Abstract
The serotonin1A (5-HT1A) receptor is an important member of the superfamily of seven transmembrane domain G-protein-coupled receptors. We have examined the modulatory role of cholesterol on the ligand binding of the bovine hippocampal 5-HT1A receptor by cholesterol complexation in native membranes using digitonin. Complexation of cholesterol from bovine hippocampal membranes using digitonin results in a concentration-dependent reduction in specific binding of the agonist 8-OH-DPAT and antagonist p-MPPF to 5-HT1A receptors. The corresponding changes in membrane order were monitored by analysis of fluorescence polarization data of the membrane depth-specific probes, DPH and TMA-DPH. Taken together, our results point out the important role of membrane cholesterol in maintaining the function of the 5-HT1A receptor. An important aspect of these results is that non-availability of free cholesterol in the membrane due to complexation with digitonin rather than physical depletion is sufficient to significantly reduce the 5-HT1A receptor function. These results provide a comprehensive understanding of the effects of the sterol-complexing agent digitonin in particular, and the role of membrane cholesterol in general, on the 5-HT1A receptor function.
Abbreviations | ||
BCA | = | bicinchoninic acid |
DMPC | = | dimyristoyl-sn-glycero-3-phosphocholine |
DPH | = | 1,6-diphenyl-1,3,5-hexatriene |
5-HT | = | 5-hydroxytryptamine |
8-OH-DPAT | = | 8-hydroxy-2(di-N-propylamino)tetralin |
p-MPPF | = | 4-(2′-methoxy)-phenyl-1-[2′-(N-2″-pyridinyl)-p-fluorobenzamido]ethyl-piperazine |
p-MPPI | = | 4-(2′-methoxy)-phenyl-1-[2′-(N-2″-pyridinyl)-p-iodobenzamido]ethyl-piperazine |
PMSF | = | phenylmethylsulfonyl fluoride |
TMA-DPH | = | 1-[4-(trimethylammonio)phenyl]-6-phenyl-1,3,5-hexatriene |
TLC | = | thin layer chromatography |
Tris | = | tris-(hydroxymethyl)aminomethane |
Introduction
G-protein-coupled receptors constitute a superfamily of transmembrane proteins whose function is to transmit information across the cell membrane from the extracellular environment to the interior of the cells, thus providing a mechanism of communication between the exterior and the interior of the cell Citation[1,2]. They represent major targets for the development of novel drug candidates in all clinical areas Citation[3,4]. It is estimated that 50% of clinically prescribed drugs act as either agonists or antagonists of G-protein-coupled receptors which points to their immense therapeutic potential Citation[5]. The membrane organization of G-protein-coupled receptors assumes significance in the light of their role in health and disease.
Interestingly, the efficiency of signal transduction processes carried out by G-protein-coupled receptors appears to be influenced by the local composition and organization of lipids within the plasma membrane Citation[6]. It has been proposed that the G-protein-coupled receptors are not uniformly present on the plasma membrane but are concentrated in specific membrane microdomains Citation[7,8]. It has been shown that some of these domains are enriched in cholesterol Citation[6]. For example, it has recently been reported that serotonin2A receptors are localized in cholesterol-enriched membrane microdomains (caveolae) and serotonergic signaling induced by serotonin2A receptors depends on the membrane cholesterol content Citation[9] and on caveolin-1, a scaffolding protein found in caveolae Citation[10]. Localization of G-protein-coupled receptors into domains has given rise to new challenges and complexities in receptor signaling since signaling has to be understood in context of the three dimensional organization of various signal transduction components which include receptors and G-proteins Citation[7].
Cholesterol is an essential component of eukaryotic membranes and plays a crucial role in membrane organization, dynamics, function, and sorting Citation[11,12]. It is often found distributed non-randomly in domains or pools in biological and model membranes Citation[13–15]. Many of these domains are believed to be important for the maintenance of membrane structure and function. Cholesterol in the plasma membrane plays a key role in regulating the activity of membrane proteins including receptors [reviewed in ref. Citation[16]]. The interaction between cholesterol and other membrane components (such as receptors) in the brain therefore assumes relevance for a comprehensive understanding of brain function.
Serotonin receptors represent one of the largest, evolutionarily ancient, and highly conserved families of G-protein-coupled receptors Citation[17]. Serotonin exerts its diverse actions by binding to distinct cell surface receptors which have been classified into many groups Citation[18]. Serotonin receptors are members of a superfamily of seven transmembrane domain receptors Citation[1,2] that couple to and transduce signals via GTP-binding regulatory proteins (G-proteins) Citation[19]. Among the 14 subtypes of serotonin receptors, the G-protein coupled 5-HT1A receptor is the best characterized for a variety of reasons Citation[20]. We have earlier partially purified and solubilized 5-HT1A receptors from bovine hippocampus in a functionally active form Citation[21,22].
