Abstract
Background
Radiation burden from CT examinations increases rapidly with the increased clinical use frequency. Previous studies have disclosed the association between radiation exposure and increased double-strand breaks (DSBs) and changes in DNA methylation. However, whether the induced DSBs by CT examination recover within 24h and whether a CT examination induces detectable gene-specific methylation changes are still unclear. The aim of the present study was to analyze γ-H2AX in the peripheral blood lymphocyte (PBL) of healthy adults before and after CT examination and to discover the differentially methylated positions (DMPs) along with an analysis of DNA methylation changes caused by CT examination.
Materials and Methods
Peripheral blood samples of 4 ml were drawn from 20 healthy volunteers at three time points: before CT examination, after CT examination 1h, and after CT examination 24h. γ-H2AX immunofluorescence and Illumina Infinium Human Methylation EPIC BeadChip (850k BeadChip) were used respectively for the test of DSBs and the epigenome-wide DNA methylation analysis. Linear mixed-effect (LME) models were used to evaluate the impacts of doses represented by different parameters and foci on genome-wide DNA methylation.
Results
The number of γ-H2AX foci per cell at 1h showed linear dose–responses for the radiation doses represented by CT index volume (CTDIvol), dose length product (DLP), and blood absorbed dose, respectively. Residual γ-H2AX foci was observed after CT examination at 24h (p < .001). DMPs and γ-H2AX foci changes could be found within 1h. One CpG site related to PAX5 was significantly changed by using most of the parameters in LME models and did not recover till 24h.
Conclusions
Residual γ-H2AX foci exist after CT examination at 24h. The DNA methylation changes induced by CT examination may not recover within 24h. The DNA methylation had been changed as early as at 1h. The PAX5-related CpG site may be a potential biomarker of low-dose radiation.
Clinical Relevance
The biological effects and the cancer risks of CT examination are still unclear. The present study is an effort to document the CT scan-induced events in 24h in vivo. The CT scanning area should be strictly limited, and non-essential repeated operations shouldn’t be performed within 24h.
Introduction
Medical exposure is extensively applied to obtaining images used for diagnosis, treatment plan, and prognosis evaluation. According to the estimation from the United Nations Scientific Committee on the Effects of Atomic Radiation (UNSCEAR), medical exposure accounts for 98% of the ionizing radiation (IR) exposure from all artificial sources, and it is now the main source of artificial exposure in the world (United Nations Environment Programme Citation2016). Further investigations from UNSCEAR show that the annual total frequency of CT examinations are increased from 220 million in the year 2020 to about 400 million in the year 2021. Meanwhile, CT examinations give the largest contribution (61.6%) to the overall annual collective effective dose of medical exposure. Among the CT examinations, 26.3% are the examinations from the head and neck including the skull, facial bones, soft tissue and brain (United Nations Scientific Committee on the Effects of Atomic Radiation Citation2022a). These data show that the radiation burden from CT examinations increases rapidly.
Contrast to the global huge increase in the number of CT examinations, the knowledge of the biological effects of diagnostic radiation doses on the human body is limited. As the doses from CT examinations are commonly under 100mGy, UNSCEAR classifies it into low-dose ionizing radiation (LDIR) (United Nations Environment Programme Citation2016). The current theory that guides the practice of radiological protection is based on the linear non-threshold (LNT) model, which assumes that no minimal amount of IR dose is too little to cause cancer effect (ICRP Citation2005; NCRP Citation2020). According to this model, even though the dose from CT examination is considered ‘low’, the dose increases potential cancer risks to the patient. However, this model is still a ‘hypothesis’ not a ‘law’. This is owing to that LNT is a model which extrapolates from adverse health outcomes assessed at high doses to low doses. According to our knowledge, there is not much solid evidence about biological processes up to date in the research field of LDIR.
DNA is the principal target and DNA double-strand break (DSB) is one of the most critical harmful biological effects of radiation. Unrepaired or mis-repaired DSBs can lead to cell death or malignant transformation (Hall and Giaccia Citation2018). Currently, the most sensitive biomarker for detecting DSB induced by CT examination is γ-H2AX foci (Vinnikov and Belyakov Citation2022). At the site of DSB, histone H2 variant H2AX is phosphorylated by PI3K-related kinases at Ser139 and becomes γ-H2AX. γ-H2AX would recruit essential DNA repair proteins in downstream and form foci in nuclei. It can be quickly formed in minutes and the γ-H2AX foci can be used to represent the induction of DSBs (Mah et al. Citation2010). This method is more sensitive than others in LDIR conditions (Mah et al. Citation2010). As a sensitive and rapid method of detecting DSBs, immunofluorescence-based γ-H2AX foci test was used by numerous studies to assess the potential cancer risk of CT examinations (Lobrich et al. Citation2005; Fukumoto et al. Citation2017; Tao et al. Citation2019; Sakane et al. Citation2020; Yang et al. Citation2020; Bogdanova et al. Citation2021). In these studies, the number of γ-H2AX foci per cell in lymphocytes have significantly increased after CT examination. However, these results are not enough to prove that CT examinations do increase the risk of cancer. As DSBs are considered repairable in normal cells, the state of repair is of great concern. In our previous studies, an significant increase of γ-H2AX foci was observed in in vitro and in vivo 5 minutes after the CT examination, and the foci per cell is linear with doses. (Yang et al. Citation2020). In the present study, we intended to explore the relationship between foci and dose at somewhat later time points.
Several studies have reported that radiation-induced γ-H2AX foci reach a plateau of maximum within 5–30 minutes (Rothkamm et al. Citation2007; Kuefner et al. Citation2009; Popp et al. Citation2016). It is generally believed that DSB and γ-H2AX presence are in a one-to-one relationship during this time period (Sedelnikova et al. Citation2002). It disappears rapidly in the first few hours after 30 min and becomes slow over the following hours. It was thought that the disappearance of γ-H2AX foci might indicate the repair of DSBs (Sharma et al. Citation2015). Many in vitro studies have investigated excess γ-H2AX foci induced by ionizing radiation over longer time scales (e.g. 24h) and have shown that there is a residual foci at 24h (Rothkamm and Löbrich Citation2003; Pustovalova et al. Citation2017; Jakl et al. Citation2020). For residual foci, it was thought that it could be used to predict the complex DNA damage, which is related lethal lesions (Banáth et al. Citation2010). However, only one study has examined the excess of γ-H2AX foci in vivo after 24 h of CT scans (Lobrich et al. Citation2005), and the result was inconsistent with the in vitro study (Rothkamm and Löbrich Citation2003). This calls for in vivo studies on the kinetics of γ-H2AX foci after CT examinations.
