ABSTRACT
Apigenin is being used by humans in the form of plant extract for the treatment of a number of disorders and inflammatory conditions, until its discovery as a core compound. Apigenin, chemically known as 4′, 5, 7,-trihydroxyflavone is a yellow crystalline powder belonging to the flavone class, that is the aglycone of several naturally occurring glycosides. It is insoluble in water but soluble in organic solvents. Numerous pharmacological activities, including anti-inflammatory, anti-toxicant, anti-cancer, etc., are attributed to apigenin. Research has shown that apigenin has numerous molecular targets involved in inflammation. Based on the in vivo, in vitro, and clinical trial studies suggested that apigenin is a potent therapeutic agent to overcome diseases such as rheumatoid arthritis, autoimmune disorders, Parkinson’s disease, Alzheimer’s disease, and various type of cancers. Delayed plasma clearance and slow decomposition in liver increases its systemic bioavailability, and makes it a strong therapeutic agent in pharmaceutical studies. In the present review, detailed accounts of the properties of apigenin have been discussed.
Introduction
Flavonoids have been known as an important plant product for over a century. Rusznyakand Szent-Gyorgyi for the first time in 1936 published literature regarding their biologicalactivity.[Citation1] The term “vitamin P” was suggested for flavonoids, but it was later dismissed.[Citation2] Flavonoids belong to the large group of polyphenolic compounds that are widely distributed in all foods of plant origin and are divided into sub classes, including flavones, flavanones, flavanols, isoflavones, anthocyanidins, and flavanols.[Citation3] Polyphenols are produced as a result of the secondary metabolism of plants and are frequently found attached to sugars (glycosides), thus tending to be water-soluble. Occasionally, polyphenols also occur in plants as aglycones. Polyphenols arise biogenetically from two main synthetic pathways: the shikimate pathway and the acetate pathway.[Citation4] More than 8000 polyphenolic structures are currently known.[Citation5]
The basic carbon skeleton of a flavonoid is the flavan nucleus, containing 15 carbons arranged in two aromatic rings connected by a 3 carbon bridge, forming a diphenyl propane structure (C6-C3-C6), which may or may not be the part of third ring.[Citation7] For convenience the rings are named as A, B, and C (). Biogenetically, ring A usually arises from a molecule of resorcinol or phloroglucinol synthesized from the acetate pathway and has a characteristic hydroxylation pattern at the 5th and 7th position.[Citation8] The B ring comes from the shikimate pathway and is usually 4´, 3´, 5´-hydroxylated. Flavonoids occur as aglycones, glycosides, and methylated derivatives.[Citation9]For the nomenclature of flavonoids A and C rings have simple numerals, while primed numerals are used for B-ring. Primed modified numbering system is not used for chalcones and the isoflavones derivatives: the pterocarpans and the rotenoids.[Citation10]
Figure 1. Basic structure of flavonoids.[Citation6]
![Figure 1. Basic structure of flavonoids.[Citation6]](/cms/asset/94ea93ec-7c79-47c8-9fe3-5c891dac9c5a/ljfp_a_1207188_f0001_b.gif)
Literature exists regarding flavonoids content in food and beverages of plant origin, such as fruits, vegetables, tea, cocoa, and wine.[Citation11–Citation16] Flavonoids have been reported to exert wide range of biological activities. These includes: anti-inflammatory, antibacterial, antiviral, antiallergic[Citation17-Citation19], cytotoxic antitumor, treatment of neurodegenerative diseases, vasodilatory action.[Citation18,Citation20–Citation22] In addition flavonoids are known to inhibit lipid-peroxidation, platelet aggregation, capillary permeability and fragility, cyclo-oxygenase, and lipoxygenase enzyme activities. They exert these effects as antioxidants, free radical scavengers, chelators of divalent cation.[Citation19,Citation22,Citation23] These are also reported to inhibit variety of enzymes like hydrolases, hyalouronidase, alkaline phosphatase (ALP), arylsulphatase, cAMP phosphodiesterase, lipase, α-glucosidase kinase.[Citation24] Apart from these studies, one group of researchers showed that there was no significant association between the total dietary intake of flavonoids in food and the incidence of site specific cancers.[Citation25] The dietary intake of flavonoids is estimated to be 1–2 g/day.[Citation26] The average intake of flavonoids as flavonols and flavones have ranged from 6 mg/day in Finland to 64 mg/day in Japan, with intermediate intake in the United States (13 mg/day), Italy (27 mg/day), and the Netherlands (33 mg/day). The estimates were based on the intake of five major flavonoids: quercitin, luteolin, apigenin, myricetin, and kaempferol in composite food.[Citation27]
Apigenin
Apigenin, chemically known as 4′, 5, 7,-trihydroxyflavone, is a natural product belonging to the flavone class that is the aglycone of several naturally occurring glycosides with molecular formula C15H10O5 and molecular weight 270.24 (). Flavones, as well as some of their synthetic derivatives, have been shown to exhibit several biological activities, including antioxidant, anti-inflammatory, antitumor, ant-genotoxic, anti-allergic, neuroprotective, cardioprotective, and antimicrobial.[Citation28] Apigenin is a yellow crystalline solid that has been used to dye wool.
Apigenin derivatives or natural analogues
Apigenin is a flavonoid derivative with three hydroxyl substituent, as suggested by its chemical name (4′, 5, 7,-trihydroxyflavone). Removal of the hydroxyl groups yields basic structure of flavones (a). Apigenin can be mono-substituted separately at positions 4′, 5 and 7, resulting in the formation of different compounds 4′-hydroxyflavone (b), 7-hydroxyflavone (c), and 5-hydroxyflavone (d). Further hydroxylation can generate three dihydroxy-flavones 4′,7-dihydroxyflavone (e), 4′,5-dihydroxyflavone (f), and 5,7-dihydroxy flavones (g; ). Overall, the apigenin has at most seven possible derivatives/analogues generated from the selective hydroxyl substitutions at positions 4′, 5, and 7 of the basic flavonoid skeleton.[Citation29]
Figure 3. Apigenin derivatives and natural analogues.[Citation29]
![Figure 3. Apigenin derivatives and natural analogues.[Citation29]](/cms/asset/d482cc5a-9ec5-4aa5-8484-867e8df2e95e/ljfp_a_1207188_f0003_b.gif)
Genkwanin which is an O-methyl derivative of apigenin has been indentified so far in plants like Daphne genkwa, seeds of Alnus glutinosa.[Citation30,Citation31] Another methoxy derivative of apigenin is acacetin (Apigenin-7-O-β-D-galactopyranoside) synthesized by the action of an enzyme apigenin 4’-O methyl transferase. Acacetin is isolated from Turnera diffusa and Chrysanthemum morifolium.[Citation32,Citation33] The enzyme apigenin 4’-O methyltransferase uses S-adenosyl methionine and 5,7,4’-trihydroxyflavone (apigenin) to produce S-adenosylhomocysteine and 4-methoxy 5,7-dihydroflavone (Acacetin). In nature, apigenin also exists as a dimer, bi-apigenin, mainly isolated from the buds and flowers of Hypericum perforatum (),[Citation34] which has neuroprotective effects.[Citation34,Citation35]
Figure 4. Structure of bi-apigenin.[Citation34]
![Figure 4. Structure of bi-apigenin.[Citation34]](/cms/asset/5d76dda5-f1b6-4a58-a086-c007b88f165d/ljfp_a_1207188_f0004_b.gif)
Biosynthesis of apigenin and its derivatives
Apigenin is synthesized in a number of plants as secondary metabolite. A variety of plants, such as parsley, celery, onions, oranges, chamomile, maize, rice, tea, wheat sprouts, some grasses, etc., are known to synthesis apigenin and its derivatives.[Citation3,Citation36–Citation38] All flavonoids are basically synthesized in plants from a single basic pathway called shikimic acid pathway. This pathway converts simple carbohydrate precursor’s erythrose-4-phosphate (E4P) and phosphoenol pyruvate (PEP) to aromatic aminoacids. E4P and PEP act as initiating molecules which fuse together under the influence of dihydroarabino heptulosonate-7-phosphate synthase (DAHP synthase) to form dihydroarabino heptulosonate-7-phosphate. The product is subsequently transformed into dehydroquinate, dehydro shikimate, shikimic acid, and aromatic ring containing aminoacids (phenylalanine and tyrosine) under the influence of respective enzymes shown in . Most abundant classes of flavonoids in plants are derived from deamination of phenylalanine and tyrosine to cinnamic acid. Cinnamic acid undergoes several downstream biosynthetic steps producing naringenin (). Naringenin is the key intermediate of the flavone/anthocyanin pathway, serving as the common precursors for a large number of downstream flavonoids as described previously.[Citation37–Citation39] A complete, well-defined proposed pathway for apigenin and its derivatives biosynthesis is given in . Certain glycosyl transferases, hydroxyl transferases, and methyl transferases are thought to be involved in O- or C-glycosylation, methylation, and hydroxylation of apigenin to form its derivatives. Recent studies have shown that O-methyl derivatives of apigenin like genkwanin can be synthesized in E.coli cells on introducing six plant derived genes viz; tyrosine ammonia lyase (TAL), 4-coumaroyl coenzyme-A ligase (4-CL), chalone synthase (CHS), chalone isomerase (CHI), flavone synthase (FNS), and apigenin 7-O-methyl transferase (POMT7) in them. Recombinant E. coli strain was able to produce 41 µg/L genkwanin (7-O-methyl apigenin) in culture.[Citation40]
Apigenin conjugates in plants
Conjugation of apigenin with sugars forms a number of naturally occurring glycosides in plants ().
Apiin (apigenin 7-O-apioglucoside), isolated from parsley and celery.[Citation41]
Apigetrin (apigenin 7-glucoside), found in Teucrium gnaphalodes[Citation42] and in the roots of dandelion coffee.
Vitexin (apigenin 8-C-glucoside), present in mung bean[Citation43] and in the bamboo leaves,Citation44 Ficus deltoidea.Citation45
Isovitexin (apigenin 6-C-glucoside), found in mung bean,[Citation43] Ficus deltoidea.[Citation45]
Rhoifolin (apigenin 7-O-neohesperidoside), isolated from the leaves of Rhus succedanea,[Citation46] Citrus grandis.[Citation47]
Schaftoside (apigenin 6-C-glucoside 8-C-arabinoside), isolated from Arisaema heterophyllum.[Citation48]
Acacetin (4’- methoxy 5,7-dihydroxyflavone), found in Turnera diffusa,[Citation32] Chrysanthemum morifolium.[Citation33]
Genkwanin (4’,5-Dihydroxy-7-methoxyflavone), it is synthesised in Daphne genkwa,[Citation30] seeds of Alnus glutinosa.[Citation31]
Figure 6. Apigenin conjugates in plants.[Citation30,Citation33,Citation41,Citation42,Citation45,Citation47,Citation48]
![Figure 6. Apigenin conjugates in plants.[Citation30,Citation33,Citation41,Citation42,Citation45,Citation47,Citation48]](/cms/asset/26ead040-6fce-4751-b623-e050351c768b/ljfp_a_1207188_f0006_b.gif)
Apigenin-6-C-β-fucopyranoside and apigenin-6-C-(2″-O-α-rhamnopyranosyl)-β-fucopyranoside obtained from Averrhoa carambola,[Citation49] cosmosiin from Citrus grandis,[Citation47] apigenin 7-O (6-malonyl β-Dglucoside) are among many other apigenin derivatives.
Bioavailability and metabolism of apigenin
Apigenin is abundantly present in common fruits such as grapefruit, plant-derived beverages, and vegetables such as parsley, onions, oranges, tea, maize, rice, wheat sprouts chamomile, wheat sprouts, and in some seasonings.[Citation3,Citation36,Citation37] Parsley (Petroselinum crispum) and peppers (Piper nigrum) are two major sources of flavones containing 13.526 mg/100 g and 4.98 mg/100 g, respectively.[Citation36] Celery is also a good source of apigenin containing 108 mg apigenin per kg.[Citation50,Citation38] One of the most common sources of apigenin consumed as single ingredient herbal tea is chamomile, prepared from the dried flowers from Matricaria chamomilla.[Citation51] Other sources for apigenin include beverages such as wine and beer brewed from natural ingredients[Citation12,Citation14] in natural sources like Chamomilla recutita, apigenin is present as apigenin-7-O-glucoside and various other acylated derivatives.[Citation52] Some among the numerous other plants from which apigenin and its derivatives are obtained include Daphne genkwa,[Citation30,Citation53] Acacia farnesianal,[Citation54] Apium graveolens,[Citation55] Mentha longifolia,[Citation56] Euterpe oleracea Mart.,[Citation57] Jatropha gossypifolia,[Citation58,Citation59] Scutellaria barbata,[Citation60] Ginkgo biloba,[Citation61] Equisetum fluviatile,[Citation62] Wedella chinensis,[Citation63] Andrographis paniculata,[Citation64] Chrysanthemum morifolium,[Citation65,Citation66] Teucrium gnaphalodes,[Citation42] Lycopodium clavatum,[Citation67] Perilla frutescens,[Citation68] and Thevetia peruviana.[Citation69]
Factors that affect the circulating levels and subsequent bio-distribution of apigenin is its conversion to larger molecules in tissues. For example, glucuronides that are formed in the intestinal mucosa can be secreted back into the lumen of the gut, reducing net absorption.[Citation70,Citation71] These conjugated flavonoids may also be transported through the efflux transporters multi-drug resistance protein-1 (also referred to P-gp, ABCB1, CD-243) and multi-drug resistance-associated protein-2 (also referred to ABCC2 and CMOAT),[Citation72] the distribution of which can be dramatically altered in cancer.[Citation73] Apigenin is also subject to methylation, sulfation, and glucuronidation, each of which affects its distribution and bioactivity.[Citation74] Apigenin metabolism within the intestinal epithelium has been studied in the Caco-2 cell monolayer system. Apigenin is a substrate for glucuronidation by the uridine 5′-diphospho-glucuronosyl transferase present in intestinal epithelial cells.[Citation75] Metabolism of scutellarin given intravenously and Chrysanthemum morifolium extract given orally to rats suggests that most first-pass metabolism of this flavonoid takes place in the intestinal mucosa, with very little contribution of presystemic hepatic elimination.[Citation76–Citation78] However, other work has shown that apigenin can be efficiently glucuronidated and also sulfated in hepatocyte subcellular fractions, suggesting that precise kinetics depend upon the form of flavonoids.[Citation79] A generalized model of apigenin absorption and distribution in various tissues/organs and its excretion is presented in (). Microbes along with some plant enzymes also play an important role in bio-transformation of different flavonoid groups, exactly mimicking mammalian and plant metabolism at least in some cases. This study may be useful with reference to the microflora present in the human gut and their effect on flavonoids.[Citation80] Flavonoids has poor bioavailabilities largely because of the metabolism via UDP-glucuronosyltransferases (UGTs). The two flavonoids namely apigenin and genistein are metabolized more rapidly in intestine than in liver through the involvement in UGTs. This was demonstrated using yeast cells expressing UGT1A isoform isolated from rats. Intestinal excretion of flavonoids in Gunn rats (deficient in UGT1A) compared to Wistar rats were found to be higher for apigenin, suggesting up-regulation of UGT isoforms in Gunn rats. Flavonoids are efficiently metabolized by UGT1A deficient Gunn rats because of compensatory up-regulation of intestinal UGT2B and hepatic anion efflux transporters, which increases their disposition and limited their bioavailabilities.[Citation81]
Figure 7. A generalized model of apigenin absorption and distribution in humans and other mammalian models.[Citation82]
![Figure 7. A generalized model of apigenin absorption and distribution in humans and other mammalian models.[Citation82]](/cms/asset/2e7b503d-fd97-4476-ab2d-5dc4a50da362/ljfp_a_1207188_f0007_b.gif)
From an in vivo and ex vivo study on rat liver it was found that apigenin undergoes phase I metabolism to produce monohydroxy derivatives viz; luteolin, scutellarein, iso-scutellarein, which in turn subjects through phase II metabolism producing conjugation products. Of them, three were monoglucurono conjugates and one monosulpho conjugate. However, luteolin a product of phase I metabolism produced four monoglucurono conjugates, two sulphoconjugates, and one methyl conjugate (identified as diosmetin) in in vitro. In contrast to this, none of the phase I metabolites were found in rat livers perfused with apigenin (ex vivo). But, two monoglucurono conjugates and one sulpho conjugate of apigenin were recovered as a part of phase II metabolism.[Citation83] In a similar study a single oral administration of radio labeled apigenin, 51.0% of radioactivity was recovered in urine, 12.0% in faeces, 1.2% in the blood, 0.4% in the kidneys, 9.4% in the intestine, 1.2% in the liver, and 24.8% in the rest of the body within 10 days. Adding to this, immature male and female rats, excreted a higher percentage of the mono-glucuronoconjugate of apigenin than the mono-sulfoconjugate of apigenin (10.0–31.6% versus 2.0–3.6%, respectively), which is similar to mature female rats. Mature male rats excreted the same compounds in an inverse ratio (4.9 and 13.9%, respectively). Results also showed the half life of apigenin in blood to be 91.8 h, a distribution volume of 259 mL, and a plasmatic clearance of 1.95 mL/h, suggesting apigenin’s slow absorption and elimination in the body.[Citation84] Apigenin as a component in Chrysanthemum morifolium extract have shown to be absorbed in the intestinal mucosa more efficiently as compared to luteolin and also showed slow elimination rate in serum. Apigenin reached highest peak level at 1.1 and 3.9 h after dosing while total recovery in urine and faeces was found to be 16.6 and 28.6%, respectively.[Citation77] This is in supported from the study on human volunteers receiving diet low in flavones and other naturally occurring antioxidants during the 2 weeks of intervention. When this basic diet was supplemented with parsley providing 3.73–4.49 mg apigenin in one of the intervention weeks, the urinary excretion of apigenin was 1.59–409.09 mg/per 24 h as compared to 0–112.27 mg per 24 h on the basic diet. The fraction of apigenin intake excreted in the urine was found to be 0.58 (SE ± 0.16%) during parsley intervention.[Citation85]
