Abstract
Type 2 diabetes (T2D) and T2D-associated comorbidities, such as obesity, are serious universally prevalent health issues among post-menopausal women. Menopause is an unavoidable condition characterized by the depletion of estrogen, a gonadotropic hormone responsible for secondary sexual characteristics in women. In addition to sexual dimorphism, estrogen also participates in glucose–lipid homeostasis, and estrogen depletion is associated with insulin resistance in the female body. Estrogen level in the gut also regulates the microbiota composition, and even conjugated estrogen is actively metabolized by the estrobolome to maintain insulin levels. Moreover, post-menopausal gut microbiota is different from the pre-menopausal gut microbiota, as it is less diverse and lacks the mucolytic Akkermansia and short-chain fatty acid (SCFA) producers such as Faecalibacterium and Roseburia. Through various metabolites (SCFAs, secondary bile acid, and serotonin), the gut microbiota plays a significant role in regulating glucose homeostasis, oxidative stress, and T2D-associated pro-inflammatory cytokines (IL-1, IL-6). While gut dysbiosis is common among post-menopausal women, dietary interventions such as probiotics, prebiotics, and synbiotics can ease post-menopausal gut dysbiosis. The objective of this review is to understand the relationship between post-menopausal gut dysbiosis and T2D-associated factors. Additionally, the study also provided dietary recommendations to avoid T2D progression among post-menopausal women.
Introduction
Almost 38 trillion microbes harbor the human intestinal tract and are collectively known as the gut microbiota. The gut microbiota functions as a virtual organ and affects host health and metabolism by producing several metabolites such as short-chain fatty acids (SCFAs; mainly acetate, propionate, and butyrate) and secondary bile acids (Valdes et al. Citation2018). Gut microbiota is a dynamic community that is easily affected by several factors such as diet, disease, metabolic variation, and age.
Natural menopause is a serious metabolic variation in mid-age women, elevating dyslipidemia, obesity, and irregular carbohydrate metabolism, which affect the gut microbial community (Santos-Marcos et al. Citation2018). Usually, menopause occurs in mid-aged women (47–52 years) when their ovaries stop maturing ovum and simultaneously ceases estrogen and progesterone secretion. According to the National Institute for Health and Care Excellence (NICE, UK) guidelines, the time after 12 successive months of the last period or menstrual cycle is regarded as post-menopause (Hope Citation2016). This permanent shift from the reproductive to post-reproductive phase causes multiple metabolic and psychotic changes in a female body, mainly due to the depleted female steroid hormones. Hormonal depletion among post-menopausal women potentially enhances their vulnerability to disorders in the hormone-responsive tissues such as brain, kidney, bones, and cardiovascular system (Lobo et al. Citation2014). Additionally, metabolic disorders including abdominal obesity, dyslipidemia, insulin resistance, impaired glucose tolerance, and type 2 diabetes (T2D), may also occur because of menopause, affecting the quality of life during the post-menopausal phase (Joon Cho et al. Citation2008; Stachowiak, Pertyński, and Pertyńska-Marczewska Citation2015).
In general, women are less susceptible to metabolic diseases than men. However, this situation reverses after menopause, particularly in cases of diabetes and cardiovascular disease (CVD) (Heianza et al. Citation2013; Joon Cho et al. Citation2008; Lisabeth and Bushnell Citation2012). The depleted levels of the gonadotropic hormone estrogen causes this susceptibility in women, leading to detrimental effects (Rosano et al. Citation2007). This is further fueled by dyslipidemia, characterized by higher low-density lipoprotein (LDL) and lower high-density lipoprotein (HDL) levels, leading to CVD (Stachowiak, Pertyński, and Pertyńska-Marczewska Citation2015). Previous studies have also addressed several associated metabolic risk factors termed as metabolic syndrome (MS), characterized by obesity, T2D, insulin resistance, lower HDL level, high blood pressure, and elevated triglyceride level, negatively affecting the quality of life and life expectancy among post-menopausal women (Liang et al. Citation2013; Pu et al. Citation2017).
Post-menopausal period is a very sensitive phase for diabetes and can even be regarded as a period of active diabetic vigilance. The association between menopausal status and T2D is also supported by multiple longitudinal cohort studies conducted in South Africa, China, and other countries (Mauvais-Jarvis et al. Citation2017; Mtintsilana et al. Citation2019). Estrogen depletion after menopause is a major cause of obesity and T2D among elderly women (Marchand et al. Citation2018; Ren et al. Citation2019). Estrogen is actively metabolized by the gut microbiota, and its depletion shifts gut microbiota, enhances intestinal permeability, and induces obesity (Vieira et al. Citation2017). This review systematically examines the discrete information regarding the roles of gut microbiota associated with post-menopausal T2D, thereby providing a detailed account of the relevance of dietary interventions in its regulation.
T2D and its relation to post-menopausal repercussions
T2D is a complex metabolic disorder where the body cannot utilize glucose in the blood. This condition arises due to a lack of insulin or imperfect response to insulin. According to the World Health Organization (WHO), the mortality of diabetes was 1.5 million in 2019 (https://www.who.int/news-room/fact-sheets/detail/diabetes), with T2D being more common (90%) than type 1 diabetes (Franzosa et al. Citation2019). T2D etiology is a complex process characterized by persistent hyperglycemia and depends on multiple factors, ranging from genetic to lifestyle choices (Carlsson Citation2019; Kommoju and Reddy Citation2011).
Insulin receptors are present in almost all tissues, when insulin binds to these receptors, glucose transport proteins are activated, enhancing glycogenesis and lipid biosynthesis, and reducing gluconeogenesis (De Paoli, Zakharia, and Werstuck Citation2021). When insulin receptors do not respond, insulin resistance absurdly increases hepatic glucose production, thus secreting insulin (De Paoli, Zakharia, and Werstuck Citation2021). Insulin resistance in skeletal muscles is the earliest detectable sign of T2D (Taylor Citation2013). T2D is one of the most common metabolic ailments among post-menopausal women, which further lead to other severe metabolic conditions such as CVD and chronic kidney diseases (Ahmed Citation2017; Koye et al. Citation2018). A major reason for this susceptibility is the depletion of a primary sex hormone, estrogen, after menopause. summarizes the ailments in several organs caused by estrogen depletion in post-menopausal women.
Table 1. A summary of post-menopausal ailments.
Estrogen actively participates in glucose metabolism; thus, a low estrogen level (∼10 pg/mL) escalates T2D etiology by increasing inflammation and inducing obesity (De Paoli, Zakharia, and Werstuck Citation2021; Ko and Kim Citation2020). The effects of estrogen on various T2D-related metabolic activities are summarized in . Estrogen binds to its receptors, then undergoes conformational changes, and are translocated to the nucleus where they act as mitogenic stimulants and regulate immunological cell expression (Moulton Citation2018; Straub Citation2007). Moreover, estrogen receptors are expressed in the visceral, hepatic, and subcutaneous adipocytes, so that a lower level of estrogens enhances fat accumulation in the body (Ko and Kim Citation2020; Marchand et al. Citation2018). This surplus fat adversely affects lipid metabolism by producing excessive triglycerides and free fatty acids. These triglycerides and free fatty acids accumulate in non-fatty tissues and impair insulin signaling and β-cell regulation, causing insulin resistance (Ko and Kim Citation2020). Estrogen also acts effectively against T2D by promoting degradation of ill-folded pro-insulin, thus reviving the endoplasmic reticulum from stress and β-cell dysfunction (Xu et al. Citation2018).
Association of gut microbiota with post-menopausal T2D
Post-menopausal gut dysbiosis, metabolic changes, and their relation with T2D
Estrogen deprivation causes gut dysbiosis, which induces and worsens the symptoms of T2D. The characteristics of post-menopausal gut dysbiosis include lower Firmicute/Bacteroidetes ratio, lower abundance of the family Lachnospiraceae, and a higher abundance of the genera Prevotella, Parabacteroides, Bacteroides, and Bilophila (Mayneris-Perxachs et al. Citation2020; Ozaki et al. Citation2021; Santos-Marcos et al. Citation2018). Other studies have reported that post-menopausal gut microbiota have few SCFA producers such as Faecalibacterium, Bifidobacterium, Alistipes, Ruminococcus, and Roseburia (Mayneris-Perxachs et al. Citation2020; Santos-Marcos et al. Citation2018; Schreurs et al. Citation2021; Zhao et al. Citation2019). Interestingly, the abundance of Faecalibacterium and Roseburia in post-menopausal women with T2D or obesity is often more decreased than that in non-T2D post-menopausal women (Alemán et al. Citation2018). Conversely, “metformin,” a commonly used antidiabetic, increases the abundance of SCFA-producing bacteria, while improving glucose regulation (Wang, Yu et al. Citation2021). In addition to the low abundance of SCFA producers, post-menopausal women also have a low abundance of other beneficial bacteria such as A. muciniphila, Eubacterium eligens, E. rectale, and L. vaginalis (Brahe et al. Citation2015b; Schreurs et al. Citation2021; Zhu et al. Citation2018). Obesity during post-menopause worsens post-menopausal gut dysbiosis due to reduced microbial richness and low abundance of A. muciniphila (Brahe et al. Citation2015b; Schreurs et al. Citation2021). As A. muciniphila is responsible for maintaining the thickness of the gut epithelial mucus layer, its reduced abundance can lead to leaky gut, enhancing the translocation of inflammatory toxins through the gut epithelial layer (Brahe et al. Citation2015b).
Estrogen and estrogen-like compounds modulate the gut microbial ecosystem and promote the proliferation of certain microbial communities over others (Chen and Madak-Erdogan Citation2016; Kaliannan et al. Citation2018). Gut microbiota with genes encoding estrogen-metabolizing enzymes, referred to as the “estrobolome,” flourishes in the presence of estrogen (Chen and Madak-Erdogan Citation2016). Studies also reported that low microbial diversity during the post-menopausal period adversely affects microbial beta-glucuronidase activity (Baker, Al-Nakkash, and Herbst-Kralovetz Citation2017). Beta-glucuronidase enzyme influences estrogen metabolism by deconjugating estrogen and phytoestrogen, making them available in the bloodstream upon absorption in the gut. These freed estrogens act on estrogen receptors to regulate blood glucose levels (Baker, Al-Nakkash, and Herbst-Kralovetz Citation2017; Kwa et al. Citation2016). Studies on ovariectomized murine models have also demonstrated that lack of estrogen reduces the abundance of the genera Bacteroidetes and Akkermansia, while inducing a significantly high level of serum cholesterol, hepatic adipogenesis, and expression of lipogenesis genes, suggesting that estrogen plays important roles in obesity, glucose, and lipid homeostasis through modulating gut microbiota (Acharya et al. Citation2021; Lei et al. Citation2021). In addition, the gut microbiome during the post-menopausal period is reported to be filled with higher trimethylamine N-Oxide (TMAO) and hydrogen sulfide (H2S), which increase gluconeogenesis and so the glucose level (Grassi et al. Citation2016; Jang and Lee Citation2021; Liu et al. Citation2020). TMAO is synthesized from trimethylamine by gut microbiota, and H2S is generated by colonic sulfur-reducing microbes (Blachier et al. Citation2021; Janeiro et al. Citation2018).