DNA methylation, which is restricted to cytosine nucleotides in the sequence CG (annotated CpG), is the most widely studied epigenetic DNA modification. It has been associated with extensive biological effects, such as gene silencing and chromatin compaction (Tharmalingam et al. Citation2017). The stability of the DNA methylation state in normal cells is essential for their normal function (Flavahan et al. Citation2017). Insults caused by environmental factors may alter the DNA methylation state (Law and Holland Citation2019). Some of them may increase chromatin resistance, resulting in a restrictive state that blocks the differentiation of cells. Others may decrease chromatin resistance, resulting in a permissive state that allows stochastic induction of oncogenes or other adaptive process (Flavahan et al. Citation2017). LDIR, as a hazardous environmental exposure, has been observed to induce global DNA hypomethylation in nuclear power plant workers (Lee et al. Citation2015), and workers with low global methylation levels had a higher frequency of chromosome aberrations. Antwih et al. found that acute irradiation of MDA-MB-231 breast cancer cells at 2 Gy and 6 Gy for 1–72h resulted in different levels of DNA methylation changes, and differentially methylated sites (DMPs) could be isolated. (Antwih et al. Citation2013). Although several studies on air pollution have identified a range of CpG sites whose methylation levels were affected by PM2.5 in acute exposure (Li et al. Citation2018), acute LDIR exposures from medical diagnostics are not studied very well. To the best of our knowledge, there are only one in vitro study investigating the DNA methylation in human peripheral blood lymphocyte (PBL) from only three healthy participants 6h after CT examination and they didn’t find any detectable changes of DNA methylation (Becker et al. Citation2022). Based on the above research, we believe that epigenotoxic effects after LDIR, such as CT examinations, require further investigation at different time points and with larger sample sizes.
The Illumina Infinium Human MethylationEPIC BeadChip (850k BeadChip), covers over 850,000 CpG sites, is a relatively high-throughput, efficient, stable, economical and analytically friendly method (Pidsley et al. Citation2016). Its predecessor, the 450k bead chip, has been used for methylation alterations induced by ionizing radiation (Antwih et al. Citation2013). 850k bead chip retains 90% of the sites of 450k bead chip, with the addition of 413,743 CpGs. It may be a convenient tool to evaluate global level of DNA methylation and ever specific CpG site induced by LDIR. Genome-wide methylation assays allow us to do a hypothesis-free assessment of changes in genomic DNA methylation in response to CT exposures and may help us figure out which CpGs are changed and formulate pathophysiological hypotheses.
Therefore, the aim of the present study was mainly the preliminary discovery of DMPs together with the analysis of DNA methylation changes caused by CT examination at three time points (baseline, 1h and 24h). Based on this, the γ-H2AX foci levels at the three time points were examined simultaneously in healthy adult PBL before and after CT examination.
Methods
Subject
According to the review in which the factors influencing individual response to IR were introduced (Applegate et al. Citation2020), the selection criteria of participants should be strictly limited to the minimization of differences in methylation baseline statue caused by different subjects.
Thus, the inclusion criteria were:
No habits of smoking and/or drinking;
No exposure to X-rays in the past three months;
No recent infection;
No major systemic diseases, no history of hematopoietic disease, surgical and systemic medication;
No history of cancer or radiation therapy;
age >20 years old and <30 years old.
A participant was excluded when:
one of the above inclusion criteria was not met;
need more X-ray examinations during the study;
Unable to cooperate to complete the experiment
Prior to CT examination, individual participant information such as age, gender, height and weight, medical history, radiographs exposed was recorded. Body mass index (BMI) was calculated by BMI = weight/height2. Written informed consent was obtained from all participants (volunteers) at enrollment. Each participant received a financial stipend and was told they could withdraw from the study at any time. This study has been registered at Chinese Clinical Trail Registry (ChiCTR2200063358,05/09/2022) and was approved by the Institutional Review Board of Peking University School and Hospital of Stomatology (PKUSSIRB-201944052)
CT examination and sample collection
In our study, we analyzed γ-H2AX foci in health adults PBL after CT examination at three time points (baseline,1h and 24h), and used 850k to test PBL methylation. We chose the earliest possible appearance of changes in DNA methylation, 1h after CT, and the time when DSBs roughly completed repair, 24h after CT, as the detection time points.
Head and neck CT examinations were performed with a 16-slice helical CT scanner (Optima CT 520, GE Healthcare, Waukesha, WI). The examination range was from the upper margin of eyebrow arch to the lower margin of thyroid gland. The detailed information on quality control inspection and basic examination parameters were presented in the previous study (Yang et al. Citation2020).
A sample collection protocol is illustrated in . All participants waited in a room away from the radiology department before the scan and had a blood sample taken at baseline level (0h), which is within 1 hour before the CT examination. They waited until the scan to enter the CT room and left quickly after the scan to ensure that all participants were not exposed to excess radiation. Blood was also taken at 24h in the same room away from the radiology department. At each time point, 4 ml blood was drawn: 2 ml for γ-H2AX foci analysis; 2 ml for 850k array analysis. In the following, we named the baseline as the ‘0h’ for the convenience of narration.
γ-H2AX foci analysis
The protocol of γ-H2AX foci analysis was the same as the ‘Lymphocyte separation and γ-H2AX immunofluorescence analysis’ part of the previous study (Yang et al. Citation2020). The changes of anti-bodies and equipment were: (1) recombinant Anti-gamma H2AX (phosphor S139) antibody (ab81299, Abcam, UK); (2) Goat Anti-Rabbit IgG H&L (ab150077, Abcam, UK); (3) Microscope camera DP74 (Olympus, Japan). Foci calculation were automatically made by ImageJ 1.54b (National Institutes of Health, Rockville, MD); (4). Anticoagulants was changed into EDTA.
Dose calculation
CTDIvol and DLP were automatically exported from the CT system. The absorbed dose was calculated by VirtualDose CT (Virtual Phantoms Inc., Albany, USA), which is a web-based CT organ dose calculator that incorporated 25 ‘virtual patient’ phantoms covering from pediatric patients, pregnant patients, normal size adult patients to overweight adult patients (Ding et al. Citation2015). For the accurate calculation of absorbed dose from each participant, we chose the most suitable virtual phantoms based on individual body size and sex. Scan fields imported to VirtualDose CT were also set manually for each participant.
To further make the dose estimation more accurate and comparable to other studies, the blood absorbed dose of whole body was calculated, too. The method for the calculation of blood absorbed dose of whole body was adapted from Rothkamm et.al (2007). We adjusted it for the VirtualDose CT with the tissue weighting factors provided in the ICRP 103. Organ-specific blood volumes were adapted from ICRP No.89 publication. Sex-specific blood volumes and radiation dose calculations were averaged. To obtain the blood absorbed dose, organ-specific doses were weighted according to the blood content in each organ and then averaged. The estimated blood dose by organ-specific blood volumes is shown in the Supplemental material 1.
Genome-wide DNA methylation analysis
We recruited 20 volunteers for this genome-wide DNA methylation analysis. This analysis was conducted using 850k BeadChip. This methylation profiling microarray covers over 850,000 CpG loci across the whole human genome. All analyses were conducted according to the manufacturer’s instruction. Data quality control was performed by removing (1) CpG probes with a detection p value > .01 in at least one sample; (2) CpG probes with bead count < 3 in all samples; (3) non-CpG probes; (4) CpG probes with SNPs (Zhou et al. Citation2017); (5) CpG probes aligning to multiple locations (Nordlund et al. Citation2013); and (6) CpG probes on the X and Y chromosome. Methylation data in the remaining type-I and type-II CpG probes were corrected and then normalized with ‘preprocessQuantile’ function in minfi.