Pharmacological properties of apigenin
Apigenin has gained importance in recent years as a beneficial and health promoting agent because of its low intrinsic toxicity and its striking effects on normal versus cancer cells, compared with other structurally related flavonoids.[Citation86] There are numerous research evidences that have shown apigenin’s strong therapeutic potential against a number of diseases (). There is very little evidence to date to suggest that apigenin promotes adverse metabolic reactions in vivo when consumed as part of a normal diet. However, some results have suggested the oxidative stress induced liver damage, which may be due to the activation of multiple genes by apigenin at higher doses in swiss mice.[Citation87] The possible cancer preventive effect of apigenin is due to its potent antioxidant and anti-inflammatory activities.[Citation87,Citation88] It has also been demonstrated that apigenin promotes metal chelation, scavenges free radicals and stimulates phase II detoxification enzymes in cell culture and in vivo tumor models.[Citation22] Apigenin plays an important role in cancer prevention by inducing apoptosis in various cell lines and animal models.[Citation89–Citation92] Indirect support to this assumption is correlated with a study where consumption of flavonoid free diets by healthy human volunteers has been reported to decrease in markers of oxidative stress in blood viz. plasma antioxidant vitamins, erythrocyte superoxide dismutase (SOD) activity and lymphocyte DNA damage commonly associated with enhanced disease risk, suggesting the beneficial effects of flavonoids.[Citation88]
A number of biological effects of apigenin in numerous mammalian systems in vitro as well as in vivo are related to its antioxidant and antigenotoxic effect and its role in scavenging free radicals.[Citation93,Citation94] Apigenin executes its anti-atherogenic effect by inducing oxidized low density lipoprotein (OxLDL)-loaded murine peritoneal macrophages apoptosis. The pro-apoptotic effect of apigenin was partly attributed to down-regulation of plasminogen activator inhibitor -2(PAI-2) through suppressing phosphorylation of AKT at Ser473.[Citation95] It is also known to reduce LPS-induced inflammation in IPEC-J2 non-transformed intestinal epithelial cells by reducing expression of COX-2, IL-8, and TNF-α.[Citation96] Goto et al. suggested that apigenin inhibits osteoblastogenesis, osteoclastogenesis, and also prevents bone loss in ovariectomized mice.[Citation97]
Several beneficial properties have been attributed to apigenin and its derivatives, including antioxidant, anti-inflammatory, and anti-carcinogenic effects.[Citation98–Citation100] Apigenin-7-glycoside a derivative of apigenin prevents LPS-induced acute lung injury via downregulation of oxidative enzyme expression and protein activation through inhibition of MAPK phosphorylation.[Citation101] It was earlier shown that apigenin induces a process called autophagia (a kind of cellular dormancy) that may well explain its chemopreventive properties, but at the same time it induces resistance against chemotherapy.[Citation102] Apigenin is a potent competitive inhibitor of CYP2C9 an enzyme responsible for metabolism of many pharmaceutical drugs in the body.[Citation103] Apigenin has been shown to reverse the adverse effects of cyclosporine. Research has been conducted to study the effects of apigenin on the reversal of cyclosporine induced damage and was assessed by immuno-histochemical estimation of bcl-2, and estimation of apoptosis in histological sections.[Citation104]
Rheumatoid arthritis
It is reported that inflammatory cytokines produced by fibroblast-like synoviocytes are involved in joint destruction and inflammation. Apigenin is known to induce ROS production and cause apoptosis through oxidative stress-activated ERK1/2 pathway in fibroblast-like synoviocytes.[Citation105] In addition to this, intake of apigenin has also showed immune-modulating effects triggered by TNF-α in a mouse model of rheumatoid arthritis.[Citation106]
Auto-immune disorders
In a study with SFN-1 mice model for lupus, it was shown that apigenin reduces response of Th1 (T-helper 1) and Th17 cells to major lupus autoantigen and consequently suppresses the lupus B cells ability to produce auto-antibodies. Moreover, apigenin also downregulated the expression of COX-2 and cellular FLICE-like inhibitory protein (c-FLIP) in immune cells and thereby, directing them toward apoptosis.[Citation107] Resistance of T cells to activation-induced cell death (AICD) is associated with autoimmunity and lymphocyte proliferation. Apigenin potentiated AICD by inhibiting NF-κB activation and suppressing NF-κB regulated anti-apoptotic molecules, cFLIP, Bcl-xL, Mcl-1, XIAP, and IAP, but not Bcl-2. Apigenin suppressed NF-κB translocation to nucleus and inhibited I-κBα phosphorylation and degradation in response to T-cell receptor (TCR) stimulation in reactivated peripheral blood CD4 T-cells, as well as in leukemic Jurkat T-cell lines.[Citation108] In a similar study Verbeek et al.[Citation109] demonstrated that flavones luteolin and apigenin inhibit in vitro antigen-specific proliferation and interferon-gamma (INF-γ) production by murine and human autoimmune T-cells.
Hemostasis
In an in vitro study, it was found that 2500 µmol/L of the flavonol quercetin and the flavone apigenin significantly inhibited collagen and ADP-induced aggregation in platelet-rich plasma and washed platelets by approximately 80–97%. However, to test the in vivo role of the two compounds, 18 healthy volunteers were fed with 220 g onions/day providing 114 mg quercetin/d, 5 g dried parsley/day providing 84 mg apigenin/day, or a placebo for 7 days each in a randomized crossover experiment with each treatment period lasting 2 weeks. The results indicate that due to the lower concentrations both the compounds had no significant effect.[Citation110] The mechanism of platelet anti-aggregating activity of apigenin and other flavonoids was studied in vitro by Landolfi et al.[Citation111] The study showed that, flavonoids inhibited platelet aggregation by depressing the cyclooxygenase pathway. In addition, the platelet cyclic AMP response to PGI2 was also reduced via inhibition of adenylyl cyclase.
Anti-viral activity
Apigenin 7-Oβ D-glucopyranoside and apigenin 7-Oβ D- (4′ caffeoyl) glucuronide extracted from medicinal herb Kummerowia striata and Chrysanthemum morifolium, respectively, have shown to induce anti-HIV activity in T-cell line (H9) transfected with HIV-I and HIV-1 (IIIB) infected MT-4 cells, respectively, by imparting strong integrase inhibitory activity.[Citation112,Citation113] Most viral infections can be suppressed by preventing viral internal ribosomal entry site (IRES) mediated translational activity and by modulating cellular c-Jun N-terminal kinase (JNK) pathway. Lv et al.[Citation114] and Qian et al.,[Citation115] in separate studies, found that apigenin restricts enterovirus or Hand, Foot, and Mouth (HFM) virus induced HFM disease (HFMD) by inhibiting cellular JNK pathway and by preventing viral entry to IRES, which is otherwise essential for viral replication. Critchfield et al.[Citation116] examined the anti-HIV effect of apigenin and other related flavonoids onTNF-α induced OM-10.1 cells. The compounds have shown varied therapeutic indices in response to their inhibition to HIV expression. They also concluded that inhibition of NF-кB pathway was not associated with the inhibition of viral replication, as the same was not downregulated by flavonoid treatment.
Behavior
Many neuropsychological disorders are due to abnormal synaptic activity which, in turn, is associated with altered monoamine transporters activity. A study with two flavonoids luteolin and apigenin isolated from Perilla frutescent on Chinese hamster ovary (CHO) cells and wild type dopaminergic cell lines have shown to act as monoamine transporter antagonists and help in improving several hyper-monoaminergic neuropsychological disorders by upregulating monoamine transporter activity.[Citation68] A behavioral study conducted on rats after intraperitoneal administration of apigenin and chrysin to them showed that chrysin had anxiolytic effect when injected at a dose of 1 mg/mL. While, at the same dose, apigenin had no effect. Chrysin might mediate its effect by binding to gama amino butyric acid-bezodiazepine (GABA-bezodiazepine) receptors. This was cross-examined by treating mice with flumazenil (benzodiazepine antagonist), which prevented the anxiolytic effect by blocking the benzodiazepine receptors and, thus, confirming the interaction of chrysin with GABA receptor to mediate anxiolytic effect.[Citation117] Similar results were obtained in another study, in which apigenin as methanolic extract was derived from the dried flowers of Matricaria chamomilla and which when injected intra peritoneally in rats was found to reduce locomotory activity but, did not demonstrate any anxiolytic, myorelaxant, or anticonvulsant activities in them. The inhibitory activity of apigenin on locomotory behavior seems to be ascribed to its interaction with GABA-benzodiazepine receptors, which was confirmed by the use of benzodiazepine receptor antagonist Ro 15-1788.[Citation52] However, as shown from the study by Viola et al.,[Citation11] the extract from the flowers of Matricaria recutita also having apigenin as a component, can induce anxiolytic effect mediated by binding to the same receptors. Thus, effect of apigenin on the behavioral activity of animal models is a bit controversial.
Ischemia reperfusion injury
Many studies were performed earlier to investigate the role of apigenin in preventing the Ischemia reperfusion injury in different tissues of model organisms like acute transient focal cerebral ischemia-reperfusion injury and spinal cord injury in rats and mice,[Citation119–Citation121] lung ischemia-reperfusion injury in rats,[Citation122] myocardial ischaemia/reperfusion injury in Langendorff-perfused rat hearts,[Citation123,Citation124] oxygen-glucose deprivation/reperfusion induced injury in rat hippocampal neurons,[Citation125] and liver ischemia reperfusion injury in rats,[Citation126] by inhibiting the production of nitric oxide (NO), prostaglandin E (PGE), Fas protein for apoptosis, glutathione peroxidise (GSH-Px), SOD, malondialdehyde (MDA), tumor necrotic factor alpha (TNF-α), etc. Although positive results were obtained with apigenin, no strong mechanism was elucidated until the very recent study by Hu et al.[Citation55] These researchers in their current study demonstrated the role of apigenin with possible mechanism in isolated rat heart model of ischemia-reperfusion. The result suggests that apigenin conferred a cardioprotective effect, showed an improved ischemic cardiac functional recovery, decreased myocardial infarct size, and reduced activities of creatine kinase isoenzyme and lactate dehydrogenase (LDH) in the coronary flow. These changes may rely on less number of cardiomyocytes undergoing apoptosis due to reduced activity of caspase-3, up-regulation of the anti-apoptotic protein Bcl-2, down-regulation of the pro-apoptotic protein Bax and inhibition of p38 MAPK (mitogen activated protein kinase) signaling during I/R. In another study, Li et al.[Citation101] demonstrated that apigenin-7-glycoside prevents lipopolysaccharide (LPS)-induced acute lung injury via downregulation of inducible nitric oxide synthase (iNOS) and COX 2 expression andprotein activation through inhibition of MAPK phosphorylation and NF-кB pathway. A number of deaths occur in the form of brain injury caused due to subarachnoid haemorrhage. In such cases there occurs increased expression of toll like receptor 4 (TLR-4), necrotic factor kappa B (NF-кB), and their downstream pro-inflammatory cytokines in CNS. Apigenin could suppress the activation of TLR-4 induced by subarachnoid haemorrhage and inhibit apoptosis of cells in the cortex.[Citation127]
Anti-inflammatory and anti-oxidant activity
Many flavonoids are now known to be the selective, as well as non-selective, inhibitors of COX, LOX, PLA, and NOS. These molecules are the major contributors in inducing inflammation. Some flavonoids inhibit only a single inflammatory enzyme while others inhibit a series of enzymes. For example, flavones such as luteolin, apigenin are known to suppress iNOS and COX 2 expression in contrast flavanols like quercetin and myricetin are preferential LOX and PLA 2 inhibitors, with quercetin also showing the ability to inhibit NOS. Moreover, where on one hand, flavonoids (quercetin, myrecetin, kaempferol, scutellarin) and biflavonoids (ochreflavone, amentoflavone, ginkgetum, and isognetum) are inhibitors of PLA, 2 amitoflavone, and apigenin, on other hand, showed no inhibition to PLA 2.[Citation128] Flavones better activity in inhibiting NO production and iNOS and COX inhibition is attributed to their C-2,3 double bond and substitution of hydroxyl groups on A and B rings, respectively.[Citation128] Apigenin, quercetin, and morin were also found to inhibit NO production in LPS induced and INF-γ activated C6 astrocytes and LPS induced RAW 264.7 cells, not by inhibiting the iNOS activity, but rather strongly suppressing the expression of iNOS.[Citation129,Citation130] In addition to iNOS/COX 2, several cytokines IL-1β, IL-6, INF-γ, IL-4, IL-5, TNF-α, MCP-1α (Monocyte chemotoactic protein), MIP-1α (Monocyte inflammatory protein), and ICAMS are also associated with the inflammatory responses. The possible role of apigenin to inhibit the expression of several cytokine genes has been shown to be associated with a number of protein kinases involved in signal transduction including PKC, ERK, and MAPK. Through the inhibition of these molecules, DNA binding capacity of transcription factors such as NF-κB, Fos-Jun, or AP-1 is regulated.[Citation128] Although, some of the signaling molecules inhibited by apigenin are already been demonstrated many others are yet to be deciphered.[Citation131] A possible pathway for inflammatory modulation induced by apigenin is shown in . In one of the studies Reuter has shown that ROS induces the activation of a number of transcription factors like NF-κB, AP-1, p53, HIF-1α, PPAR-γ, β-catenin/Wnt, STAT-3, Sp-1, and Nrf2, most of which are also known to be involved in cancer and inflammatory diseases. Activation of these transcription factors can lead to the expression of more than 500 different genes, including those for growth factors, inflammatory cytokines, chemokines, cell cycle regulatory molecules, and anti-inflammatory molecules.[Citation131,Citation132] Apigenin has shown its role in modulating the inflammatory pathways by interacting with these transcription factors. Inhibition of TNF-α induced NF-κB, CCL2/MCP-1, and CXCL 1/KC expression by apigenin has been reported. NF-κB is responsible for the activation of transcription factors involved in COX 2 and iNOS synthesis. The inhibition of NF-κB is achieved through the inhibition of LPS induced IκB kinase activity in mouse macrophages.[Citation133] However, apigenin had no effect on the degradation of IκB proteins and nuclear translocation and DNA binding activity of NF-κB p65.[Citation210] Possible role of apigenin in inducing anti-inflammatory affect is shown in .
Figure 9. Possible role of apigenin in inducing anti-inflammatory affect by modulating the expression of cytokines and other inflammatory molecules at both transcriptional and post transcriptional level. COX 1/2 (Cycloxygenase 1/2), LOX (Lipoxygenase), HO-1 (Haeme oxygenase 1), PLA (Prostaglandins), IL (Interleukins), TNF-α (Tumor necrotic factor alpha), iNOS (inhibitor of nitric oxide synthase), INF-γ (Interferon gamma), XIAP (X-linked inhibitor of apoptosis protein), c-FLIP (Cellular FLICE like inhibitory protein). Lines with arrow heads represent activation while lines without arrow heads represent inhibition.
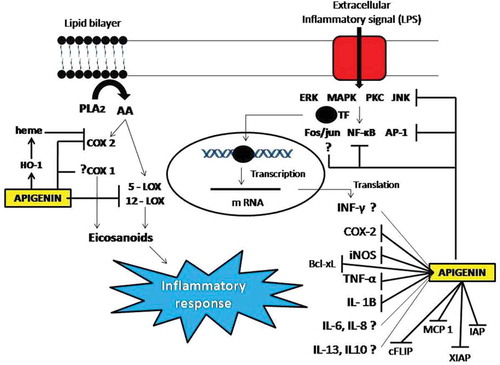
In addition to anti-inflammatory properties apigenin also shows anti-oxidant properties mediated by anti oxidant enzymes like SOD, GSH-Px, catalase (CAT), NOS, glutathione reductase (GR) and also by reduced glutathione (GSH). Human subjects supplemented with parsley providing 3.73–4.49 mg apigenin for 2 weeks resulted in increased levels of GR and SOD in comparison to group supplemented with low flavones rich diet.[Citation85] One of the studies demonstrated that apigenin at low concentrations (10, 20, and 40 mg/kg b.w) provides protection to rat livers against reactive oxygen species (ROS) induced oxidative damage by reducing lipid peroxidation (LPO) and membrane protein damage and also by reducing the secretion of blood serum enzyme markers viz; LDH, ALP, alanine amino transferase (ALT), and aspartate transaminase (AST).[Citation93] shows the effect of apigenin on the regulation of various inflammatory and antioxidant molecules.