We have recently shown the requirement of membrane cholesterol in modulating ligand binding activity of the 5-HT1A receptor from the bovine hippocampus Citation[23]. This was achieved by the use of methyl-β-cyclodextrin which physically depletes cholesterol from membranes. Treatment of bovine hippocampal membranes with methyl-β-cyclodextrin therefore resulted in specific removal of membrane cholesterol without any change in phospholipid content. Removal of cholesterol from bovine hippocampal membranes in this manner resulted in a reduction in ligand binding and G-protein coupling to the 5-HT1A receptor Citation[23]. If cholesterol is necessary for ligand binding of the 5-HT1A receptor, modulating cholesterol availability by other means could lead to similar effects on the 5-HT1A receptor function. In this paper, we have tested this proposal by treating the membranes with the sterol-complexing agent digitonin which does not physically deplete membrane cholesterol, yet would effectively reduce cholesterol-receptor interactions in the membrane due to complexation Citation[24,25]. Digitonin is a plant glycoalkaloid saponin detergent (see ) obtained from Digitalis purpurea. It forms water-insoluble 1:1 complexes (termed ‘digitonides’) with cholesterol and other steroids, which possess a planar sterol nucleus, a 3β-hydroxy-Δ5 configuration and a hydrophobic side chain at C17Citation[26]. Digitonin treatment has been shown to result in the formation of cholesterol-digitonin rich domains in the membrane Citation[27], thus reducing the freely available cholesterol capable of interacting with other membrane constituents such as receptors. This property of digitonin has resulted in its use as an agent to distinguish between cholesterol-rich and -poor membranes in the ultrastructure analysis of cell membranes Citation[28].
In this paper, we have monitored the modulatory role of cholesterol on the function (specific agonist and antagonist binding) of the bovine hippocampal 5-HT1A receptor by cholesterol complexation in native membranes using digitonin. The corresponding changes in membrane dynamics were monitored by analysis of fluorescence polarization data of the membrane depth-specific probes, DPH and TMA-DPH.
Materials and methods
Materials
BCA, DMPC, DPH, EDTA, EGTA, MgCl2, MnCl2, Na2HPO4, p-MPPI, PMSF, TMA-DPH, Tris, iodoacetamide, polyethylenimine, serotonin, sodium azide, and sucrose were obtained from Sigma Chemical Co. (St. Louis, MO). Digitonin was from Research Organics (Cleveland, OH). Amplex Red cholesterol assay kit was from Molecular Probes (Eugene, OR). [3H]8-OH-DPAT (specific activity = 135.0 Ci/mmol) and [3H]p-MPPF (specific activity = 70.5 Ci/mmol) were purchased from DuPont New England Nuclear (Boston, MA). BCA reagent kit for protein estimation was obtained from Pierce (Rockford, IL). All other chemicals used were of the highest available quality. GF/B glass microfiber filters were from Whatman International (Kent, UK). Pre-coated silica gel 60 thin layer chromatography plates were from Merck (Merck KGaA, Germany). All solvents used were of analytical grade. Water was purified through a Millipore (Bedford, MA) Milli-Q system and used throughout. Fresh bovine brains were obtained from a local slaughterhouse within 10 min of death and the hippocampal region was carefully dissected out. The hippocampi were immediately flash frozen in liquid nitrogen and stored at −70°C till further use.
Preparation of native hippocampal membranes
Native hippocampal membranes were prepared as described earlier Citation[23]. Protein concentration was determined using the BCA reagent with bovine serum albumin as a standard Citation[29].
Preparation of digitonin stock solutions and treatment of hippocampal membranes
Stock solutions of digitonin in water were prepared as described previously Citation[30]. Briefly, digitonin was dissolved in ethanol by mild shaking in a water bath at 37°C and the solution was evaporated to dryness under a gentle stream of N2. This procedure results in the deposition of a fine film of digitonin on the walls of the glass tube that dissolves instantly upon addition of water. Aliquots of this aqueous stock solution were immediately added to hippocampal membranes at a total protein concentration of 0.5 mg/ml in 50 mM Tris, pH 7.4 buffer and incubated for 1 h at 25°C with constant shaking.
Membrane solubilization mediated by digitonin was assayed as described previously Citation[31]. Briefly, membrane samples treated with increasing concentrations of digitonin for 1 h at 25°C were spun down at 100,000×g for 45 min at 4°C. The lipid phosphate and cholesterol content of the membrane pellet dissolved in 50 mM Tris, pH 7.4 buffer was assayed as described below.
Estimation of inorganic phosphate and cholesterol
The concentration of lipid phosphate was determined subsequent to total digestion by perchloric acid Citation[32] using Na2HPO4 as standard. DMPC was used as an internal standard to assess lipid digestion. Samples without perchloric acid digestion produced negligible readings. Membrane cholesterol was estimated using the Amplex Red cholesterol assay kit Citation[33].