The M-value was calculated [M = log (methylated signal/unmethylated signal)] to represent methylation level of each CpG loci in each sample and was used in statistical analyses. The Beta-value was calculated [β = methylated signal/(methylated signal + unmethylated signal)] to illustrate the changes of CpG sites. As DNA was extracted mainly from leukocytes, we estimated leukocyte compositions to allow for the adjustment of leukocyte subtypes, including CD8+T lymphocytes, CD4+T lymphocytes, natural killer cells, B cells, monocytes and granulocytes, using a previously-established method for each blood sample (Houseman et al. Citation2012).
Statistical analysis
Considering that there were possible differences between individuals for the repeated measurement of the same CpG site in response to radiation, we established linear mixed effect (LME) models to evaluate the change of methylation level in each CpG site. First, the changes of methylation levels at 0h and 1h, 24h after CT examinations were analyzed. The fixed-effect covariates, which was considered correlation to methylation statue of individual whole blood samples (Bergstedt et al. Citation2022), such as age, sex, body-mass index (BMI), and proportion of leukocyte compositions (with the exception of CD8+T cells, since this parameter would have led to a lot of model-fit errors) were included. Then, we replaced time-point variables with individual radiation dose represented by parameters of CTDIvol, DLP, and blood absorbed dose, respectively. The γ-H2AX foci of participants without missing value were finally replaced by the dose represented by different parameters. To address the possible batch effect in microarray analysis, we first conducted a principal component regression analysis to identify potential batch effect from plates. If the results show any significant batch effects, we will include a categorical variable in the LME model. The leukocyte components in the three groups were analyzed by Friedman test.
The ‘minfi’ (Fortin et al. Citation2017) and ‘ENmix’ (Xu et al. Citation2016) packages in bioconductor were used for pre-processing and quality control of the genome-wide methylation data. All probes were annotated in the ‘IlluminaHumanMethylationEPICanno.ilm10b4.hg19’ package, which used the UCSC gene database to link CpG loci to the corresponding genes nearby. The LME model was fitted using the ‘lme4’ (Bates et al. Citation2015) package in R (version 4.2.2; R Foundation for Statistical Computing, Vienna, Austria). To correct for the type-I error in multiple tests, we calculated the false discovery rate (FDR) with the use of unadjusted p values using the ‘qvalue’ (Storey et al. Citation2022) package. FDR < 0.05 was considered ‘significant’, FDR < 0.1 was considered to be ‘marginally significant’. To correct the bias caused by differing numbers of probes per gene in array, ‘missMethyl’ (Phipson et al. Citation2016) package was used to perform the functional enrichment analysis. Cutoff p value for functional analysis is .001.
The paired samples Wilcoxon test was used to analyze the difference in the number of γ-H2AX foci per cell in pairwise time points comparison in R. A linear regression analysis of foci and doses represented by different parameters was performed in Excel (Microsoft, USA)
Results
Descriptive statistics
In the present study, a total of 20 healthy participants were recruited from January 2020 to November 2022. Among the 20 subjects, five were males and 15 were females. Sample sizes and gender ratios were limited by ethical, financial and recruitment constraints. We adopted relatively strict inclusion and exclusion criteria compared with other studies. This undoubtedly narrowed the number of people who could be recruited while ensuring the results relevant. The recruitment did not proceed smoothly, and only 20 agreed to have blood taken at multiple time points. Based on the above and the conclusion from the previous studies that the difference between genders lies in the level of damage rather than in the trend (Sharma et al. Citation2015; Applegate et al. Citation2020), we did not exclude either sex from the current study. Gender differences were not the main target of analysis in this study but were added as one of the fixed factors during the analysis of methylation microarrays.
The average age was 26.2 (SD = 1.9) years and the average BMI was 21.8 (SD = 2.6) kg/m2. No significant correlation was found between γ-H2AX and individual information, like age, sex or BMI. Doses represented by different parameters were shown in .
Table 1. The minimum, maximum and average CTDLvol, DLP and blood absorbed doses.
γ-H2AX foci analysis
The number of γ-H2AX foci per cell was detected and analyzed in 17 of 20 recruited participants. Data of participant No. 8 were excluded for the cell counts insufficient to analysis. In pairwise comparisons and three-group comparison, participants with missing value were excluded. Thus, a total of 13 participants’ data were included for γ-H2AX foci analysis. The foci of individuals were illustrated in . The mean number of γ-H2AX foci was 0.24 ± 0.052 (SE), 0.55 ± 0.07, and 0.34 ± 0.05 foci/cell for three time points (0h, 1h, 24h), respectively.
Figure 2. Number of γ-H2AX foci per cell at different time points. (a) and (b) are Plots illustrating the number of foci per cell at three time points; (c) bar plot showing number of foci per cell at 1h and 24h minus number of foci per cell at 0h (participants with missing value were excluded in (a) and (C)).
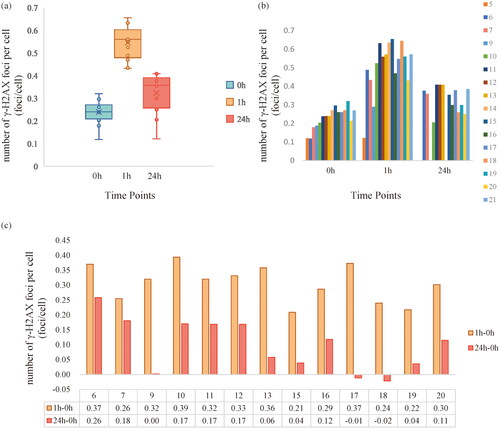
The number of γ-H2Ax foci per cell in the 13 participants were significantly different among the three sample groups (p < .001). Further analyses indicated that in pairwise comparation, the number of γ-H2AX foci per cell was significantly increased at 1h (p < .001) and followed with significant decrease at 24h (P < .001). However, the number of γ-H2AX foci per cell at 24h was still higher than that before the CT examinations (p < .001). illustrates the number of γ-H2AX foci per cell differences between 1h\24h and 0h among the 13 participants.
Figure 3. Linear relationship between the increased number of γ-H2AX foci per cell and doses represented by (3a) CT dose index Volume (CTDIvol), (3d) dose length-product (DLP) and (3c) blood absorbed dose at 1h. Each blue dot represents a participant’s dose-focus counterpart, and the dotted lines are linear tend lines. The dotted line represents linear trends. R2 is regression coefficient, y is linear regression expression.