Studies on intestinal metabolic differences between pre and post-menopausal gut microbiota showed that metabolic activities involved in the pentose phosphate pathway were enriched in pre-menopausal microbiota, while those involved in homocysteine synthesis was enriched in post-menopausal microbiota (Zhao et al. Citation2019). The pentose phosphate pathway generates acetyl-CoA, which forms butyrate-CoA, a substrate that directly participates in butyrate production (Ge et al. Citation2020; Louis and Flint Citation2017), suggesting a low amount of SCFAs in the gut of post-menopausal women. Together, menopause induces gut dysbiosis, and direct hormonal functions are prone to induce obesity and diabetes (). Other than that, post-menopausal osteoporosis is another common ailment induced due to estrogen deficiency resulting in lower bone density due to higher bone resorption and lower bone rate of bone formation, which leads to the higher occurrence of bone fracture (Eastell et al. Citation2016). Common gut-associated adversities among post-menopausal women are summarized in .
Figure 2. Impact of Post-menopausal Type 2 Diabetes; Post-menopausal type 2 diabetes affects the multiple regulators including gut microbiota to metabolism, and their impact can be seen on various T2D specific markers i.e., BMI, blood glucose level.
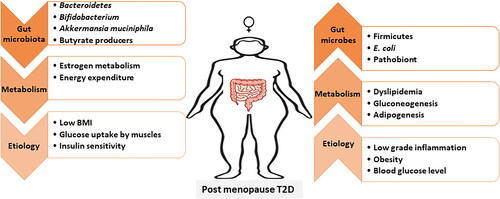
Table 2. Common gut associated adversities in post-menopausal women.
Regulatory microbial metabolites in T2D
Decreased SCFAs adversely influences the signal transduction linked to T2D factors, such as glucose utilization and dyslipidemia (Alexander et al. Citation2019). SCFAs enhance glucose uptake in skeletal muscles via glucose transporter protein (GLUT4) (Frampton et al. Citation2020). GPCR41- and GPCR43-bound SCFAs increase plasma GLP-1 levels, maintain glucose homeostasis, and control appetite (Delzenne et al. Citation2015). Propionate also controls blood glucose levels by suppressing gluconeogenesis in the liver by increasing the phosphorylation of AMP-activated protein kinase (AMPK) (Jang and Lee Citation2021). Activated AMPK inhibits gluconeogenesis by inhibiting various transcription factors that control the expression of gluconeogenesis enzymes, such as glucose-6-phosphatase and phosphoenolpyruvate carboxykinase (Jeon Citation2016). Additionally, propionate also enhances GLP-1, which reduces hepatic visceral fat by limiting triglyceride synthesis. Propionate regulates cellular lipid metabolism by modulating Fiaf, GPCR43, and histone deacetylases (HDAC) (Lukovac et al. Citation2014). Members of the phylum Bacteroidetes are the largest propionate producers in the gut (Chambers et al. Citation2015; Rios-Covian et al. Citation2017), thus a decreased Bacteroidetes abundance can result in increased blood glucose level (Cunningham, Stephens, and Harris Citation2021).
Reduced SCFAs are linked to decreased production of mucin 2, a major component of mucin protein present in the gut mucus layer, thus compromising the gut epithelial barrier (Fang et al. Citation2021). The reduction of the mucus layer decreases the abundance of the genus Akkermansia, which exerts antidiabetic effects through the Amuc_1100 outer membrane protein. Amuc_1100 plays an immunoregulatory role in the gut and promotes the secretion of serotonin, a glucose-homeostatic neurotransmitter (Wang, Xu et al. Citation2021). A. muciniphila also produces propionate in the gut that regulates the blood glucose as discussed above. Moreover, A. muciniphila and Bacteroides species are negatively associated with T2D markers, such as insulin resistance, low-grade inflammation, and lipid dysregulation (Brahe et al. Citation2015b). Hence, A. muciniphila regulates T2D and obesity via glucose homeostasis. Even oral administration of A. muciniphila is also effective in countering insulin resistance (Depommier et al. Citation2020; Greer et al. Citation2016). Interestingly, metformin, the first line of defense medicine against T2D, also enhances A. muciniphila abundance in T2D patients, which further supports the antidiabetic role of Akkermansia (Lee, Ko, and Griffiths Citation2014).
Gut microbiota also participates in glucose homeostasis regulation by controlling serotonin secretion from enterochromaffin cells (EC cells) in the gut lining (Martin et al. Citation2019). Serotonin is a neurotransmitter associated with glucose homeostasis as it regulates insulin secretion from pancreatic β-cells, while improving insulin sensitivity and hyperglycemia (Al-Zoairy et al. Citation2017; Watanabe, Rose, and Aso Citation2011). Approximately 90% serotonin is secreted into the body from EC cells, and the gut microbiota controls its secretion by signaling EC cells through metabolites, such as SCFAs and secondary bile acids (Martin et al. Citation2019). In addition, Amuc_1100, an outer surface protein of the mucolytic A. muciniphila, also participates in serotonin biosynthesis (Wang, Xu et al. Citation2021).
Secondary bile acids are also a major type of metabolites that play an important role in T2D development. Bile acids are synthesized in the liver, help in lipid digestion, and also function as endocrine molecules that enhance glucagon-like peptide-1 (GLP-1) secretion in the gut (Taylor et al. Citation2014; Zaborska and Cummings Citation2018). Most of the bile acids are actively absorbed back into the ileum through bile acid transporters, and only a small fraction of them reaches the colon (Legry et al. Citation2017). Gut microbiota metabolizes bile acids into secondary bile acids, such as deoxycholic acid (DCA) and lithocholic acid (LCA). Secondary bile acids are bactericidal and play a critical role in balancing the microbial community by suppressing pathobionts. However, excessive amounts of secondary bile acids may inhibit the growth of Bacteroidetes and Actinobacteria phyla, increasing the overall abundance of phylum Firmicutes (Ridlon et al. Citation2014). Secondary bile acids also interrupt glucose homeostasis by inhibiting TGR5, which participates in glucose homeostasis at high energy expenditure in muscles and brown adipose tissues while releasing GLP-1 (Molinaro, Wahlström, and Marschall Citation2018). Although some gut microbes are also known to reduce the secondary bile acid toxicity by transforming DCA and LCA into iso-DCA and iso-LCA, respectively (Legry et al. Citation2017).
Gut microbiota in regulation of oxidative stress and systemic inflammation
Oxidative stress indicates inner mitochondrial and cellular damage caused by reactive oxygen species (ROS) and reactive nitrogen species (RNS). ROS and RNS are extremely reactive and unstable free radicals that can activate various pathways with an adverse impact on the body (Ceriello, Testa, and Genovese Citation2016). Oxidative stress overloads glucose, and increased oxidative stress can lead to insulin resistance (Molehin, Adefegha, and Adeyanju Citation2020; Wright, Scism‐Bacon, and Glass Citation2006). Several studies have also reported associations between increased oxidative stress and T2D among post-menopausal women due to low free thiol (R–SH) level, a key extracellular antioxidant (Bourgonje et al. Citation2021; Song et al. Citation2009).
Imbalanced gut microbial communities allow the propagation of opportunistic pathogens in the gut. Invasion by these bacteria secreting toxic metabolites (such as lipopolysaccharides (LPS) and enterotoxins) causes leaky gut syndrome and systemic inflammation. Systemic inflammation is associated with insulin resistance and T2D in post-menopausal women. Pro-inflammatory cytokine levels, such as IL-1, IL-6, and TNF-α, are high among T2D patients (Spranger et al. Citation2003). These pro-inflammatory cytokines induce insulin resistance (Kanmani et al. Citation2019) and regulate C-reactive protein, a major inflammatory marker of T2D. Chronic inflammation with elevated C-reactive protein levels is associated with T2D (Kanmani et al. Citation2019; Masala et al. Citation2020). On the other hand, IL-1 hinders insulin secretion and induces apoptosis in pancreatic β-cells, thus contributing to T2D occurrence (Banerjee and Saxena Citation2012). Moreover, an inflammatory load is negatively associated with circulatory estrogen levels and obesity among post-menopausal women (Masala et al. Citation2020).
Dietary interventions and their efficacy in controlling T2D severity
Diet directly affects most T2D components, such as glucose metabolism, caloric intake, lipid metabolism, and gut microbiota. Since gut microbiota largely impacts the metabolic regulation among T2D patients, various dietary interventions have been studied to understand their possible beneficial roles in post-menopausal T2D. The effect of these dietary interventions are mostly mediated by gut microbiota, and they benefit the host in multiple ways by simultaneously affecting the inflammatory markers and glucose-regulatory metabolism ().
Biologically active compounds in diet
Isoflavones are polyphenolic compounds classified as phytoestrogens, as their functions are similar to those of human estrogen. Isoflavone-rich dietary sources such as soybean, fermented soy products, and chickpea sprouts are advised for post-menopausal T2D women to maintain a healthy gut microbiome (Fukuda, Kobayashi, and Honda Citation2017; Ma et al. Citation2013). Studies also reported that isoflavone administration effectively enhances the abundance of butyrogenic E. rectale (Zhao et al. Citation2019). Daidzin, an isoflavonoid abundant in a soy-based diet, can be metabolized by gut microbes into compounds similar to estrogen (Chen and Madak-Erdogan Citation2016). Another isoflavone, Genistein is metabolized by the gut microbiota into 4-ethylphenyl sulfate, 3-phenylpropionate, and methyl-4-hydroxybenzoate, which improves the oxidative capacity of muscles and ameliorates T2D symptoms in post-menopausal women (Braxas et al. Citation2019; Guevara-Cruz et al. Citation2020). Genistein also decreases endotoxemia and controls body glucose levels by supporting Akkermansia growth (Braxas et al. Citation2019). Soy isoflavone administration in post-menopausal women has increased the count of Bifidobacterium, while decreasing the abundance of family Clostridiaceae, which participates in systemic inflammation among post-menopausal obese women (Vieira et al. Citation2017).
Dietary polyphenols also enrich A. muciniphila and reduce glucose-6-phosphate mRNA expression in a T2D mouse model, which eventually reduces gluconeogenesis and improves fasting hyperglycemia (Everard et al. Citation2013). Most polyphenols are indigestible, thus reaching the large intestine where more than 90% polyphenols are metabolized by gut microbiota to low molecular weight active polyphenolic compounds, such as phenolic acids and hydroxyphenyl moieties (Cardona et al. Citation2013; Wan, Co, and El-Nezami Citation2021). Green tea is a rich source of polyphenol “catechins,” which account for 30–40% brewed tea solids, and is metabolized by Eubacterium, Enterobacter, B. longum, L. plantarum, and Bacillus subtilis (Chen and Sang Citation2014; Guo et al. Citation2019). Catechins also limit the abundance of possible harmful intestinal microbes such as Helicobacter pylori, Escherichia coli, and Staphylococcus aureus, while maintaining a high abundance of beneficial bacteria such as Bifidobacterium and Lactobacillus (Guo et al. Citation2019). Tea catechin also decreases Firmicutes/Bacteroidetes ratio, generally referred to as an obesity indicator, while effectively controlling the postprandial glucose level and oxidative stress among post-menopausal T2D patients (Guo et al. Citation2019; Takahashi et al. Citation2014).
Bioactive compounds obtained from turmeric herb also have proven effective in T2D treatment. Turmerin is a secondary metabolite of turmeric with an antidiabetic property (Kalaycıoğlu, Gazioğlu, and Erim Citation2017; Lekshmi et al. Citation2012). Turmeric oil inhibits the activity of glucosidase, which is commonly used to control postprandial hyperglycemic conditions (Lekshmi et al. Citation2012). On the other hand, curcumin, a polyphenolic compound present in turmeric, boosts the abundance of probiotics (Bifidobacterium and Lactobacillus) while reducing pathogenic strains such as Enterobacteria and Enterococci (Di Meo et al. Citation2019). Curcumin also manages oxidative stress and inhibits several pro-inflammatory cytokines in adipose tissue to control systemic inflammation in T2D patients (Pivari et al. Citation2019). A murine study also reported that curcumin shifts the gut microbiota and reduces weight gain in estrogen-deficient ovariectomized rats (Zhang et al. Citation2017). Spent turmeric has significant antioxidant activity and contains almost 45% fiber that can be metabolized by the gut microbiota to produce SCFAs (Sowbhagya Citation2019).