Table 1. Effect of apigenin on the regulation of various inflammatory molecules.
However, apigenin administration (intragastric) at concentrations as high as 468 and 936 mg/kg b.w had inverse effect on rat livers, showing that apigenin can induce oxidative stress at very high doses. This might be conferred from reduction in the levels of SOD, CAT, GSH-Px, and total antioxidant capacity (T-AOC).[Citation134] Lotito and Frei,[Citation135]and Panes et al.[Citation136] demonstrated that flavones apigenin and its other counterparts inhibit expression of intracellular adhesion molecules (ICAM), thereby providing protection against inflammation in different organs including atherosclerosis in human aortic endothelial cells. Authors also suggested that 5,7-dihydroxyl substitution of a flavonoid A ring; 2,3 double bond and 4-keto group of the C ring were the main structural requirements for inhibition of adhesion molecule expression. Hydroxyl substitutions only on B and C rings but not on ring A were essential for antioxidant activity. Chemosensitivity assays with control transfected and multi-drug resistant protein (MRP1)-transfected HeLa cell lines showed that the IC50 values for apigenin, naringenin, genistein, and quercetin were similar, demonstrating that overexpression of MRP1 does not confer resistance to these bio-flavonoids. The results from the study suggest that flavonoids stimulate MRP1-mediated GSH transport by increasing the apparent affinity of the transporter for GSH and thus conferring antioxidant property to them.[Citation137,Citation138] Xanthineoxidase causes gout and is responsible for oxidative damage to living tissues. Lin et al.[Citation139] studied the effect of various flavonoids including apigenin on xanthine oxidase (XO) induced oxidative stress in human promyelocytic leukemic (HL-60) cells. Few studies have shown to have no anti-inflammatory effect on application of some selected flavonoids, which also include apigenin as a therapeutic agent. In one of the researches, mice treated with selected flavonoids showed no reduction in the serum uric acid levels. As far as XO activity is concerned, it was not significantly affected in vitro but significant changes were found in in vivo systems.[Citation140] Among all flavonoids studied, apigenin was found to be the most potent inhibitor of XO. Other studies indicate that apigenin, chrysin, and luteolin dose-dependently up-regulated the protein expression of heme oxygenase 1 (HO-1) and glutamate cysteine ligase (GCL) catalytic (GCLC) and modifier subunit (GCLM). They also increased the intracellular glutathione (GSH) content and the ratio of GSH to oxidized GSH (GSSG) by activating extracellular signal-regulated protein kinase 2 (ERK2), nuclear factor erythroid 2-related factor 2 (Nrf2), both of which are in turn responsible for activating the TRE and EpRE genes involved in GCL synthesis.[Citation141,Citation154] The magnitude of HO-1 induction after apigenin uptake and the wide distribution of this enzyme in systemic tissues coupled with the intriguing biological activities of the catalytic byproducts of heme, i.e., carbon monoxide (CO), iron (Fe), and bilirubin (bil) makes it an important molecule for stress response, as on one hand CO is known to have anti-inflammatory and anti-apoptotic affect, on the other, ferritin and bilirubin act as antioxidants (bilirubin enhances SOD and CAT activity and inhibits adiponectin).[Citation142,Citation143]
Thus, the literature searched suggests that apigenin reduces oxidative stress induced inflammation in tissues by modulating various oxidative stress markers, blood enzyme markers, interleukins, and expression of several other related enzymes. However, in contrast to the studies discussed above, some reports have showed oxidative damage and hepatic architecture loss in rats exposed to sub-chronic or higher doses of apigenin.[Citation144] The antioxidant activity of apigenin could be mediated through the increased production of antioxidant enzymes. Based on the observations shown in of this section and antioxidant properties given in other sections of this review, a sketch of oxidative mechanism and possible apigenin targets are depicted in .
Figure 10. Antioxidant enzyme targets of apigenin at both transcriptional and post transcriptional level. AST (Aspartate aminotransferase), ALT (Alanine aminotransferase), ALP (Alkaline phosphatase), LDH (Lactate dehydrogenase) GSH (Glutathione), GST (Glutathione S transferase), GPx (Glutathione peroxidase), GR (Glutathione reductase), P.Red (Peroxireductase), CAT (Catalase), SOD (Superoxide dismutase, 4-HNE (4 hydroxy 2 nonenal), LPO (Lipid peroxidation), GCL (Glutamylcysteine ligase), GS (Glutathione synthase), TF (Transcriptional factors). Lines with arrow heads represent activation while lines without arrow heads represent inhibition.
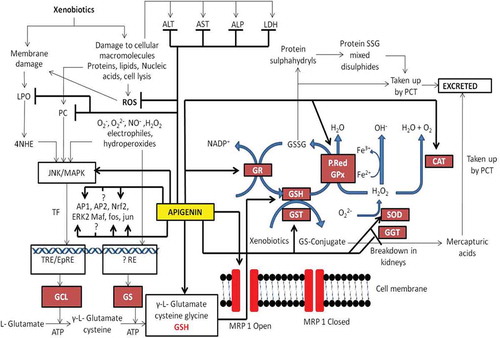
Anti-toxicant
Numerous studies have been performed using apigenin and its analogues to demonstrate its protective effect in vivo and in vitro. The results from one of the studies suggested that apigenin ameliorates cisplatin-induced apoptosis in human renal proximal tubular epithelial (HK-2) cells through reduction of p53 activation and promotion of PI3K/Akt pathway.[Citation160] LPS is known to disturb the anti-oxidative mechanism, impair mitochondrial redox activity and consequently induce inflammation in the brain. Farkas et al.[Citation96] demonstrated that LPS-induced inflammation in IPEC-J2 non-transformed intestinal epithelial cells can be reduced on application of apigenin and its methylated derivative (apigenin-trimethylether) by down-regulating the expression of IL-6, IL-8, COX-2, and extracellular H2O2. Endoplasmic reticulum (ER) stress induced by tunicamycin also brings about mitochondrial alterations in adipocytes. These mitochondrial alterations were significantly reduced on treatment of cells with apigenin and quercitin.[Citation161] From the study by Hussein et al.,[Citation162] it was concluded that apigenin reduces LPS induced COX-1 and COX-2 expression, LPO, NO, and monoamines synthesis along with an increase in serum paraoxonase activity (PON-1). Another study has shown that trinitrobenzene sulphonic acid and dextran sulphate sodium induced intestinal inflammation (Colitis) in rat models can be minimized on their pre-treatment with a soluble form of apigenin, namely apigenin K. There was a reduction of 30 and 21% in myeloperoxidase (MPO) and ALP activity, respectively, in apigenin K treated rats, in addition to its ameliorating effect on internal morphological damage and reduction in the expression of immunological inflammatory marker like TNF-α, TGF-β, IL-6, and ICAM-1.[Citation163] Protective effect of apigenin against some of the numerous toxic compounds has been listed in .
Table 2. Protective effect of apigenin against some of the numerous toxic compounds. Carbon tetrachloride (CCl4), N-nitrosodiethylamine (NDEA), trinitrobenzene-sulfonic acid (TNBS), dextran sulphte sodium (DSS).
Antigenotoxic
Genotoxicity is the property of chemical agents that alters the genetic information within a cell causing mutations, which may lead to cancer. Every cell has the property to prevent chemical induced genotoxicity either by DNA repair mechanisms or diverting cell toward apoptosis. However, the damage may not always be fixed leading to mutations. To evaluate the genotoxic effects of chemical substances in vitro and in vivo, various methods are available differing in their sensitivity, their practicability, and their genetic end-points considered. The most commonly used genotoxic assays include micronucleus assay, sister chromatid exchange, and comet assay. All these assays hold well, when the DNA or chromosomes get fragmented in response to toxicity of a chemical compound but, for other nuclear abnormalities such as nucleoplasmic bridges and nuclear bud formation which are also the biomarkers of genotoxic events and chromosomal instability, recently developed method called cytokinesis block micronucleus cytome assay is used. Another widely applied and one of the oldest methods employed for in vitro testing is Ames test, which employs the estimation of number of mutant revertants in the concerned cell culture after the application of test compound. Drosophila wing spot test is also a simple method to determine genotoxicity without involving cellular processing. A method which provides results with better resolution is fluorescent in situ hybridization (FISH). By this method it can be determined whether the chemical employed for genotoxic test is aneugenic or clastogenic. The protective effect of apigenin against various genotoxic compounds has been widely studied. Variable parameters are use by different research groups to detect the protective effect of apigenin as a parent compound or as plant extracts against numerous genotoxic compounds in vivo and in vitro[Citation93,Citation171,Citation172] (). Apigenin may also act as a pro-oxidant, genotoxicant, or as an inhibitor of key enzymes to produce clastogenic effect in cancer cell lines, depending upon the levels consumed[Citation173,Citation174] ().
Table 3. Antigenotoxic potential of apigenin on various cell lines and animal models against different genotoxic agents.
Table 4. Apigenin as pro-oxidant, genotoxicant, or as an inhibitor of key enzymes to produce clastogenic effect in cancer cell lines.
Alzheimer’s disease
Choi et al.[Citation156] treated LPS induced RAW 264.7 mice macrophage cells with apigenin and its two c-glycosylated derivatives, vitexin, and iso-vitexin. From the study, iso-vitexin was found to be more powerful inhibitor of rat eye lense aldolase reductase (RLAR), human recombinant aldolase reductase (HRAR), acetylcholine esterase (AChE), and butyrylcholine esterase (BChE) enzymes and advanced glycation end products (AGE). On the other hand, vitexin showed to inhibit only protein tyrosine phosphatase 1B (PTP 1B). In contrast, both these derivatives apigenin showed powerful anti-inflammatory activity by inhibiting NO production and iNOS and COX-2 expression. In this context, vitexin and iso-vitexin were found to be inactive. Using invitro screens, AChE, and BChE inhibitory activities of various phenolic compounds including apigenin were analyzed by Orhan et al.[Citation188] Upon evaluating various polyphenolic compounds at a concentration of 1 mg/mL, using microplate reader assay it was found that only quercetin had inhibitory effect against AChE while, genistein, luteolin-7-O rutinoside, and silibinin had moderate inhibitory effect on BChE and apigenin exerted no inhibitory effect on either of the enzymes. In an in vivo study, 3-month oral treatment with apigen inrescued learning deficits and relieved memory retention in APP/PS1 double transgenic AD mice. Apigenin has shown its ameliorating effect not only by reducing the oxidative stress (by increasing SOD and GSH-Px activity), but also by preventing Aβ burden through down-regulating BACE1 and β-CTF levels.[Citation189] Apigenin treatment to copper-induced Aβ-toxicity in neuronal cells showed reduction in neuronal apoptosis, regulated redox imbalance, and preserved mitochondrial function by blocking ROS induced p38 mitogen activated protein kinase (p38 MAPK) and stress activated protein kinase (SAPK)/c-JNK pathways.[Citation190] ER is the main organelle for the synthesis and processing of almost all kinds of proteins including Aβ peptides responsible for developing AD. It is suggested that stress in ER may activate ER specific pathways causing the accumulation of misfolded proteins within the ER lumen. Many studies have proposed that neuronal death in AD arises from dysfunction of the ER. Thus, these findings lay insights for therapeutic strategies that can be made to target ER for treating AD.[Citation191]Apigenin (IC50 =38.5 µmol L−1) inhibits the activity of β-secretase (the principle enzyme responsible for break releasing the β-amyloid fragment) in a concentration dependent manner in both cell-free and cell-based assay systems.[Citation192,Citation193]
Parkinson’s disease (PD)
Expression of parkinsonism biomarkers, i.e., brain derived neutrotrophic factor (BDNF) and tyrosine hydroxylase (TH) were decreased and glial fibrillary acid protein (GFAP) was increased in mice treated with 1-methyl-4-phenyl-1,2,3,6-tetrahydropyridine (MPTP). On the other hand, treatment of MPTP induced mice group with different doses of apigenin, luteolin, and bromocriptine brought about significant reversal in the levels of the previoulsy mentioned PD biomarkers by reducing oxidative damage, neuro inflammation, and microglial activation.[Citation194]
Immunomodulatory effect
It is well known that IgE antibody produced by mast cells mediate allergic reactions in living systems. Yano et al.[Citation195] demonstrated that the generation of IgE can be suppressed on pre-feeding C57BL/6N mice, with diet rich in apigenin. Moreover, the expression of regulated upon activation normal T cell expressed and secreted (RANTES) and soluble tumor necrosis factor receptor I (sTNFRI) in mice sera was also down regulated in apigenin supplemented diet. Studies on the murine models of asthma have also shown that apigenin can decrease the degree of the inflammatory cell infiltration, airway hyper-responsiveness, and total immunoglobulin E levels in the serum and also diverting the allergic response towards Th 1 profile.[Citation211]
Miscellaneous
TNF-α has recently been identified to be a mediator of retinal ganglion cell (RGC) death, while glial cells are relatively protected against this death stimulus. Exposure of RGCs to TNF-α is thought to contribute to RGC apoptosis. In one study, MTT assays showed that apigenin significantly inhibited the decrease of RGC viability induced by TNF-α in a dose-dependent manner. Pretreatment with apigenin prevented TNF-α induced apoptosis through inhibition of caspase-dependent apoptotic pathway and activation of nuclear factor-kappa B.[Citation196] Apigenin has shown to prevent alloxan induced hyperglycaemia, thyroid dysfunction, and LPO in rat models. Apigenin reduces inflammation and imparts its protective effect by reducing LPO and an increasing the activity of cellular antioxidants, such as CAT and SOD, and in glutathione (GSH) content.[Citation159] In a similar kind of study, Crazolli et al.[Citation49] found that apigenin-6-C-β-fucopyranoside and apigenin-6-C-(2″-O-α-rhamnopyranosyl)-β-fucopyranoside, derivatives of apigenin induced glucose uptake via alternate pathways in rats treated with insulin signal inhibitors like wortmannin, an inhibitor of phosphoinositide 3-kinase (PI3K), RO318220, an inhibitor of protein kinase C (PKC), PD98059, an inhibitor of mitogen-activated protein kinase (MAK), and HNMPA(AM)3, an inhibitor of insulin receptor tyrosine kinase.
One study demonstrated that apigenin inhibited ovalbumin induced asthma in mice models characterized by increase in airway resistance and eosinophil count. In addition, levels of interleukin (IL)-6, TNF-α, and IL-17A were also suppressed after treatment with apigenin.[Citation197] Apigenin and tangeretin enhance gap junctional intercellular communication in rat liver epithelial cells which could be one of the major mechanisms responsible for apigenin’s anti-tumor promoting action in vivo.[Citation198] From their study with apigenin treatment on isolated rat atria, Lorenzo et al.[Citation199] concluded that apigenin possesses the property to increase the atrial rate, by minimizing noradrenaline uptake and by reducing monoamine oxidase activity. A number of amyloid related diseases exist in nature, which are due to the deposition of proteins either inside or outside the cell. Inhibiting or reversing amyloid aggregation via the use of small molecules is proposed as two useful approaches in hampering the development of these diseases. In one such approach, Amini et al.[Citation200] have shown that apigenin reduces human insulin fibrillation in vitro and protects SK-N-MC cells against insulin induced amyloids. A reduction in the expression of cellular adhesion molecules (CAMs) viz, ICAM, vascular cell adhesion molecules (VCAM) and E-selectins was observed in the culture of vascular endothelial cells when treated with apigenin, dissolved in the medium as such and after being processed through hepatocytes conditioned medium. The results of this study also suggested that the C ring double bond, the keto group and the A ring 5,7-dihydroxyl groups but not the B ring are essential requirements for the flavonoids to function as inhibitors of CAM expression.[Citation135] Choi et al.[Citation201] investigated the effect of apigenin against ovalbumin (OVA) induced asthma in female BALB/c mice. Results obtained reveal that administration of apigenin in mice leads to a reduction in the expression of MMP-9 and GATA-3 mRNA and also in Th2 cytokine (IL-4 and IL-5) levels in BAL fluid. Thus, suggesting its role in ameliorating pathogenesis of OVA induced asthma in mice. Hossein et al.[Citation202] have demonstrated that apigenin can impart anti-diabetic affect in animal models by modulating GLUT4 and CD38 trans-membrane proteins. The flavonols (fisetin, quercetin, and 3,3′,4′trihydroxyflavone) and flavones (apigenin, chrysin, and luteolin) are known to show concentration dependent vasorelaxant effect in isolated rat thoracic aorta. Moreover, all the flavonoids have shown a similar potency in inhibiting contraction caused by the influx of extracellular CaCitation2+, except for 3,3′,4′trihydroxyflavone.[Citation203,Citation204] In one of the recent studies apigenin has shown to impart protection against Leishmaniasis caused by leishmanial parasite by generating ROS and inducing an extensive swelling in parasite mitochondria, leading to an alteration of the mitochondrial membrane potential, rupture of the trans-Golgi network, and cytoplasmic vacuolization.[Citation205] One of the studies has shown that apigenin at a concentration as high as 25 mg/kg b.w. resulted in impaired reproductive health of male mice. The adverse effect on the reproductive system was in terms of reduced sperm density and sperm motility, which in turn is due to slow proliferation of germ cells and blockage of spermatogonia in G0-G1 stage.[Citation206]
Apigenin extract from plant Thevetia peruviana at concentrations 3.65 mg/L for 24 h, 2.98 mg/L for 48 h, 1.63 mg/L for 72 h, and 0.73 mg/L for 96 h showed molluscicidal effect on snail (Lymnaea acuminata) without affecting the non-target species Channa punctatus. Exposure to sub-lethal doses of apigenin (3.65 mg/L, 24 h) caused significant (p ˂ 0.05) alteration in carbohydrate and nitrogen metabolism in nervous, hepatic, pancreatic, testicular, and ovarian tissues in snail. However, at the same time the non-target fish species when exposed to the same doses for 96 h showed similar pathological hallmarks, which were withdrawn after 7 days of treatment. So, as far as this study is concened direct conclusion cannot be reached, suggesting the use of apigenin as molluscicide.[Citation207]
Zhang et al.[Citation208] demonstrated that apigenin could promote the type-I and type-III collagen synthesis of dermal fibroblasts in vitro as well as in vivo by activating Smad2/3 pathways, without affecting cell viability and survival. This suggested the use of apigenin for esthetic and reconstructive skin rejuvenation and delaying ageing signs.[Citation208] One report has suggested that Chrysanthemum morifolium extract containing apigenin as an active compound prevented oxazolone and phorbol 12-myristate 13-acetate (TPA) induced acute allergic contact dermatitis and acute irritant contact dermatitis, respectively, in murine rat models. This was evidenced by significant reduction in trans-epidermal water loss, lowered skin surface pH, and increased stratum corneum hydration. Although, proper mechanism for the protective effect was not provided it may be thought that Smad2/3 signaling pathways may be involved in skin recovery as suggested in the previous study.[Citation209]
Anti-tumor and anti-cancer
There are number of studies suggesting the protective role of apigenin against various types of cancers. A brief description of the protective role of apigenin againstvarious cancer cell lines and experimental animal models is shown in .