Thin layer chromatography of lipids extracted from native membranes
Lipid extraction was carried out according to Bligh and Dyer Citation[34]. The extracts were dried under a stream of nitrogen at 45°C. The dried extracts were resuspended in a mixture of chloroform/methanol (1:1, v/v). Thin layer chromatography of the extracted lipids was carried out using chloroform/methanol/water (65:25:4, v/v/v). The separated lipids were visualized by charring with a solution containing cupric sulfate (10% w/v) and phosphoric acid (8% v/v) at 150°C. The TLC plates were scanned and lipids band intensities were analyzed using the Adobe Photoshop software version 5.0 (Adobe Systems Inc., San Jose, CA).
Radioligand binding assays
Receptor binding assays were carried out as described earlier Citation[35,36] in presence of increasing concentrations of digitonin using 0.5 mg total protein.
Fluorescence polarization measurements
Fluorescence polarization experiments were carried out with membranes containing 50 nmol of total phospholipids suspended in 1.5 ml of 50 mM Tris, pH 7.4 buffer with increasing concentrations of digitonin as described earlier Citation[23,31]. Stock solutions of the fluorescent probes (DPH and TMA-DPH) were prepared in methanol. The amount of probe added was such that the final probe concentration was 1 mol% with respect to the total phospholipid content. This ensures optimal fluorescence intensity with negligible membrane perturbation. Membranes were vortexed for 1 min after addition of the probe and kept in the dark for 1 h before measurements. Background samples were prepared the same way except that the probe was omitted. The final probe concentration was 0.33 µM in all cases and the methanol content was low (0.03% v/v). Control experiments showed that at this concentration of methanol, the ligand binding properties of the receptor are not altered.
Steady state fluorescence was measured in a Hitachi F-4010 spectrofluorometer using 1 cm path length quartz cuvettes at room temperature (23°C). Excitation and emission wavelengths were set at 358 and 430 nm. Excitation and emission slits with nominal bandpasses of 1.5 nm and 20 nm were used. The excitation slit was kept low to avoid any photoisomerization of DPH and TMA-DPH. In addition, fluorescence was measured with a 30 sec interval between successive openings of the excitation shutter (when the sample was in the dark in the fluorimeter) to reverse any photoisomerization of DPH and TMA-DPH Citation[37]. Fluorescence polarization experiments were performed using a Hitachi polarization accessory. Polarization values were calculated from the equation Citation[38]:1 where IVV and IVH are the measured fluorescence intensities (after appropriate background subtraction) with the excitation polarizer vertically oriented and the emission polarizer vertically and horizontally oriented, respectively. G is the instrumental correction factor and is the ratio of the efficiencies of the detection system for vertically and horizontally polarized light and is equal to IHV/IHH. The optical density (measured with a Hitachi U-2000 spectrophotometer using 1 cm path length quartz cuvettes) of the membrane samples in the absence of digitonin measured at 358 nm was ∼0.2 which increased in presence of digitonin. To avoid any scattering artifacts due to the presence of digitonin, the fluorescence polarization values reported in were corrected for scattering by diluting the samples with 50 mM Tris, pH 7.4 buffer as described earlier Citation[39]. Fluorescence polarization values of samples (control and digitonin-treated, undiluted and diluted with the same buffer) were plotted against their corresponding optical densities as shown in . The intercept on the ordinate (y-axis) from linear fits of this data was considered as the corrected fluorescence polarization value.
Results
Digitonin complexes membrane cholesterol without altering the cholesterol and phospholipid contents of hippocampal membranes
Digitonin is a widely used detergent and has been used to solubilize specific membrane components such as receptors Citation[40]. It is therefore important to choose a range of concentration where digitonin would be present predominantly as a monomer and exert its cholesterol-complexing activity without causing any significant loss of membrane lipids (cholesterol and/or phospholipids). We estimated the loss of membrane lipids in the presence of digitonin by assaying the amount of total cholesterol and phospholipids present in the insoluble pellet obtained by high speed centrifugation following digitonin treatment Citation[31]. The total cholesterol content was analyzed by TLC of lipids extracted from digitonin-treated membranes. shows a thin layer chromatogram of lipids extracted from native membranes, and membranes treated with 0.6 and 1 mM digitonin. Quantitative densitometric analysis of the cholesterol content on the TLC plate () indicates that the treatment with digitonin does not deplete membrane cholesterol. However, analysis of the cholesterol content of digitonin-treated membranes using the cholesterol oxidase enzyme based Amplex Red cholesterol assay kit Citation[33] reveals a reduction in the free (available) cholesterol content (). Thus, treatment of membranes with 1 mM digitonin reduces the available cholesterol content by ∼50%. These results suggest that the presence of digitonin reduces the ability of the enzyme cholesterol oxidase to catalyze the oxidation of cholesterol thereby demonstrating the cholesterol-complexing ability of digitonin. Importantly, shows that in the concentration range of digitonin used (up to 1 mM), there is minimal loss of phospholipid content. All experiments were therefore carried out with this range (up to 1 mM) of digitonin concentration that complexes membrane cholesterol to a significant extent without physically depleting membrane lipids.
