Abstract
Diabetic kidney disease (DKD) is one of the most serious complications of diabetes and has become the leading cause of end-stage kidney disease, causing serious health damage and a huge economic burden. Tubulointerstitial fibrosis play important role in the development of DKD. Itaconate, a macrophage-specific metabolite, has been reported to have anti-oxidant, anti-inflammatory effects. However, it is unknown whether it perform anti-fibrotic effect in renal tubular epithelial cells. In this current study, we observed that in human renal tubular epithelial cells (HK2), high glucose induced an increase in transforming growth factor β (TGF-β) production, and upregulated the expressions of fibronectin and collagen I through the TGF-β receptor as verified by administration of TGF-β receptor blocker LY2109761. Treatment with 4-octyl itaconate (4-OI), a derivant of itaconic acid, reduced the TGF-β production induced by high glucose and inhibited the pro-fibrotic effect of TGF-β in a dose-dependent manner. In addition, we found that 4-OI exerted its anti-fibrotic effect by inhibiting the excessive production of ROS induced by high glucose and TGF-β. In summary, 4-OI could ameliorate high glucose-induced pro-fibrotic effect in HK2 cell, and blocking the expression of TGF-β and reducing the excessive ROS production may be involved in its anti-fibrotic effect.
Introduction
DKD is one of the most common, devastating and expensive complications of diabetes. Currently, the prevalence of diabetes is increasing globally. The latest data from the International Diabetes Federation (IDF) show that approximately 537 million adults (aged 20–79 years) have diabetes worldwide in 2021, and approximately 20–40% of people with diabetes develop DKD [Citation1, Citation2]. Data show that DKD occurs in nearly half of all patients with type 2 diabetes and is the leading cause of chronic kidney disease (CKD) and end stage renal disease (ESRD) worldwide [Citation3, Citation4]. Recently, several clinical anti-diabetic drugs with promising effect in reducing the progression of DKD have been reported, including sodium-glucose cotransporter-2 inhibitors, glucagon-like peptide-1 receptor agonists and mineralocorticoid receptor antagonists [Citation5, Citation6]. Despite recent advances, in facing with devastating outcome of DKD, there are still urgently needs for exploring novel underlying molecular mechanisms involved in the progression of DKD. It is well known that progressive fibrosing feature in kidney is one of the core histological characteristics of DKD [Citation7]. The transforming growth factor β (TGF-β) signaling pathway, activated by the disturbance of metabolism and the overwhelming inflammation, plays a critical role in the progression in renal fibrosis in DKD [Citation8]. Blocking the progressive fibrosis process may protect the kidney from the metabolic chronic renal injury in DKD.
Itaconate, an α, β-unsaturated dicarboxylic acid (C5H6O4), was initially found to be synthesized by cis-aconitate decarboxylase which catalyze decarboxylation of the tricarboxylic acid (TCA) cycle intermediate cis-aconitate in Aspergillus terreus [Citation9]. It has been shown to play a key role in the host defense against pathogens. Studies have found that itaconate has antibacterial and antiviral effects [Citation10–13]. Moreover, endogenous itaconate synthesis has also found in immune cells under pro-inflammatory conditions [Citation14], and has emerged as a key immunoregulator by inducing antioxidant response, suppressing the expressions of pro-inflammatory cytokines, or inducing innate immune tolerance [Citation15, Citation16]. Recently, decreased immune responsive gene 1 protein (IRG1) expression in alveolar macrophages and low concentrations of itaconate in bronchoalveolar lavage fluid were observed in patients with idiopathic pulmonary fibrosis [Citation17]. As such, it implies that itaconate should play an important role in the progression of fibrosis. Currently, no transporter or receptor capable of transporting exogenous itaconate into the cytoplasm has been identified. Therefore, scientists designed membrane-permeable derivatives of itaconate, dimethyl itaconate (DI) and 4-octyl-itaconate (4-OI), both of which can permeate cells in the absence of a transporter [Citation18, Citation19]. And 4-OI becomes a better exogenous itaconate mimetic because of its lower electrophilic activity and superior lipid solubility [Citation18, Citation19].
As aforementioned, itaconate has been proved to have anti-pathogenic, anti-oxidative and anti-inflammatory effect. However, it is unknown whether and how itaconate exert its anti-fibrotic effect in a high glucose condition. In this study, we first observed whether administration of 4-OI alleviate the epithelial-mesenchymal transition (EMT) and fibrosis induced by high glucose in human renal tubular epithelial cell line HK2 cells. Additionally, we explored the possible mechanisms involved in its anti-fibrotic effect.