Table 5. Protective role of apigenin among various cancer cell lines and in experimental animal models.
An overview of molecular targets of apigenin
In one of the recent studies about 160 high confidence candidate apigenin target molecules were identified which were divided into three functional categories: GTPase activation, membrane transport, and mRNA metabolism/alternative splicing.[Citation314] Other research papers have also revealed a number of apigenin targets, summarised in given below.
Table 6. Molecular targets of apigenin.
Apigenin drug delivery systems
Numerous studies are performed to develop and evaluate a novel delivery system for apigenin. In one of the recent studies Shen et al.[Citation148] and Vanic[Citation315] demonstrated that the efficiency of apigenin encapsulation increases with an increase in the amount of phospholipids in ethosome formulations. Ethosomes are small hydrophobic molecules formed by the combination of phospholipids (lipoid S 75) and short chain alcohols (propylene glycol and ethanol). Apigenin loaded ethosomes showed effective targeting on mice skin tumourigenesis induced by UV-B radiations in both in vivo and in vitro models. Apigenin’s solubility in water was increased by 148 times, thereby increasing its protective efficiency when used against HepG2 and MCF-7 cancer cell lines in the form of polymeric micelles. Moreover, the in vitro drug release study showed nearly 84% apigenin release from the micelles within 36 h, marking its sustainable release. The micelles were made up of either pluronic p123 + solutol HS15 having a diameter of 16.9 nm or 60% Cremophor EL + 30% Transcutol HP and 10% capryol 90 having a diameter of 17.1 nm.[Citation316,Citation317] In another study pre-cutaneous absorption of apigenin was investigated in three vehicles viz; DMSO (D) alone, acetone+DMSO(A/D; 4:1), and polypropylene glycol+DMSO (PG/D; 4:1). Apigenin absorption in mouse skin in vitro followed the order of A/D > D > PG/D. However, sub-tissue distribution analysis showed that DMSO deliverd more apigenin in epidermis than A/D while, A/D deposited more apigenin in stratum corneum. In an in vivo study both DMSO and A/D showed saturation kinetics while apigenin in PG/D showed very low absorption in initial periods of the experiment which was later increased but well below saturable limits. In further study, the same group of researchers demonstrated that 12-O-tetradecanoylphorbol-13 acetate (TPA) induced ornithine decarboxylase (ODC) activity in dorsal skin of mouse cannot be inhibited by apigenin (5 µmol) in A/D delivered from abdominal skin. Thus, they suggested that topical delivery of apigenin should target the local affected tissue for better activity.[Citation247,Citation248] Sen et al.[Citation318] reported that simultaneous administration of apigenin along with 5-fluorouracil (clinically approve drug), loaded in a single liposome can successfully impart strong anti-neoplastic and anti-tumorigenic effect in nude mice xenograft model, by overcoming the problem of drug resistance and 5-fluorouracil associated toxicity. Carbon nanopowder (CNP) solid dispersion is another much improved drug delivery system developed by Ding et al.[Citation319] Here the mixture of CNP and apigenin in ratio 6:1 has shown to tremendously improve the pharmacokinetic properties and bioavailability of the drug (apigenin) in both in vivo and in vitro systems. The efficiency of the drug was increased by 275% as compared to treatment with apigenin alone. Looking at the future aspects of better drug delivery systems the organogels prepared from lecithin (phopholipid) could also be used to deliver the drugs (bioactive agents) to the target sites. Such kind of gels can thus be used for treating disorders like skin aging, skin cancers, etc.
Conclusion
All evidences gathered so far clearly indicate that apigenin protects against cancer, cardiovascular diseases, arthritis, and diabetes, the most common ailments across the world. This compound has also shown preventive as well as therapeutic effects against Alzheimer’s disease, PD, AIDS, auto-immune disorders, and reperfusion injury. In addition behavioural changes, anti-genotoxic, and anti-inflammatory effects are also attributed to apigenin, against a number of toxic compounds. Some of the studies have established apigenin’s potential in in vitro cultures, while some in animal models. Human trials are only performed in the form of dietary supplements. Further testing of apigenin in humans is underway to confirm these observations. Apigenin’s quick absorption in intestine and slow elimination increases its bioavailability thus, making it a good therapeutic agent. The discovery of new drug delivery systems like micelles, liposomes, nanocomposites, and others may aid in further enhancing the potency of such drugs in future. Although, it is not clearly understood of how apigenin produces its therapeutic effects, but they may probably be mediated through antioxidant and anti-inflammatory actions of apigenin. More than two dozen different cellular proteins and enzymes have been identified to be the molecular targets of apigenin. High throughput ligand-interacting technology and microarray technology have begun to reveal more molecular targets and genes affected by apigenin.
References
- Rusznyak, S.P.; Szent-Gyorgyi, A. Vitamin P as Flavonoids. Nature 1936, 138, 27.
- Kuo, S.M. Dietary Flavonoid and Cancer Prevention: Evidence and Potential Mechanism. Critical Reviews in Oncogenesis 1997, 8, 47–69.
- Patel, D.; Shukla, S.; Gupta, S. Apigenin and Cancer Chemoprevention: Progress, Potential and Promise (Review). International Journal Oncology 2007, 30, 233–246.
- Bravo, L. Polyphenols: Chemistry, Dietary Sources, Metabolism, and Nutritional Significance. Nutrition Reviews 1998, 56, 317–333.
- Crozier, A.; Del-Rio, D.; Clifford, M.N. Bioavailability of Dietary Flavonoids and Phenolic Compounds. Molecular Aspects of Medicine 2010, 31, 446–467.
- Ross, J.A.; Kasum, C.M. Dietary Flavonoids: Bioavailability, Metabolic Effects, and Safety. Annual Review of Nutrition 2002, 22, 19–34.
- Middleton, E. The Flavonoids, Trends in Pharmaceutics. Science 1984, 5, 335–380.
- Croft, K.D. The Chemistry and Biological Effects of Flavonoids and Phenolic Acidsa. Annals of the New York Academy of Sciences 1988, 854, 435–442.
- Giannasi, D.E.; Harborne, J.B. The Flavonoids: Advances in Research Since 1980, Chapmann and Hall, 1988. ISBN 978-0-412-28770-1, DOI 10.1007/978-1-4899-2913-6.
- Harvsteen, B. Flavonoids, a Class of Natural Products of High Pharmacological Potency. Biochemical Pharmacology 1983, 32, 1141–1148.
- McKay, D.L.; Blumberg, J.B. A Review of the Bioactivity and Potential Health Benefits of Peppermint Tea (Mentha Piperita L.). Phytotherapy Research 2006, 20, 619–633.
- Bevilacqua, L.; Buiarelli, F.; Coccioli, F.; Jasionowska, R. Identification of Compounds in Wine by HPLC—Tandem Mass Spectrometry. Annali di Chimica 2004, 94, 679–690.
- Gerhäuser, C.; Klimo, K.; Heiss, E.; Neumann, I.; Gamal-Eldeen, A.; Knauft, J.; Frank, N. Mechanism-Based in Vitro Screening of Potential Cancer Chemopreventive Agents. Mutation Research 2003, 523, 163–172.
- Gerhauser, C. Beer Constituents as Potential Cancer Chemopreventive Agents. European Journal of Cancer 2005, 41, 1941–1954.
- Hollman, P.H.; Katan, M.B. Dietary Flavonoids: Intake, Health Effects and Bioavailability. Food and Chemical Toxicology 1999, 37, 937–942.
- Ren, W.; Qiao, Z.; Wang, H.; Zhu, L.; Zhang, L. Flavonoids: Promising Anticancer Agents. Medical Research Review 2003, 23, 519–534.
- Cushnie, T.P.; Lamb, A.J. Antimicrobial Activity of Flavonoids. International Journal of Antimicrobial Agents 2005, 26, 343–356.
- Murray, M.T. Quercetin: Nature’s Antihistamine. Better Nutrition 1998, 60, 10.
- Cook, N.C.; Samman, S. Flavonoids—Chemistry, Metabolism, Cardioprotective Effects and Dietary Sources. The Journal of Nutition Biochemistry 1996, 7, 66–76.
- Williams, R.J.; Spencer, J.P.; Rice-Evans, C. Flavonoids: Antioxidants or Signalling Molecules? Free Radical Biology and Medicine 2004, 36, 838–849.
- Tsuchiya, H. Structure-Dependent Membrane Interaction of Flavonoids Associated with Their Bioactivity. Food Chemistry 2010, 120, 1089–1096.
- Middleton, E.; Kandaswami, C.; Theoharides, T.C. The Effects of Plant Flavonoids on Mammalian Cells: Implications for Inflammation, Heart Disease, and Cancer. Pharmacological Reviews 2000, 52, 673–751.
- Chebil, L.; Humeau, C.; Falcimaigne, A.; Engasser, J.M.; Ghoul, M. Enzymatic Acylation of Flavonoids. Process Biochemistry 2006, 41, 2237–2251.
- Narayana, K.R.; Reddy, M.S.; Chaluvadi, M.R.; Krishna, D.R. Bioflavonoids Classification, Pharmacological, Biochemical Effects and Therapeutic Potential. Indian Journal of Pharmacology 2001, 33, 2–16.
- Wang, L.; Lee, I.M.; Zhang, S.M.; Blumberg, J.B.; Buring, J.E.; Sesso, H.D. Dietary Intake of Selected Flavonols, Flavones, and Flavonoid-Rich Foods and Risk of Cancer in Middle-Aged and Older Women. The American Journal of Clinical Nutrition 2009, 89, 905–912.
- Heim, K.E.; Tagliaferro, A.R.; Bobilya, D.J. Flavonoid Antioxidants: Chemistry, Metabolism and Structure-Activity Relationships. The Journal of Nutritional Biochemistry 2002, 13, 572–584.
- Hertog, M.G.; Kromhout, D.; Aravanis, C.; Blackburn, H.; Buzina, R.; Fidanza, F.; Katan, M.B. Flavonoid Intake and Long-Term Risk of Coronary Heart Disease and Cancer in the Seven Countries Study. Archives of Internal Medicine 1995, 155, 381–386.
- Catarino, D.M.; Alves-Silva, J.; Pereira, R.O.; Cardoso, M.S. Antioxidant Capacities of Flavones and Benefits in Oxidative-Stress Related Diseases. Current Topics in Medicinal Chemistry 2015, 15, 105–119.
- Lu, J.; Zhang, Z.; Ni, Z.; Shen, H.; Tu, Z.; Liu, H.; Shi, H. The Non-Additive Contribution of Hydroxyl Substituents to Akt Kinase–Apigenin Affinity. Molecular Simulation 2015, 41, 653–662.
- Li, S; Chou, G.; Hseu, Y.; Yang, H.; Kwan, H.; Yu, Z. Isolation of Anticancer Constituents from Flos Genkwa (Daphne Genkwa Sieb. et Zucc.) Through Bioassay-Guided Procedures. Chemistry Central Journal 2013, 7, 1.
- O’Rourke, C.; Byres, M.; Delazar, A.; Kumarasamy, Y.; Nahar, L.; Stewart, F.; Sarker, S.D. Hirsutanonol, Oregonin and Genkwanin from the Seeds of Alnus Glutinosa (Betulaceae). Biochemical Systematics and Ecology 2005, 33, 749–752.
- Zhao, J.; Dasmahapatra, A.K.; Khan, S.I.; Khan, I.A. Anti-Aromatase Activity of the Constituents from Damiana (Turnera Diffusa). Journal of Ethnopharmacology 2008, 120, 387–393.
- Hu, C.Q.; Chen, K.; Shi, Q.; Kilkuskie, R.E.; Cheng, Y.C.; Lee, K.H. Anti-AIDS Agents, 10. Acacetin-7-O-β-D-Galactopyranoside, an Anti-HIV Principle from Chrysanthemum Morifolium and a Structure-Activity Correlation with Some Related Flavonoids. Journal of Natural Products 1994, 57, 42–51.
- Silva, B.; Oliveira, P.J.; Dias, A.; Malva, J.O. Quercetin, Kaempferol and Biapigenin from Hypericum Perforatum Are Neuroprotective Against Excitotoxic Insults. Neurotoxicity Research 2008, 13, 265–279.
- Cheung, Z.H.; Leung, M.C.; Yip, H.K.; Wu, W.; Siu, F.K.; So, K.F. A Neuroprotective Herbal Mixture Inhibits Caspase-3-Independent Apoptosis in Retinal Ganglion Cells. Cellular and Molecular Neurobiology 2008, 28, 137–155.
- Haytowitz, D.B.; Bhagwat, S.; Harnly, J.; Holden, J.M.; Gebhardt, S.E. Sources of Flavonoids in the US Diet Using USDA’s Updated Database on the Flavonoid Content of Selected Foods. U.S. Department of Agriculture (USDA). Agricultural Research Service, Beltsville Human Nutrition Research Center, Nutrient Data Laboratory and Food Composition Laboratory: Beltsville, MD, 2006.
- Wen, W.; Li, D.; Li, X.; Gao, Y.; Li, W.; Li, H.; Yan, J. Metabolome-Based Genome-Wide Association Study of Maize Kernel Leads to Novel Biochemical Insights. Nature Communications 2014, 5, 1–10.
- Yan, J.; Yu, L.; Xu, S.; Gu, W.; Zhu, W. Apigenin Accumulation and Expression Analysis of Apigenin Biosynthesis Relative Genes in Celery. Scientia Horticulturae 2014, 165, 218–224.
- Wang, Y.; Chen, S.; Yu, O. Metabolic Engineering of Flavonoids in Plants and Microorganisms. Micro Biotech 2011, 91, 949–956.
- Lee, H.; Kim, B.G.; Kim, M.; Ahn, J.H. Biosynthesis of Two Flavones, Apigenin and Genkwanin in Escherichia Coli. Journal of Microbiology and Biotechnology 2015, 25, 1442–1448.
- Meyer, H.; Bolarinwa, A.; Wolfram, G.; Linseisen, J. Bioavailability of Apigenin from Apiin-Rich Parsley in Humans. Annals of Nutrition and Metatabolism 2006, 50, 167–172.
- Barberan, F.A.T.; Gil, M.I.; Tomas, F.; Ferreres, F.; Arques, A. Flavonoid Aglycones and Glycosides from Teucriumgnaphalodes. Journal of Natural Products 1985, 48, 859–860.
- Peng, X.; Zheng, Z.; Cheng, K.W.; Shan, F.; Ren, G.X.; Chen, F.; Wang, M. Inhibitory Effect of Mung Bean Extract and Its Constituents Vitexin and Isovitexin on the Formation of Advanced Glycation End Products. Food Chemistry 2008, 106, 475–481.
- Zhang, Y.; Jiao, J.; Liu, C.; Wu, X.; Zhang, Y. Isolation and Purification of Four Flavone C-Glycosides from Antioxidant of Bamboo Leaves by Macroporous Resin Column Chromatography and Preparative High-Performance Liquid Chromatography. Food Chemistry 2008, 107, 1326–1336.
- Choo, C.Y.; Sulong, N.Y.; Man, F.; Wong, T.W. Vitexin and Isovitexin from the Leaves of Ficus Deltoidea with in Vivo Α-Glucosidase Inhibition. Journal of Ethnopharmacology 2012, 142, 776–781.