Figure 2. Cholesterol-complexing effect of digitonin. Total lipids extracted from digitonin-treated membranes were separated by thin layer chromatography as shown in (a). The lanes represent lipids extracted from native membranes (lane 2), and membranes treated with 0.6 mM (lane 3) and 1 mM (lane 4) digitonin. The arrow represents position of cholesterol on the thin layer chromatogram identified using a standard in lane 1. The total and freely available cholesterol content in digitonin-treated membranes were distinguished as shown in (b). The total cholesterol content (grey bars) in the lipid extract was determined by a densitometric analysis of the thin layer chromatogram. Values are expressed as a percentage of the cholesterol content in native membranes in the absence of digitonin. The amount of freely available cholesterol content (white bars) in membranes treated with digitonin was determined by the Amplex Red cholesterol assay kit. Values are expressed as a percentage of available cholesterol in native membranes in the absence of digitonin. Data represents the mean±SD of two independent experiments. See Materials and methods for further details.
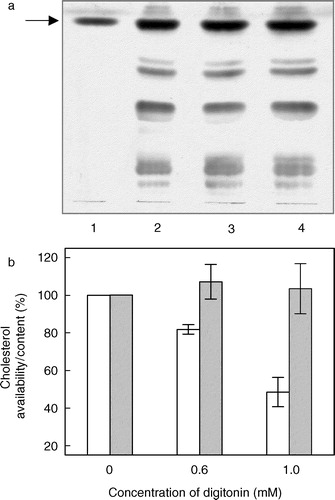
Figure 3. Effect of increasing concentrations of digitonin on the phospholipid content of bovine hippocampal membranes. Phospholipid contents were assayed as described in Materials and methods. Values are expressed as a percentage of the phospholipid content in native membranes in the absence of digitonin. Data represent the means±SE of three independent experiments.
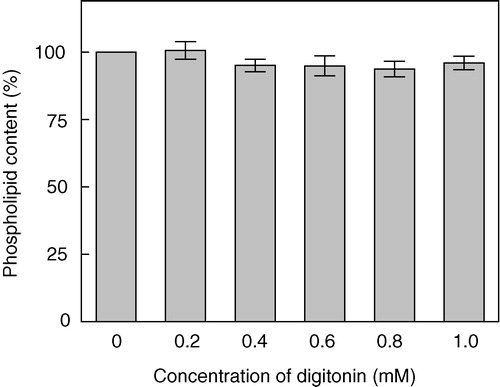
Ligand binding to 5-HT1A receptors is differentially affected in presence of digitonin
shows the reduction in the specific binding of the selective 5-HT1A receptor agonist [3H]8-OH-DPAT with increasing concentrations of digitonin, which results in progressive complexation of membrane cholesterol. This shows that complexation of cholesterol from hippocampal membranes results in loss of specific agonist binding to the 5-HT1A receptor. Thus, the specific agonist binding reduces by 86% in the presence of 1 mM digitonin. To the best of our knowledge, this result represents the first observation of reduction in specific ligand binding to the 5-HT1A receptor due to complexation of membrane cholesterol by digitonin.
Figure 4. Effect of increasing concentrations of digitonin on specific [3H]8-OH-DPAT binding to the 5-HT1A receptor in hippocampal membranes. Values are expressed as a percentage of specific binding for native membranes in the absence of digitonin. Data shown are the means±SE of at least three independent experiments. See Materials and methods for other details.
![Figure 4. Effect of increasing concentrations of digitonin on specific [3H]8-OH-DPAT binding to the 5-HT1A receptor in hippocampal membranes. Values are expressed as a percentage of specific binding for native membranes in the absence of digitonin. Data shown are the means±SE of at least three independent experiments. See Materials and methods for other details.](/cms/asset/615b7520-53f7-4366-b2c1-1d4f72f5cf49/imbc_a_109328_uf0004_b.jpg)
shows the decrease in specific binding of the 5-HT1A selective antagonist [3H]p-MPPF in the presence of increasing concentrations of digitonin. In contrast to the observed reduction in specific agonist binding (), the reduction in specific antagonist binding appears to be more drastic at lower digitonin concentrations used. For example, the reduction in specific antagonist binding is ∼83% in the presence of 0.4 mM digitonin. The corresponding reduction in specific agonist binding is ∼42% (see ). Taken together, these results show that the complexation of free membrane cholesterol in hippocampal membranes results in the loss of the 5-HT1A receptor ligand binding ability. Although the requirement of membrane cholesterol for ligand binding to 5-HT1A receptors Citation[23], and other G-protein-coupled receptors Citation[41] has been demonstrated earlier, the present results constitute the first observation where the availability of free cholesterol rather than its mere physical presence in the membrane is essential for receptor function.