Dietary glucosinolates are abundant in broccoli and metabolized by gut microbiota to isothiocyanate sulforaphane, which reduces blood glucose levels in T2D patients (Angelino et al. Citation2015; Liou et al. Citation2020). Additionally, broccoli is rich in phytoestrogens and helps to curb post-menopausal hormone-dependent dysregulations, including obesity and T2D (Petrine and Bianco-Borges Citation2021; Shoff et al. Citation1998). Gut microbiota metabolizes phytoestrogens to produce bioactive substances that interact with estrogen receptors and induce estrogenic effects (Stojanov and Kreft Citation2020).
Plant seed mucilage shifts gut microbiota, improves serum lipid profiles, and increases insulin sensitivity among post-menopausal women (Soukoulis, Gaiani, and Hoffmann Citation2018). Flaxseed mucilage, for example, improves postprandial glucose, insulin sensitivity, and lipid profile (Kay et al. Citation2017; Soltanian and Janghorbani Citation2018), while modulating gut microbiota in post-menopausal obese women (Brahe et al. Citation2015a). Flaxseed mucilage treatment in mice also enhances the abundance of genera Lactobacillus and Clostridium, which are often depleted in post-menopausal women. Some Clostridium species chiefly metabolize flaxseed mucilage to produce acetate and propionate (Luo et al. Citation2018). Another study has showed the anti-obesity effects of flaxseed by significantly increasing the abundance of genera Akkermansia and Bifidobacterium and decreasing that of obesity-associated bacteria (i.e., Oscillospiraceae) in mice gut microbiota (Xu, Chen et al. Citation2020). Flaxseed polysaccharides also limit oxidative stress and reduce pro-inflammatory markers, such as IL-6 and IL-1, while enhancing the anti-inflammatory cytokine IL-10 (Xu, Chen et al. Citation2020). Yellow mustard mucilage also has antioxidant properties and improves postprandial glycemic conditions (Lett, Thondre, and Rosenthal Citation2013; Wu et al. Citation2016). Fenugreeks have antidiabetic effects because they improve postprandial glycemic conditions and glucose homeostasis in T2D patients (Hannan et al. Citation2007; Kay et al. Citation2017). Moreover, fenugreek seed mucilage and spent turmeric are rich dietary fiber sources that effectively limit the higher disaccharidase (maltase, sucrase, and lactase) activity in diabetics (Kumar, Shetty, and Salimath Citation2005). Additionally, in vitro colonic fermentation of fenugreek enhances the abundance of Bifidobacterium and Lactobacillus, which are depleted in post-menopausal T2D patients (Kumar, Shetty, and Salimath Citation2005; Navarro del Hierro et al. Citation2020). Murine studies have also reported the therapeutic relevance of fenugreek fiber in T2D by improving glucose tolerance, dyslipidemia, and weight gain. In addition, fenugreek fiber also enhances the abundance of phylum Bacteroidetes and family Lachnospiraceae, members of which are often depleted among obese people and post-menopausal T2D patients (Bruce-Keller et al. Citation2020; Ozaki et al. Citation2021; Shtriker et al. Citation2018). Lastly, clinical studies have proven that fenugreek seed is effective against hyperlipidemia, inflammation, and oxidative stress in T2D patients. Thus, regular fenugreek seed administration may help T2D control in post-menopausal women (Chaturvedi et al. Citation2013; Roberts Citation2011; Tavakoly et al. Citation2018). Besides fenugreek seed, chia seed mucilage is also a potential remedy for post-menopausal T2D patients because it has antidiabetic properties and increases the abundance of Lactobacillus and the amount of SCFAs in vitro, although further studies are required to investigate how it modulates the gut microbiome in T2D patients (Tamargo et al. Citation2018).
A healthy diet normally comprises omega 3 fatty acids (n-3) and omega 6 fatty acids (n-6), where n-3 is effective in significantly controlling insulin resistance, triglycerides, and pro-inflammatory IL-6 among post-menopausal women with metabolic syndromes (Tardivo et al. Citation2015). Clinical evidences suggest that n-3 reverses dysbiosis by restoring Firmicutes/Bacteroidetes proportion and enhancing the Lachnospiraceae members producing anti-inflammatory metabolites such as butyrate (Costantini et al. Citation2017). Moreover, studies have also shown that n-3 controls endotoxemia by increasing the abundance of probiotics (i.e., Bifidobacterium) and decreasing that of gram-negative bacteria whose LPS causes systemic inflammation (Cani et al. Citation2007; Costantini et al. Citation2017). Interestingly, further studies have suggested that a rationalized mixture of n-3 and n-6 is more effective in controlling the elevated triglycerides in post-menopausal T2D women than individual treatments (Griffin et al. Citation2006).
Effect of prebiotics and probiotics against T2D progression
Prebiotics are non-digestible polysaccharides and oligosaccharides that confer beneficial effects to the host by supporting beneficial bacterial growth. summarizes some prebiotics reported to reduce insulin resistance and T2D progression. However, clinical trials have shown that the administration of oligosaccharides such as fructooligosaccharides (FOS) and galactooligosaccharides (GOS) has a limited impact on insulin resistance and glycemic control (Luo et al. Citation2000; Nie et al. Citation2019).
Table 3. Prebiotics and their role in T2D management in post-menopausal women.
Probiotics are live beneficial microbes and their administration usually positively modulates the microbial community and improves the intestinal barrier function and immunity, which supports host health. Evidence from numerous studies have reported that Bifidobacterium, Lactobacillus, Bacillus, Streptococcus, Akkermansia, and Roseburia administration showed beneficial health effects in T2D patients (Gurung et al. Citation2020). Lactobacillus is a lactic acid bacteria (LAB) that commonly alters the microbial flora by reducing the gut pH, which inhibits the growth of opportunistic pathogens. Lactic acid produced by LAB is further cross-fed by SCFA-producing bacteria (Li et al. Citation2017). Bifidobacterium, another LAB, is also a well-known beneficial bacterium that inhibits opportunistic pathogens, produces antioxidants, reduces inflammation, and cross-feeds SCFA producers (Rivière et al. Citation2016). Bifidobacterium also improves glucose levels, endotoxemia, fat accumulation, and insulin resistance (Cani et al. Citation2007; Cano et al. Citation2013; Kikuchi, Ben Othman, and Sakamoto Citation2018). Clinical studies in T2D patients have also found that probiotics are effective in improving the glycemic condition and dyslipidemia (Kocsis et al. Citation2020; Tao et al. Citation2020). The murine T2D model showed that probiotics increases SCFA production, improves gut barrier function, and enhances insulin secretion via GLP-1 secretion (Xu, Wang et al. Citation2020). The co-administration of different Bifidobacterium and Lactobacillus strains significantly improves obesity, insulin resistance, glycemic condition, dyslipidemia, hyperuricemia, pro-inflammatory markers, and LPS levels in post-menopausal obese women (Skrypnik et al. Citation2019; Szulińska et al. Citation2018). summarizes the strain-specific antidiabetic effects of these probiotic strains.
Table 4. Probiotic strains used in previous studies and their roles against T2D (L. = Lactobacillus, B. =Bifidobacterium).
Fermented dairy products such as yogurt and kefir also proven their roles against obesity and T2D. Milk fermentation involves various probiotic Lactobacillus and Streptococcus strains, whose ingestion contributes to maintaining a healthy microbial ecosystem by producing SCFAs, reverting dysbiosis, and limiting harmful microbes by decreasing the gut pH (Fernandez and Marette Citation2018; Marco et al. Citation2017). Moreover, milk fermentation by LAB also increases the conjugated linoleic acid content, which significantly decreases obesity and adipogenesis among post-menopausal T2D patients (Norris et al. Citation2009). The antidiabetic role of fermented milk products is also supported by their ability to reduce colonic pro-inflammatory cytokines and ROS in intestinal enterocytes, and the presence of a high amount of bioactive peptides (Marco et al. Citation2017). Bioactive peptides are derived from the enzymatic digestion of milk proteins by LAB, which is hypothesized to be antidiabetic through regulating the genes participating in glucose uptake, inflammatory responses, and insulin production (Fernandez and Marette Citation2018). Studies on ovariectomized mice have shown that kefir peptide consumption increased gut microbial richness and SCFA-producing bacteria such as Anaerostipes, Ruminococcus, and Streptococcus (Tu et al. Citation2020).
Effects of synbiotic treatment against T2D progression
Synbiotic treatments involve the co-administration of probiotics and prebiotics. Synbiotic treatments often have a better impact than individual treatments. For example, L. acidophilus DSM20079 produced 14.5 times more butyrate in the presence of inulin or pectin than that in their absence (Kim, Keogh, and Clifton Citation2018). Several studies have found that synbiotic administration effectively reduces insulin resistance and plasma lipid profiles in T2D patients (Kim, Keogh, and Clifton Citation2018; Sáez-Lara et al. Citation2016). A previous study on an estrogen-deficient murine model has reported that synbiotic treatments alters the gut microbiota and alleviates dyslipidemia and insulin resistance (Jeong et al. Citation2017). Synbiotic administration also significantly reduced blood glucose levels and insulin resistance in post-menopausal T2D patients (Lee et al. Citation2020; Raji Lahiji et al. Citation2021). Synbiotic treatment of fermented milk containing L. paracasei and inulin enhances the bioavailability of soy-derived isoflavones in post-menopausal women, thus enhancing the conversion of isoflavones into estrogen-like compounds (Timan et al. Citation2014). summarizes the antidiabetic effects of synbiotic treatments.
Table 5. Synbiotic administration used in different T2D studies (L.=Lactobacillus, B.=Bifidobacterium, S. = Streptococcus).
Effects of postbiotic treatment against T2D progression
The postbiotic treatment is a comparatively new strategy in which non-viable microbial products such as SCFAs, functional proteins, extracellular polysaccharides, and cell lysate can be administered without evoking the host-immune response (Chaudhari and Dwivedi Citation2022). Recently, various postbiotic approaches have been successfully used to treat obesity and diabetes, which includes the anti-obesity impact of lipoteichoic acid from Bifidobacterium animalis subsp. lactis BPL1 (Balaguer et al. Citation2022), and insulin-sensitizing role of bacterial cell wall-derived muramyl dipeptide (Cavallari et al. Citation2017). Postbiotic were also found to be effective in controlling the post-menopausal osteoporosis in the murine model (Jang et al. Citation2021; Montazeri-Najafabady et al. Citation2021), but their efficacy against post-menopausal T2D has yet to be tested, as till date there is no information is available regarding it.
Conclusion
Permanent estrogen depletion poses a significant pressure on metabolic regulation and intestinal dysbiosis. As the gut microbiota is a pivotal component that regulates the glucose homeostasis, oxidative stress, and systemic inflammation, it is important to maintain intestinal health and to avoid the vicious spread of T2D among post-menopausal women. To exploit the possible antidiabetic potential of gut microbiota and its regulatory impact on T2D, different dietary interventions can be administered under close medical guidance to ease post-menopausal diabetic conditions.
Contribution
VS and TU conceptualized the manuscript, VS wrote and drafted the manuscript, YP and GL participated in illustration, TU and JS supervised and finalized the final draft of the manuscript.
Declaration of interest statement
The author declares no conflict of interest.