- Hattori, S.; Matsuda, H. Rhoifolin, a New Flavone Glycoside, Isolated from the Leaves of Rhus Succedanea. Archives of Biochemistry and Biophysics 1952, 37, 85–89.
- Rao, Y.K.; Lee, M.J.; Chen, K.; Lee, Y.C.; Wu, W.S.; Tzeng, Y.M. Insulin-Mimetic Action of Rhoifolin and Cosmosiin Isolated from Citrus Grandis (L.) Osbeck Leaves: Enhanced Adiponectin Secretion and Insulin Receptor Phosphorylation in 3T3-L1 Cells. Evidence-Based Complementry and Alternative Medicine 2011, 2011, 1–9.
- Chen, L.; Liu, X. Determination of Effective Components in Traditional Chinese Medicines. People’s Medical Publishing House: Beijing, 2009.
- Cazarolli, L.H.; Kappel, V.D.; Pereira, D.F.; Moresco, H.H.; Brighente, I.M.C.; Pizzolatti, M.G.; Silva, F.R.M.B. Anti-Hyperglycemic Action of Apigenin-6-C-β-Fucopyranoside from Averrhoa Carambola. Fitoterapia 2012, 83, 1176–1183.
- Hertog, M.G.; Hollman, P.C.; Katan, M.B. Content of Potentially Anti-Carcinogenic Flavonoids of 28 Vegetables and 9 Fruits Commonly Consumed in the Netherlands. Journal of Agricultural and Food Chemistry 1992, 40, 2379–2383.
- Avallone, R.; Zanoli, P.; Puia, G. Kleinschnitz, M.; Schreier, P.; Baraldi, M. Pharmacological Profile of Apigenin, a Flavonoid Isolated from Matricaria Chamomilla. Biochemical Pharmacology 2000, 59, 1387–1394.
- Svehlı́ková, V.; Bennett, R.N.; Mellon, F.A.; Needs, P.W.; Piacente, S.; Kroon, P A.; Bao, Y. Isolation, Identification and Stability of Acylated Derivatives of Apigenin 7-O-Glucoside from Chamomile (Chamomilla Recutita [L.] Rauschert). Phytochemistry 2004, 65, 2323–2332.
- Kim, M.A.; Kang, K.; Lee, H.J.; Kim, M.; Kim, C.Y.; Nho, C.W. Apigenin Isolated from Daphne Genkwa Siebold et Zucc. Inhibits 3T3-L1 Preadipocyte Differentiation Through a Modulation of Mitotic Clonal Expansion. Life Sciences 2014, 101, 64–72.
- Thieme, H.; Khogali, A. Isolation of Apigenin-6, 8-Bis-C-Beta-D-Glucopyranoside from Leaves of Acacia Farnesianal (L.) Willd]. Die Pharmazie 1974, 29, 352–352.
- Hu, J.; Li, Z.; Xu, L.T.; Sun, A.J.; Fu, X.Y.; Zhang, L.; Jia, Z.P. Protective Effect of Apigenin on Ischemia/Reperfusion Injury of the Isolated Rat Heart. Cardiovascular Toxicology 2014, 15, 241–249.
- Gulluce, M.; Orhan, F.; Yanmis, D.; Arasoglu, T.; Guvenalp, Z.; Dermirezer, L.O. Isolation of a Flavonoid, Apigenin 7-O-Glucoside, from Mentha Longifolia (L.) Hudson Subspecies Longifolia and Its Genotoxic Potency. Toxicology and Industrial Health 2015, 31, 831–840.
- Ribeiro, J.C.; Antunes, L.M.G.; Aissa, A.F.; Darin, J.D.; De Rosso, V.V.; Mercadante, A.Z.; Bianchi, M.D.L.P. Evaluation of the Genotoxic and Antigenotoxic Effects after Acute and Subacute Treatments with Acai Pulp (Euterpe Oleracea Mart.) on Mice Using the Erythrocytes Micronucleus Test and the Comet Assay. Mutation Research 2010, 695, 22–28.
- Tinto, W.F.; John, M.D. Triterpenoids of Jatropha Gossypifolia. Journal of Natural Products 1992, 55, 807–809.
- Subramanian, S.S.; Nagarajan, S.; Sulochana, N. Flavonoids of the Leaves of Jatropha Gossypifolia. Phytochemistry 1971, 10, 1690.
- Kim, D.I.; Lee, T.K.; Lim, I.S.; Kim, H.; Lee, Y.C; Kim, C.H. Regulation of IGF-I Production and Proliferation of Human Leiomyomal Smooth Muscle Cells by Scutellaria Barbata D. Don in Vitro: Isolation of Flavonoids of Apigenin and Luteolin as Acting Compounds. Toxicology and Applied Pharmacology 2005, 205, 213–224.
- Cao, Y.; Chu, Q.; Fang, Y.; Ye, J. Analysis of Flavonoids in Ginkgo Biloba L. and Its Phyto-Pharmaceuticals by Capillary Electrophoresis with Electrochemical Detection. Analytical and Bioanalytical Chemistry 2002, 374, 294–299.
- Milovanović, V.; Radulović, N.; Todorović, Z.; Stanković, M.; Stojanović, G. Antioxidant, Antimicrobial and Genotoxicity Screening of Hydro-Alcoholic Extracts of Five Serbian Equisetum Species. Plant Foods for Human Nutrition 2007, 62, 113–119.
- Tsai, C.H.; Lin, F.M.; Yang, Y.C.; Lee, M.T.; Cha, T.L.; Wu, G.J.; Hsiao, P.W. Herbal Extract of Wedelia Chinensis Attenuates Androgen Receptor Activity and Orthotopic Growth of Prostate Cancer in Nude Mice. Clinical Cancer Research 2009, 15, 5435–5444.
- Chao, W.W.; Lin, B.F. Review Isolation and Identification of Bioactive Compounds in Andrographis Paniculata (Chuanxinlian). Growth 2010, 10, 44.
- Chen, D.; Landis-Piwowar, K.R.; Chen, M.S.; Dou, Q.P. Inhibition of Proteasome Activity by the Dietary Flavonoid Apigenin Is Associated with Growth Inhibition in Cultured Breast Cancer Cells and Xenografts. Breast Cancer Research 2007, 9, R80.
- Lu, X.Y.; Sun, D.L.; Chen, Z.J.; Chen, T. Relative Contribution of Small and Large Intestine to Deglycosylation and Absorption of Flavonoids from Chrysanthemun Morifolium Extract. Journal of Agricultural and Food Chemistry 2010, 58, 10661–10667.
- Das, S.; Das, J.; Samadder, A.; Boujedaini, N.; Khuda-Bukhsh, A.R. Apigenin-Induced Apoptosis in A375 and A549 Cells Through Selective Action and Dysfunction of Mitochondria. Experimental Biology and Medicine 2012, 237, 1433–1448.
- Zhao, G.; Qin, G.W.; Wang, J.; Chu, W.J.; Guo, L.H. Functional Activation of Monoamine Transporters by Luteolin and Apigenin Isolated from the Fruit of Perilla Frutescens (L.) Britt. Neurochemistry International 2010, 56, 168–176.
- Singh, S.K.; Singh, S.K.; Singh, A. Toxicological and Biochemical Alterations of Apigenin Extracted from Seed of Thevetia Peruviana, a Medicinal Plant. Journal of Biology and Earth Sciences 2013, 3, B110–B119.
- Crespy, V.; Morand, C.; Manach, C.; Besson, C. Part of Quercetin Absorbed in the Small Intestine Is Conjugated and Further Secreted in the Intestinal Lumen. American Journal of Physiology 1999, 277, G120–G126.
- Andlauer, W.; Kolb, J.; Furst, P. Absorption and Metabolism of Genistein in the Isolated Rat Small Intestine. FEBS Letters 2000, 475, 127–130.
- Walle, U.K.; Galijatovic, A.; Walle, T. Transport of the Flavonoid Chrysin and Its Conjugated Metabolites by the Human Intestinal Cell Line Caco-2. Biochemical Pharmacology 1999, 58, 431–438.
- Kool, M.; de Haas, M.; Scheffer, G.L.; Scheper, R.J; van Eijk, M.J.T.; Juijn, J.A.; Baas, F.; Borst, P. Analysis of Expression of cMOAT (MRP2), MRP3, MRP4, and MRP5 Homologues of the Multidrug Resistance-Associated Protein Gene (MRP1), in Human Cancer Cell Lines. Cancer Research 1997, 57, 3537–3547.
- Manach, C.; Scalbert, A.; Morand, C.; Remesy, C. Polyphenols: Food Sources and Bioavailability. The American Journal of Clinical Nutrition 2004, 79, 727–747.
- Ng, S.P.; Wong, K.Y.; Zhang, L.; Zuo, Z. Evaluation of the First-Pass Glucuronidation of Selected Flavones in Gut by Caco-2 Monolayer Model. Journal of Pharmacy and Pharmaceutical Sciences 2004, 8, 1–9.
- Hao, X.; Cheng, G.; Yu, J.E.; He, Y. Study on the Role of Hepatic First-Pass Elimination in the Low Oral Bioavailability of Scutellarin in Rats. Pharmazie 2005, 60, 477–478.
- Chen, T.; Li, L.P.; Lu, X.Y.; Jiang, H.D.; Zeng, S. Absorption and Excretion of Luteolin and Apigenin in Rats after Oral Administration of Chrysanthemum Morifolium Extract. Journal of Agriculture and Food Chemistry 2007, 55, 273–277.
- Chen, Z.; Kong, S.; Song, F.; Li, L.; Jiang, H. Pharmacokinetic Study of Luteolin, Apigenin, Chrysoeriol and Diosmetin after Oral Administration of Flos Chrysanthemi Extract in Rats. Fitoterapia 2012, 83, 1616–1622.
- Cai, H.; Boocock, D.J.; Steward, W.P.; Gescher, A.J. Tissue Distribution in Mice and Metabolism in Murine and Human Liver of Apigenin and Tricin, Flavones with Putative Cancer Chemopreventive Properties. Cancer Chemotherapy and Pharmacology 2007, 60, 257–266.
- Das, S.; Rosazza, J.P. Microbial and Enzymatic Transformations of Flavonoids. Journal of Natural Products 2006, 69, 499–508.
- Wang, S.W.; Kulkarni, K.H.; Tang, L.; Wang, J.R.; Yin, T.; Daidoji, T.; Yokota, H.; Hu, M. Disposition of Flavonoids Via Enteric Recycling: UDP-Glucuronosyltransferase (UGT) 1As Deficiency in Gunn Rats Is Compensated by Increases in UGT2Bs Activities. Journal of Pharmacology and Experimental Therapeutics 2009, 329, 1023–1031.
- Scalbert, A.; Williamson, G. Dietary Intake and Bioavailability of Polyphenols. The Journal of Nutrition 2000, 130, 2073S–2085S.
- Gradolatto, A.; Canivenc-Lavier, M.C.; Basly, J.P.; Siess, M.H.; Teyssier, C. Metabolism of Apigenin by Rat Liver Phase I and Phase II Enzymes and by Isolated Perfused Rat Liver. Drug Metabolism and Disposition 2004, 32, 58–65.
- Gradolatto, A.; Basly, J.P.; Berges, R.; Teyssier, C.; Chagnon, M.C.; Siess, M.H.; Canivenc-Lavier, M.C. Pharmacokinetics and Metabolism of Apigenin in Female and Male Rats after a Single Oral Administration. Drug Metabolism And Disposition 2005, 33, 49–54.
- Nielsen, S.E.; Young, J.F.; Daneshvar, B.; Lauridsen, S.T.; Knuthsen, P.; Sandström, B.; Dragsted, L.O. Effect of Parsley (Petroselinum Crispum) Intake on Urinary Apigenin Excretion, Blood Antioxidant Enzymes and Biomarkers for Oxidative Stress in Human Subjects. British Journal of Nutrition 1999, 81, 447–455.
- Gupta, S.; Afaq, F.; Mukhtar, H. Selective Growth-Inhibitory, Cell-Cycle Deregulatory and Apoptotic Response of Apigenin in Normal Versus Human Prostate Carcinoma Cells. Biochemical and Biophysical Research Communications 2001, 87, 914–920.
- Singh, P.; Mishra, S.K.; Noel, S.; Sharma, S.; Rath, S.K. Acute Exposure of Apigenin Induces Hepatotoxicity in Swiss Mice. PLoS One 2012, 7, e31964.
- Kim, H.Y.; Kim, O.H.; Sung, M.K.J. Effects of Phenol-Depleted and Phenol-Rich Diets on Blood Markers of Oxidative Stress, and Urinary Excretion of Quercetin and Kaempferol in Healthy Volunteers. Journal of the American College of Nutrition 2003, 22, 217–223.
- Kaur, P.; Shukla, S.; Gupta, S. Plant Flavonoid Apigenin Inactivates Akt to Trigger Apoptosis in Human Prostate Cancer: An in Vitro and in Vivo Study. Carcinogenesis 2008, 29, 2210–2217.
- Choi, E.J.; Kim, G.H. 5-Fluorouracil Combined with Apigenin Enhances Anticancer Activity Through Induction of Apoptosis in Human Breast Cancer MDA-MB-453 Cells. Oncology Reports 2009, 22, 1533–1537.
- Das, A.; Banik, N.L.; Ray, S.K. Mechanism of Apoptosis with the Involvement of Calpain and Caspase Cascades in Human Malignant Neuroblastoma SH‐SY5Y Cells Exposed to Flavonoids. International Journal of Cancer 2006, 119, 2575–2585.
- Nicholas, C.; Batra, S.; Vargo, M.A.; Voss, O.H.; Gavrilin, M.A.; Wewers, M.D.; Guttridge, D.C.; Grotewold, E.; Doseff, A.I. Apigenin Blocks Lipopoly-Saccharide Induced Lethality in Vivo and Proinflammatory Cytokines Expression by Inactivating NF-κB Through the Suppression of p65 Phosphorylation. The Journal of Immunology 2007, 179, 7121–7127.
- Ali, F.; Naz, F.; Jyoti, S.; Siddique, Y.H. Protective Effect of Apigenin Against N-Nitrosodiethylamine (NDEA)-Induced Hepatotoxicity in Albino Rats. Mutation Research/Genetic Toxicology and Environmental Mutagenesis 2014, 767, 13–20.
- Liu, R.H. Potential Synergy of Phytochemicals in Cancer Prevention: Mechanism of Action. The Journal of Nutrition 2004, 134, 3479S–3485S.
- Zeng, P.; Liu, B.; Wang, Q.; Fan, Q.; Diao, J.X.; Tang, J.; Fu, X.Q.; Sun, X.G. Apigenin Attenuates Atherogenesis Through Inducing Macrophage Apoptosis Via Inhibition of AKT Ser473 Phosphorylation and Downregulation of Plasminogen Activator Inhibitor-2. Oxidative Medicine and Cellular Longevity 2015, 1, 12.
- Farkas, O.; Palócz, O.; Pászti-Gere, E.; Gálfi, P. Polymethoxyflavone Apigenin-Trimethylether Suppresses LPS-Induced Inflammatory Response in Nontransformed Porcine Intestinal Cell Line IPEC-J2. Oxidative Medicine and Cellular Longevity 2015, 1, 10.
- Goto, T.; Hagiwara, K.; Shirai, N.; Yoshida, K.; Hagiwara, H. Apigenin Inhibits Osteoblastogenesis and Osteoclastogenesis and Prevents Bone Loss in Ovariectomized Mice. Cytotechnology 2015, 67, 357–365.
- Dimitrios, B. Sources of Natural Phenolic Antioxidants. Trends in Food Science & Technology 2006, 17, 505–512.
- O’Prey, J.; Brown, J.; Fleming, J.; Harrison, P.R. Effects of Dietary Flavonoids on Major Signal Transduction Pathways in Human Epithelial Cells. 2Biochemical Pharmacology 2003, 66, 2075–2088.
- Škerget, M.; Kotnik, P.; Hadolin, M.; Hraš, A.R.; Simonič, M.; Knez, Ž. Phenols, Proanthocyanidins, Flavones and Flavonols in Some Plant Materials and Their Antioxidant Activities. Food Chemistry 2005, 89, 191–198.
- Li, K.C.; Ho, Y.L.; Hsieh, W.T.; Huang, S.S.; Chang, Y.S.; Huang, G.J. Apigenin-7-Glycoside Prevents LPS-Induced Acute Lung Injury Via Downregulation of Oxidative Enzyme Expression and Protein Activation Through Inhibition of MAPK Phosphorylation. International Journal of Molecular Sciences 2015, 16, 1736–1754.
- Ruela-de-Sousa, R.R.; Fuhler, G.M.; Blom, N.; Ferreira, C.V.; Aoyama, H.; Peppelenbosch, M.P. Cytotoxicity of Apigenin on Leukemia Cell Lines: Implications for Prevention and Therapy. Cell Death and Disease 2010, 1(1), e19.
- Si, D.; Wang, Y.; Zhou, Y.H.; Guo, Y.; Wang, J.; Zhou, H.; Li, Z.S.; Fawcett, J.P. Mechanism of CYP2C9 Inhibition by Flavones and Flavonols. Drug Metabolism and Disposition 2009, 37, 629–634.