Figure 5. Effect of increasing concentrations of digitonin on specific [3H]p-MPPF binding to the 5-HT1A receptor in hippocampal membranes. Values are expressed as a percentage of specific binding for native membranes in the absence of digitonin. Data shown are the means±SE of at least three independent experiments. See Materials and methods for other details.
![Figure 5. Effect of increasing concentrations of digitonin on specific [3H]p-MPPF binding to the 5-HT1A receptor in hippocampal membranes. Values are expressed as a percentage of specific binding for native membranes in the absence of digitonin. Data shown are the means±SE of at least three independent experiments. See Materials and methods for other details.](/cms/asset/8200cf41-4f5d-4db6-8246-335ce5ad4d2e/imbc_a_109328_uf0005_b.jpg)
Change in hippocampal membrane dynamics due to digitonin treatment
The overall membrane order and dynamics could be an important determinant for the function of a transmembrane receptor, a large portion of which remains in contact with membrane lipids. In order to explore the possible changes in membrane order induced by digitonin treatment, we measured the fluorescence polarization of membrane depth-specific fluorescence probes DPH and TMA-DPH. In general, fluorescence polarization is correlated to the rotational diffusion Citation[38] of membrane embedded probes, which is sensitive to the packing of fatty acyl chains and cholesterol. Since membranes display a considerable degree of anisotropy in terms of motional properties of constituent lipids and proteins Citation[42], a comprehensive understanding of membrane dynamics can be achieved by the use of more than one probe which localize at different depths in the membrane. DPH and its derivatives represent popular probes for monitoring organization and dynamics in membranes Citation[43]. On account of its hydrophobicity, DPH readily partitions into the membrane and localizes at the fatty acyl chain region in the membrane. TMA-DPH is a derivative of DPH with a cationic moiety attached to the para position of one of the phenyl rings Citation[44]. The amphipathic TMA-DPH is oriented in the membrane with its positive charge localized at the membrane interface Citation[45]. The DPH moiety in TMA-DPH is localized at ∼11 Å from the center of the membrane and reports the interfacial region of the membrane Citation[46]. In contrast, the average location of DPH has been shown to be ∼8 Å from the center of the membrane Citation[46].
We observed an increase in the optical density of samples upon increasing the concentration of digitonin which could complicate fluorescence polarization measurements using DPH and TMA-DPH. The fluorescence polarization data obtained under these conditions were corrected for scattering artifacts according to the formalism previously developed by Lentz et al. Citation[39]. Representative plots (at 0.6 mM digitonin) of experimentally-measured fluorescence polarization as a function of optical density at the excitation wavelength are shown in . The intercept on the ordinate (y-axis) from linear fits of this data gives the corrected fluorescence polarization value Citation[39]. The correction significantly altered the polarization values of samples containing digitonin while the polarization values of control samples (without digitonin) remained unaltered.
Figure 6. Representative plots of fluorescence polarization as a function of optical density. The plots represent the fluorescence polarization of (a) DPH and (b) TMA-DPH for native membranes (○) which serve as control and 0.6 mM digitonin-treated membranes (•) upon dilution with 50 mM Tris, pH 7.4 buffer. Fluorescence polarization was calculated as described in Materials and methods at 23°C. The solid lines are linear fits to the data with the intercept on the ordinate (y-axis) considered as the corrected fluorescence polarization Citation[39]. The plots are data from at least three independent experiments. See Materials and methods for other details.
![Figure 6. Representative plots of fluorescence polarization as a function of optical density. The plots represent the fluorescence polarization of (a) DPH and (b) TMA-DPH for native membranes (○) which serve as control and 0.6 mM digitonin-treated membranes (•) upon dilution with 50 mM Tris, pH 7.4 buffer. Fluorescence polarization was calculated as described in Materials and methods at 23°C. The solid lines are linear fits to the data with the intercept on the ordinate (y-axis) considered as the corrected fluorescence polarization Citation[39]. The plots are data from at least three independent experiments. See Materials and methods for other details.](/cms/asset/2be9ee52-2c49-4936-9736-3bbe01f2f8d4/imbc_a_109328_uf0006_b.jpg)
Corrected fluorescence polarization values of DPH and TMA-DPH obtained this way as a function of digitonin concentration are shown in . As apparent from the figure, the corrected fluorescence polarization of DPH (∼0.35) in hippocampal membranes in the absence of digitonin is lower than that of TMA-DPH (∼0.37). The higher polarization of TMA-DPH compared to DPH is indicative of the shallower interfacial location of TMA-DPH in the membrane Citation[23]. The fluorescence polarization of both the probes shows an overall decrease with increasing concentrations of digitonin. Interestingly, the extent of reduction in fluorescence polarization is higher for DPH (11%) compared to TMA-DPH (7%) up to 1 mM digitonin used. The presence of digitonin (at concentrations that do not deplete membrane lipids, see and ) therefore induces asymmetric reduction in the membrane order in different regions of the membrane. Importantly, the relatively modest alterations in fluorescence polarization values of DPH and TMA-DPH in hippocampal membranes treated with digitonin ensure that the overall morphology of the membrane remains intact and rule out any possibility of gross changes in membrane architecture.