Additional information
Funding
References
- Acharya, K. D., H. L. Noh, M. E. Graham, S. Suk, R. H. Friedline, C. C. Gomez, A. E. R. Parakoyi, J. Chen, J. K. Kim, and M. J. Tetel. 2021. Distinct changes in gut microbiota are associated with estradiol-mediated protection from diet-induced obesity in female mice. Metabolites 11 (8):499. doi: 10.3390/metabo11080499.
- Ahmed, S. B. 2017. Menopause and chronic kidney disease. Seminars in Nephrology 37 (4):404–11. doi: 10.1016/j.semnephrol.2017.05.013.
- Al-Zoairy, R., M. T. Pedrini, M. I. Khan, J. Engl, A. Tschoner, C. Ebenbichler, G. Gstraunthaler, K. Salzmann, R. Bakry, and A. Niederwanger. 2017. Serotonin improves glucose metabolism by serotonylation of the small GTPase Rab4 in L6 skeletal muscle cells. Diabetology & Metabolic Syndrome 9:1. doi: 10.1186/s13098-016-0201-1.
- Alemán, J. O., N. A. Bokulich, J. R. Swann, J. M. Walker, J. C. De Rosa, T. Battaglia, A. Costabile, A. Pechlivanis, Y. Liang, J. L. Breslow, et al. 2018. Fecal microbiota and bile acid interactions with systemic and adipose tissue metabolism in diet-induced weight loss of obese postmenopausal women. Journal of Translational Medicine 16 (1):244.
- Alexander, C., K. S. Swanson, G. C. Fahey, Jr., and K. A. Garleb. 2019. Perspective: Physiologic importance of short-chain fatty acids from nondigestible carbohydrate fermentation. Advances in Nutrition 10 (4):576–89.
- Aliasgharzadeh, A., P. Dehghan, B. P. Gargari, and M. Asghari-Jafarabadi. 2015. Resistant dextrin, as a prebiotic, improves insulin resistance and inflammation in women with type 2 diabetes: A randomised controlled clinical trial. British Journal of Nutrition 113 (2):321–30. doi: 10.1017/S0007114514003675.
- Andreasen, A. S., N. Larsen, T. Pedersen-Skovsgaard, R. M. G. Berg, K. Møller, K. D. Svendsen, M. Jakobsen, and B. K. Pedersen. 2010. Effects of Lactobacillus acidophilus NCFM on insulin sensitivity and the systemic inflammatory response in human subjects. British Journal of Nutrition 104 (12):1831–8. doi: 10.1017/S0007114510002874.
- Angelino, D., E. B. Dosz, J. Sun, J. L. Hoeflinger, M. L. Van Tassell, P. Chen, J. M. Harnly, M. J. Miller, and E. H. Jeffery. 2015. Myrosinase-dependent and –independent formation and control of isothiocyanate products of glucosinolate hydrolysis. Frontiers in Plant Science 6:831. doi: 10.3389/fpls.2015.00831.
- Asemi, Z., S.-A. Alizadeh, K. Ahmad, M. Goli, and A. Esmaillzadeh. 2016. Effects of beta-carotene fortified synbiotic food on metabolic control of patients with type 2 diabetes mellitus: A double-blind randomized cross-over controlled clinical trial. Clinical Nutrition 35 (4):819–25. doi: 10.1016/j.clnu.2015.07.009.
- Asemi, Z., A. Khorrami-Rad, S.-A. Alizadeh, H. Shakeri, and A. Esmaillzadeh. 2014. Effects of synbiotic food consumption on metabolic status of diabetic patients: A double-blind randomized cross-over controlled clinical trial. Clinical Nutrition 33 (2):198–203. doi: 10.1016/j.clnu.2013.05.015.
- Baker, J. M., L. Al-Nakkash, and M. M. Herbst-Kralovetz. 2017. Estrogen-gut microbiome axis: Physiological and clinical implications. Maturitas 103:45–53.
- Balaguer, F., M. Enrique, S. Llopis, M. Barrena, V. Navarro, B. Álvarez, E. Chenoll, D. Ramón, M. Tortajada, and P. Martorell. 2022. Lipoteichoic acid from Bifidobacterium animalis subsp. lactis BPL1: A novel postbiotic that reduces fat deposition via IGF-1 pathway. Microbial Biotechnology 15 (3):805–16. doi: 10.1111/1751-7915.13769.
- Banerjee, M., and M. Saxena. 2012. Interleukin-1 (IL-1) family of cytokines: Role in type 2 diabetes. Clinica Chimica Acta 413 (15-16):1163–70. doi: 10.1016/j.cca.2012.03.021.
- Blachier, F., M. Andriamihaja, P. Larraufie, E. Ahn, A. Lan, and E. Kim. 2021. Production of hydrogen sulfide by the intestinal microbiota and epithelial cells and consequences for the colonic and rectal mucosa. American Journal of Physiology. Gastrointestinal and Liver Physiology 320 (2):G125–35.
- Bourgonje, M. F., A. R. Bourgonje, A. E. Abdulle, L. M. Kieneker, S. la Bastide-van Gemert, R. T. Gansevoort, S. J. L. Bakker, D. J. Mulder, A. Pasch, J. Saleh, et al. 2021. Systemic oxidative stress, aging and the risk of cardiovascular events in the general female population. Frontiers in Cardiovascular Medicine 8:630543. doi: 10.3389/fcvm.2021.630543.
- Brahe, L. K., E. L. Chatelier, E. Prifti, N. Pons, S. Kennedy, T. Blaedel, J. Håkansson, T. K. Dalsgaard, T. Hansen, O. Pedersen, et al. 2015a. Dietary modulation of the gut microbiota—A randomised controlled trial in obese postmenopausal women. British Journal of Nutrition 114 (3):406–17. doi: 10.1017/S0007114515001786.
- Brahe, L. K., E. L. Chatelier, E. Prifti, N. Pons, S. Kennedy, T. Hansen, O. Pedersen, A. Astrup, S. D. Ehrlich, and L. H. Larsen. 2015b. Specific gut microbiota features and metabolic markers in postmenopausal women with obesity. Nutrition & Diabetes 5 (6):e159. doi: 10.1038/nutd.2015.9.
- Braxas, H., M. Rafraf, S. K. Hasanabad, and M. A. Jafarabadi. 2019. Effectiveness of genistein supplementation on metabolic factors and antioxidant status in postmenopausal women with type 2 diabetes mellitus. Canadian Journal of Diabetes 43 (7):490–7. doi: 10.1016/j.jcjd.2019.04.007.
- Bruce-Keller, A. J., A. J. Richard, S.-O. Fernandez-Kim, D. M. Ribnicky, J. M. Salbaum, S. Newman, R. Carmouche, and J. M. Stephens. 2020. Fenugreek counters the effects of high fat diet on gut microbiota in mice. Scientific Reports 10 (1):1245.
- Cani, P. D., A. M. Neyrinck, F. Fava, C. Knauf, R. G. Burcelin, K. M. Tuohy, G. Gibson, and N. M. Delzenne. 2007. Selective increases of bifidobacteria in gut microflora improve high-fat-diet-induced diabetes in mice through a mechanism associated with endotoxaemia. Diabetologia 50 (11):2374–83. doi: 10.1007/s00125-007-0791-0.
- Cano, P. G., A. Santacruz, F. M. Trejo, and Y. Sanz. 2013. Bifidobacterium CECT 7765 improves metabolic and immunological alterations associated with obesity in high-fat diet-fed mice. Obesity 21 (11):2310–21.
- Cardona, F., C. Andrés-Lacueva, S. Tulipani, F. J. Tinahones, and M. I. Queipo-Ortuño. 2013. Benefits of polyphenols on gut microbiota and implications in human health. The Journal of Nutritional Biochemistry 24 (8):1415–22.
- Carlsson, S. 2019. Etiology and pathogenesis of latent autoimmune diabetes in adults (LADA) compared to type 2 diabetes. Frontiers in Physiology 10:320.
- Cavallari, J. F., M. D. Fullerton, B. M. Duggan, K. P. Foley, E. Denou, B. K. Smith, E. M. Desjardins, B. D. Henriksbo, K. J. Kim, B. R. Tuinema, et al. 2017. Muramyl dipeptide-based postbiotics mitigate obesity-induced insulin resistance via IRF4. Cell Metabolism 25 (5):1063–74.e3.
- Ceriello, A., R. Testa, and S. Genovese. 2016. Clinical implications of oxidative stress and potential role of natural antioxidants in diabetic vascular complications. Nutrition, Metabolism, and Cardiovascular Diseases 26 (4):285–92.
- Chambers, E. S., A. Viardot, A. Psichas, D. J. Morrison, K. G. Murphy, S. E. K. Zac-Varghese, K. MacDougall, T. Preston, C. Tedford, G. S. Finlayson, et al. 2015. Effects of targeted delivery of propionate to the human colon on appetite regulation, body weight maintenance and adiposity in overweight adults. Gut 64 (11):1744–54. doi: 10.1136/gutjnl-2014-307913.
- Chaturvedi, U., A. Shrivastava, S. Bhadauria, J. K. Saxena, and G. Bhatia. 2013. A Mechanism-based pharmacological evaluation of efficacy of Trigonella foenum graecum (fenugreek) seeds in regulation of dyslipidemia and oxidative stress in hyperlipidemic rats. Journal of Cardiovascular Pharmacology 61:505–12.
- Chaudhari, A., and M. K. Dwivedi. 2022. Chapter 1—The concept of probiotics, prebiotics, postbiotics, synbiotics, nutribiotics, and pharmabiotics. In Probiotics in the prevention and management of human diseases, ed. M. K. Dwivedi, et al., 1–11. Cambridge, MA: Academic Press.
- Chen, H., and S. Sang. 2014. Biotransformation of tea polyphenols by gut microbiota. Journal of Functional Foods 7:26–42. doi: 10.1016/j.jff.2014.01.013.
- Chen, K. L., and Z. Madak-Erdogan. 2016. Estrogen and microbiota crosstalk: Should we pay attention? Trends in Endocrinology and Metabolism 27 (11):752–5. doi: 10.1016/j.tem.2016.08.001.
- Chen, K. L., and Z. Madak-Erdogan. 2018. Estrogens and female liver health. Steroids 133:38–43.
- Chen, P., Q. Zhang, H. Dang, X. Liu, F. Tian, J. Zhao, Y. Chen, H. Zhang, and W. Chen. 2014. Antidiabetic effect of Lactobacillus casei CCFM0412 on mice with type 2 diabetes induced by a high-fat diet and streptozotocin. Nutrition 30 (9):1061–8. doi: 10.1016/j.nut.2014.03.022.
- Costantini, L., R. Molinari, B. Farinon, and N. Merendino. 2017. Impact of omega-3 fatty acids on the gut microbiota. International Journal of Molecular Sciences 18 (12):2645. doi: 10.3390/ijms18122645.
- Cunningham, A. L., J. W. Stephens, and D. A. Harris. 2021. Gut microbiota influence in type 2 diabetes mellitus (T2DM). Gut Pathogens 13 (1):50.
- Dashti, S. G., J. A. Simpson, A. Karahalios, V. Viallon, M. Moreno-Betancur, L. C. Gurrin, R. J. MacInnis, B. M. Lynch, L. Baglietto, H. A. Morris, et al. 2020. Adiposity and estrogen receptor‐positive, postmenopausal breast cancer risk: Quantification of the mediating effects of fasting insulin and free estradiol. International Journal of Cancer 146 (6):1541–52.
- De Paoli, M., A. Zakharia, and G. H. Werstuck. 2021. The role of estrogen in insulin resistance: A review of clinical and pre-clinical data. The American Journal of Pathology 191 (9):1490–8. doi: 10.1016/j.ajpath.2021.05.011.