- Chakravarthi, S.; Wen, C.F.; Haleagrahara, N. Apoptosis and Expression of bcl-2 in Cyclosporine Induced Renal Damage and Its Reversal by Beneficial Effects of 4’,5’,7’ Trihydroxyflavone. Journal of Analytical Bio-Science 2009, 32, 320–327.
- Shin, G.C.; Kim, C.; Lee, J.M.; Cho, W.S.; Lee, S.G.; Jeong, M.; Cho, J.; Lee, K. Apigenin-Induced Apoptosis is Mediated by Reactive Oxygen Species and Activation of ERK1/2 in Rheumatoid Fibroblast-Like Synoviocytes. Chemico-Biological Interactions 2009, 182, 29–36.
- Kumazawa, Y.; Kawaguchi, K.; Takimoto, H. Immunomodulating Effects of Flavonoids on Acute and Chronic Inflammatory Responses Caused by Tumor Necrosis Factor α. Current Pharmaceutical Design 2006, 12, 4271–4279.
- Kang, H.K.; Ecklund, D.; Liu, M.; Datta, S.K. Apigenin, a Non-Mutagenic Dietary Flavonoid, Suppresses Lupus by Inhibiting Autoantigen Presentation for Expansion of Autoreactive Th1 and Th17 Cells. Arthritis Research and Therapy 2009, 11, R59.
- Xu, L.; Zhang, L.; Bertucci, A.M.; Pope, R.M.; Datta, S.K. Apigenin, a Dietary Flavonoid, Sensitizes Human T Cells for Activation-Induced Cell Death by Inhibiting PKB/Akt and NF-κB Activation Pathway. Immunology Letters 2008, 121, 74–83.
- Verbeek, R.; Plomp, A.C.; van Tol, E.A.; van Noort, J.M. The Flavones Luteolin and Apigenin Inhibit in Vitro Antigen-Specific Proliferation and Interferon-Gamma Production by Murine and Human Autoimmune T Cells. Biochemical Pharmacology 2004, 68, 621–629.
- Janssen, K.P.L.T.M.; Mensink, R.P.; Cox, F.J.; Harryvan, J.L.; Hovenier, R.; Hollman, P.C.; Katan, M.B. Effects of the Flavonoids Quercetin and Apigenin on Hemostasis in Healthy Volunteers: Results from an in Vitro and a Dietary Supplement Study. The American Journal of Clinical Nutrition 1998, 67, 255–262.
- Landolfi, R.; Mower, R.L.; Steiner, M. Modification of Platelet Function and Arachidonic Acid Metabolism by Bioflavonoids: Structure-Activity Relations. Biochemical Pharmacology 1984, 33, 1525–1530.
- Tang, R.; Chen, K.; Cosentino, M.; Lee, K.H. Apigenin-7-O-β-D-Glucopyranoside, an Anti-HIV Principle from Kummerowia Striata. Bioorganic and Medicinal Chemistry Letters 1994, 4, 455–458.
- Lee, J.S.; Kim, H.J.; Lee, Y.S. A New Anti-HIV Flavonoid Glucuronide from Chrysanthemum Morifolium. Planta Medica 2003, 69, 859–861.
- Lv, X.; Qiu, M.; Chen, D.; Zheng, N.; Jin, Y.; Wu, Z. Apigenin Inhibits Enterovirus 71 Replication Through Suppressing Viral IRES Activity and Modulating Cellular JNK Pathway. Antiviral Research 2014, 109, 30–41.
- Qian, S.; Fan, W.; Qian, P.; Zhang, D.; Wei, Y.; Chen, H.; Li, X. Apigenin Restricts FMDV Infection and Inhibits Viral IRES Driven Translational Activity. Viruses 2015, 7, 1613–1626.
- Critchfield, J.W.; Butera, S.T.; Folks, T.M. Inhibition of HIV Activation in Latently Infected Cells by Flavonoid Compounds. AIDS Research and Human Retroviruses 1996, 12, 39–46J.
- Zanoli, P.; Avallone, R.; Baraldi, M. Behavioral Characterisation of the Flavonoids Apigenin and Chrysin. Fitoterapia 2000, 71, S117–S123.
- Viola, H.; Wasowski, C.; Levi, D.S.M.; Wolfman, C.; Silveira, R.; Dajas, F.; Medina, J.H.; Paladini, A.C. Apigenin, A Component of Matricaria Recutita Flowers, Is a Central Benzodiazepine Receptors-Ligand with Anxiolytic Effects. Planta Medica 1995, 61, 213–216.
- Liu, C.; Tu, F.X.; Chen, X. Neuroprotective Effects of Apigenin on Acute Transient Focal Cerebral Ischemia-Reperfusion Injury in Rats. Journal of Chinese Medicinal Materials 2008, 31, 870–873.
- Ha, S.K.; Lee, P.; Park, J.A.; Oh, H.R.; Lee, S.Y.; Park, J.H.; Lee, E.H.; Ryu, J.H.; Lee, K.R.; Kim, S.Y. Apigenin Inhibits the Production of NO and PGE 2 in Microglia and Inhibits Neuronal Cell Death in a Middle Cerebral Artery Occlusion-Induced Focal Ischemia Mice Model. Neurochemistry International 2008, 52, 878–886.
- Zhang, F.; Li, F.; Chen, G. Neuroprotective Effect of Apigenin in Rats after Contusive Spinal Cord Injury Neurological Sciences 2014, 35, 583–588.
- Bougioukas, I.; Jebran, A.F.; Stojanovic, T.; Didilis, V.; Bireta, C.; Waldmann-Beushausen, R.; Schondube, F.A.; Danner, B.C. Protective Effect of Apigenin in a Rat Model of Lung Ischemia-Reperfusion. The Thoracic and Cardiovascular Surgeon 2014, 62, SC188.
- Testai, L.; Martelli, A.; Cristofaro, M.; Breschi, M.C.; Calderone, V. Cardioprotective Effects of Different Flavonoids Against Myocardial Ischaemia/Reperfusion Injury in Langendorff‐Perfused Rat Hearts. Journal of Pharmacy and Pharmacology 2013, 65, 750–756.
- Buwa, C.C.; Mahajan, U.B.; Patil, C.R.; Goyal, S.N. Apigenin. Attenuates β-Receptor-Stimulated Myocardial Injury Via Safeguarding Cardiac Functions and Escalation of Antioxidant Defence System. Cardiovascular Toxicology 2015, 16, 286–297.
- Liu, S.Q.; Su, F.; Fang, L.M.; Xia, Q.; Zhang, X. Protective Effect of Apigenin on Neurons Against Oxygen-Glucose Deprivation/Reperfusion Induced Injury. The FASEB Journal, 24(1_MeetingAbstracts) 2010, 24, 604–615.
- Tsalkidou, E.G.; Tsaroucha, A.K.; Chatzaki, E.; Lambropoulou, M.; Papachristou, F.; Trypsianis, G.; Pitiakoudis, M.; Vaos, G.; Simopoulos, C. The Effects of Apigenin on the Expression of Fas/FasL Apoptotic Pathway in Warm Liver Ischemia-Reperfusion Injury in Rats. BioMed Research International 2014, 2014, 1–7.
- Zhang, T.; Su, J.; Guo, B.; Wang, K.; Li, X.; Liang, G. Apigenin Protects Blood–Brain Barrier and Ameliorates Early Brain Injury by Inhibiting TLR4-Mediated Inflammatory Pathway in Subarachnoid Hemorrhage Rats. International Immunopharmacology 2015, 28, 79–87.
- Kim, H.P.; Son, K.H.; Chang, H.W.; Kang, S.S. Anti-Inflammatory Plant Flavonoids and Cellular Action Mechanisms. Journal of Pharmacological Sciences 2004, 96, 229–245.
- Soliman, K.F.; Mazzio, E.A. In Vitro Attenuation of Nitric Oxide Production in C6 Astrocyte Cell Culture by Various Dietary Compounds. Experimental Biology and Medicine 1998, 218, 390–397.
- Kim, H.K.; Cheon, B.S.; Kim, Y.H.; Kim, S.Y.; Kim, H.P. Effects of Naturally Occurring Flavonoids on Nitric Oxide Production in the Macrophage Cell Line RAW 264.7 and Their Structure–Activity Relationships. Biochemical Pharmacology 1999, 58, 759–765.
- Ribeiro, D.; Freitas, M.; Lima, J.L.; Fernandes, E. Proinflammatory Pathways: The Modulation by Flavonoids. Medicinal Research Reviews 2015, 35, 877–936.
- Reuter, S.; Gupta, S.C.; Chaturvedi, M.M.; Aggarwal, B.B. Oxidative Stress, Inflammation, and Cancer: How Are They Linked? Free Radical Biology and Medicine 2010, 49, 1603–1616.
- Liang, Y.C.; Huang, Y.T.; Tsai, S.H.; Lin-Shiau, S.Y.; Chen, C.F.; Lin, J.K. Suppression of Inducible Cyclooxygenase and Inducible Nitric Oxide Synthase by Apigenin and Related Flavonoids in Mouse Macrophages. Carcinogenesis 1999, 20, 1945–1952.
- Lin, C.C.; Li, C.W.; Shih, Y.T.; Chuang, L.T. Antioxidant and Anti-Inflammatory Properties of Lower-Polymerized Polyphenols in Oolong Tea. International Journal of Food Properties 2014, 17, 752–764.
- Lotito, S.B.; Frei, B. Dietary Flavonoids Attenuate Tumor Necrosis Factor Α-Induced Adhesion Molecule Expression in Human Aortic Endothelial Cells Structure-Function Relationships and Activity after First Pass Metabolism. Journal of Biological Chemistry 2006, 281, 37102–37110.
- Panes, J.; Gerritsen, M.E.; Anderson, D.C.; Miyasaka, M.; Granger, D.N. Apigenin Inhibits Tumor Necrosis Factor-Induced Intercellular Adhesion Molecule-1 Upregulation in Vivo. Microcirculation 1996, 3, 279–286.
- Leslie, E.M.; Deeley, R.G.; Cole, S.P. Bioflavonoid Stimulation of Glutathione Transport by the 190-Kda Multidrug Resistance Protein 1 (MRP1). Drug Metabolism and Disposition 2003, 31, 11–15.
- Wong, I.L.; Chan, K.F.; Tsang, K.H.; Lam, C.Y.; Zhao, Y.; Chan, T.H.; Chow, L.M.C. Modulation of Multidrug Resistance Protein 1 (MRP1/ABCC1)-Mediated Multidrug Resistance by Bivalent Apigenin Homodimers and Their Derivatives. Journal of Medicinal Chemistry 2009, 52, 5311–5322.
- Lin, C.M.; Chen, C.S.; Chen, C.T.; Liang, Y.C.; Lin, J.K. Molecular Modeling of Flavonoids That Inhibits Xanthine Oxidase. Biochemical and Biophysical Research Communications 2002, 294, 167–172.
- Huang, J.; Wang, S.; Zhu, M.; Chen, J.; Zhu, X. Effects of Genistein, Apigenin, Quercetin, Rutin and Astilbin On Serum Uric Acid Levels and Xanthine Oxidase Activities in Normal and Hyperuricemic Mice. Food and Chemical Toxicology 2011, 49, 1943–1947.
- Huang, C.S.; Lii, C.K.; Lin, A.H.; Yeh, Y.W.; Yao, H.T.; Li, C.C.; Wang, T.S.; Chen, H.W. Protection by Chrysin, Apigenin, and Luteolin Against Oxidative Stress Is Mediated by the Nrf2-Dependent Up-Regulation of Heme Oxygenase 1 and Glutamate Cysteine Ligase in Rat Primary Hepatocytes. Archives of Toxicology 2013, 87, 167–178.
- Choi, A.M.; Alam, J. Heme Oxygenase-1: Function, Regulation, and Implication of a Novel Stress-Inducible Protein in Oxidant-Induced Lung Injury. American Journal of Respiratory Cell and Molecular Biology 1996, 15, 9–19.
- Turkseven, S.; Kruger, A.; Mingone, C.J.; Kaminski, P.; Inaba, M.; Rodella, L.F.; … Abraham, N.G. Antioxidant Mechanism of Heme Oxygenase-1 Involves an Increase in Superoxide Dismutase and Catalase in Experimental Diabetes. American Journal of Physiology-Heart and Circulatory Physiology 2005, 289, H701–H707.
- Liu, J.; Wang, Y.; Chen, W.; Li, S.; Liu, L.; Dang, Y.; Li, Z. Subchronic Exposure of Apigenin Induces Hepatic Oxidative Stress in Male Rats. Health 2014, 6, 989.
- Al-Fayez, M.; Cai, H.; Tunstall, R.; Steward, W.P.; Gescher, A.J. Differential Modulation of Cyclooxygenase-Mediated Prostaglandin Production by the Putative Cancer Chemopreventive Flavonoids Tricin, Apigenin and Quercetin.Cancer Chemotherapy and Pharmacology 2006, 58, 816–825.
- Tong, X.; Van Dross, R.T.; Abu-Yousif, A.; Morrison, A.R.; Pelling, J.C. Apigenin Prevents UVB-Induced Cyclooxygenase 2 Expression: Coupled mRNA Stabilization and Translational Inhibition. Molecular and Cellular Biology 2007, 27, 283–296.
- Van Dross, R.T.; Hong, X.; Essengue, S.; Fischer, S.M.; Pelling, J.C. Modulation of UVB‐Induced and Basal Cyclooxygenase‐2 (COX‐2) Expression by Apigenin in Mouse Keratinocytes: Role of USF Transcription Factors. Molecular Carcinogenesis 2007, 46, 303–314.
- Shen, L.N.; Zhang, Y.T.; Wang, Q.; Xu, L.; Feng, N.P. Enhanced in Vitro And In Vivo Skin Deposition of Apigenin Delivered Using Ethosomes. International Journal of Pharmaceutics 2014, 460, 280–288.
- Wang, E.; Chen, F.; Hu, X.; Yuan, Y. Protective Effects of Apigenin Against Furan-Induced Toxicity in Mice. Food & Function 2014, 5, 1804–1812.
- Zhao, J.; Zhang, Z.; Dai, J.; Zhang, C.; Ye, Y.; Li, L. Synergistic Protective Effect of Chlorogenic Acid, Apigenin and Caffeic Acid Against Carbon Tetrachloride-Induced Hepatotoxicity in Male Mice. RSC Advances 2014, 4, 43057–43063.
- Khan, T.H.; Sultana, S. Antioxidant and Hepatoprotective Potential of Soy Isoflavones Against Ccl4 Induced Oxidative Stress and Early Tumor Events. Indo Global Journal of Pharmaceutical Sciences 2011, 1, 39–56.
- Zhang, X.; Wang, G.; Gurley, E.C.; Zhou, H. Flavonoid Apigenin Inhibits Lipopolysaccharide-Induced Inflammatory Response Through Multiple Mechanisms in Macrophages. PLoS One 2014, 9, e107072.
- Simeonova, R.; Kondeva-Burdina, M.; Vitcheva, V.; Krasteva, I.; Manov, V.; Mitcheva, M. Protective Effects of the Apigenin-O/C-Diglucoside Saponarin from Gypsophila Trichotoma on Carbone Tetrachloride-Induced Hepatotoxicity in Vitro/in Vivo in Rats. Phytomedicine 2014, 21, 148–154.
- Paredes‐Gonzalez, X.; Fuentes, F.; Jeffery, S.; Saw, C.L.L.; Shu, L.; Su, Z.Y.; Kong, A.N.T. Induction of Nrf2‐Mediated Gene Expression by Dietary Phytochemical Flavones Apigenin and Luteolin. Biopharmaceutics and Drug Disposition 2015, 36, 440–451.
- Zhao, L.; Wang, J.L.; Wang, Y.R.; Fa, X.Z. Apigenin Attenuates Copper-Mediated β-Amyloid Neurotoxicity Through Antioxidation, Mitochondrion Protection and MAPK Signal Inactivation in an AD Cell Model. Brain Research 2013, 492, 33–45.
- Choi, J.S.; Islam, M.N.; Ali, M.Y.; Kim, E.J.; Kim, Y.M.; Jung, H.A. Effects of C-Glycosylation on Anti-Diabetic, Anti-Alzheimer’s Disease and Anti-Inflammatory Potential of Apigenin. Food and Chemical Toxicology 2014, 64, 27–33.
- Singh, P.V.J.; Selvendiran, K.; Mumtaz Banu, S.; Padmavathi, R.; Sakthisekaran, D. Protective Role of Apigenin on the Status of Lipid Peroxidation and Antioxidant Defense Against Hepatocarcinogenesis in Wistar Albino Rats. Phytomedicine 2004, 11, 309–314.
- Hougee, S.; Sanders, A.; Faber, J.; Graus, Y.M.; van den Berg, W.B.; Garssen, J.; Smit, H.F.; Hoijer, MA. Decreased Pro-Inflammatory Cytokine Production by LPS-Stimulated PBMC upon in Vitro Incubation with the Flavonoids Apigenin, Luteolin or Chrysin, Due to Selective Elimination of Monocytes/Macrophages. Biochemical Pharmacology 2005, 69, 241–248.