Figure 7. Effect of increasing concentrations of digitonin on fluorescence polarization of membrane probes DPH (○) and TMA-DPH (•) in hippocampal membranes. Fluorescence polarization experiments were carried out with membranes containing 50 nmol phospholipid at a probe to phospholipid ratio of 1:100 (mol/mol) at room temperature (23°C). The data show corrected fluorescence polarization values (as described in Materials and methods, and representative plots shown in ) and represent the means±SE of at least three independent experiments. The solid lines are linear fits of the data. See Materials and methods for other details.
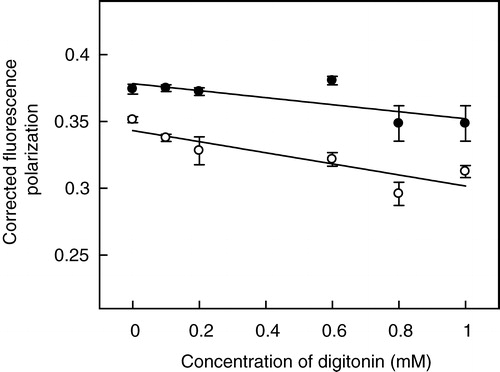
Discussion
Lipid-protein interactions play a crucial role in maintaining the structure and function of integral membrane proteins and receptors Citation[47,48]. Monitoring lipid-receptor interactions is of particular importance because a cell has the ability of varying the lipid composition of its membrane in response to a variety of stress and stimuli, thus changing the environment and the activity of the receptors in its membrane. In view of the importance of cholesterol in relation to membrane domains Citation[11,15], the interaction of cholesterol with membrane receptors Citation[16] represents an important determinant in functional studies of such receptors, especially in the nervous system.
We show here that complexation of cholesterol in hippocampal membranes using digitonin results in a concentration-dependent reduction in specific agonist and antagonist binding to 5-HT1A receptors. Complexation of membrane cholesterol with digitonin is believed to reduce the availability of free cholesterol (present data and Citation[26]) essential to support important membrane functions. This is supported by the observation that the presence of digitonin restores the otherwise suppressed phase transition Citation[49] of dipalmitoylphosphatidylcholine membranes containing cholesterol Citation[25]. The accompanying changes in membrane order were reported by corresponding changes in fluorescence polarization of membrane probes, such as DPH and TMA-DPH, which are localized at different positions (depths) in the membrane. These results point out the important role of membrane cholesterol in maintaining the function of the 5-HT1A receptor. This result assumes significance in the light of a tightly bound cholesterol molecule in the recently reported crystallographic structure of metarhodopsin I, an important photointermediate of rhodopsin which is a representative member of the G-protein-coupled receptor family Citation[50].
Our results on the inhibition of ligand binding to 5-HT1A receptors in the presence of the cholesterol-complexing agent digitonin confirm and extend our previous observation on the requirement of membrane cholesterol for efficient 5-HT1A receptor function Citation[23]. Importantly, since digitonin does not physically deplete cholesterol from membranes unlike agents such as methyl-β-cyclodextrin, our results serve to delineate the mechanism by which cholesterol exerts its influence on the 5-HT1A receptor function. We conclude that the mere non-availability of free cholesterol in the membrane is sufficient to significantly reduce the 5-HT1A receptor function. These results provide a comprehensive understanding of the effects of the sterol-complexing agent digitonin in particular, and the role of membrane cholesterol in general, on the 5-HT1A receptor function from a lipid-protein interaction perspective.
This paper was first published online on prEview on 28 April 2005.
This work was supported by the Council of Scientific and Industrial Research, Government of India. A.C. is an honorary faculty member of the Jawaharlal Nehru Centre for Advanced Scientific Research, Bangalore (India). Y.D.P. and T.J.P. thank the Council of Scientific and Industrial Research for the award of Junior and Senior Research Fellowships. We thank S. Rajanna, Shanti Kalipatnapu, Md. Jafurulla and Sandeep Shrivastava for help with the tissue collection and members of our laboratory for critically reading the manuscript.