Materials and methods
Cell culture
CCK8 assays were performed to screen the optimal intervention concentration for 4-OI (Supplementary Figure S1). HK2 cells were cultured in different media according to the groups. The Normal (NC) group was cultured in DMEM medium with 5 mM glucose, the High glucose (HG) group in DMEM medium with 30 mM glucose, the TGF-β group in DMEM medium with 10 ng/ml TGF-β, and the other intervention groups were cultured in the above medium with 100 μM 4-OI, 10 μM LY2109761 and 10 mM N-acetyl-l-cysteine (NAC) respectively according to the intervention conditions. The cells were incubated at 37 °C for 48h in a 5% CO2 incubator, followed by assays and protein extraction.
Western blot analysis
Proteins were extracted using cell lysate (RIPA) and protease inhibitor Proteins were separated on SDS-PAGE gels and transferred to PVDF membranes, which were then placed in 5% BSA for 2 h at room temperature, after which the membranes were incubated overnight at 4 °C with primary antibody (Tubulin, Fibronectin, Vimentin, α-SMA). Next, secondary antibodies conjugated to horseradish peroxidase were incubated for 1 h at room temperature, rinsed and then added to the luminescent solution and incubated away from light (PMSF). Finally, images were developed using a chemiluminescent machine gel imaging system and the results were analyzed using Image J software.
Detection of reactive oxygen species (ROS)
Dilute the DCFH-DA probe according to the instructions. After 48h of intervention, the cell culture supernatant was removed and the cells were washed 3 times with sterile PBS. 1.5 ml of diluted DCFH-DA was added to each well and incubated for 20–30min in a CO2 incubator at 37 °C, protected from light. After incubation, cells were washed 3 times with serum-free DMEM medium, then 1 ml of saline was added to each well. Observe and acquire images in the dark with a fluorescent microscope.
Immunofluorescence
HK2 cells were configured as a cell suspension, 1 ml per well was dropped onto a crawl plate and incubated in 5% CO2, 37 °C in a constant temperature incubator, with stimulating factors added at the appropriate time according to experimental needs. Cells were washed three times with PBS and 4% formaldehyde was added to fix them at room temperature for 15 min. The cells were then punched with 0.2% Triton × 100 for 7 min at room temperature and then closed with 10% goat serum for 60 min at room temperature. Next, primary antibodies were added and incubated overnight in the dark at 4 °C in a refrigerator. The next day, the cells were washed and incubated with fluorescent secondary antibody for 60 min at room temperature in the dark, followed by the addition of DAPI for 10 min in the dark, followed by the addition of anti-fluorescence quencher dropwise and blocking. The cells were observed under a fluorescent microscope and photographed.
Human TGF-β ELISA
Supernatants of HK2 cells were collected after stimulation with different drugs and the supernatants were collected after centrifugation. The TGF-β ELISA kit was then used for testing. Ten standard wells were set up at concentrations of 2400, 1600, 800, 400 and 200 ng/ml, as well as two blank wells. The supernatant samples of each group of cells were added to the wells of the samples to be tested and incubated at 37 °C for 30 min. After washing, enzyme reagents were added, except for the blank wells. The wells were then incubated and washed again. Chromogenic Agent A and Chromogenic Agent B were added successively to each well, protected from light and incubated at 37 °C for 15 min before the reaction was terminated by adding the termination solution. The absorbance (OD) of each well was measured with a spectrophotometer set at 450 nm. The standard curve was plotted according to the OD value of the standard wells, and the OD value of each experimental group was substituted into the standard curve to calculate the concentration of TGF-β in the cell supernatant of each group.
Statistical analysis
Statistical analysis was performed using SPSS 19.0 software, and bar charts were plotted using GraphPad Prism software. Measures that were normally distributed were expressed as mean ± SEM. Means between multiple groups were analyzed and compared using one-way ANOVA, and multiple comparisons between groups were made using the LSD-t method. For all tests, a p value < 0.05 was considered statistically significant.
Results
HG induce a significant increase in the production of TGF-β and promote its pro-fibrosis through activating TGF-β receptors
Numerous studies have shown that TGF-β is one of the most important pro-fibrotic cytokines and plays an important role in almost all organ fibrosis processes [Citation20]. Therefore, we examined the expression levels of TGF-β in HK2 cells. The levels of TGF-β increased significantly after high glucose stimulation (). to demonstrate whether the activation of TGF-β signaling pathway mediates the pro-fibrotic effect induced by high glucose, TGF-β receptor blocker LY2109761 was used to treat HK2 cells cultured with 40 mM glucose. The results showed that high glucose promoted the expression of Fibronectin and Collagen I in HK2 cells, while the expression levels of these proteins were obviously inhibited after intervention with LY2109761(). These data suggest that high glucose can promote TGF-β production in renal tubular epithelial cells, and the increased TGF-β exhibit its profibrotic by activating TGF-β receptor signaling pathway.