- Simolinski, A.T.; Pestka, J.J. Modulation of Lipopolysaccharide-Induced Proinflammatory Cytokine Production in Vitro and in Vivo by the Herbal Constituent’s Apigenin (Chamomile), Ginsenoside Rb 1 (Ginseng) and Parthenolide (Feverfew). Food and Chemical Toxicology 2003, 41, 1381–1390.
- Ju, S.M.; Kang, J.G.; Bae, J.S.; Pae, H.O.; Lyu, Y.S.; Jeon B.H. The Flavonoid Apigenin Ameliorates Cisplatin-Induced Nephrotoxicity through Reduction of p53 Activation and Promotion of PI3K/Akt Pathway in Human Renal Proximal Tubular Epithelial Cells. Evidence Based Complmetary Alternative Medicine 2015, 2015, 1–9.
- Nisha, V.M.; Anusree, S.S.; Priyanka, A.; Raghu, K.G. Apigenin and Quercetin Ameliorate Mitochondrial Alterations by Tunicamycin-Induced ER Stress in 3T3-L1 Adipocytes. Applied Biochemistry and Biotechnology 2014, 174, 1365–1375.
- Hussein, J.S.; Abdel Latif, Y.; El-Bana, M.A.; Medhat, D. New Approach in Treatment of Brain Injury: Neurotrophic Effects of Apigenin. Journal of Chemical and Pharmaceutical Research 2015, 7, 996–1004.
- Mascaraque, C.; González, R.; Suárez, M.D.; Zarzuelo, A.; Sánchez de M.F.; Martínez-A.O. Intestinal Anti-Inflammatory Activity of Apigenin K in Two Rat Colitis Models Induced by Trinitrobenzenesulfonic Acid and Dextran Sulphate Sodium. British Journal of Nutrition 2015, 113, 618–626.
- Yang, J.; Wang, X.Y.; Xue, J.; Gu, Z.L.; Xie, M.L. Protective Effect of Apigenin on Mouse Acute Liver Injury Induced by Acetaminophen Is Associated with Increment of Hepatic Glutathione Reductase Activity. Food and Function 2013, 4, 939–943.
- Haleagrahara, N.; Chakravarthi, S.; Kulur, A.B.; Yee, T.M. Plant Flavone Apigenin Protects Against Cyclosporine-Induced Histological and Biochemical Changes in the Kidney in Rats. Biomedicine and Preventive Nutrition 2014, 4, 589–593.
- Khan, T.H.; Jahangir, T.; Prasad, L.; Sultana S. Inhibitory Effect of Apigenin on Benzo (A) Pyrene‐Mediated Genotoxicity in Swiss Albino Mice. Journal of Pharmacy and Pharmaceutical Sciences 2006, 58, 1655–1660.
- Panda, S.; Kar, A. Apigenin (4‘, 5, 7‐Trihydroxyflavone) Regulates Hyperglycaemia, Thyroid Dysfunction and Lipid Peroxidation in Alloxan‐Induced Diabetic Mice. Journal of Pharmacy and Pharmaceutical Sciences 2007, 59, 1543–1548.
- Wang, L.; Kuang, L.; Hitron, J.A.; Son, Y.O.; Budhraja, A.; Lee, J.C.; Pratheeshkumar, P.; Chen, G.; Zhang, Z.; Luo, J. Apigenin Suppresses Migration and Invasion of Transformed Cells Through Down-Regulation of CXC Chemokine Receptor 4 Expressions. Toxicology and Applied Pharmacology 2013, 272, 108–116.
- Siddique, Y.H.; Ara, G.; Jyoti, S.; Naz, F.; Afzal, M. Protective Effect of Apigenin Against Ethinylestradiol Induced Toxic Effects in the Third Instar Larvae of Transgenic Drosophila Melanogaster (hsp70-lacZ) Bg9. Pharacology Online 2011, 3, 667–684.
- Silvan, S.; Manoharan, S.; Baskaran, N.; Karthikeyan, S.; Prabhakar, M.M. Protective Effect of Apigenin on 7, 12-Dimethylbenz (A) Anthracene Induced Glycoconjugates in the Plasma and Buccal Mucosa of Golden Syrian Hamsters. International Journal of Pharmaceutical Science Research 2011, 2, 1753.
- Siddique, Y.H.; Afzal, M. Antigenotoxic Effect of Apigenin Against Mitomycin C Induced Genotoxic Damage in Mice Bone Marrow Cells. Food and Chemical Toxicology 2009, 47, 536–539.
- Siddique, Y.H.; Beg, T.; Afzal, M. Antigenotoxic Effect of Apigenin Against Anti-Cancerous Drugs. Toxicology in Vitro 2008, 22, 625–631.
- Noel, S.; Kasinathan, M.; Rath, S.K. Evaluation of Apigenin Using in Vitro Cytochalasin Blocked Micronucleus Assay. Toxicology in Vitro 2006, 20, 1168–1172.
- Papachristou, F.; Chatzaki, E.; Petrou, A.; Kougioumtzi, I.; Katsikogiannis, N.; Papalambros, A.; Tripsianis, G.; Simopoulos, C.; Tsaroucha, A.K. Time Course Changes of Anti- and Pro-Apoptotic Proteins in Apigenin-Induced Genotoxicity. Chinese Medicine 2013, 8, 8546–8548.
- Bokulić, A.; Garaj-Vrhovac, V.; Brajša, K.; Ðurić, K.; Glojnarić, I.; and Šitum, K. The Effect of Apigenin on Cyclophosphamide and Doxorubicin Genotoxicity in Vitro and in Vivo. Journal of Environmental Science and Health 2011, 46, 526–533.
- Das, S.; Das, J.; Samadder, A.; Paul, A.; Khuda-Bukhsh, A.R. Efficacy of PLGA-Loaded Apigenin Nanoparticles in Benzo [A] Pyrene and Ultraviolet-B Induced Skin Cancer of Mice: Mitochondria Mediated Apoptotic Signalling Cascades. Food and Chemical Toxicology 2013, 62, 670–680.
- Kuo, M.L.; Lee, K.C.; Lin, J.K. Genotoxicities of Nitropyrenes and Their Modulation by Apigenin, Tannic Acid, Ellagic Acid and Indole-3-Carbinol in the Salmonella and CHO Systems.Mutation Research 1992, 270, 87–95.
- Rithidech, K.N.; Tungjai, M.; Whorton, E.B. Protective Effect of Apigenin on Radiation-Induced Chromosomal Damage in Human Lymphocytes. Mutation Research 2005, 585, 96–104.
- Anter, J.; Romero-Jiménez, M.; Fernández-Bedmar, Z.; Villatoro-Pulido, M.; Analla, M.; Alonso-Moraga, Á.; Muñoz-Serrano, A. Antigenotoxicity, Cytotoxicity, and Apoptosis Induction by Apigenin, Bisabolol, and Protocatechuic Acid. Journal of Medicinal Food 2011, 14, 276–283.
- Siddique, Y.H.; Ara, G.; Beg, T.; Afzal, M. Anticlastogenic Effect of Apigenin in Human Lymphocytes Treated with Ethinylestradiol.Fitoterapia 2010, 81, 590–594.
- Romero-Jimenez, M.; Campos-Sanchez, J.; Analla, M.; Muñoz-Serrano, A.; Alonso-Moraga, Á. Genotoxicity and Anti-Genotoxicity of Some Traditional Medicinal Herbs. Mutation Research 2005, 585, 147–155.
- Siddique, Y.H.; Afzal, M. Protective Effect of Apigenin Hydrogen Peroxide Induced Genotoxic Damage on Cultured Human Peripheral Blood Lymphocytes. Journal of Applied Biomedicine 2009, 7, 35–43.
- Sharma, N.K. Modulation of Radiation-Induced and Mitomycin C-Induced Chromosome Damage by Apigenin in Human Lymphocytes in Vitro. Journal of Radiation Research 2013, 54, 789–797.
- Begum, N.; Prasad, N.R.; Kanimozhi, G.; Hasan, A.Q. Apigenin Ameliorates Gamma Radiation-Induced Cytogenetic Alterations in Cultured Human Blood Lymphocytes. Mutation Research 2012, 74, 71–76.
- Flamand, N.; Marrot, L.; Belaidi, J.P.; Bourouf, L.; Dourille, E.; Feltes, M.; Meunier, J.R. Development of Genotoxicity Test Procedures with Episkin®, a Reconstructed Human Skin Model: Towards New Tools for in Vitro Risk Assessment of Dermally Applied Compounds.? Mutation Research 2006, 606, 39–51.
- Singh, P.; Dabas, A.; Srivastava, R.; Nagpure, N.S.; Singh, A. Evaluation of Genotoxicity Induced by Medicinal Plant Jatropha Gossypifolia in Fresh Water Fish Channa Punctatus (Bloch.). Turkish Journal of Fish Aquaculture and Science 2014, 14, 421–428.
- Czeczot, H.; Kusztelak, A Study of Genotoxic Potentialof Flavonoids Using Short-Term Bacterial Assays. Journal Acta Biochemica Polonica-EngEd 1993, 40, 549–549.
- Orhan, I.; Kartal, M.; Tosun, F.; Şener, B. Screening of Various Phenolic Acids and Flavonoid Derivatives for Their Anticholinesterase Potential. Zeitschrift für Naturforschung 2007, 62, 829–832.
- Zhao, L.; Wang, J.L.; Liu, R.; Li, X.X.; Li, J.F.; Zhang, L. Neuroprotective, Anti-Amyloidogenic and Neurotrophic Effects of Apigenin in an Alzheimer’s Disease Mouse Model. Molecules 2013, 18, 9949–9965.
- Zhao, L.; Wang, J.L.; Wang, Y.R.; Fa, X.Z. Apigenin Attenuates Copper-Mediated β-Amyloid Neurotoxicity Through Antioxidation, Mitochondrion Protection and MAPK Signal Inactivation in an AD Cell Model. Brain Research 2013, 1492, 33–45.
- Ansari, N.; Khodagholi, F. Molecular Mechanism Aspect of ER Stress in Alzheimer’s Disease: Current Approaches and Future Strategies. Current Drug Tarets 2013, 14, 114–122.
- Ebrahimi, A.; Schluesener, H. Natural Polyphenols Against Neurodegenerative Disorders: Potentials and Pitfalls. Ageing Research and Reviews 2012, 11, 329–345.
- Shimmyo, Y.; Kihara, T.; Akaike, A.; Niidome, T.; Sugimoto, H. Flavonols and Flavones As BACE-1 Inhibitors: Structure–Activity Relationship in Cell-Free, Cell-Based and in Silico Studies Reveal Novel Pharmacophore Features. Biochemica et Biophysica Acta (BBA) 2008, 1780, 819–825.
- Patil, S.P.; Jain, P.D.; Sancheti, J.S.; Ghumatkar, P.J.; Tambe, R.; Sathaye, S. Neuroprotective and Neurotrophic Effects of Apigenin and Luteolin in MPTP Induced Parkinsonism in Mice. Neuropharmacology 2014, 86, 192–202.
- Yano, S.; Umeda, D.; Maeda, N.; Fujimura, Y.; Yamada, K.; Tachibana, H. Dietary Apigenin Suppresses IgE and Inflammatory Cytokines Production in C57BL/6N Mice. Journal of Agriculture Food Chemistry 2006, 54, 5203–5207.
- Fu, M.S.; Zhu, B.J.; Luo, D.W. Apigenin Prevents TNF-α Induced Apoptosis of Primary Rat Retinal Ganglion Cells. Cellular and Molecular Biology 2014, 60, 37.
- Li, J.; Zhang, B. Apigenin Protects Ovalbumin-Induced Asthma Through the Regulation of Th17 Cells. Fitoterapia 2013, 91, 298–304.
- Chaumontet, C.; Bex, V.; Gaillard-Sanchez, I.; Seillan-Heberden, C.; Suschetet, M.; Martel, P. Apigenin and Tangeretin Enhance Gap Junctional Intercellular Communication in Rat Liver Epithelial Cells. Carcinogenesis 1994, 15, 2325–2330.
- Lorenzo, P.S.; Rubio, M.C.; Medina, J.H.; Adler-Graschinsky, E. Involvement of Monoamine Oxidase and Noradrenaline Uptake in the Positive Chronotropic Effects of Apigenin in Rat Atria. European Journal of Pharmacology 1996, 312, 203–207.
- Amini, R.; Yazdanparast, R.; Bahramikia, S. Apigenin Reduces Human Insulin Fibrillation in Vitro and Protects SK-N-MC Cells Against Insulin Amyloids. International Journal of Biological Macromolecules 2013, 60, 334–340.
- Choi, J.R.; Lee, C.M.; Jung, I.D.; Lee, J.S.; Jeong, Y.I.; Chang, J.H.; Park, H.J.; Choi, I.W.; Kim, J.S.; Shin, Y.K.; Park, S.N. Apigenin Protects Ovalbumin-Induced Asthma Through the Regulation of GATA-3 Gene. International Immunopharmacology 2009, 9, 918–924.
- Hossain, C.M.; Ghosh, M.K.; Satapathy, B.S.; Dey, N.S.; Mukherjee, B. Apigenin Causes Biochemical Modulation, GLUT4 and CD38 Alterations to Improve Diabetes and to Protect Damages of Some Vital Organs in Experimental Diabetes. American Journal of Pharmacology and Toxicology 2014, 9, 39–52.
- Chan, E.C.; Pannangpetch, P.; Woodman, O.L. Relaxation to Flavones and Flavonols in Rat Isolated Thoracic Aorta: Mechanism of Action and Structure-Activity Relationships. Journal of Cardiovascular Pharmacology 2000, 35, 326–333.
- Ko, F.N.; Huang, T.F.; Teng, C.M. Action Mechanisms of Apigenin Isolated from Apium Graveolens in Rat Thoracic Aorta. Biochimica et Biophysica Acta (BBA)-General Subject 1991, 1115, 69–74.
- Fonseca-Silva, F.; Canto-Cavalheiro, M.M.; Menna-Barreto, R.F.; Almeida-Amaral, E.E. Effect of Apigenin on Leishmania Amazonensis Is Associated with Reactive Oxygen Species Production Followed by Mitochondrial Dysfunction. Journal of Natural Products 2015, 78, 880–884.
- Li, H.; Li, H.B.; Zhang, M.; Yan, F.; Zhang, Z.X.; Li, Z.L. Effect of Apigenin on the Reproductive System in Male Mice. Health 2010, 2, 435.
- Singh, S.K.; Singh, S.K.; Singh, A. Toxicological and Biochemical Alterations of Apigenin Extracted from Seed of Thevetia Peruviana, a Medicinal Plant. Journal of Biological Earth Sciences 2013, 3, B110–B119.
- Zhang, Y.; Wang, J.; Cheng, X.; Yi, B.; Zhang, X.; Li, Q. Apigenin Induces Dermal Collagen Synthesis Via Smad2/3 Signaling Pathway. European Journal of Histochemistry 2015, 59, 2.
- Man, M.Q.; Hupe, M.; Sun, R.; Man, G.; Mauro, T.M.; Elias, P.M. Topical Apigenin Alleviates Cutaneous Inflammation in Murine Models. Evidence Based Complementary and Alternate Medicine 2012, 2012, 1–7.
- Funakoshi-Tago, M.; Nakamura, K.; Tago, K.; Mashino, T.; Kasahara, T. Anti-Inflammatory Activity of Structurally Related Flavonoids, Apigenin, Luteolin and Fisetin. International Immunopharmacology 2011, 11, 1150–1159.
- Li, R.R.; Pang, L.L.; Du, Q.; Shi, Y.; Dai, W.J.; Yin, K.S. Apigenin Inhibits Allergen-Induced Airway Inflammation and Switches Immune Response in a Murine Model of Asthma. Immunopharma. Immunotoxicology 2010, 32, 364–370.
- Knowles, L.M.; Zigrossi, D.A.; Tauber, R.A.; Hightower, C.; Milner, J.A. Flavonoids Suppress Androgen Independent Human Prostate Tumor Proliferation. Nutrition Cancer 2000, 38, 116–122.
- Mirzoeva, S.; Kim, N.D.; Chiu, K.; Franzen, C.A.; Bergan, R.C.; Pelling, J.C. Inhibition of HIF‐1 Alpha and VEGF Expression by the Chemopreventive Bioflavonoid Apigenin Is Accompanied by Akt Inhibition in Human Prostate Carcinoma PC3‐M Cells. Molecular Carcinogenesis 2008, 47, 686–700.
- Franzen, C.A.; Amargo, E.; Todorović, V.; Desai, B.V.; Huda, S.; Mirzoeva, S.; Chiu, K.; Grzybowski, B.A.; Chew, T.L.; Green, K.J.; Pelling, J.C. The Chemopreventive Bioflavonoid Apigenin Inhibits Prostate Cancer Cell Motility Through the Focal Adhesion Kinase/Src Signaling Mechanism. Cancer Prevention and Research 2009, 2, 830–841.
- Shukla, S.; MacLennan, G.T.; Hartman, D.J.; Fu, P.; Resnick, M.I.; Gupta, S. Activation of PI3K‐Akt Signaling Pathway Promotes Prostate Cancer Cell Invasion. International Journal of Cancer 2007, 121, 1424–1432.