- Dehghan, P., B. Pourghassem Gargari, and M. Asghari Jafar-abadi. 2014. Oligofructose-enriched inulin improves some inflammatory markers and metabolic endotoxemia in women with type 2 diabetes mellitus: A randomized controlled clinical trial. Nutrition 30 (4):418–23. doi: 10.1016/j.nut.2013.09.005.
- Delzenne, N. M., P. D. Cani, A. Everard, A. M. Neyrinck, and L. B. Bindels. 2015. Gut microorganisms as promising targets for the management of type 2 diabetes. Diabetologia 58 (10):2206–17.
- Depommier, C., M. Van Hul, A. Everard, N. M. Delzenne, W. M. De Vos, and P. D. Cani. 2020. Pasteurized Akkermansia muciniphila increases whole-body energy expenditure and fecal energy excretion in diet-induced obese mice. Gut Microbes 11 (5):1231–45.
- Di Meo, F., S. Margarucci, U. Galderisi, S. Crispi, and G. Peluso. 2019. Curcumin, gut microbiota, and neuroprotection. Nutrients 11:2426.
- Eastell, R., T. W. O’Neill, L. C. Hofbauer, B. Langdahl, I. R. Reid, D. T. Gold, and S. R. Cummings. 2016. Postmenopausal osteoporosis. Nature Reviews. Disease Primers 2:16069. doi: 10.1038/nrdp.2016.69.
- Everard, A., C. Belzer, L. Geurts, J. P. Ouwerkerk, C. Druart, L. B. Bindels, Y. Guiot, M. Derrien, G. G. Muccioli, and N. M. Delzenne. 2013. Cross-talk between Akkermansia muciniphila and intestinal epithelium controls diet-induced obesity. Proceedings of the National Academy of Sciences of the United States of America 110:9066–71.
- Everard, A., S. Matamoros, L. Geurts, N. M. Delzenne, and P. D. Cani. 2014. Saccharomyces boulardii administration changes gut microbiota and reduces hepatic steatosis, low-grade inflammation, and fat mass in obese and type 2 diabetic db/db mice. mBio 5 (3):e01011–01014. doi: 10.1128/mBio.01011-14.
- Fang, J., H. Wang, Y. Zhou, H. Zhang, H. Zhou, and X. Zhang. 2021. Slimy partners: The mucus barrier and gut microbiome in ulcerative colitis. Experimental & Molecular Medicine 53 (5):772–87. doi: 10.1038/s12276-021-00617-8.
- Fernandez, M. A., and A. Marette. 2018. Novel perspectives on fermented milks and cardiometabolic health with a focus on type 2 diabetes. Nutrition Reviews 76 (Suppl 1):16–28.
- Frampton, J., K. G. Murphy, G. Frost, and E. S. Chambers. 2020. Short-chain fatty acids as potential regulators of skeletal muscle metabolism and function. Nature Metabolism 2 (9):840–8.
- Franzosa, E. A., A. Sirota-Madi, J. Avila-Pacheco, N. Fornelos, H. J. Haiser, S. Reinker, T. Vatanen, A. B. Hall, H. Mallick, L. J. McIver, et al. 2019. Gut microbiome structure and metabolic activity in inflammatory bowel disease. Nature Microbiology 4 (2):293–305. doi: 10.1038/s41564-018-0306-4.
- Fukuda, M. M. Kobayashi, and Y. Honda. 2017. Chapter 6—Functional components and health benefits of fermented soymilk. In Soft chemistry and food fermentation, ed. A. M. Grumezescu and A. M. Holban, 145–78. Cambridge, MA: Academic Press.
- Ge, T., J. Yang, S. Zhou, Y. Wang, Y. Li, and X. Tong. 2020. The role of the pentose phosphate pathway in diabetes and cancer. Frontiers in Endocrinology 11:365. doi: 10.3389/fendo.2020.00365.
- Gobinath, D., A. N. Madhu, G. Prashant, K. Srinivasan, and S. G. Prapulla. 2010. Beneficial effect of xylo-oligosaccharides and fructo-oligosaccharides in streptozotocin-induced diabetic rats. British Journal of Nutrition 104 (1):40–7. doi: 10.1017/S0007114510000243.
- Grassi, F., A. M. Tyagi, J. W. Calvert, L. Gambari, L. D. Walker, M. Yu, J. Robinson, J.-Y. Li, G. Lisignoli, C. Vaccaro, et al. 2016. Hydrogen sulfide is a novel regulator of bone formation implicated in the bone loss induced by estrogen deficiency. Journal of Bone and Mineral Research 31 (5):949–63.
- Greer, R. L., X. Dong, A. C. F. Moraes, R. A. Zielke, G. R. Fernandes, E. Peremyslova, S. Vasquez-Perez, A. A. Schoenborn, E. P. Gomes, A. C. Pereira, et al. 2016. Akkermansia muciniphila mediates negative effects of IFNγ on glucose metabolism. Nature Communications 7:13329.
- Griffin, M. D., T. A. Sanders, I. G. Davies, L. M. Morgan, D. J. Millward, F. Lewis, S. Slaughter, J. A. Cooper, G. J. Miller, and B. A. Griffin. 2006. Effects of altering the ratio of dietary n− 6 to n− 3 fatty acids on insulin sensitivity, lipoprotein size, and postprandial lipemia in men and postmenopausal women aged 45–70 y: The OPTILIP Study. The American Journal of Clinical Nutrition 84 (6):1290–8. doi: 10.1093/ajcn/84.6.1290.
- Guevara-Cruz, M., E. T. Godinez-Salas, M. Sanchez-Tapia, G. Torres-Villalobos, E. Pichardo-Ontiveros, R. Guizar-Heredia, L. Arteaga-Sanchez, G. Gamba, R. Mojica-Espinosa, A. Schcolnik-Cabrera, et al. 2020. Genistein stimulates insulin sensitivity through gut microbiota reshaping and skeletal muscle AMPK activation in obese subjects. BMJ Open Diabetes Research & Care 8 (1):e000948. doi: 10.1136/bmjdrc-2019-000948.
- Guo, T., D. Song, L. Cheng, and X. Zhang. 2019. Interactions of tea catechins with intestinal microbiota and their implication for human health. Food Science and Biotechnology 28 (6):1617–25.
- Gurung, M., Z. Li, H. You, R. Rodrigues, D. B. Jump, A. Morgun, and N. Shulzhenko. 2020. Role of gut microbiota in type 2 diabetes pathophysiology. EBioMedicine 51:102590.
- Hannan, J. M. A., L. Ali, B. Rokeya, J. Khaleque, M. Akhter, P. R. Flatt, and Y. H. A. Abdel-Wahab. 2007. Soluble dietary fibre fraction of Trigonella foenum-graecum (fenugreek) seed improves glucose homeostasis in animal models of type 1 and type 2 diabetes by delaying carbohydrate digestion and absorption, and enhancing insulin action. British Journal of Nutrition 97 (3):514–21. doi: 10.1017/S0007114507657869.
- Heianza, Y., Y. Arase, S. Kodama, S. D. Hsieh, H. Tsuji, K. Saito, H. Shimano, S. Hara, and H. Sone. 2013. Effect of postmenopausal status and age at menopause on type 2 diabetes and prediabetes in Japanese individuals: Toranomon Hospital Health Management Center Study 17 (TOPICS 17). Diabetes Care 36 (12):4007–14. doi: 10.2337/dc13-1048.
- Hevener, A. L., V. Ribas, T. M. Moore, and Z. Zhou. 2020. The impact of skeletal muscle ERα on mitochondrial function and metabolic health. Endocrinology 161 (2):1–16. doi: 10.1210/endocr/bqz017.
- Hope, S. 2016. The NICE menopause guidelines. Maturitas 85:71–2.
- Hsieh, M.-C., W.-H. Tsai, Y.-P. Jheng, S.-L. Su, S.-Y. Wang, C.-C. Lin, Y.-H. Chen, and W.-W. Chang. 2018. The beneficial effects of Lactobacillus reuteri ADR-1 or ADR-3 consumption on type 2 diabetes mellitus: A randomized, double-blinded, placebo-controlled trial. Scientific Reports 8 (1):16791. doi: 10.1038/s41598-018-35014-1.
- Hsieh, P.-S., H.-H. Ho, S.-H. Hsieh, Y.-W. Kuo, H.-Y. Tseng, H.-F. Kao, and J.-Y. Wang. 2020. Lactobacillus salivarius AP-32 and Lactobacillus reuteri GL-104 decrease glycemic levels and attenuate diabetes-mediated liver and kidney injury in db/db mice. BMJ Open Diabetes Research & Care 8 (1):e001028. doi: 10.1136/bmjdrc-2019-001028.
- Janeiro, M. H., M. J. Ramírez, F. I. Milagro, J. A. Martínez, and M. Solas. 2018. Implication of trimethylamine N-oxide (TMAO) in disease: Potential biomarker or new therapeutic target. Nutrients 10 (10):1398. doi: 10.3390/nu10101398.
- Jang, A.-R., J.-S. Park, D.-K. Kim, J.-Y. Park, J.-H. Ahn, D.-Y. Kim, T.-S. Lee, J.-Y. Chang, J.-H. Choi, and J.-H. Park. 2021. Cell-free culture supernatant of Lactobacillus curvatus Wikim38 inhibits RANKL-induced osteoclast differentiation and ameliorates bone loss in ovariectomized mice. Letters in Applied Microbiology 73 (3):383–91. doi: 10.1111/lam.13525.
- Jang, H. R., and H.-Y. Lee. 2021. Mechanisms linking gut microbial metabolites to insulin resistance. World Journal of Diabetes 12 (6):730–44.
- Jeon, S.-M. 2016. Regulation and function of AMPK in physiology and diseases. Experimental & Molecular Medicine 48 (7):e245. doi: 10.1038/emm.2016.81.
- Jeong, S.-Y., S. Kang, C. S. Hua, Z. Ting, and S. Park. 2017. Synbiotic effects of β-glucans from cauliflower mushroom and Lactobacillus fermentum on metabolic changes and gut microbiome in estrogen-deficient rats. Genes & Nutrition 12:31. doi: 10.1186/s12263-017-0585-z.
- Jia, L., D. Li, N. Feng, M. Shamoon, Z. Sun, L. Ding, H. Zhang, W. Chen, J. Sun, and Y. Q. Chen. 2017. Anti-diabetic effects of Clostridium butyricum CGMCC0313.1 through promoting the growth of gut butyrate-producing bacteria in type 2 diabetic mice. Scientific Reports 7:1–15.
- Joon Cho, G., J. Hyun Lee, H. Tae Park, J. Ho Shin, S. Cheol Hong, T. Kim, J. Young Hur, K. Wan Lee, Y. Kyun Park, and S. Haeng Kim. 2008. Postmenopausal status according to years since menopause as an independent risk factor for the metabolic syndrome. Menopause 15 (3):524–9. doi: 10.1097/gme.0b013e3181559860.
- Kalaycıoğlu, Z., I. Gazioğlu, and F. B. Erim. 2017. Comparison of antioxidant, anticholinesterase, and antidiabetic activities of three curcuminoids isolated from Curcuma longa L. Natural Product Research 31 (24):2914–7.
- Kaliannan, K., R. C. Robertson, K. Murphy, C. Stanton, C. Kang, B. Wang, L. Hao, A. K. Bhan, and J. X. Kang. 2018. Estrogen-mediated gut microbiome alterations influence sexual dimorphism in metabolic syndrome in mice. Microbiome 6 (1):205. doi: 10.1186/s40168-018-0587-0.