Figure 1. High glucose induces an increase in TGF-β production and promotes its pro-fibrotic mediated by TGF-β receptors.
HK2 cells were cultured with 5 mM (NC) or 30 mM glucose (HG) for 48h. (a) The levels of TGF-β in the supernatant of HK2 was detected by ELISA; (b) The protein expressions of Fibronectin in HK2 were detected by western blot in HK2 cells sitimulated with 30 mM glucose with or without TGF-β receptor blocker LY2109761; (c) The protein expressions of collagen I protein in HK2 was detected by immunofluorescence. (*P < 0.05, vs NC; #P < 0.05, vs HG).
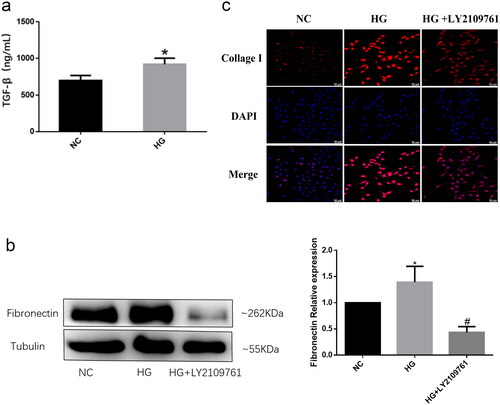
4-OI inhibits the profibrotic effect and epithelial-mesenchymal transition (EMT) induced by high glucose in human renal tubular epithelial cells
Next, we explored whether 4-OI has protective effect on pro-fibrotic effect induced by high glucose in HK2 cells. It was showed that the expression of extracellular matrix proteins Fibronectin, Collagen I and interstitial cell marker α-SMA and Vimentin increased significantly after high glucose stimulation, but this phenomenon was improved after 4-OI intervention (). The results show that 4-OI treatment ameliorate profibrotic effect and EMT induced by HG.
Figure 2. 4-OI inhibits the profibrotic effect and EMT induced by high glucose in HK2 cells.
HK2 cells were co-cultured with 30mM glucose and 100μM 4-OI (HG + OI). (a) The proteins expressions of Fibronectin, α - SMA and Vimentin in HK2 were detected by western blot; (b) The protein expressions of collagen I protein in HK2 was detected by immunofluorescence from each group. (*P < 0.05, vs NC; #P < 0.05, vs HG).
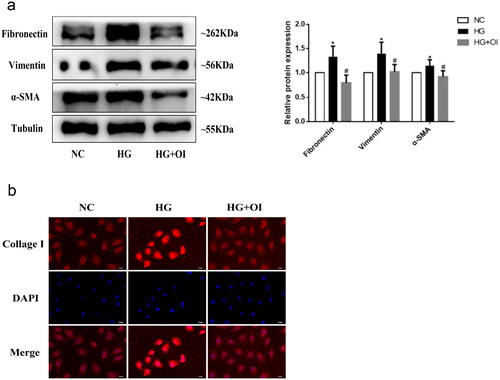
4-OI reduce the production of TGF-β induced by HG and inhibit the pro-fibrotic effect of TGF-β
The aforementioned results showed that high glucose induced an increase in TGF-β production, however, TGF-β levels were significantly reduced in the culture media from HK2 cells treated with 4-OI (). Moreover, 4-OI administration also blocked TGF-β-induced Fibronectin protein expression in a dose-dependent manner (). These data suggest that 4-OI can exert an anti-fibrotic effect by inhibiting high glucose-induced TGF-β production and by blocking the profibrotic effect of TGF-β.
Figure 3. 4-OI reduces the TGF-β production induced by high glucose and inhibited the pro-fibrotic effect of TGF-β.
(a) High glucose stimulated HK2 cells were cultured with or without 100μM 4-OI (HG + OI). The levels of TGF-β in the supernatant of HK2 was detected by ELISA; (b) The HK2 cells were administrated with 25 μM, 50 μM, or 100 μM 4-OI respectively, and simultanously co-cultured with 10 ng/ml TGF-β. The protein expressions of Fibronectin in HK2 were detected by western blot. (*P < 0.05, vs NC; NS P ≥ 0.05, vs TGF-β; #P < 0.05, vs TGF-β).