References
- Pierce KL, Premont RT, Lefkowitz RJ. Seven-transmembrane receptors. Nat Rev Mol Cell Biol 2002; 3: 639–650
- Cohen-Tannoudji J, Counis R, Hervé D, Shi DL, Vergé D. G protein coupled receptors: new insights into signaling and regulation. Biol Cell 2004; 96: 325–326
- Edwards SW, Tan CM, Limbird LE. Localization of G-protein-coupled receptors in health and disease. Trends Pharmacol Sci 2000 2003; 21: 304–308
- Bikker JA, Trumpp-Kallmeyer S, Humblet C. G-protein coupled receptors: models, mutagenesis, and drug design. J Med Chem 1998; 41: 2911–2927
- Karnik SS, Gogonea S, Patil S, Saad Y, Takezako T. Activation of G-protein-coupled receptors: a common molecular mechanism. Trends Endocrinol Metab 2003; 14: 431–437
- Ostrom RS, Insel PA. The evolving role of lipid rafts and caveolae in G protein-coupled receptor signaling: implications for molecular pharmacology. Br J Pharmacol 2004; 143: 235–245
- Ostrom RS, Post SR, Insel PA. Stoichiometry and compartmentation in G protein-coupled receptor signaling: implications for therapeutic interventions involving Gs. J Pharmacol Exp Ther 2000; 294: 407–412
- Ostrom RS. New determinants of receptor-effector coupling: trafficking and compartmentation in membrane microdomains. Mol Pharmacol 2002; 61: 473–476
- Dreja K, Voldstedlund M, Vinten J, Tranum-Jensen J, Hellstrand P, Swärd K. Cholesterol depletion distrupts caveolae and differentially impairs agonist-induced arterial contraction. Arterioscler Thromb Vasc Biol 2002; 22: 1267–1272
- Bhatnagar A, Sheffler DJ, Kroeze WK, Compton-Toth B, Roth BL. Caveolin-1 interacts with 5-HT2A serotonin receptors and profoundly modulates the signaling of selected Gαq-coupled protein receptors. J Biol Chem 2004; 279: 34614–34623
- Liscum L, Underwood KW. Intracellular cholesterol transport and compartmentation. J Biol Chem 1995; 270: 15443–15446
- Simons K, Ikonen E. How cells handle cholesterol. Science 2000; 290: 1721–1725
- Schroeder F, Woodford JK, Kavecansky J, Wood WG, Joiner C. Cholesterol domains in biological membranes. Mol Membr Biol 1995; 12: 113–119
- Rukmini R, Rawat SS, Biswas SC, Chattopadhyay A. Cholesterol organization in membranes at low concentrations: effects of curvature stress and membrane thickness. Biophys J 2001; 81: 2122–2134
- Simons K, Ikonen E. Functional rafts in cell membranes. Nature 1997; 387: 569–572
- Burger K, Gimpl G, Fahrenholz F. Regulation of receptor function by cholesterol. Cell Mol Life Sci 2000; 57: 1577–1592
- Peroutka SJ, Howell TA. The molecular evolution of G protein-coupled receptors: focus on 5-hydroxytryptamine receptors. Neuropharmacology 1994; 33: 319–324
- Hoyer D, Hannon JP, Martin GR. Molecular, pharmacological and functional diversity of 5-HT receptors. Pharmacol Biochem Behav 2002; 71: 533–554
- Clapham DE. The G-protein nanomachine. Nature 1996; 379: 297–299
- Pucadyil, TJ, Kalipatnapu, S, Chattopadhyay, A. The serotonin1A receptor: a representative member of the serotonin receptor family. Cell Mol Neurobiol 2005; (in press).
