Physical Science, 241(105), 20–22. https://doi.org/10.1038/physci241020a0
- Hladik, M. L., Main, A. R., & Goulson, D. (2018). Environmental risks and challenges associated with neonicotinoid insecticides. Environmental Science & Technology, 52(6), 3329−3335. https://doi.org/10.1021/acs.est.7b06388
- Hu, W. W., Chen, Q. S., Li, H. H., Ouyang, Q., & Zhao, J. W. (2016). Fabricating a novel label-free aptasensor for acetamiprid by fluorescence resonance energy transfer between NH2-NaYF4: Yb, ho@SiO2 and Au nanoparticles. Biosensors & Bioelectronics, 80, 398–404. https://doi.org/10.1016/j.bios.2016.02.001
- Hua, X. D., Qian, G. L., Yang, J. F., Hu, B. S., Fan, J. Q., Qin, N., Li, G., Wang, Y. Y., & Liu, F. Q. (2010). Development of an immunochromatographic assay for the rapid detection of chlorpyrifos-methyl in water samples. Biosensors & Bioelectronics, 26(1), 189–194. https://doi.org/10.1016/j.bios.2010.06.005
- Hun, X., & Zhang, Z. J. (2007). Functionalized fluorescent core-shell nanoparticles used as a fluorescent labels in fluoroimmunoassay for IL-6. Biosensors & Bioelectronics, 22(11), 2743–2748. https://doi.org/10.1016/j.bios.2007.01.022
- Imamura, T., Yanagawa, Y., Nishikawa, K., Matsumoto, N., & Sakamoto, T. (2010). Two cases of acute poisoning with acetamiprid in humans. Clinical Toxicology, 48(8), 851–853. https://doi.org/10.3109/15563650.2010.517207
- Kumar, A., Verma, A., & Kumar, A. (2013). Accidental human poisoning with a neonicotinoid insecticide, imidacloprid: A rare case report from rural India with a brief review of literature. Egyptian Journal of Forensic Sciences, 3(4), 123–126. https://doi.org/10.1016/j.ejfs.2013.05.002
- Liu, L. Q., Suryoprabowo, S., Zheng, Q. K., Song, S. S., & Kuang, H. (2017). Development of an immunochromatographic strip for detection of acetamiprid in cucumber and apple samples. Food and Agricultural Immunology, 28(5), 767–778. https://doi.org/10.1080/09540105.2017.1312294
- Liu, Y., Zhao, Y., Zhang, T. Y., Chang, Y. Y., Wang, S. J., Zou, R. B., Shen, L. R., & Guo, Y. R. (2019). Quantum dots-based immunochromatographic strip for rapid and sensitive detection of acetamiprid in agricultural products. Frontiers in Chemistry, 7, 76. https://doi.org/10.3389/fchem.2019.00076
- Long, Q., Li, H., Zhang, Y., & Yao, S. (2015). Upconversion nanoparticle-based fluorescence resonance energy transfer assay for organophosphorus pesticides. Biosensors & Bioelectronics, 68, 168–174. https://doi.org/10.1016/j.bios.2014.12.046
- Matsuda, K., Shimomura, M., Ihara, M., Akamatsu, M., & Sattelle, D. B. (2005). Neonicotinoids show selective and diverse actions on their nicotinic receptor targets: Electrophysiology, molecular biology, and receptor modeling studies. Bioscience Biotechnology Biochemistry, 69(8), 1442–1452. https://doi.org/10.1271/bbb.69.1442
- Nowinski, R. C., Lostrom, M. E., Tam, M. R., Stone, M. R., & Burnette, W. N. (1979). The isolation of hybrid cell lines producing monoclonal antibodies against the p15 (E) protein of ecotropic murine leukemia viruses. Virology, 93(1), 113–126. https://doi.org/10.1016/0042-6822(79)90280-0
- Saha, S., Mondal, R., Mukherjee, S., Sarkar, M., & Kole, R. K. (2017). Persistence of acetamiprid in paddy and soil under west bengal agro-climatic conditions. Environmental Monitoring and Assessment, 189(4), 150. https://doi.org/10.1007/s10661-017-5871-0
- Sanchez-Bayo, F., Goulson, D., Pennacchio, F., Nazzi, F., Goka, K., & Desneux, N. (2016). Are bee diseases linked to pesticides? − a brief review. Environment International, 89−90, 7–11. https://doi.org/10.1016/j.envint.2016.01.009
- Si, F. F., Zou, R. B., Jiao, S. S., Qiao, X. S., Guo, Y. R., & Zhu, G. N. (2018). Inner filter effect-based homogeneous immunoassay for rapid detection of imidacloprid residue in environmental and food samples. Ecotoxicology and Environmental Safety, 148, 862–868. https://doi.org/10.1016/j.ecoenv.2017.11.062