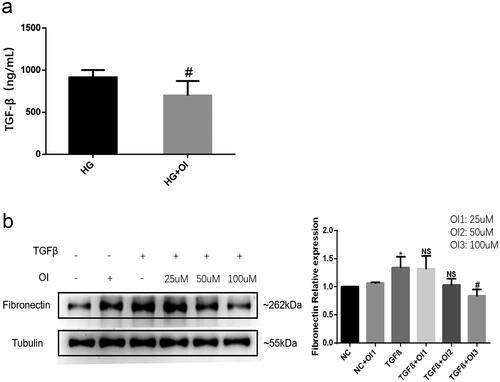
4-OI exerts its anti-fibrotic effect by inhibiting the excessive production of ROS induced by HG and TGF-β
Next, in order to evaluate whether 4-OI can exert its anti-fibrosis effect by inhibiting ROS overproduction, we detected the levels of ROS and fibrosis-related proteins in cells of each group. The results of ROS probe DCFH-DA staining showed that HG significantly increase the production of ROS in HK2 cells, and 4-OI intervention significantly reduced the level of ROS (). Similarly, TGF-β administration induced increased ROS production, which was reversed by the addition of 4-OI (). In addition, 4-OI can obviously inhibit the up-regulation of Fibronectin, Collagen I, α -SMA and Vimentin protein expression caused by TGF-β (). N-acetylcysteine (NAC) is a synthetic derivative of the endogenous amino acid L-cysteine and a precursor of the antioxidant glutathione (GSH) [Citation21]. In vivo, NAC exerts antioxidant effects, and in vitro, NAC has antifibrotic effects on lung fibroblasts [Citation21]. Thus, we used NAC as a positive control drug to inhibit ROS overproduction. Consistent with the results of in cells treated with 4-OI, the antioxidant NAC can inhibit the excessive production of ROS caused by TGF-β, and at the same time reduce the expression levels of the above-mentioned extracellular matrix proteins and mesenchymal cell marker proteins. collectively, the results suggest that 4-OI intervention reduce the excessive production of ROS caused by HG and TGF-β, and this effect may be one of the main mechanisms by which 4-OI inhibits HG-induced fibrosis and epithelial-mesenchymal transition of HK2 cells.
Figure 4. 4-OI exerts its anti-fibrotic effect by inhibiting the excessive production of ROS induced by high glucose and TGF-β.
HK2 cells were treated with 10 mM NAC or 100 μM 4-OI, and simultaneously stimulated with 10 ng/ml TGF-β or 30 mM glucose. (a)(b) Fluorescence images of ROS within HK2 detected using the DCFH-DA probe after the different treatments; (c) The proteins expressions of Fibronectin, α - SMA and Vimentin in HK2 were detected by western blot from each group; (d) The protein expressions of collagen I protein in HK2 was detected by immunofluorescence from each group. (*P < 0.05, vs NC; #P < 0.05, vs TGF-β).
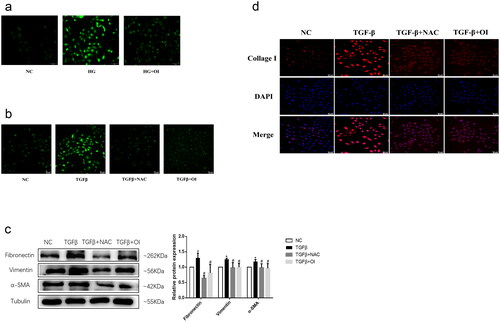
Discussion
In this current study, we observed that in HK2 cells, high glucose induced an increase in TGF-β production, and upregulated the expressions of fibronectin and collagen I through the TGF-β receptor as verified by administration of TGF-β receptor blocker LY2109761. Interestingly, treatment with 4-octyl itaconate (4-OI) reduced the TGF-β production induced by high glucose and inhibited the pro-fibrotic effect of TGF-β in a dose-dependent manner. Additionally, we found that 4-OI exerted its anti-fibrotic effect by inhibiting the excessive production of ROS induced by high glucose and TGF-β.
The pathological changes in DKD include glomerular hypertrophy, increased and deposited extracellular matrix (ECM), and later progression to glomerulosclerosis and tubulointerstitial fibrosis [Citation22, Citation23]. This abnormal ECM deposition can lead to disruption of the physiological structure of the kidney, causing organ remodeling and organ dysfunction and ultimately the development of ESRD. With emerging evidence, it is highlighted that tubular damage is important in diabetic kidney injury and can be an important predictor of deteriorating renal function in the setting of DKD [Citation24]. Tubulointerstitial fibrosis is a key component in the development of DKD to the end stage, mainly manifested by inflammatory cell infiltration, EMT in the tubular epithelium, excessive proliferation of interstitial fibroblasts and excessive deposition of ECM. Our results also show that HG induces increased ECM production and EMT in HK2 cells, suggesting that high glucose has a role in promoting fibrosis in human renal tubular epithelial cells.