- Kanmani, S., M. Kwon, M.-K. Shin, and M. K. Kim. 2019. Association of C-reactive protein with risk of developing type 2 diabetes mellitus, and role of obesity and hypertension: A large population-based Korean cohort study. Scientific Reports 9 (1):1–8. doi: 10.1038/s41598-019-40987-8.
- Kay, B. A., K. Trigatti, M. B. MacNeil, S. L. Klingel, N. Repin, H. D. Goff, A. J. Wright, and A. M. Duncan. 2017. Pudding products enriched with yellow mustard mucilage, fenugreek gum or flaxseed mucilage and matched for simulated intestinal viscosity significantly reduce postprandial peak glucose and insulin in adults at risk for type 2 diabetes. Journal of Functional Foods 37:603–11. doi: 10.1016/j.jff.2017.08.017.
- Kikuchi, K., M. Ben Othman, and K. Sakamoto. 2018. Sterilized bifidobacteria suppressed fat accumulation and blood glucose level. Biochemical and Biophysical Research Communications 501 (4):1041–7.
- Kim, S.-H., C.-S. Huh, I.-D. Choi, J.-W. Jeong, H.-K. Ku, J.-H. Ra, T.-Y. Kim, G.-B. Kim, J.-H. Sim, and Y.-T. Ahn. 2014. The anti-diabetic activity of Bifidobacterium lactis HY8101 in vitro and in vivo. Journal of Applied Microbiology 117 (3):834–45.
- Kim, Y. A., J. B. Keogh, and P. M. Clifton. 2018. Probiotics, prebiotics, synbiotics and insulin sensitivity. Nutrition Research Reviews 31 (1):35–51.
- Ko, S.-H., and H.-S. Kim. 2020. Menopause-associated lipid metabolic disorders and foods beneficial for postmenopausal women. Nutrients 12 (1):202. doi: 10.3390/nu12010202.
- Kocsis, T., B. Molnár, D. Németh, P. Hegyi, Z. Szakács, A. Bálint, A. Garami, A. Soós, K. Márta, and M. Solymár. 2020. Probiotics have beneficial metabolic effects in patients with type 2 diabetes mellitus: A meta-analysis of randomized clinical trials. Scientific Reports 10 (1):11787. doi: 10.1038/s41598-020-68440-1.
- Kommoju, U. J., and B. M. Reddy. 2011. Genetic etiology of type 2 diabetes mellitus: A review. International Journal of Diabetes in Developing Countries 31 (2):51–64. doi: 10.1007/s13410-011-0020-8.
- Koye, D. N., D. J. Magliano, R. G. Nelson, and M. E. Pavkov. 2018. The global epidemiology of diabetes and kidney disease. Advances in Chronic Kidney Disease 25 (2):121–32.
- Kumar, G. S., A. Shetty, and P. Salimath. 2005. Modulatory effect of fenugreek seed mucilage and spent turmeric on intestinal and renal disaccharidases in streptozotocin induced diabetic rats. Plant Foods for Human Nutrition 60 (2):87–91.
- Kwa, M., C. S. Plottel, M. J. Blaser, and S. Adams. 2016. The intestinal microbiome and estrogen receptor–positive female breast cancer. JNCI: Journal of the National Cancer Institute 108 (8):djw029.
- Lee, H., G. Ko, and M. W. Griffiths. 2014. Effect of metformin on metabolic improvement and gut microbiota. Applied and Environmental Microbiology 80 (19):5935–43.
- Lee, S. H., H. S. You, H.-G. Kang, S. S. Kang, and S. H. Hyun. 2020. Association between altered blood parameters and gut microbiota after synbiotic intake in healthy. Elderly Korean Women. Nutrients 12:3112.
- Legry, V., S. Francque, J. T. Haas, A. Verrijken, S. Caron, O. Chávez-Talavera, E. Vallez, L. Vonghia, E. Dirinck, A. Verhaegen, et al. 2017. Bile acid alterations are associated with insulin resistance, but not with NASH, in obese subjects. The Journal of Clinical Endocrinology & Metabolism 102 (10):3783–94. doi: 10.1210/jc.2017-01397.
- Lei, Z., H. Wu, Y. Yang, Q. Hu, Y. Lei, W. Liu, Y. Nie, L. Yang, X. Zhang, C. Yang, et al. 2021. Ovariectomy impaired hepatic glucose and lipid homeostasis and altered the gut microbiota in mice with different diets. Frontiers in Endocrinology 12:708838. doi: 10.3389/fendo.2021.708838.
- Lekshmi, P. C., R. Arimboor, K. G. Raghu, and A. N. Menon. 2012. Turmerin, the antioxidant protein from turmeric (Curcuma longa) exhibits antihyperglycaemic effects. Natural Product Research 26 (17):1654–8.
- Lett, A. M., P. S. Thondre, and A. J. Rosenthal. 2013. Yellow mustard bran attenuates glycaemic response of a semi-solid food in young healthy men. International Journal of Food Sciences and Nutrition 64 (2):140–6. doi: 10.3109/09637486.2012.728201.
- Li, K.-K., P.-J. Tian, S.-D. Wang, P. Lei, L. Qu, J.-P. Huang, Y.-J. Shan, and B-l. Li. 2017. Targeting gut microbiota: Lactobacillus alleviated type 2 diabetes via inhibiting LPS secretion and activating GPR43 pathway. Journal of Functional Foods 38:561–70. doi: 10.1016/j.jff.2017.09.049.
- Liang, H., X. Chen, Q. Chen, Y. Wang, X. Wu, Y. Li, B. Pan, H. Liu, and M. Li. 2013. The metabolic syndrome among postmenopausal women in rural Canton: Prevalence, associated factors, and the optimal obesity and atherogenic indices. PLoS One 8 (9):e74121. doi: 10.1371/journal.pone.0074121.
- Liou, C. S., S. J. Sirk, C. A. C. Diaz, A. P. Klein, C. R. Fischer, S. K. Higginbottom, A. Erez, M. S. Donia, J. L. Sonnenburg, and E. S. Sattely. 2020. A metabolic pathway for activation of dietary glucosinolates by a human gut symbiont. Cell 180 (4):717–28.e19. doi: 10.1016/j.cell.2020.01.023.
- Lisabeth, L., and C. Bushnell. 2012. Stroke risk in women: The role of menopause and hormone therapy. The Lancet. Neurology 11 (1):82–91.
- Liu, G., J. Bei, L. Liang, G. Yu, L. Li, and Q. Li. 2018. Stachyose improves inflammation through modulating gut microbiota of high-fat diet/streptozotocin-induced type 2 diabetes in rats. Molecular Nutrition & Food Research 62 (6):1700954. doi: 10.1002/mnfr.201700954.
- Liu, Y., Y.-L. Guo, S. Meng, H. Gao, L.-J. Sui, S. Jin, Y. Li, and S.-G. Fan. 2020. Gut microbiota-dependent Trimethylamine N-Oxide are related with hip fracture in postmenopausal women: A matched case-control study. Aging 12 (11):10633–41. doi: 10.18632/aging.103283.
- Lobo, R. A., S. R. Davis, T. J. De Villiers, A. Gompel, V. W. Henderson, H. N. Hodis, M. A. Lumsden, W. J. Mack, S. Shapiro, and R. J. Baber. 2014. Prevention of diseases after menopause. Climacteric 17 (5):540–56. doi: 10.3109/13697137.2014.933411.
- Louis, P., and H. J. Flint. 2017. Formation of propionate and butyrate by the human colonic microbiota. Environmental Microbiology 19 (1):29–41. doi: 10.1111/1462-2920.13589.
- Lucas, S., Y. Omata, J. Hofmann, M. Böttcher, A. Iljazovic, K. Sarter, O. Albrecht, O. Schulz, B. Krishnacoumar, G. Krönke, et al. 2018. Short-chain fatty acids regulate systemic bone mass and protect from pathological bone loss. Nature Communications 9 (1):55.
- Lukovac, S., C. Belzer, L. Pellis, B. J. Keijser, W. M. de Vos, R. C. Montijn, and G. Roeselers. 2014. Differential modulation by Akkermansia muciniphila and Faecalibacterium prausnitzii of host peripheral lipid metabolism and histone acetylation in mouse gut organoids. mBio 5 (4):e01438-14. doi: 10.1128/mBio.01438-14.
- Luo, J., Y. Li, Y. Mai, L. Gao, S. Ou, Y. Wang, L. Liu, and X. Peng. 2018. Flaxseed gum reduces body weight by regulating gut microbiota. Journal of Functional Foods 47:136–42. doi: 10.1016/j.jff.2018.05.042.
- Luo, J., M. Van Yperselle, S. W. Rizkalla, F. Rossi, F. R. J. Bornet, and G. Slama. 2000. Chronic consumption of short-chain fructooligosaccharides does not affect basal hepatic glucose production or insulin resistance in type 2 diabetics. The Journal of Nutrition 130 (6):1572–7. doi: 10.1093/jn/130.6.1572.
- Ma, H.-r., J. Wang, H.-x. Qi, Y.-h. Gao, L.-j. Pang, Y. Yang, Z.-h. Wang, M.-j. Duan, H. Chen, X. Cao, et al. 2013. Assessment of the estrogenic activities of chickpea (Cicer arietinum L) sprout isoflavone extract in ovariectomized rats. Acta Pharmacologica Sinica 34 (3):380–6.
- Marchand, G. B., A.-M. Carreau, S. J. Weisnagel, J. Bergeron, F. Labrie, S. Lemieux, and A. Tchernof. 2018. Increased body fat mass explains the positive association between circulating estradiol and insulin resistance in postmenopausal women. American Journal of Physiology-Endocrinology and Metabolism 314 (5):E448–E456. doi: 10.1152/ajpendo.00293.2017.
- Marco, M. L., D. Heeney, S. Binda, C. J. Cifelli, P. D. Cotter, B. Foligné, M. Gänzle, R. Kort, G. Pasin, A. Pihlanto, et al. 2017. Health benefits of fermented foods: Microbiota and beyond. Current Opinion in Biotechnology 44:94–102.
- Martin, A. M., J. M. Yabut, J. M. Choo, A. J. Page, E. W. Sun, C. F. Jessup, S. L. Wesselingh, W. I. Khan, G. B. Rogers, G. R. Steinberg, et al. 2019. The gut microbiome regulates host glucose homeostasis via peripheral serotonin. Proceedings of the National Academy of Sciences of the United States of America 116:19802.
- Masala, G., B. Bendinelli, C. D. Bella, M. Assedi, S. Tapinassi, I. Ermini, D. Occhini, M. Castaldo, C. Saieva, S. Caini, et al. 2020. Inflammatory marker changes in a 24-month dietary and physical activity randomised intervention trial in postmenopausal women. Scientific Reports 10 (1):21845. doi: 10.1038/s41598-020-78796-z.
- Mauvais-Jarvis, F., J. E. Manson, J. C. Stevenson, and V. A. Fonseca. 2017. Menopausal hormone therapy and type 2 diabetes prevention: Evidence, mechanisms, and clinical implications. Endocrine Reviews 38 (3):173–88.
- Mayneris-Perxachs, J., M. Arnoriaga-Rodríguez, D. Luque-Córdoba, F. Priego-Capote, V. Pérez-Brocal, A. Moya, A. Burokas, R. Maldonado, and J.-M. Fernández-Real. 2020. Gut microbiota steroid sexual dimorphism and its impact on gonadal steroids: Influences of obesity and menopausal status. Microbiome 8 (1):136. doi: 10.1186/s40168-020-00913-x.