- Stalder, K., & Stöber, W. (1965). Haemolytic activity of suspensions of different silica modifications and inert dusts. Nature, 207(999), 874–875. https://doi.org/10.1038/207874a0
- Sun, Y. J., Chen, Y., Tian, L. J., Yu, Y., Kong, X. G., Zhao, J. W., & Zhang, H. (2007). Controlled synthesis and morphology dependent upconversion luminescence of NaYF4:Yb, Er nanocrystals. Nanotechnology, 18(27), 275609. https://doi.org/10.1088/0957-4484/18/27/275609
- Terayama, H., Qu, N., Endo, H., Ito, M., Tsukamoto, H., Umemoto, K., Kawakami, S., Fujino, Y., Tatemichi, M., & Sakabe, K. (2018). Effect of acetamiprid on the immature murine testes. International Journal of Environmental Health Research, 28(6), 683–696. https://doi.org/10.1080/09603123.2018.1504897
- Wanatabe, S., Ito, S., Kamata, Y., Omoda, N., Yamazaki, T., Munakata, H., Kaneko, T., & Yuasa, Y. (2001). Development of competitive enzyme-linked immunosorbent assays (ELISAs) based on monoclonal antibodies for chloronicotinoid insecticides imidacloprid and acetamiprid. Analytica Chimica Acta, 427(2), 211–219. https://doi.org/10.1016/S0003-2670(00)01126-0
- Wang, L. M., Zhang, Q., Chen, D., Liu, Y., Li, C., Hu, B., Du, D., & Liu, F. (2011). Development of a specific enzyme-linked immunosorbent assay (ELISA) for the analysis of the organophosphorous pesticide fenthion in real samples based on monoclonal antibody. Analytical Letters, 44(9), 1591–1601. https://doi.org/10.1080/00032719.2010.520391
- Watanabe, E., Miyake, S., Baba, K., Eun, H., & Endo, S. (2006). Immunoassay for acetamiprid detection: Application to residue analysis and comparison with liquid chromatography. Analytical & Bioanalytical Chemistry, 386(5), 1441–1448. https://doi.org/10.1007/s00216-006-0683-z
- Watanabe, E., Tomomi, Y., Yuki, H., Harada, A., Iwasa, S., & Miyake, S. (2018). Organic solvent-free immunoassay for quantitative detection of neonicotinoid acetamiprid residues in agricultural products. Analytical Methods, 10(26), 3162–3169. https://doi.org/10.1039/C8AY01061G
- Wilhelm, S., Hirsch, T., Patterson, W. M., Scheucher, E., Mayr, T., & Wolfbeis, O. S. (2013). Multicolor upconversion nanoparticles for protein conjugation. Theranostics, 3(4), 239–248. https://doi.org/10.7150/thno.5113
- Wu, Q., Chen, H., Fang, A., Wu, X., Liu, M., Li, H., Zhang, Y., & Yao, S. (2017). Universal multifunctional nanoplatform based on target-induced in situ promoting au seeds growth to quench fluorescence of upconversion nanoparticles. ACS Sensors, 2(12), 1805–1813. https://doi.org/10.1021/acssensors.7b00616
- Xu, X. H., Yuna, G., Liu, W., He, K., Guo, Y., Quan, W. X., & Sundaram, G. (2018). Hapten-grafted programmed probe as a corecognition element for a competitive immunosensor to detect acetamiprid residue in agricultural products. Journal of Agricultural and Food Chemistry, 66(29), 7815–7821. https://doi.org/10.1021/acs.jafc.8b02487
- Yao, X. H., Min, H., Lü, Z. H., & Yuan, H. P. (2006). Influence of acetamiprid on soil enzymatic activities and respiration. European Journal of Soil Biology, 42(2), 120–126. https://doi.org/10.1016/j.ejsobi.2005.12.001
- You, H. J., Hua, X. D., Feng, L., Sun, N. N., Rui, Q., Wang, L. M., & Wang, M. H. (2017). Competitive immunoassay for imidaclothiz using upconversion nanoparticles and gold nanoparticles as labels. Microchimica Acta, 184(4), 1085–1092. https://doi.org/10.1007/s00604-017-2097-3
- Yun, W., Jiang, J. L., Cai, D. Z., Zhao, P. X., Liao, J. S., & Sang, G. (2016). Ultrasensitive visual detection of DNA with tunable dynamic range by using unmodified gold nanoparticles and target catalyzed hairpin assembly amplification. Biosensors & Bioelectronics, 77, 421–427. https://doi.org/10.1016/j.bios.2015.09.065