In mammals, itaconate is produced by the decarboxylation of cis-aconitate catalyzed by the enzyme cis-aconitate decarboxylase, which is localized in the mitochondria and is encoded by the immune responsive gene 1 (Irg1). Irg1 is not expressed in unactivated macrophages. Stimulation by inflammatory factors such as LPS and interferon can lead to macrophage activation and induce Irg1 expression, resulting in the production of itaconic acid. In recent years, an increasing number of studies have shown that itaconate plays an important function in the regulation of inflammation, pathogenic infections and metabolism-related diseases. Emerging evidence showed that itaconate has anti-bacteria, anti-inflammatory and antioxidant effects. Recently, decreased IRG1 expression in alveolar macrophages and low concentrations of itaconate in bronchoalveolar lavage fluid were observed in patients with idiopathic pulmonary fibrosis [Citation17]. It has also been found that itaconate can alleviate the degree of renal fibrosis in unilateral ureteral obstruction and adenosine-induced renal fibrosis models, while in vitro cellular assays have also confirmed the anti-fibrotic effect of 4-OI, which provides a potential application for itaconate in renal fibrosis [Citation25]. In our current study, we observed that intervention of HK2 cells with 4-OI reduced high glucose-induced ECM production and EMT occurrence and improved renal tubular epithelial cell fibrosis. These data suggest that itaconate is much more potent in preventing the progress of diabetic renal fibrosis.
Numerous experimental studies have shown that TGF-β is the most important pro-fibrotic cytokine involved in fibrosis and that it is important in mediating the pro-fibrotic effects induced by high glucose and in the pathogenesis of DKD. TGF-β binds to cell surface-specific receptors and activates downstream smad-dependent cascades or smad-independent signaling pathways to perform a variety of regulatory functions, which are involved in a variety of physiological and pathological processes including cell growth, differentiation, apoptosis and regulation of ECM synthesis and degradation [Citation26, Citation27]. TGF-β stimulates the secretion of large amounts of ECM components such as Fibronectin, Collagen, laminin and proteoglycans by renal tubular epithelial cells and induces fibroblasts to proliferate and activate into myofibroblasts, leading to massive secretion and deposition of ECM [Citation28]. In addition, TGF-β reduces ECM protein degradation, triggers ECM remodeling, inhibits the expression of the epithelial marker E-calmodulin and causes EMT in renal tubular epithelial cells, resulting in loss of tight junctions between adjacent cells, loss of cell polarity and integrity, and massive intracellular expression of the mesenchymal markers Vimentin and α-SMA, ultimately leading to fibrosis [Citation27, Citation29]. Previous studies have shown that high glucose culture of HK2 cells can increase the expression level and bioactivity of TGF-β, accompanied by ECM accumulation, mediating the development of renal fibrosis [Citation30, Citation31]. In contrast, application of inhibitors to block the expression of TGF-β significantly alleviated the development of renal fibrosis [Citation32, Citation33]. In the present study, TGF-β protein expression was increased in the high-glucose group and fibrosis levels were also significantly increased, while the administration of TGF-β receptor blockers improved fibrosis in HK2. This is consistent with the expression trends of previous studies, suggesting that high glucose can promote fibrosis by inducing increased TGF-β production and by activating TGF-β-specific signaling pathways. Interestingly, intervention with itaconate resulted in a significant reduction in the expression of the pro-fibrotic factor TGF-β and an improvement in the level of fibrosis, as well as alleviating the level of TGF-β-induced cellular fibrosis. These data suggested that itaconate has a protective effect against high glucose-induced fibrosis, and that inhibition of TGF-β production and bioactivity may be involved in the mechanism of the anti-fibrotic effect of itaconate.
Oxidative stress manifests itself in the excessive production of ROS and is closely associated with fibrosis in various organs. Under normal conditions, the level of ROS in the body remains within a certain range and there is a balance between oxidative stress and the antioxidant system in the body, which does not cause significant damage to the body. However, in diabetic patients, the production of ROS exceeds the scavenging capacity of the body’s antioxidant system, resulting in a disruption of the balance between the body’s oxidative and antioxidant systems, exposing the body to oxidative stress and resulting in oxidative damage to cells. Many studies have shown that hyperglycemia induces excessive production of ROS which led to oxidative damage in renal and promote the development of DKD [Citation34–36]. There is growing evidence that the oxidative stress state resulting from excessive ROS production is interlinked with the production and activation of various growth factors and cytokines, which play a key role in the fibrosis process [Citation37, Citation38]. Among them, TGF-β is a key factor in the formation and progression of DKD and plays an important role in almost all organ fibrosis processes [Citation20]. In diabetic patients, the high glucose environment can directly cause oxidative damage to renal tubular epithelial cells and stimulate them to produce large amounts of ROS. Excess ROS can transduce cascade reactions by activating signaling factors such as Protein kinase C (PKC) and can also act as an intracellular signal to activate transcription factors such as nuclear factor-κB (NF-κB), causing increased expression of TGF-β [Citation39]. The above series of signaling molecules and cascade reactions can further cause damage to renal tubular epithelial cells and induce an increasing number of ROS. TGF-β and ROS interact and promote each other, thus continuously amplifying the oxidative damage to renal tubular epithelial cells caused by high glucose, causing kidney damage and eventually leading to tubulointerstitial fibrosis and even renal failure [Citation40]. It is proposed that targeting excessive ROS production should be a promissing therapeutic strategy. A recent study reported that elimination of ROS production could rescue Islet β Cells in T2DM mice using selenium nanodots (SENDs), a prodrug of the antioxidant enzyme glutathione peroxidase 1 (GPX1) [Citation41]. Our results show that high glucose stimulation leads to increased ROS production in HK2 cells, however, 4-OI intervention significantly reduces the excessive production of ROS. Similarly, TGF-β stimulation increased ROS production in HK2 cells, inducing EMT and HK2 fibrosis levels, while blocking ROS production with the ROS-specific inhibitor NAC improved fibrosis levels and EMT markers. 4-OI had similar results to NAC in that it also reduced intracellular ROS production levels and reversed fibrosis and EMT, suggesting that itaconate may play a protective role in reversing tubular epithelial fibrosis and EMT by blocking the excessive production of ROS caused by high glucose and TGF-β.