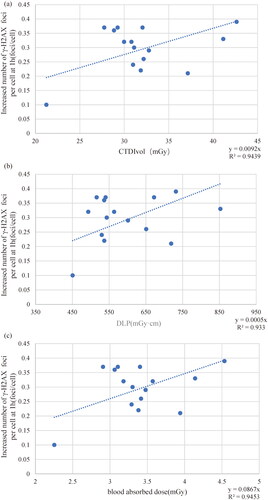
The results for genome-wide DNA methylation analyses
After filtering out probes that did not meet the quality control criteria, a total of 745144 probes were finally included in the LME models. No batch effects were found in these arrays. The principal component regression analyses were shown in the Supplemental material 2. Comparisons of different leukocyte cells at three time points was shown in and the box plot of different leukocyte cells was shown in . The proportion of CD8+ T cell, neutrophile granulocyte, NK cells (p < .01) were significantly changed. As participant No.9 was missed in the 24h blood collection, the data were excluded in leukocyte composition analysis.
Figure 4. Box plot of different leukocyte cells. Abbreviations: CD8 + T: CD8+ T lymphocytes; CD4 + T: CD4 + T lymphocytes; NK: natural killer cells.
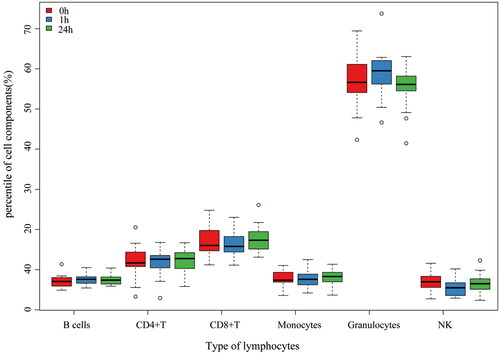
Table 2. Comparisons of different leukocyte cells at three time points.
The number of ‘significant’ and ‘marginally significant’ DMPs discovered by different parameters in LME model were shown in . However, the results were diverse by replacing doses represented by different parameters. Only one CpG site ‘cg14283649’ was significant both in the 0h-1h and 0h-24 comparison groups and by using most of parameters in the LME model. shows the changes of beta-value of cg14283649.This site located on chromosome 1 and at the body of PAX5 gene. No significant (FDR < 0.05) pathway was found in this study. More detailed information of DMPs could be found in Supplemental material 3.
Table 3. FDR and the number of differentially methylated Positions with doses represented by different parameters.
Discussion
There have been concerns about the cancer risk from CT scanning, but we have not reached a consensus on this issue so far. The findings of epidemiological surveys of cancer risk induced by LDIR from CT examination in adults are conflicting (Berrington de González and Darby Citation2004; Pearce et al. Citation2012; Memon et al. Citation2019; Auvinen et al. Citation2022). Epidemiological studies are often retrospective and do not explain what differences exist between those who developed the disease and those who did not. Accordingly, some researchers have suggested combining epidemiological and radiobiological methods to study the health effects of LDIR (NCRP 2020). Patients undergoing single CT examination have more accurate radiation dose and less interference, which may be an ideal window for the investigation of acute LDIR effect on healthy human body.
The basal level of γ-H2AX foci is not entirely consistent across studies, ranging from 0.17 to 0.94 foci per cell (Fukumoto et al. Citation2017; Tao et al. Citation2019; Sakane et al. Citation2020; Yang et al. Citation2020). Our results are within this range (0.24 foci per cell), with slightly higher than the results of our previous study (Yang et al. Citation2020). This could be caused by us changing to a higher resolution camera or changing antibodies. Additionally, our results showed that γ-H2AX increased significantly at 1h after CT with a linear relationship with three dose parameters. Beels et al. established a dose-response curve in 0–10 mGy(slope: 0.1 foci/mGy) for whole blood samples taken 15 min after X-ray irradiation (Beels et al. Citation2010). The slope of the dose response curve (slope: 0.087 foci/mGy) fitted by blood absorbed dose in is slightly lower than the slope of the Beels’s curve. Considering that our results were obtained at 1h, this difference is acceptable. This indicates that our calculated blood absorbed dose is relatively accurate. Similar result was obtained by Jakl et al. at 2h after 100mGy 60Co γ-rays with a slope of 0.0892 foci/mGy (Jakl et al. Citation2020). This suggests that γ-H2AX foci remain a useful biological dosimetry method at 1 h after CT.
In the present study, the in vivo γ-H2AX foci was tested at 24h and it was found to remain significantly different from 0h. This result is contrary to the results by Lobrich et al. (Lobrich et al. Citation2005). γ-H2AX foci that is residual at 24h, the so-called residual foci, have been reported in several in-vitro studies. γ-H2AX foci was detected for a delayed repair at low dose (1.2mGy), but no similar effect was observed at a relative high dose, which may indicate that LDIR induced more residual foci than higher dose (Rothkamm and Löbrich Citation2003). Similar results were found in another in vitro study, in which the repair of foci of γ-H2AX and 53BP1 after CT examinations (20mGy) was weakened at 24h and did not decrease to a statistically insignificant level until 48 h later (Bogdanova et al. Citation2021). The results of all these studies showed that residual foci caused by low-dose ionizing radiation accounted for a great proportion of excess foci than those caused by high-dose ionizing radiation. In the present study, residual foci at 24h accounted for about 32% of the excess foci at 1h. This result is slightly lower than that of Beels et al. (Beels et al. Citation2010) who performed residual foci at 24h as a percentage of approximately 43% of the 1h excess foci under 5mGy conditions on whole blood in an in vitro conditin. However, considering that only a portion of the blood was directly irradiated in the head and neck CT examination and that circulation is more likely to dilute these cells, 32% is still higher than expected. We believe that the reasons for this higher-than-expected number of residual foci are speculated to be the following: First mechanism might be that systemic blood circulation carries oxidative stress products or related signals throughout the body, which in turn causes bystander effects, resulting in more cells producing DSBs or an increase in the focus of γ-H2AX (Tomita and Maeda Citation2015). Tomita and Maeda also conclude that there is an association between p53 status and the bystander effect. The inclusion of 53BP1, p53 binding protein, is essential in future studies. Secondly, our experiments involved a population which may be relatively sensitive to radiation. Sharm et al. reported higher residual levels in young people (21-30) than in elder people (31–50) (Sharma et al. Citation2015). However, this point is only speculative on our part and Lobrich et al. did not report the average age of their participants (Lobrich et al. Citation2005). Both of these inferences need to be confirmed by further experiments. It should be noted that in , both participants 17 and 18 had lower focal levels at 24h than at 0h, which is consistent with the results of Lobrich et al. for the majority of their participants (Lobrich et al. Citation2005). Similarly, Lorbrich et al. had two participants with higher foci than basal levels at 24h.This suggests that there are some unknown inter-individual differences that influence the level of residual foci.