- Mirmiranpour, H., H. F. Huseini, H. Derakhshanian, Z. Khodaii, and B. Tavakoli-Far. 2020. Effects of probiotic, cinnamon, and synbiotic supplementation on glycemic control and antioxidant status in people with type 2 diabetes; a randomized, double-blind, placebo-controlled study. Journal of Diabetes and Metabolic Disorders 19 (1):53–60.
- Molehin, O. R. S. A. Adefegha, and A. A. Adeyanju. 2020. Role of oxidative stress in the pathophysiology of type 2 diabetes and cardiovascular diseases. In Role of oxidative stress in pathophysiology of diseases, ed. P. K. Maurya and K. Dua, 277–97. Singapore: Springer.
- Molinaro, A., A. Wahlström, and H.-U. Marschall. 2018. Role of bile acids in metabolic control. Trends in Endocrinology and Metabolism 29 (1):31–41. doi: 10.1016/j.tem.2017.11.002.
- Montazeri-Najafabady, N., Y. Ghasemi, M. H. Dabbaghmanesh, Y. Ashoori, P. Talezadeh, F. Koohpeyma, S. N. Abootalebi, and A. Gholami. 2021. Exploring the bone sparing effects of postbiotics in the post-menopausal rat model. BMC Complementary Medicine and Therapies 21 (1):155.
- Moroti, C., L. F. Souza Magri, M. de Rezende Costa, D. C. U. Cavallini, and K. Sivieri. 2012. Effect of the consumption of a new symbiotic shake on glycemia and cholesterol levels in elderly people with type 2 diabetes mellitus. Lipids in Health and Disease 11 (1):29. doi: 10.1186/1476-511X-11-29.
- Moulton, V. R. 2018. Sex hormones in acquired immunity and autoimmune disease. Frontiers in Immunology 9:2279. doi: 10.3389/fimmu.2018.02279.
- Mtintsilana, A., L. K. Micklesfield, E. Chorell, T. Olsson, and J. H. Goedecke. 2019. Fat redistribution and accumulation of visceral adipose tissue predicts type 2 diabetes risk in middle-aged black South African women: A 13-year longitudinal study. Nutrition & Diabetes 9 (1):12. doi: 10.1038/s41387-019-0079-8.
- Navarro del Hierro, J., C. Cueva, A. Tamargo, E. Núñez-Gómez, M. V. Moreno-Arribas, G. Reglero, and D. Martin. 2020. In vitro colonic fermentation of saponin-rich extracts from quinoa, lentil, and fenugreek. Effect on sapogenins yield and human gut microbiota. Journal of Agricultural and Food Chemistry 68 (1):106–16.
- Nie, Q., H. Chen, J. Hu, S. Fan, and S. Nie. 2019. Dietary compounds and traditional Chinese medicine ameliorate type 2 diabetes by modulating gut microbiota. Critical Reviews in Food Science and Nutrition 59 (6):848–63.
- Niness, K. R. 1999. Inulin and oligofructose: What are they? The Journal of Nutrition 129 (7):1402S–6S. doi: 10.1093/jn/129.7.1402S.
- Norris, L. E., A. L. Collene, M. L. Asp, J. C. Hsu, L.-F. Liu, J. R. Richardson, D. Li, D. Bell, K. Osei, R. D. Jackson, et al. 2009. Comparison of dietary conjugated linoleic acid with safflower oil on body composition in obese postmenopausal women with type 2 diabetes mellitus. The American Journal of Clinical Nutrition 90 (3):468–76.
- Ozaki, D., R. Kubota, T. Maeno, M. Abdelhakim, and N. Hitosugi. 2021. Association between gut microbiota, bone metabolism, and fracture risk in postmenopausal Japanese women. Osteoporosis International 32 (1):145–56.
- Petrine, J. C. P., and B. D. Bianco-Borges. 2021. The influence of phytoestrogens on different physiological and pathological processes: An overview. Phytotherapy Research 35 (1):180–97.
- Pivari, F., A. Mingione, C. Brasacchio, and L. Soldati. 2019. Curcumin and type 2 diabetes mellitus: Prevention and treatment. Nutrients 11 (8):1837. doi: 10.3390/nu11081837.
- Pu, D., R. Tan, Q. Yu, and J. Wu. 2017. Metabolic syndrome in menopause and associated factors: A meta-analysis. Climacteric 20 (6):583–91.
- Qureshi, R., M. Picon-Ruiz, I. Aurrekoetxea-Rodriguez, V. Nunes de Paiva, M. D’Amico, H. Yoon, R. Radhakrishnan, C. Morata-Tarifa, T. Ince, M. E. Lippman, et al. 2020. The major pre- and postmenopausal estrogens play opposing roles in obesity-driven mammary inflammation and breast cancer development. Cell Metabolism 31 (6):1154–72.e9. doi: 10.1016/j.cmet.2020.05.008.
- Raji Lahiji, M., S. Najafi, L. Janani, B. Yazdani, E. Razmpoosh, and M. Zarrati. 2021. The effect of synbiotic on glycemic profile and sex hormones in overweight and obese breast cancer survivors following a weight-loss diet: A randomized, triple-blind, controlled trial. Clinical Nutrition 40 (2):394–403. doi: 10.1016/j.clnu.2020.05.043.
- Ren, Y., M. Zhang, Y. Liu, X. Sun, B. Wang, Y. Zhao, D. Liu, X. Liu, D. Zhang, F. Liu, et al. 2019. Association of menopause and type 2 diabetes mellitus. Menopause 26 (3):325–30.
- Rettedal, E. A., B. L. Ilesanmi-Oyelere, N. C. Roy, J. Coad, and M. C. Kruger. 2021. The gut microbiome is altered in postmenopausal women with osteoporosis and osteopenia. JBMR Plus 5 (3):e10452.
- Ridlon, J. M., D. J. Kang, P. B. Hylemon, and J. S. Bajaj. 2014. Bile acids and the gut microbiome. Current Opinion in Gastroenterology 30 (3):332–8.
- Rios-Covian, D., N. Salazar, M. Gueimonde, and C. G. de los Reyes-Gavilan. 2017. Shaping the metabolism of intestinal bacteroides population through diet to improve human health. Frontiers in Microbiology 8:376. doi: 10.3389/fmicb.2017.00376.
- Rivière, A., M. Selak, D. Lantin, F. Leroy, and L. D. Vuyst. 2016. Bifidobacteria and butyrate-producing colon bacteria: Importance and strategies for their stimulation in the human gut. Frontiers in Microbiology 7:979. doi: 10.3389/fmicb.2016.00979.
- Roberts, K. T. 2011. The potential of fenugreek (Trigonella foenum-graecum) as a functional food and nutraceutical and its effects on glycemia and lipidemia. Journal of Medicinal Food 14 (12):1485–9.
- Rosano, G. M. C., C. Vitale, G. Marazzi, and M. Volterrani. 2007. Menopause and cardiovascular disease: The evidence. Climacteric 10 (sup1):19–24. doi: 10.1080/13697130601114917.
- Sáez-Lara, M. J., C. Robles-Sanchez, F. J. Ruiz-Ojeda, J. Plaza-Diaz, and A. Gil. 2016. Effects of probiotics and synbiotics on obesity, insulin resistance syndrome, type 2 diabetes and non-alcoholic fatty liver disease: A review of human clinical trials. International Journal of Molecular Sciences 17 (6):928. doi: 10.3390/ijms17060928.
- Sanborn, V. E., M. A. Azcarate-Peril, and J. Gunstad. 2020. Lactobacillus rhamnosus GG and HbA1c in middle age and older adults without type 2 diabetes mellitus: A preliminary randomized study. Diabetes & Metabolic Syndrome 14 (5):907–9.
- Santos-Marcos, J. A., O. A. Rangel-Zuñiga, R. Jimenez-Lucena, G. M. Quintana-Navarro, S. Garcia-Carpintero, M. M. Malagon, B. B. Landa, M. Tena-Sempere, P. Perez-Martinez, J. Lopez-Miranda, et al. 2018. Influence of gender and menopausal status on gut microbiota. Maturitas 116:43–53.
- Schreurs, M. P. H., P. J. de Vos van Steenwijk, A. Romano, S. Dieleman, and H. M. J. Werner. 2021. How the gut microbiome links to menopause and obesity, with possible implications for endometrial cancer development. Journal of Clinical Medicine 10 (13):2916. doi: 10.3390/jcm10132916.
- Shafi, A., H. Naeem Raja, U. Farooq, K. Akram, Z. Hayat, A. Naz, and H. R. Nadeem. 2019. Antimicrobial and antidiabetic potential of synbiotic fermented milk: A functional dairy product. International Journal of Dairy Technology 72 (1):15–22. doi: 10.1111/1471-0307.12555.
- Shakeri, H., H. Hadaegh, F. Abedi, M. Tajabadi-Ebrahimi, N. Mazroii, Y. Ghandi, and Z. Asemi. 2014. Consumption of synbiotic bread decreases triacylglycerol and VLDL levels while increasing HDL levels in serum from patients with type-2 diabetes. Lipids 49 (7):695–701.
- Shieh, A., M. Epeldegui, A. S. Karlamangla, and G. A. Greendale. 2020. Gut permeability, inflammation, and bone density across the menopause transition. JCI Insight 5 (2):e134092. doi: 10.1172/jci.insight.134092.
- Shoff, S. M., P. A. Newcomb, J. A. Mares‐Perlman, B. E. K. Klein, S. M. Haffner, B. E. Storer, and R. Klein. 1998. Usual consumption of plant foods containing phytoestrogens and sex hormone levels in postmenopausal women in Wisconsin. Nutrition and Cancer 30 (3):207–12.
- Shtriker, M. G., M. Hahn, E. Taieb, A. Nyska, U. Moallem, O. Tirosh, and Z. Madar. 2018. Fenugreek galactomannan and citrus pectin improve several parameters associated with glucose metabolism and modulate gut microbiota in mice. Nutrition 46:134–42.e3. doi: 10.1016/j.nut.2017.07.012.
- Skrypnik, K., P. Bogdański, M. Sobieska, and J. Suliburska. 2019. The effect of multistrain probiotic supplementation in two doses on iron metabolism in obese postmenopausal women: A randomized trial. Food & Function 10 (8):5228–38.
- Soltanian, N., and M. Janghorbani. 2018. A randomized trial of the effects of flaxseed to manage constipation, weight, glycemia, and lipids in constipated patients with type 2 diabetes. Nutrition & Metabolism 15 (1):1–9. doi: 10.1186/s12986-018-0273-z.
- Song, Y., N. R. Cook, C. M. Albert, M. Van Denburgh, and J. E. Manson. 2009. Effects of vitamins C and E and β-carotene on the risk of type 2 diabetes in women at high risk of cardiovascular disease: A randomized controlled trial. The American Journal of Clinical Nutrition 90 (2):429–37. doi: 10.3945/ajcn.2009.27491.
- Soukoulis, C., C. Gaiani, and L. Hoffmann. 2018. Plant seed mucilage as emerging biopolymer in food industry applications. Current Opinion in Food Science 22:28–42. doi: 10.1016/j.cofs.2018.01.004.
- Sowbhagya, H. 2019. Value-added processing of by-products from spice industry. Food Quality and Safety 3 (2):73–80. doi: 10.1093/fqsafe/fyy029.
- Spranger, J., A. Kroke, M. Möhlig, K. Hoffmann, M. M. Bergmann, M. Ristow, H. Boeing, and A. F. H. Pfeiffer. 2003. Inflammatory cytokines and the risk to develop type 2 diabetes: Results of the prospective population-based European Prospective Investigation into Cancer and Nutrition (EPIC)-Potsdam study. Diabetes 52 (3):812–7. doi: 10.2337/diabetes.52.3.812.