In summary, 4-OI could ameliorate high glucose-induced pro-fibrotic effect in HK2 cell, and blocking the expression of TGF-β induced by high glucose and inhibited the pro-fibrotic effect of TGF-β in a dose-dependent manner. Additionally, we found that 4-OI exerted its anti-fibrotic effect by inhibiting the excessive production of ROS induced by high glucose and TGF-β. However, there are several limitations of this study. First, we just observed anti-fibrotic effect of 4-OI in HK2 cells cultured in vitro, but not test its effects on animal models of DKD. Second, there were no existing anti-fibrotic agents, such as angiotensin-converting enzyme inhibitors or angiotensin receptor blockers, included as positive control to compare the relative advantages and disadvantages of 4-OI in terms of efficacy and safety of 4-OI. Furthermore, detailed studies are still urgently needed to explore the underlying molecular mechanisms by which 4-OI inhibits TGF-β production and ROS generation.
Authors’ contributions
Xiaoli Zou: Data curation, Formal analysis, Writing-original draft. Maoyan Wu: Data curation, Formal analysis, Writing-original draft. Mengqin Tu: Conceptualization, Formal analysis. Xiaozhen Tan: Conceptualization, Formal analysis. Yang Long: Project administration, Supervision, Writing-review & editing. Yong Xu: Project administration, Supervision, Writing-review & editing. Mingxiu Li: Project administration, Supervision, Writing-review & editing.
Ethical approval statement
This experiment is basic research and does not involve ethical approval.
Supplemental Material
Download TIFF Image (151.3 KB)Acknowledgments
We would like to thank anyone who contributed to this article.
Disclosure statement
The authors have no relevant financial or non-financial interests to disclose.
Data availability statement
The datasets generated during and/or analyzed during the current study are available from the corresponding author on reasonable request.
Additional information
Funding
References
- Sun H, Saeedi P, Karuranga S, et al. IDF diabetes atlas: global, regional and country-level diabetes prevalence estimates for 2021 and projections for 2045. Diabetes Res Clin Pract. 2022;183:109119. doi:10.1016/j.diabres.2021.109119.
- Ma X, Ma J, Leng T, et al. Advances in oxidative stress in pathogenesis of diabetic kidney disease and efficacy of TCM intervention. Ren Fail. 2023;45(1):2146512. doi:10.1080/0886022X.2022.2146512.
- Kuenz A, Krull S. Biotechnological production of itaconic acid-things you have to know. Appl Microbiol Biotechnol. 2018;102(9):3901–3914. doi:10.1007/s00253-018-8895-7.
- Shin JH, Yang JY, Jeon BY, et al. (1)H NMR-based metabolomic profiling in mice infected with Mycobacterium tuberculosis. J Proteome Res. 2011;10(5):2238–2247. doi:10.1021/pr101054m.
- Agarwal R, Filippatos G, Pitt B, et al. Cardiovascular and kidney outcomes with finerenone in patients with type 2 diabetes and chronic kidney disease: the FIDELITY pooled analysis. Eur Heart J. 2022;43(6):474–484. Erratum in: eur Heart J. 2022 May 21;43(20):1989. doi:10.1093/eurheartj/ehab777.
- Zhang Y, Jiang L, Wang J, et al. Network meta-analysis on the effects of finerenone versus SGLT2 inhibitors and GLP-1 receptor agonists on cardiovascular and renal outcomes in patients with type 2 diabetes mellitus and chronic kidney disease. Cardiovasc Diabetol. 2022;21(1):232. doi:10.1186/s12933-022-01676-5.