Some of studies have concluded that residual foci represent a failure of DSB repair (Beels et al. Citation2010; Sharma et al. Citation2015). However, this may not be the case, and in other studies, it was suggested that the presence of a residual foci represents one of two possible scenarios: DSBs do fail to repair, or γ-H2AX focal dephosphorylation is not completed after DSB reparation is completed (Grudzenski et al. Citation2010; Pustovalova et al. Citation2017; Bogdanova et al. Citation2021). Jakl et al. used pulse field gel electrophoresis (PFGE) to compare the repair kinetics of γ-H2AX and DSB under 3 Gy irradiation. Their results showed that at 24 h, PFGE did not detect DSB, while γ-H2AX still remained at 48.31%. Despite the lack of intuitive physical quantification methods to measure DSB under low-dose conditions, this result is also sufficient to show that γ-H2AX foci cannot be used as sufficient evidence for the presence of DSB at 24h. Therefore, with all of our participants in a healthy state, even if we found in-vivo the presence of residual foci at 24h and a significant difference in focus between 0h and 24h, we cannot simply assume that the DSB repair function itself is abnormal. The significance of residual foci still needs to be further investigated.
In contrast to SSBs, which can be repaired on the basis of undamaged strands, DSB repair relies on two pathways homology-directed recombination (HDR) and nonhomologous end joining (NHEJ). The former is often considered high-fidelity because of the sister chromatid being a template, while the latter is considered error-prone. It should be noted that DSB induced by LDIR from CT examination could be a factor contributing to genomic instability (United Nations Scientific Committee on the Effects of Atomic Radiation Citation2022b). However, in healthy people, in other words, people with normal DNA repair function, DSBs would be dealt with in such a way that the entire body comes to a good end. It has been shown that LDIR-induced residual γH2AX foci in bone marrow mesenchymal stem cells do not result in accelerated senescence of irradiated cell progeny (Pustovalova et al. Citation2017). This result implied that the residual foci are not necessarily a negative effect on daughter cells.
It should be noted that the number of residual foci in the present study depends only on fluorescence focus analysis, not intuitive physical quantification. More evidence would be needed.
The influence of environment to DNA methylation is increasingly recognized as a key process to cause cancer (Law and Holland Citation2019; Monk et al. Citation2019). For the convenience of sampling, peripheral blood is often sampled to study cancer risk or cancer prediction (Kresovich et al. Citation2022). Although methylation is currently the most study area in the field of epigenetics, there are still no unified conclusions about the effects of ionizing radiation on DNA methylation (United Nations Scientific Committee on the Effects of Atomic Radiation Citation2022b). Specific to the field of LDIR from CT examination, there are almost no reference, and the experimental content of human Epigenome-wide association study (EWAS) is rare. Only one study was found to investigate the DNA methylation after CT examination (Becker et al. Citation2022) in-vitro, and it was reported that no changes of DNA-methylation profiles in PBL induced by radiation. In that study, PBL were collected from three health male donors who were with age 30, 32 and 53 years, and then irradiated with CT scan in-vitro. After the PBL was incubated for 6 h at 37 °C, DNA was extracted for analysis. In the present study, we recruited 20 participants, and the blood was collected in vivo at each time point. We believe that our results are more consistent with real changes in DNA methylation of peripheral blood cells after CT examination. Up to our knowledge, this is the first in vivo study discovering DMPs in human peripheral blood after CT examinations with 850k microarray.
We found that CD8+T cells, neutrophils and NK cells in leukocyte components of the three groups had statistical differences. These types of lymphocytes were closely related to inflammation and anti-tumor process. As illustrated in , CD8+T cells and NK cells decreased slightly, while neutrophils increased slightly at 1h compared with 24h before and after the irradiation. Therefore, CT irradiation may cause some changes in immune cells in a short term with the number of anti-tumor cells decreased while the number of inflammatory neutrophils increased. This suggests that CT examination has short-term effect on the immune system. This is consistent with the conclusion of a recent exhaustive review of the effects of LDIR on the immune system (Lumniczky et al. Citation2021).
DNA methylation changes induced by radiation may occur very rapidly. Numbers of DMPs in different groups were shown in . Although we have known that radiation could influence DNA methylation, we have no idea about when it is changed. In the present study, several DMPs were detectable with 850k BeadChip as early as 1h after CT examination. This suggests that DNA methylation changes start at least 1h after CT examination in vivo.
The greatest number of DMPs were found by using parameter ‘Foci per cell’. It may suggest that the DNA methylation may highly correlated with the number of DSBs. A researcher has found that DSBs can cause methylation changes in their vicinity (O'Hagan et al. Citation2008). In 1h–24h comparison with two parameters of ‘Time points’ and ‘Foci per cell’, the number of DMPs are considerable. These results suggest that the variation direction of DNA methylation in PBL at 1h and 24h may be diverse. The regulation of DNA methylation after CT examinations may be complex and need a more efficient study design to further investigate it. According to the present results, the methylation statue of part of CpG sites had been changed earliest at 1h, and it did not recover to the level before the CT examinations until 24h later.
Due to the stochastic nature of the location of LDIR-induced DSB damage, the correlation between DSBs and methylation mentioned above poses some difficulties in screening for DNA methylation sites characteristic of LDIR. Therefore, we believe that performing multiple time point assays can overcome this instability to some extent. Despite the poor consistency between groups, the site ‘cg14283649’ still showed a relatively stable significance across multiple groups comparison, and implied that this site should be concerned. This site corresponds to the gene body of ‘PAX5’. Paired box (PAX) gene family encodes nine transcription factors (PAX1-9). Among these members, PAX5 has been extensively studied for its role in the pathogenesis of cancer (Nasri Nasrabadi et al. Citation2022). In a recent study, the critical role of the PAX5 dysfunctional mutation coupled with activation of the Jak3/Stat5 signaling pathway as a basis for the rapid development of radiation-induced precursor B-cell lymphoma in mouse was found (Tachibana et al. Citation2022). It is the basis of rapid development of radiation-induced precursor B-cell acute lymphoblastic leukemia. In addition, the up-regulation of PAX5 methylation has been observed not only in hematopoietic tumors, but also in a variety of other tissue tumors, so PAX5 is considered to be a tumor suppressor gene. The mechanism may be that expression silencing caused by hypermethylation of PAX5 leads to under-activation of trans expression of TP53 (Nasri Nasrabadi et al. Citation2022). However, the location of site ‘cg14283649’ is at the gene body, and a review showed that the methylation site in the gene may help suppress cancerogenic process (Wang et al. Citation2022). It should also be noted that, as shown in , although there is an upward trend in the methylation changes of PAX5-related sites, the inter-individual variations are very complex, and the associations behind them deserve further investigation.
Recently, PAX5 was found that it can promote PI3K-signaling pathway by down-regulating the phosphatase and tensing homologue (PTEN) (Calderón et al. Citation2021). While PI3K-Akt signaling pathway is one of the key pathways related to radiation-induced DSBs repair, it inhibits the PI3K/Akt/mTOR pathway in radioresistant prostate cancer cells enhances radiosensitivity (Chang et al. Citation2014). The expression of BAX gene, which down-regulated by PTEN, was to be up-regulated in irradiated peripheral blood, after cultured at 37 °C for 6h (Becker et al. Citation2022). All the results from the above studies may suggest that PAX5 has the potential to be a marker of radiation damage in PBL.