- Chattopadhyay A, Harikumar KG, Kalipatnapu S. Solubilization of high affinity G-protein-coupled serotonin1A receptors from bovine hippocampus using pre-miceller CHAPS at low concentration. Mol Membr Biol 2002; 19: 211–220
- Chattopadhyay A, Jafurulla MD, Kalipatnapu S, Pucadyil TJ, Harikumar KG. Role of cholesterol in ligand binding and G-protein coupling of serotonin1A receptors solubilized from bovine hippocampus. Biochem Biophys Res Commun 2005; 327: 1036–1041
- Pucadyil TJ, Chattopadhyay A. Cholesterol modulates ligand binding and G-protein coupling to serotonin1A receptors from bovine hippocampus. Biochim Biophys Acta 2004; 1663: 188–200
- Elias PM, Goerke J, Friend DS, Brown BE. Freeze-fracture identification of sterol-digitonin complexes in cell and liposome membranes. J Cell Biol 1978; 78: 577–596
- Nishikawa M, Nojima S, Akiyama T, Sankawa U, Inoue K. Interaction of digitonin and its analogs with membrane cholesterol. J Biochem 1984; 96: 1231–1239
- Severs NJ, Robenek H. Detection of microdomains in biomembranes: an appraisal of recent developments in freeze-fracture cytochemistry. Biochim Biophys Acta 1983; 737: 373–408
- Akiyama T, Takagi S, Sankawa U, Inari S, Saitô H. Saponin-cholesterol interaction in the multibilayers of egg yolk lecithin as studied by deuterium nuclear magnetic resonance: digitonin and its analogues. Biochemistry 1980; 19: 1904–1911
- Dontchev V, Ichev K, Ovtscharoff W, Surchev L. Light and electron microscopic demonstration of cholesterol distribution in membrane structures of the rat auditory cortex. Acta Histochem 1994; 96: 165–174
- Smith PK, Krohn RI, Hermanson GT, Mallia AK, Gartner FH, Provenzano MD, Fujimoto EK, Goeke NM, Olson BJ, Klenk DC. Measurement of protein using bicinchoninic acid. Anal Biochem 1985; 150: 76–85
- Winter CG. Digitonin as an uncoupler of the partial reactions of Na+, K+-ATPase. Ann NY Acad Sci 1974; 242: 149–157
- Pucadyil TJ, Chattopadhyay A. Exploring detergent insolubility in bovine hippocampal membranes: a critical assessment of the requirement for cholesterol. Biochim Biophys Acta 2004; 1661: 9–17
- McClare CWF. An accurate and convenient organic phosphorus assay. Anal Biochem 1971; 39: 527–530
- Amundson DM, Zhou M. Fluorometric method for the enzymatic determination of cholesterol. J Biochem Biophys Methods 1999; 38: 43–52
- Bligh EG, Dyer WJ. A rapid method of total lipid extraction and purification. Can J Biochem Physiol 1959; 37: 911–917
- Harikumar KG, Chattopadhyay A. Differential discrimination of G-protein coupling of serotonin1A receptors from bovine hippocampus by an agonist and an antagonist. FEBS Lett 1999; 457: 389–392
- Kalipatnapu S, Jafurulla MD, Chandrasekaran N, Chattopadhyay A. Effect of Mg2 + on guanine nucleotide sensitivity of ligand binding to serotonin1A receptors from bovine hippocampus. Biochem Biophys Res Commun 2004; 323: 372–376
- Chattopadhyay A, London E. Fluorimetric determination of critical micelle concentration avoiding interference from detergent charge. Anal Biochem 1984; 139: 408–412
- Lakowicz JR. Principles of fluorescence spectroscopy2nd ed. Kluwer-Plenum Press, New York 1999
- Lentz BR, Moore BM, Barrow DA. Light-scattering effects in the measurement of membrane microviscosity with diphenylhexatriene. Biophys J 1979; 25: 489–494
- Bahouth SW, Malbon CC. Human β-adrenergic receptors: simultaneous purification of β1- and β2-adrenergic-receptor peptides. Biochem J 1987; 248: 557–566
- Klein U, Gimpl G, Fahrenholz F. Alteration of the myometrial plasma membrane cholesterol content with beta-cyclodextrin modulates the binding affinity of the oxytocin receptor. Biochemistry 1995; 34: 13784–13793
- Chattopadhyay A. Exploring membrane organization and dynamics by the wavelength-selective fluorescence approach. Chem Phys Lipids 2003; 122: 3–17
- Lentz BR. Membrane fluidity as detected by diphenylhexatriene probes. Chem Phys Lipids 1989; 50: 171–190
- Prendergast FG, Haugland RP, Callahan PJ. 1-[4-(Trimethylamino)phenyl]-6-phenylhexa-1,3,5-triene: synthesis, fluorescence properties, and use as a fluorescence probe of lipid bilayers. Biochemistry 1981; 20: 7333–7338
- Sojcic Z, Toplak H, Zuehlke R, Honegger UE, Buhlmann R, Wiesmann UN. Cultured human skin fibroblasts modify their plasma membrane lipid composition and fluidity according to growth temperature suggesting homeoviscous adaptation at hypothermic (30°C) but not at hyperthermic (40°C) temperatures. Biochim Biophys Acta 1992; 1104: 31–37
- Kaiser RD, London E. Location of diphenylhexatriene (DPH) and its derivatives within membranes: comparison of different fluorescence quenching analyses of membrane depth. Biochemistry 1998; 37: 8180–8190
- Lee AG. How lipids affect the activities of integral membrane proteins. Biochim Biophys Acta 2004; 1666: 62–87
- Jensen MØ, Mouritsen OG. Lipids do influence protein function – the hydrophobic matching hypothesis revisited. Biochim Biophys Acta 2004; 1666: 205–226
- Estep TN, Mountcastle DB, Biltonen RL, Thompson TE. Studies on the anomalous thermotropic behavior of aqueous dispersions of dipalmitoylphosphatidylcholine-cholesterol mixtures. Biochemistry 1978; 17: 1984–1989
- Ruprecht JJ, Mielke T, Vogel R, Villa C, Schertler GF. Electron crystallography reveals the structure of metarhodopsin. EMBO J 2004; 23: 3609–3620