- Zhang Y, Jin D, Kang X, et al. Signaling pathways involved in diabetic renal fibrosis. Front Cell Dev Biol. 2021;9:696542. doi:10.3389/fcell.2021.696542.
- Wu W, Huang XR, You Y, et al. Latent TGF-β1 protects against diabetic kidney disease via arkadia/Smad7 signaling. Int J Biol Sci. 2021;17(13):3583–3594. Aug 19 doi:10.7150/ijbs.61647.
- Peace CG, O'Neill LA. The role of itaconate in host defense and inflammation. J Clin Invest. 2022;132(2):e148548. doi:10.1172/JCI148548.
- Hersch SJ, Navarre WW. The Salmonella LysR family regulator RipR activates the SPI-13-Encoded itaconate degradation cluster. Infect Immun. 2020;88(10):e00303-20. doi:10.1128/IAI.00303-20.
- Daniels BP, Kofman SB, Smith JR, et al. The nucleotide sensor ZBP1 and kinase RIPK3 induce the enzyme IRG1 to promote an antiviral metabolic state in neurons. Immunity. 2019;50(1):64–76. e4. Jan 15Epub 2019 Jan 8. doi:10.1016/j.immuni.2018.11.017.
- Olagnier D, Farahani E, Thyrsted J, et al. SARS-CoV2-mediated suppression of NRF2-signaling reveals potent antiviral and anti-inflammatory activity of 4-octyl-itaconate and dimethyl fumarate. Nat Commun. 2020;11(1):5419. Oct 2;11(1):4938. Erratum in: nat Commun. 2020 Oct 21 doi:10.1038/s41467-020-19363-y.
- Sohail A, Iqbal AA, Sahini N, et al. Itaconate and derivatives reduce interferon responses and inflammation in influenza a virus infection. PLoS Pathog. 2022;18(1):e1010219. Erratum in: PLoS Pathog. 2022 Nov 2918(11):e1011002. doi:10.1371/journal.ppat.1011002.
- Chen F, Lukat P, Iqbal AA, et al. Crystal structure of cis-aconitate decarboxylase reveals the impact of naturally occurring human mutations on itaconate synthesis. Proc Natl Acad Sci U S A. 2019;116(41):20644–20654. doi:10.1073/pnas.1908770116.
- Mills EL, Ryan DG, Prag HA, et al. Itaconate is an anti-inflammatory metabolite that activates Nrf2 via alkylation of KEAP1. Nature. 2018;556(7699):113–117. doi:10.1038/nature25986.
- Domínguez-Andrés J, Novakovic B, Li Y, et al. The itaconate pathway is a Central regulatory node linking innate immune tolerance and trained immunity. Cell Metab. 2019;29(1):211–220.e5. doi:10.1016/j.cmet.2018.09.003.
- Ogger PP, Albers GJ, Hewitt RJ, et al. Itaconate controls the severity of pulmonary fibrosis. Sci Immunol. 2020;5(52):eabc1884. doi:10.1126/sciimmunol.abc1884.
- Puchalska P, Huang X, Martin SE, et al. Isotope tracing untargeted metabolomics reveals macrophage Polarization-State-specific metabolic coordination across intracellular compartments. iScience. 2018;9:298–313. doi:10.1016/j.isci.2018.10.029.
- Li R, Zhang P, Wang Y, et al. Itaconate: a metabolite regulates inflammation response and oxidative stress. Oxid Med Cell Longev. 2020;2020:5404780. doi:10.1155/2020/5404780.
- Rittenhouse JW, McFadden BA. Inhibition of isocitrate lyase from Pseudomonas indigofera by itaconate. Arch Biochem Biophys. 1974;163(1):79–86. doi:10.1016/0003-9861(74)90456-1.
- Raghu G, Berk M, Campochiaro PA, et al. The multifaceted therapeutic role of N-Acetylcysteine (NAC) in disorders characterized by oxidative stress. Curr Neuropharmacol. 2021;19(8):1202–1224.
- Nair S, Huynh JP, Lampropoulou V, et al. Irg1 expression in myeloid cells prevents immunopathology during M. tuberculosis infection. J Exp Med. 2018;215(4):1035–1045. doi:10.1084/jem.20180118.
- Zhao C, Jiang P, He Z, et al. Dimethyl itaconate protects against lippolysacchride-induced mastitis in mice by activating MAPKs and Nrf2 and inhibiting NF-κB signaling pathways. Microb Pathog. 2019;133:103541. doi:10.1016/j.micpath.2019.05.024.