The limitations of our study are as follows: (1) Due to the tissue specificity of methylation, the methylation of tissues in different parts of the body is not consistent, and the methylation degree of peripheral blood cannot fully reflect the methylation changes of other radiation-sensitive organs; (2) Although the extraction of whole peripheral blood for methylation analysis is simple and has more extensive application value, the results are not accurate enough. There are various cellular and non-cellular DNA components in whole blood. Therefore, in future experiments, more narrow cell lineages should be extracted to make the target cells more homogeneous. (3) The comparison of 0h and 24h may not be reliable. Several experiments have demonstrated that some environmental exposures, such as air pollution, can also cause methylation changes over a short period of time. Therefore, the interaction factors between participants and the environment should be designed more rigorously. (4) A larger and sex-balanced cohort study is needed to confirm our results. However, considering most of the current knowledge about LDIR comes from animal and in vitro experiments, our results are valuable and instructive. (5) In the present study, neither the γ-H2AX foci nor the DNA methylation changes recovered to the 0h level at 24h. The outcome of these CT examination-induced changes still needs to be observed for a longer period of time. However, longer-term in-vivo investigation is limited by ethical requirements and by the participants’ own wishes—most participants in this trial declined verbal inquiries from the researchers to participate in a longer-term follow-up. (6) In practice, the CT examination may take pressure to patients. Unternaehrer et al. reported that acute psychosocial stress does induce changes on DNA methylation (Unternaehrer et al. Citation2012). However, we think that this factor had little effect on the results of the present study, as the participants in the present study were well informed on the study process and purpose, they may not be as psychologically stressed as the subjects in the aforementioned experiment.
Conclusion
A delayed disappear of γ-H2AX foci in vivo at 24h after CT examination was observed in the present study. CT examination can cause detectable changes of DNA methylation as early as at 1h later in whole blood sample. By using different parameters, PAX5-related methylation sites showed a relatively stable and persistent increase in beta value. However, the complex mechanism and long-term effects of low-dose ionizing radiation on human body have not been explained effectively, and further research on this issue is still needed.
Ethical approval
All procedures performed in studies involving human participants were in accordance with the ethical standards of the institutional and/or national research committee and with the 1964 Declaration of Helsinki and its later amendments or comparable ethical standards. This study has been registered at Chinese Clinical Trail Registry (ChiCTR2200063358,05/09/2022) and was approved by the Institutional Review Board of Peking University School and Hospital of Stomatology (PKUSSIRB-201944052)
Informed consent
Informed consent was obtained from all individual participants included in this study. They received oral and written information about the design of the study and their right to withdraw at any time without negative effect.
Data and/or code availability
The datasets used and/or analyzed during the current study are available from the corresponding author on reasonable request.
Geolocation information
Beijing, China.
Supplemental Material
Download Zip (336.1 KB)Disclosure statement
No potential conflict of interest was reported by the author(s).
Additional information
Funding
Notes on contributors
Shuo Wang
Shuo Wang, PhD, is a Researcher of Oral and Maxillofacial Radiology and Radiobiology at Peking University School and Hospital of Stomatology, Beijing, China.
Gang Li
Gang Li, PhD, is a Professor of Oral and Maxillofacial Radiology at Peking University School and Hospital of Stomatology, Beijing, China.
Han Du
Han Du, PhD, is a Researcher of Oral and Maxillofacial Radiology at Peking University School and Hospital of Stomatology, Beijing, China.
Jiling Feng
Jiling Feng, PhD, is a Researcher of Oral and Maxillofacial Radiology at Peking University School and Hospital of Stomatology, Beijing, China.
References
- Antwih DA, Gabbara KM, Lancaster WD, Ruden DM, Zielske SP. 2013. Radiation-induced epigenetic DNA methylation modification of radiation-response pathways. Epigenetics. 8(8):839–848. doi:10.4161/epi.25498
- Applegate KE, Rühm W, Wojcik A, Bourguignon M, Brenner A, Hamasaki K, Imai T, Imaizumi M, Imaoka T, Kakinuma S, et al. 2020. Individual response of humans to ionising radiation: governing factors and importance for radiological protection. Radiat Environ Biophys. 59(2):185–209. doi:10.1007/s00411-020-00837-y
- Auvinen A, Cardis E, Blettner M, Moissonnier M, Sadetzki S, Giles G, Johansen C, Swerdlow A, Cook A, Fleming S, et al. 2022. Diagnostic radiological examinations and risk of intracranial tumours in adults—findings from the interphone study. Int J Epidemiol. 51(2):537–546. doi:10.1093/ije/dyab140
- Banáth JP, Klokov D, MacPhail SH, Banuelos CA, Olive PL. 2010. Residual γH2AX foci as an indication of lethal DNA lesions. BMC Cancer. 10(1):4. doi:10.1186/1471-2407-10-4
- Bates D, Mächler M, Bolker B, Walker S. 2015. Fitting linear mixed-effects models using lme4. J Stat Softw. 67(1):1–48. doi:10.18637/jss.v067.i01
- Becker BV, Kaatsch HL, Nestler K, Jakobi J, Schäfer B, Hantke T, Brockmann MA, Waldeck S, Port M, Ullmann R. 2022. Impact of medical imaging on the epigenome – low-dose exposure in the course of computed tomography does not induce detectable changes of DNA-methylation profiles in peripheral blood cells. Int J Radiat Biol. 98(5):980–985. doi:10.1080/09553002.2021.2004329
- Beels L, Werbrouck J, Thierens H. 2010. Dose response and repair kinetics of gamma-H2AX foci induced by in vitro irradiation of whole blood and T-lymphocytes with X- and gamma-radiation. Int J Radiat Biol. 86(9):760–768. doi:10.3109/09553002.2010.484479
- Bergstedt J, Azzou SAK, Tsuo K, Jaquaniello A, Urrutia A, Rotival M, Lin DTS, MacIsaac JL, Kobor MS, Albert ML, et al. 2022. The immune factors driving DNA methylation variation in human blood. Nat Commun. 13(1):5895. doi:10.1038/s41467-022-33511-6
- Berrington de González A, Darby S. 2004. Risk of cancer from diagnostic X-rays: estimates for the UK and 14 other countries. Lancet. 363(9406):345–351. doi:10.1016/s0140-6736(04)15433-0
- Bogdanova NV, Jguburia N, Ramachandran D, Nischik N, Stemwedel K, Stamm G, Werncke T, Wacker F, Dörk T, Christiansen H. 2021. Persistent DNA double-strand breaks after repeated diagnostic CT scans in breast epithelial cells and lymphocytes. Front Oncol. 11:634389. doi:10.3389/fonc.2021.634389
- Calderón L, Schindler K, Malin SG, Schebesta A, Sun Q, Schwickert T, Alberti C, Fischer M, Jaritz M, Tagoh H, et al. 2021. Pax5 regulates B cell immunity by promoting PI3K signaling via PTEN down-regulation. Sci Immunol. 6(61):eabg5003. doi:10.1126/sciimmunol.abg5003
- Chang L, Graham PH, Hao J, Ni J, Bucci J, Cozzi PJ, Kearsley JH, Li Y. 2014. PI3K/Akt/mTOR pathway inhibitors enhance radiosensitivity in radioresistant prostate cancer cells through inducing apoptosis, reducing autophagy, suppressing NHEJ and HR repair pathways. Cell Death Dis. 5(10):e1437–e1437. doi:10.1038/cddis.2014.415
- Ding A, Gao Y, Liu H, Caracappa PF, Long DJ, Bolch WE, Liu B, Xu XG. 2015. VirtualDose: a software for reporting organ doses from CT for adult and pediatric patients. Phys Med Biol. 60(14):5601–5625. doi:10.1088/0031-9155/60/14/5601
- Flavahan WA, Gaskell E, Bernstein BE. 2017. Epigenetic plasticity and the hallmarks of cancer. Science. 357(6348):eaal2380. doi:10.1126/science.aal2380
- Fortin J-P, Triche TJ, Jr, Hansen KD. 2017. Preprocessing, normalization and integration of the Illumina HumanMethylationEPIC array with minfi. Bioinformatics. 33(4):558–560. doi:10.1093/bioinformatics/btw691
- Fukumoto W, Ishida M, Sakai C, Tashiro S, Ishida T, Nakano Y, Tatsugami F, Awai K. 2017. DNA damage in lymphocytes induced by cardiac CT and comparison with physical exposure parameters. Eur Radiol. 27(4):1660–1666. doi:10.1007/s00330-016-4519-8
- Grudzenski S, Raths A, Conrad S, Rübe CE, Löbrich M. 2010. Inducible response required for repair of low-dose radiation damage in human fibroblasts. Proc Natl Acad Sci USA. 107(32):14205–14210. doi:10.1073/pnas.1002213107
- Hall EJ, Giaccia AJ. 2018. Radiobiology for the radiologist. 8th ed. Philadelphia (USA): Wolters Kluwer. https://www.wolterskluwer.com/en/solutions/ovid/radiobiology-for-the-radiologist-500.