- Stachowiak, G., T. Pertyński, and M. Pertyńska-Marczewska. 2015. Metabolic disorders in menopause. Przeglad Menopauzalny = Menopause Review 14 (1):59–64.
- Stojanov, S., and S. Kreft. 2020. Gut microbiota and the metabolism of phytoestrogens. Revista Brasileira de Farmacognosia 30 (2):145–54. doi: 10.1007/s43450-020-00049-x.
- Straub, R. H. 2007. The complex role of estrogens in inflammation. Endocrine Reviews 28 (5):521–74.
- Szulińska, M., I. Łoniewski, S. Van Hemert, M. Sobieska, and P. Bogdański. 2018. Dose-dependent effects of multispecies probiotic supplementation on the lipopolysaccharide (LPS) level and cardiometabolic profile in obese postmenopausal women: A 12-week randomized clinical trial. Nutrients 10 (6):773. doi: 10.3390/nu10060773.
- Takahashi, M., M. Miyashita, K. Suzuki, S-r. Bae, H.-K. Kim, T. Wakisaka, Y. Matsui, M. Takeshita, and K. Yasunaga. 2014. Acute ingestion of catechin-rich green tea improves postprandial glucose status and increases serum thioredoxin concentrations in postmenopausal women. British Journal of Nutrition 112 (9):1542–50. doi: 10.1017/S0007114514002530.
- Tamargo, A., C. Cueva, L. Laguna, M. V. Moreno-Arribas, and L. A. Muñoz. 2018. Understanding the impact of chia seed mucilage on human gut microbiota by using the dynamic gastrointestinal model simgi®. Journal of Functional Foods 50:104–11. doi: 10.1016/j.jff.2018.09.028.
- Tao, Y.-W., Y.-L. Gu, X.-Q. Mao, L. Zhang, and Y.-F. Pei. 2020. Effects of probiotics on type II diabetes mellitus: A meta-analysis. Journal of Translational Medicine 18 (1):30. doi: 10.1186/s12967-020-02213-2.
- Tardivo, A. P., J. Nahas-Neto, C. L. Orsatti, F. B. Dias, P. F. Poloni, E. B. Schmitt, and E. A. P. Nahas. 2015. Effects of omega-3 on metabolic markers in postmenopausal women with metabolic syndrome. Climacteric 18 (2):290–8. doi: 10.3109/13697137.2014.981521.
- Tavakoly, R., M. R. Maracy, M. Karimifar, and M. H. Entezari. 2018. Does fenugreek (Trigonella foenum-graecum) seed improve inflammation, and oxidative stress in patients with type 2 diabetes mellitus? A parallel group randomized clinical trial. European Journal of Integrative Medicine 18:13–7. doi: 10.1016/j.eujim.2018.01.005.
- Taylor, D. R., J. Alaghband-Zadeh, G. F. Cross, S. Omar, C. W. Le Roux, and R. P. Vincent. 2014. Urine bile acids relate to glucose control in patients with type 2 diabetes mellitus and a body mass index below 30 kg/m2. PLoS One. 9 (4):e93540. doi: 10.1371/journal.pone.0093540.
- Taylor, R. 2013. Type 2 diabetes: Etiology and reversibility. Diabetes Care 36 (4):1047–55.
- Timan, P., N. Rojanasthien, M. Manorot, C. Sangdee, and S. Teekachunhatean. 2014. Effect of synbiotic fermented milk on oral bioavailability of isoflavones in postmenopausal women. International Journal of Food Sciences and Nutrition 65 (6):761–7.
- Tu, M.-Y. K.-Y. Han, G. R. Chang, G.-D. Lai, K.-Y. Chang, C.-F. Chen, J.-C. Lai, C.-Y. Lai, H.-L. Chen, and C.-M. Chen. 2020. Kefir peptides prevent estrogen deficiency-induced bone loss and modulate the structure of the gut microbiota in ovariectomized mice. Nutrients 12 (11):3432. doi: 10.3390/nu12113432.
- Valdes, A. M., J. Walter, E. Segal, and T. D. Spector. 2018. Role of the gut microbiota in nutrition and health. BMJ 361:k2179.
- Velayati, A., I. Kareem, M. Sedaghat, G. Sohrab, O. Nikpayam, M. Hedayati, K. Abhari, and E. Hejazi. 2021. Does symbiotic supplementation which contains Bacillus coagulans Lactobacillus rhamnosus, Lactobacillus acidophilus and fructooligosaccharide has favourite effects in patients with type-2 diabetes? A randomised, double-blind, placebo-controlled trial. Archives of Physiology and Biochemistry 1–8. doi: 10.1080/13813455.2021.1928225.
- Vieira, A. T., P. M. Castelo, D. A. Ribeiro, and C. M. Ferreira. 2017. Influence of oral and gut microbiota in the health of menopausal women. Frontiers in Microbiology 8:1884. doi: 10.3389/fmicb.2017.01884.
- Wan, M. L. Y., V. A. Co, and H. El-Nezami. 2021. Dietary polyphenol impact on gut health and microbiota. Critical Reviews in Food Science and Nutrition 61 (4):690–711.
- Wang, G., X. Li, J. Zhao, H. Zhang, and W. Chen. 2017. Lactobacillus casei CCFM419 attenuates type 2 diabetes via a gut microbiota dependent mechanism. Food & Function 8 (9):3155–64. doi: 10.1039/C7FO00593H.
- Wang, J., W. Xu, R. Wang, R. Cheng, Z. Tang, and M. Zhang. 2021. The outer membrane protein Amuc_1100 of Akkermansia muciniphila promotes intestinal 5-HT biosynthesis and extracellular availability through TLR2 signalling. Food & Function 12 (8):3597–610.
- Wang, L., X. Yu, X. Xu, J. Ming, Z. Wang, B. Gao, Y. Xing, J. Zhou, J. Fu, T. Liu, et al. 2021. The fecal microbiota is already altered in normoglycemic individuals who go on to have type 2 diabetes. Frontiers in Cellular and Infection Microbiology 11:598672. doi: 10.3389/fcimb.2021.598672.
- Wang, M., F. Gorelick, and A. Bhargava. 2021. Sex differences in the exocrine pancreas and associated diseases. Cellular and Molecular Gastroenterology and Hepatology 12 (2):427–41. doi: 10.1016/j.jcmgh.2021.04.005.
- Wang, S., Y. Xiao, F. Tian, J. Zhao, H. Zhang, Q. Zhai, and W. Chen. 2020. Rational use of prebiotics for gut microbiota alterations: Specific bacterial phylotypes and related mechanisms. Journal of Functional Foods 66:103838. doi: 10.1016/j.jff.2020.103838.
- Watanabe, H., M. T. Rose, and H. Aso. 2011. Role of peripheral serotonin in glucose and lipid metabolism. Current Opinion in Lipidology 22 (3):186–91.
- Won, G., S.-I. Choi, N. Park, J.-E. Kim, C.-H. Kang, and G.-H. Kim. 2021. In vitro antidiabetic, antioxidant activity, and probiotic activities of Lactiplantibacillus plantarum and Lacticaseibacillus paracasei strains. Current Microbiology 78 (8):3181–91.
- Wright, E., Jr., J. Scism‐Bacon, and L. Glass. 2006. Oxidative stress in type 2 diabetes: The role of fasting and postprandial glycaemia. International Journal of Clinical Practice 60 (3):308–14.
- Wu, Y., D. Hui, N. A. M. Eskin, and S. W. Cui. 2016. Water-soluble yellow mustard mucilage: A novel ingredient with potent antioxidant properties. International Journal of Biological Macromolecules 91:710–5. doi: 10.1016/j.ijbiomac.2016.05.088.
- Xu, B., C. Allard, A. I. Alvarez-Mercado, T. Fuselier, J. H. Kim, L. A. Coons, S. C. Hewitt, F. Urano, K. S. Korach, E. R. Levin, et al. 2018. Estrogens promote misfolded proinsulin degradation to protect insulin production and delay diabetes. Cell Reports 24 (1):181–96.
- Xu, X., X. Jia, L. Mo, C. Liu, L. Zheng, Q. Yuan, and X. Zhou. 2017. Intestinal microbiota: A potential target for the treatment of postmenopausal osteoporosis. Bone Research 5:17046.
- Xu, Y., N. Wang, H.-Y. Tan, S. Li, C. Zhang, and Y. Feng. 2020. Function of Akkermansia muciniphila in obesity: Interactions with lipid metabolism, immune response and gut systems. Frontiers in Microbiology 11:219. doi: 10.3389/fmicb.2020.00219.
- Xu, Z., W. Chen, Q. Deng, Q. Huang, X. Wang, C. Yang, and F. Huang. 2020. Flaxseed oligosaccharides alleviate DSS-induced colitis through modulation of gut microbiota and repair of the intestinal barrier in mice. Food & Function 11 (9):8077–88.
- Yan, F., N. Li, J. Shi, H. Li, Y. Yue, W. Jiao, N. Wang, Y. Song, G. Huo, and B. Li. 2019. Lactobacillus acidophilus alleviates type 2 diabetes by regulating hepatic glucose, lipid metabolism and gut microbiota in mice. Food & Function 10 (9):5804–15.
- Yan, J., S. Shi, H. Wang, R. Liu, N. Li, Y. Chen, and S. Wang. 2016. Neutral monosaccharide composition analysis of plant-derived oligo- and polysaccharides by high performance liquid chromatography. Carbohydrate Polymers 136:1273–80.
- Yun, S. I., H. O. Park, and J. H. Kang. 2009. Effect of Lactobacillus gasseri BNR17 on blood glucose levels and body weight in a mouse model of type 2 diabetes. Journal of Applied Microbiology 107 (5):1681–6. doi: 10.1111/j.1365-2672.2009.04350.x.
- Zaborska, K. E., and B. P. Cummings. 2018. Rethinking bile acid metabolism and signaling for type 2 diabetes treatment. Current Diabetes Reports 18 (11):109.
- Zeng, Z., Q. Yuan, R. Yu, J. Zhang, H. Ma, and S. Chen. 2019. Ameliorative effects of probiotic Lactobacillus paracasei NL41 on insulin sensitivity, oxidative stress, and beta-cell function in a type 2 diabetes mellitus rat model. Molecular Nutrition & Food Research 63 (22):1900457. doi: 10.1002/mnfr.201900457.
- Zhang, J., S. Wang, Z. Zeng, Y. Qin, Q. Shen, and P. Li. 2020. Anti-diabetic effects of Bifidobacterium animalis 01 through improving hepatic insulin sensitivity in type 2 diabetic rat model. Journal of Functional Foods 67:103843. doi: 10.1016/j.jff.2020.103843.
- Zhang, Z., Y. Chen, L. Xiang, Z. Wang, G. G. Xiao, and J. Hu. 2017. Effect of curcumin on the diversity of gut microbiota in ovariectomized rats. Nutrients 9 (10):1146. doi: 10.3390/nu9101146.
- Zhao, D., H. Zhu, F. Gao, Z. Qian, W. Mao, Y. Yin, J. Tan, and D. Chen. 2020. Antidiabetic effects of selenium-enriched Bifidobacterium longum DD98 in type 2 diabetes model of mice. Food & Function 11 (7):6528–41.
- Zhao, H., J. Chen, X. Li, Q. Sun, P. Qin, and Q. Wang. 2019. Compositional and functional features of the female premenopausal and postmenopausal gut microbiota. FEBS Letters 593 (18):2655–64.
- Zhu, J., M. Liao, Z. Yao, W. Liang, Q. Li, J. Liu, H. Yang, Y. Ji, W. Wei, A. Tan, et al. 2018. Breast cancer in postmenopausal women is associated with an altered gut metagenome. Microbiome 6 (1):136.