- Lampropoulou V, Sergushichev A, Bambouskova M, et al. Itaconate links inhibition of succinate dehydrogenase with macrophage metabolic remodeling and regulation of inflammation. Cell Metab. 2016;24(1):158–166. doi:10.1016/j.cmet.2016.06.004.
- Shen H, Campanello GC, Flicker D, et al. The human knockout gene CLYBL connects itaconate to vitamin B (12). Cell. 2017;171(4):771–782.e711. doi:10.1016/j.cell.2017.09.051.
- Sporn MB, Liby KT. NRF2 and cancer: the good, the bad and the importance of context. Nat Rev Cancer. 2012;12(8):564–571. doi:10.1038/nrc3278.
- Shan Q, Li X, Zheng M, et al. Protective effects of dimethyl itaconate in mice acute cardiotoxicity induced by doxorubicin. Biochem Biophys Res Commun. 2019;517(3):538–544. doi:10.1016/j.bbrc.2019.07.046.
- Liu H, Feng Y, Xu M, et al. Four-octyl itaconate activates Keap1-Nrf2 signaling to protect neuronal cells from hydrogen peroxide. Cell Commun Signal. 2018;16(1):81. doi:10.1186/s12964-018-0294-2.
- Bambouskova M, Gorvel L, Lampropoulou V, et al. Electrophilic properties of itaconate and derivatives regulate the IκBζ-ATF3 inflammatory axis. Nature. 2018;556(7702):501–504. doi:10.1038/s41586-018-0052-z.
- Tang C, Tan S, Zhang Y, et al. Activation of Keap1-Nrf2 signaling by 4-octyl itaconate protects human umbilical vein endothelial cells from high glucose. Biochem Biophys Res Commun. 2019;508(3):921–927. doi:10.1016/j.bbrc.2018.12.032.
- Cordes T, Lucas A, Divakaruni AS, et al. Itaconate modulates tricarboxylic acid and redox metabolism to mitigate reperfusion injury. Mol Metab. 2020;32:122–135. doi:10.1016/j.molmet.2019.11.019.
- Zhang D, Lu Z, Zhang Z, et al. A likely protective effect of dimethyl itaconate on cerebral ischemia/reperfusion injury. Int Immunopharmacol. 2019;77:105924. doi:10.1016/j.intimp.2019.105924.
- Tian F, Wang Z, He J, et al. 4-Octyl itaconate protects against renal fibrosis via inhibiting TGF-β/smad pathway, autophagy and reducing generation of reactive oxygen species. Eur J Pharmacol. 2020;873:172989. doi:10.1016/j.ejphar.2020.172989.
- Tryfonidis K, Basaran G, Bogaerts J, et al. A european organisation for research and treatment of cancer randomized, double-blind, placebo-controlled, multicentre phase II trial of anastrozole in combination with gefitinib or placebo in hormone receptor-positive advanced breast cancer (NCT00066378). Eur J Cancer. 2016;53:144–154. doi:10.1016/j.ejca.2015.10.012.
- Weiss JM, Davies LC, Karwan M, et al. Itaconic acid mediates crosstalk between macrophage metabolism and peritoneal tumors. J Clin Invest. 2018;128(9):3794–3805. doi:10.1172/JCI99169.
- Pan J, Zhao XY, Lin CN, et al. Immune responsive gene 1, a novel oncogene, increases the growth and tumorigenicity of glioma. Oncol Rep. 2014;32(5):1957–1966. doi:10.3892/or.2014.3474.
- Shi HZ, Wang D, Sun XN, et al. MicroRNA-378 acts as a prognosis marker and inhibits cell migration, invasion and epithelial-mesenchymal transition in human glioma by targeting IRG1. Eur Rev Med Pharmaco. 2018;22(12):3837–3846.
- Wang Q, Li XL, Mei Y, et al. The anti-inflammatory drug dimethyl itaconate protects against colitis-associated colorectal cancer. J Mol Med (Berl). 2020;98(10):1457–1466. doi:10.1007/s00109-020-01963-2.
- Kobayashi EH, Suzuki T, Funayama R, et al. Nrf2 suppresses macrophage inflammatory response by blocking proinflammatory cytokine transcription. Nat Commun. 2016;7(1):11624. doi:10.1038/ncomms11624.
- McFadden BA, Purohit S. Itaconate, an isocitrate lyase-directed inhibitor in Pseudomonas indigofera. J Bacteriol. 1977;131(1):136–144. doi:10.1128/jb.131.1.136-144.1977.
- Huang Q, Liu Z, Yang Y, et al. Selenium nanodots (SENDs) as antioxidants and antioxidant-prodrugs to rescue islet β cells in type 2 diabetes mellitus by restoring mitophagy and alleviating endoplasmic reticulum stress. Adv Sci (Weinh). 2023;10(19):e2300880.