- Houseman EA, Accomando WP, Koestler DC, Christensen BC, Marsit CJ, Nelson HH, Wiencke JK, Kelsey KT. 2012. DNA methylation arrays as surrogate measures of cell mixture distribution. BMC Bioinformatics [Internet]. 13(1). doi:10.1186/1471-2105-13-86
- ICRP. 2005. Low-dose extrapolation of radiation-related cancer risk. Ann ICRP. 35(4):1–140.
- Jakl L, Marková E, Koláriková L, Belyaev I. 2020. Biodosimetry of low dose ionizing radiation using DNA repair foci in human lymphocytes. Genes (Basel). 11(1):58. doi:10.3390/genes11010058
- Kresovich JK, Xu Z, O'Brien KM, Shi M, Weinberg CR, Sandler DP, Taylor JA. 2022. Blood DNA methylation profiles improve breast cancer prediction. Mol Oncol. 16(1):42–53. doi:10.1002/1878-0261.13087
- Kuefner MA, Grudzenski S, Schwab SA, Wiederseiner M, Heckmann M, Bautz W, Lobrich M, Uder M. 2009. DNA double-strand breaks and their repair in blood lymphocytes of patients undergoing angiographic procedures. Invest Radiol. 44(8):440. doi:10.1097/RLI.0b013e3181a654a5
- Law P-P, Holland ML. 2019. DNA methylation at the crossroads of gene and environment interactions. Essays Biochem. 63(6):717–726. doi:10.1042/EBC20190031
- Lee Y, Kim YJ, Choi YJ, Lee JW, Lee S, Cho YH, Chung HW. 2015. Radiation-induced changes in DNA methylation and their relationship to chromosome aberrations in nuclear power plant workers. Int J Radiat Biol. 91(2):142–149. doi:10.3109/09553002.2015.969847
- Li H, Chen R, Cai J, Cui X, Huang N, Kan H. 2018. Short-term exposure to fine particulate air pollution and genome-wide DNA methylation: a randomized, double-blind, crossover trial. Environ Int. 120:130–136. doi:10.1016/j.envint.2018.07.041
- Lobrich M, Rief N, Kuhne M, Heckmann M, Fleckenstein J, Rube C, Uder M. 2005. In vivo formation and repair of DNA double-strand breaks after computed tomography examinations. Proc Natl Acad Sci USA. 102(25):8984–8989. doi:10.1073/pnas.0501895102
- Lumniczky K, Impens N, Armengol G, Candéias S, Georgakilas AG, Hornhardt S, Martin OA, Rödel F, Schaue D. 2021. Low dose ionizing radiation effects on the immune system. Environ Int. 149:106212. doi:10.1016/j.envint.2020.106212
- Mah LJ, El-Osta A, Karagiannis TC. 2010. γ-H2AX: a sensitive molecular marker of DNA damage and repair. Leukemia. 24(4):679–686. doi:10.1038/leu.2010.6
- Memon A, Rogers I, Paudyal P, Sundin J. 2019. Dental X-rays and the risk of thyroid cancer and meningioma: a systematic review and meta-analysis of current epidemiological evidence. Thyroid. 29(11):1572–1593. doi:10.1089/thy.2019.0105
- Monk D, Mackay DJG, Eggermann T, Maher ER, Riccio A. 2019. Genomic imprinting disorders: lessons on how genome, epigenome and environment interact. Nat Rev Genet. 20(4):235–248. doi:10.1038/s41576-018-0092-0
- Nasri Nasrabadi P, Martin D, Gharib E, Robichaud GA. 2022. The pleiotropy of PAX5 gene products and function. Int J Mol Sci. 23(17):10095. doi:10.3390/ijms231710095
- NCRP, editor. 2020. Approaches for integrating radiation biology and epidemiology for enhancing low dose risk assessment. [Internet]. Bethesda: National Council on Radiation Protection and Measurements; [accessed 2022 Oct 17]. https://ncrponline.org/shop/reports/report-no-186-approaches-for-integrating-information-from-radiation-biology-and-epidemiology-to-enhance-low-dose-health-risk-assessment-2020/.
- Nordlund J, Bäcklin CL, Wahlberg P, Busche S, Berglund EC, Eloranta M-L, Flaegstad T, Forestier E, Frost B-M, Harila-Saari A, et al. 2013. Genome-wide signatures of differential DNA methylation in pediatric acute lymphoblastic leukemia. Genome Biol. 14(9):r105. doi:10.1186/gb-2013-14-9-r105
- O'Hagan HM, Mohammad HP, Baylin SB. 2008. Double strand breaks can initiate gene silencing and SIRT1-dependent onset of DNA methylation in an exogenous promoter CpG island. PLOS Genet. 4(8):e1000155. doi:10.1371/journal.pgen.1000155
- Pearce MS, Salotti JA, Little MP, McHugh K, Lee C, Kim KP, Howe NL, Ronckers CM, Rajaraman P, Sir Craft AW, et al. 2012. Radiation exposure from CT scans in childhood and subsequent risk of leukaemia and brain tumours: a retrospective cohort study. Lancet. 380(9840):499–505. doi:10.1016/S0140-6736(12)60815-0
- Phipson B, Maksimovic J, Oshlack A. 2016. missMethyl: an R package for analysing methylation data from Illuminas HumanMethylation450 platform. Bioinformatics. 32(2):286–288. doi:10.1093/bioinformatics/btv560
- Pidsley R, Zotenko E, Peters TJ, Lawrence MG, Risbridger GP, Molloy P, Van Djik S, Muhlhausler B, Stirzaker C, Clark SJ. 2016. Critical evaluation of the Illumina MethylationEPIC BeadChip microarray for whole-genome DNA methylation profiling. Genome Biol. 17(1):208. doi:10.1186/s13059-016-1066-1
- Popp HD, Meyer M, Brendel S, Prinzhorn W, Naumann N, Weiss C, Seifarth W, Schoenberg SO, Hofmann W-K, Henzler T, Fabarius A. 2016. Leukocyte DNA damage after reduced and conventional absorbed radiation doses using 3rd generation dual-source CT technology. Eur J Radiol Open. 3:134–137. doi:10.1016/j.ejro.2016.06.001
- Pustovalova M, Astrelina ТA, Grekhova A, Vorobyeva N, Tsvetkova A, Blokhina T, Nikitina V, Suchkova Y, Usupzhanova D, Brunchukov V, et al. 2017. Residual γH2AX foci induced by low dose x-ray radiation in bone marrow mesenchymal stem cells do not cause accelerated senescence in the progeny of irradiated cells. Aging. 9(11):2397–2410. doi:10.18632/aging.101327
- Rothkamm K, Balroop S, Shekhdar J, Fernie P, Goh V. 2007. Leukocyte DNA damage after multi-detector row CT: a quantitative biomarker of low-level radiation exposure. Radiology. 242(1):244–251. doi:10.1148/radiol.2421060171
- Rothkamm K, Löbrich M. 2003. Evidence for a lack of DNA double-strand break repair in human cells exposed to very low x-ray doses. Proc Natl Acad Sci USA. 100(9):5057–5062. doi:10.1073/pnas.0830918100
- Sakane H, Ishida M, Shi L, Fukumoto W, Sakai C, Miyata Y, Ishida T, Akita T, Okada M, Awai K, et al. 2020. Biological effects of low-dose chest CT on chromosomal DNA. Radiology. 295(2):439–445. doi:10.1148/radiol.2020190389
- Sedelnikova OA, Rogakou EP, Panyutin IG, Bonner WM. 2002. Quantitative detection of 125IdU-induced DNA double-strand breaks with γ-H2AX antibody. Radiat Res. 158(4):486–492. doi:10.1667/0033-7587(2002)158[0486:QDOIID]2.0.CO;22.0.CO;2]
- Sharma PM, Ponnaiya B, Taveras M, Shuryak I, Turner H, Brenner DJ. 2015. High throughput measurement of γH2AX DSB repair kinetics in a healthy human population. PLOS One. 10(3):e0121083. doi:10.1371/journal.pone.0121083
- Storey JD, Bass AJ, Dabney A, Robinson D. 2022. qvalue: Q-value estimation for false discovery rate control [Internet]. http://github.com/jdstorey/qvalue.
- Tachibana H, Daino K, Ishikawa A, Morioka T, Shang Y, Ogawa M, Matsuura A, Shimada Y, Kakinuma S. 2022. Genomic profile of radiation-induced early-onset mouse B-cell lymphoma recapitulates features of Philadelphia chromosome-like acute lymphoblastic leukemia in humans. Carcinogenesis. 43(7):693–703. doi:10.1093/carcin/bgac034
- Tao SM, Zhou F, Schoepf UJ, Johnson AA, Lin ZX, Zhou CS, Lu GM, Zhang LJ. 2019. The effect of abdominal contrast-enhanced CT on DNA double-strand breaks in peripheral blood lymphocytes: an in vitro and in vivo study. Acta Radiol. 60(6):687–693. doi:10.1177/0284185118799513
- Tharmalingam S, Sreetharan S, Kulesza AV, Boreham DR, Tai TC. 2017. Low-dose ionizing radiation exposure, oxidative stress and epigenetic programing of health and disease. Radiat Res. 188(4.2):525–538. doi:10.1667/rr14587.1
- Tomita M, Maeda M. 2015. Mechanisms and biological importance of photon-induced bystander responses: do they have an impact on low-dose radiation responses. J Radiat Res. 56(2):205–219. doi:10.1093/jrr/rru099
- United Nations Environment Programme. 2016. Radiation effects and sources United Nations. [Internet]. https://www.un-ilibrary.org/content/books/9789210599597.
- United Nations Scientific Committee on the Effects of Atomic Radiation. 2022a. Sources, Effects and Risks of Ionizing Radiation, United Nations Scientific Committee on the Effects of Atomic Radiation (UNSCEAR) 2020/2021 Report, Volume I. New York (USA): United Nations. doi:10.18356/9789210010030
- United Nations Scientific Committee on the Effects of Atomic Radiation. 2022b. Sources, Effects and Risks of Ionizing Radiation, United Nations Scientific Committee on the Effects of Atomic Radiation (UNSCEAR) 2020/2021 Report, Volume III. New York (USA): United Nations. doi:10.18356/9789210010054
- Unternaehrer E, Luers P, Mill J, Dempster E, Meyer AH, Staehli S, Lieb R, Hellhammer DH, Meinlschmidt G. 2012. Dynamic changes in DNA methylation of stress-associated genes (OXTR, BDNF ) after acute psychosocial stress. Transl Psychiatry. 2(8):e150–e150. doi:10.1038/tp.2012.77
- Vinnikov V, Belyakov O. 2022. Clinical applications of biological dosimetry in patients exposed to low dose radiation due to radiological, imaging or nuclear medicine procedures. Semin Nucl Med. 52(2):114–139. doi:10.1053/j.semnuclmed.2021.11.008
- Wang Q, Xiong F, Wu G, Liu W, Chen J, Wang B, Chen Y. 2022. Gene body methylation in cancer: molecular mechanisms and clinical applications. Clin Epigenet. 14(1):154. doi:10.1186/s13148-022-01382-9
- Xu Z, Niu L, Li L, Taylor JA. 2016. ENmix: a novel background correction method for illumina humanmethylation450 BeadChip. Nucleic Acids Res. 44(3):e20–e20. doi:10.1093/nar/gkv907
- Yang P, Wang S, Liu D, Zhao H, Li G. 2020. DNA double-strand breaks of human peripheral blood lymphocyte induced by CT examination of oral and maxillofacial region. Clin Oral Investig. 24(12):4617–4624. doi:10.1007/s00784-020-03331-3
- Zhou W, Laird PW, Shen H. 2017. Comprehensive characterization, annotation and innovative use of Infinium DNA methylation BeadChip probes. Nucleic Acids Res. 45(4):e22. doi:10.1093/nar/gkw967