- Shukla, S.; Gupta, S. Suppression of Constitutive and Tumor Necrosis Factor Alpha-Induced Nuclear Factor (NF)-KappaB Activation and Induction of Apoptosis by Apigenin in Human Prostate Carcinoma PC-3 Cells: Correlation with Down-Regulation of NF-Kappa B Responsive Genes. Clinical Cancer Research 2004, 10, 3169–3178.
- Shukla, S.; Gupta, S. Molecular Targets for Apigenin-Induced Cell Cycle Arrest and Apoptosis in Prostate Cancer Cell Xenograft. Molecular Cancer Therapeutics 2006, 5, 843–852.
- Shukla, S.; Mishra, A.; Fu, P.; MacLennan, G.T.; Resnick, M.I.; Gupta, S. Up-Regulation of Insulin-Like Growth Factor Binding Protein-3 by Apigenin Leads to Growth Inhibition and Apoptosis of 22Rv1 Xenograft in Athymic Nude Mice. The FASEB Journal 2005, 19, 2042–2044.
- Shukla, S.; Gupta, S. Apigenin-Induced Prostate Cancer Cell Death Is Initiated by Reactive Oxygen Species and P53 Activation. Free Radical Biology and Medicine 2008, 44, 1833–1845.
- Shukla, S.; Fu, P.; Gupta, S. Apigenin Suppresses Inhibitor of Apoptosis Family Protein Expression and Disrupts Ku70-Bax Interaction in Prostate Cancer Cells in Culture and in Vivo. Cancer Research 2014, 74, 1238–1238.
- Shukla, S.; Gupta, S. Apigenin-Induced Cell Cycle Arrest Is Mediated by Modulation of MAPK, PI3K-Akt and Loss of Cyclin D1 Associated Retinoblastoma Dephosphorylation in Human Prostate Cancer Cells. Cell Cycle-landes Biosciences 2007, 6, 1102.
- Shukla, S.; MacLennan, G.T.; Flask, C.A.; Fu, P,; Mishra, A.; Resnick, M.I.; Gupta, S. Blockage of β-Catenin Signaling by Plant Flavonoid Apigenin Suppresses Prostate Carcinogenesis in TRAMP Mice. Cancer Research 2007, 67, 6925–6935.
- Shukla, S.; Gupta, S. Apigenin Suppresses Insulin‐Like Growth Factor I Receptor Signaling in Human Prostate Cancer: An in Vitro and in Vivo Study. Molecular Carcinogenesis 2009, 48, 243–252.
- Zhu, Y.; Wu, J.; Li, S.; Wang, X.; Liang, Z.; Xu, X.; Xu, X.; Hu, Z.; Lin, Y.; Chen, H.; Qin, J. Apigenin Inhibits Migration and Invasion Via Modulation of Epithelial Mesenchymal Transition in Prostate Cancer. Molecular Medicine Reports 2015, 11, 1004–1008.
- Shukla, S.; Bhaskaran, N.; Babcook, M.A.; Fu, P.; MacLennan, G.T.; Gupta, S. Apigenin Inhibits Prostate Cancer Progression in TRAMP Mice Via Targeting PI3K/Akt/FoxO Pathway. Carcinogenesis 2014, 35, 452–460.
- Hessenauer, A.; Montenarh, M.; Götz, C. Inhibition of CK2 Activity Provokes Different Responses in Hormone-Sensitive and Hormone-Refractory Prostate Cancer Cells. International Journal of Oncology 2003, 22, 1263–1270.
- Singh, V.; Sharma, V.; Verma, V.; Pandey, D.; Yadav, S.K.; Maikhuri, J.P.; Gupta, G. Apigenin Manipulates the Ubiquitin–Proteasome System to Rescue Estrogen Receptor-β from Degradation and Induce Apoptosis in Prostate Cancer Cells. European Journal of Nutrition 2015, 54, 1255–1267.
- Wätjen, W.; Weber, N.; Lou, Y.J.; Wang, Z.Q.; Chovolou, Y.; Kampkötter, A.; Kahl, R.; Proksch, P. Prenylation Enhances Cytotoxicity of Apigenin and Liquiritigenin in Rat H4IIE Hepatoma and C6 Glioma Cells. Food and Chemical Toxicology 2007, 45, 119–124.
- Bog, Y.S.; Hwa, L.J.; Young, C.H.; Ja, B.S.; Soo, C.J.; Deuk, K.N. Inhibitory Effects of Luteolin Isolated Fromixeris Sonchifolia Hance on the Proliferation of HepG2 Human Hepatocellular Carcinoma Cells. Archives of Pharmaceutical Research 2003, 26, 151–156.
- Eaton, E.A.; Walle, U.K.; Lewis, A.J.; Hudson, T.; Wilson, A.A.; Walle, T. Flavonoids, Potent Inhibitors of the Human P-Form Phenolsulfotransferase. Potential Role in Drug Metabolism and Chemoprevention. Drug Metabolism and Disposition 1996, 24, 232–237.
- Gao, A.M.; Ke, Z.P.; Wang, J.N.; Yang, J.Y.; Chen, S.Y.; Chen, H. Apigenin Sensitizes Doxorubicin-Resistant Hepatocellular Carcinoma BEL-7402/ADM Cells to Doxorubicin Via Inhibiting PI3K/Akt/Nrf2 Pathway. Carcinogenesis 2013, 34, 1806–1814.
- Chiang, L.C.; Ng, L.T.; Lin, I.C.; Kuo, P.L.; Lin, C.C. Anti-Proliferative Effect of Apigenin and Its Apoptotic Induction in Human Hepg2 Cells. Cancer Letters 2006, 237, 207–214.
- Xu, Y.; Xin, Y.; Diao, Y.; Lu, C.; Fu, J.; Luo, L.; Yin, Z. Synergistic Effects of Apigenin and Paclitaxel on Apoptosis of Cancer Cells. PLoS One 2011, 6, 29169.
- Jeyabal, P.V.S.; Syed, M.B.; Venkataraman, M.; Sambandham, J.K.; Sakthisekaran, D. Apigenin Inhibits Oxidative Stress‐Induced Macromolecular Damage in N‐Nitrosodiethylamine (NDEA) Induced Hepatocellular Carcinogenesis in Wistar Albino Rats. Molecular Carcinogenesis 2005, 44, 11–20.
- Sanderson, J.T.; Hordijk, J.; Denison, M.S.; Springsteel, M.F. Induction and Inhibition of Aromatase (CYP19) Activity by Natural and Synthetic Flavonoid Compounds in H295R Human Adrenocortical Carcinoma Cells. Toxicological Sciences 2004, 82, 70–79.
- Ohno, S.; Shinoda, S.; Toyoshima, S.; Nakazawa, H.; Makino, T.; Nakajin, S. Effects of Flavonoid Phytochemicals on Cortisol Production and on Activities of Steroidogenic Enzymes in Human Adrenocortical H295R Cells. The Journal of Steroid Biochemistry and Molecular Biology 2002, 80, 355–363.
- Wang, I.K.; Lin-Shiau, S.Y.; Lin, J.K. Induction of Apoptosis by Apigenin and Related Flavonoids Through Cytochrome C Release and Activation of Caspase-9 and Caspase-3 in Leukaemia HL-60 Cells. European Journal of Cancer 1999, 35, 1517–1525.
- Vargo, M.A.; Voss, O.H.; Poustka, F.; Cardounel, A.J.; Grotewold, E.; Doseff, A.I. Apigenin-Induced-Apoptosis Is Mediated by the Activation of PKCδ and Caspases in Leukemia Cells. Biochemical Pharmacology 2006, 72, 681–692.
- Navarro-Nunez, L.; Lozano, M.L.; Palomo, M.; Martinez, C.; Vicente, V.; Castillo, J.; Benavente-García, O.; Diaz-Ricart, M. Apigenin Inhibits Platelet Adhesion and Thrombus Formation and Synergizes with Aspirin in the Suppression of the Arachidonic Acid Pathway. Journal of Agriculture Food Chememistry 2008, 56, 2970–2976.
- Monasterio, A.; Urdaci, M.C.; Pinchuk, I.V.; Lopez-Moratalla, N.; Martinez-Irujo, J.J. Flavonoids Induce Apoptosis in Human Leukemia U937 Cells Through Caspase-and Caspase-Calpain-Dependent Pathways. Nutrition Cancer 2004, 50, 90–100.
- Strick, R.; Strissel, P.L.; Borgers, S.; Smith, S.L.; Rowley, J.D. Dietary Bioflavonoids Induce Cleavage in the MLL Gene and May Contribute to Infant Leukemia. Proceedings of the National Academy of Sciences 2000, 97, 4790–4795.
- Paredes-Gonzalez, X.; Fuentes, F.; Su, Z.Y.; Kong, A.N.T. Apigenin Reactivates Nrf2 Anti-Oxidative Stress Signaling in Mouse Skin Epidermal JB6 P+ Cells Through Epigenetics Modifications. The AAPS Journal 2014, 16, 727–735.
- Ye, Y.; Chou, G.X.; Wang, H.; Chu, J.H.; Yu, Z.L. Flavonoids, Apigenin and Icariin Exert Potent Melanogenic Activities in Murine B16 Melanoma Cells. Phytomedicine 2010, 18, 32–35.
- Birt, D.F.; Mitchell, D.; Gold, B.; Pour, P.; Pinch, H.C. Inhibition of Ultraviolet Light Induced Skin Carcinogenesis in SKH-1 Mice by Apigenin, a Plant Flavonoid. Anticancer Research 1996, 17, 85–91.
- Lepley, D.M.; Pelling, J.C. Induction of p21/WAF1 and G1 Cell‐Cycle Arrest by the Chemopreventive Agent Apigenin. Molecular Carcinogenesis 1997, 19, 74–82.
- McVean, M.; Xiao, H.; Isobe, K.I.; Pelling, J.C. Increase in Wild-Type p53 Stability and Transactivational Activity by the Chemopreventive Agent Apigenin in Keratinocytes. Carcinogenesis 2000, 21, 633–639.
- Li, B.; Birt, D.F. In Vivo and in Vitro Percutaneous Absorption of Cancer Preventive Flavonoid Apigenin in Different Vehicles in Mouse Skin. Pharmaceutical Research 1996, 13, 1710–1715.
- Li, B.; Pinch, H.; Birt, D.R. Influence of Vehicle, Distant Topical Delivery, and Biotransformation on the Chemopreventive Activity of Apigenin, a Plant Flavonoid, in Mouse Skin. Pharmacology Research 1996, 13, 1530–1534.
- Tong, X.; Mirzoeva, S.; Veliceasa, D.; Bridgeman, B.B.; Fitchev, P.; Cornwell, M.L.; Crawford, S.E.; Pelling, J.C.; Volpert, O.V. Chemopreventive Apigenin Controls UVB-Induced Cutaneous Proliferation and Angiogenesis Through HuR and Thrombospondin-1. Oncotarget 2014, 5, 11413.
- Wei, H.; Tye, L.; Bresnick, E.; Birt, D.F. Inhibitory Effect of Apigenin, a Plant Flavonoid, on Epidermal Ornithine Decarboxylase and Skin Tumor Promotion in Mice. Cancer Research 1990, 50, 499–502.
- Lepley, D.M.; Li, B.; Birt, D.F.; Pelling, J.C. The Chemopreventive Flavonoid Apigenin Induces G2/M Arrest in Keratinocytes. Carcinogenesis 1996, 17, 2367–2375.
- Balasubramanian, S.; Eckert, R.L. Keratinocyte Proliferation, Differentiation, and Apoptosis—Differential Mechanisms of Regulation by Curcumin, EGCG and Apigenin. Toxicology and Applied Pharmacology 2007, 224, 214–219.
- Torkin, R.; Lavoie, J.F.; Kaplan, D.R.; Yeger, H. Induction of Caspase-Dependent, p53-Mediated Apoptosis by Apigenin in Human Neuroblastoma. Molecular Cancer Therapy 2005, 4, 1–11.
- Guo, H.; Kong, S.; Chen, W.; Dai, Z.; Lin, T.; Su, J.; Li, S.; Xie, Q.; Su, Z.; Xu, Y.; Lai, X. Apigenin Mediated Protection of OGD-Evoked Neuron-Like Injury in Differentiated PC12 Cells. Neurochemistry Reearch 2014, 39, 2197–2210.
- Das, A.; Banik, N.L.; Ray, S.K. Flavonoids Activated Caspases for Apoptosis in Human Glioblastoma T98G and U87MG Cells But Not in Human Normal Astrocytes. Cancer 2010, 116, 164–176.
- Yin, F.; Giuliano, A.E.; Van Herle, A.J. Signal Pathways Involved in Apigenin Inhibition of Growth and Induction of Apoptosis of Human Anaplastic Thyroid Cancer Cells (ARO). Anticancer Research 1998, 19, 4297–4303.
- Smit, J.W.; Elst, J.P.S.V.D.; Karperien, M.; Que, I.; van der Pluijm, G.; Goslings, B.; Romijn, J.A.; van der Heide, D. Reestablishment of in Vitro and in Vivo Iodide Uptake by Transfection of the Human Sodium Iodide Symporter (hNIS) in a hNIS Defective Human Thyroid Carcinoma Cell Line. Thyroid 2000, 10, 939–943.
- Kim, S.H.; Kang, J.G.; Kim, C.S.; Ihm, S.H.; Choi, M.G.; Yoo, H.J.; Lee, S.J. Apigenin Induces c-Myc-Mediated Apoptosis in FRO Anaplastic Thyroid Carcinoma Cells. Molecular and Cell Endocrinology 2013, 369, 130–139.
- Zhu, F.; Liu, X.G.; Liang, N.C. Effect of Emodin and Apigenin on Invasion of Human Ovarian Carcinoma HO-8910PM Cells in Vitro. Ai Zheng 2003, 22, 358–362.
- Suh, Y.A.; Jo, S.Y.; Lee, H.Y.; Lee, C. Inhibition of IL-6/STAT3 Axis and Targeting Axl and Tyro3 Receptor Tyrosine Kinases by Apigenin Circumvent Taxol Resistance in Ovarian Cancer Cells. International Journal of Oncology 2015, 46, 1405–1411.
- Fang, J.; Xia, C.; Cao, Z.; Zheng, J.Z.; Reed, E.; Jiang, B.H. Apigenin Inhibits VEGF and HIF-1 Expression via PI3K/AKT/p70S6K1 and HDM2/p53 Pathways. The FASEB Journal 2005, 19, 342–353.
- Fang, J.; Zhou, Q.; Liu, L.Z.; Xia, C.; Hu, X.; Shi, X.; Jiang, B.H. Apigenin Inhibits Tumor Angiogenesis Through Decreasing HIF-1α and VEGF Expression. Carcinogenesis 2006, 28, 858–864.
- Li, Z.D.; Hu, X.W.; Wang, Y.T.; Fang, J. Apigenin Inhibits Proliferation of Ovarian Cancer A2780 Cells Through Id1. FEBS Letters 2009, 583, 1999–2003.
- O’Toole, S.A.; Sheppard, B.L.; Sheils, O.; O’Leary, J.J.; Spengler, B.; Christoffel, V. Analysis of DNA in Endometrial Cancer Cells Treated with Phyto-Estrogenic Compounds Using Comparative Genomic Hybridisation Microarrays. Planta Medica 2005, 71, 435–439.
- Banerjee, K.; Mandal, M. Oxidative Stress Triggered by Naturally Occurring Flavone Apigenin Results in Senescence and Chemotherapeutic Effect in Human Colorectal Cancer Cells. Redox Biology 2015, 5, 153–162.
- Lee, Y.; Sung, B.; Kang, Y.J.; Kim, D.H.; Jang, J.Y.; Hwang, SY.; Kim, M.; Lim, H.S.; Yoon, J.H.; Chung, H.Y.; Kim, N.D. Apigenin-Induced Apoptosis Is Enhanced by Inhibition of Autophagy Formation in HCT116 Human Colon Cancer Cells. International Journal Oncology 2014, 44, 1599–1606.
- Wang, W.; Van Alstyne, P.C.; Irons, K.A.; Chen, S.; Stewart, J.W.; Birt, D.F. Individual and Interactive Effects of Apigenin Analogs on G2/M Cell-Cycle Arrest in Human Colon Carcinoma Cell Lines. Nutrition and Cancer 2004, 48, 106–114.
- Iizumi, Y.; Oishi, M.; Taniguchi, T.; Goi, W.; Sowa, Y.; Sakai, T. The Flavonoid Apigenin Downregulates CDK1 by Directly Targeting Ribosomal Protein S9. PloS One 2013, 8, 8.
- Caltagirone, S.; Rossi, C.; Poggi, A.; Ranelletti, F.O.; Natali, P.G.; Brunetti, M.; Aiello, F.B.; Piantelli, M. Flavonoids Apigenin and Quercetin Inhibit Melanoma Growth and Metastatic Potential. International Journal of Cancer 2000, 87, 595–600.
- Kim, K.C.; Choi, E.H.; Lee, C. Axl Receptor Tyrosine Kinase Is a Novel Target of Apigenin for the Inhibition of Cell Proliferation. International Journal of Molecular Medicine 2014, 34, 592–598.
- Watanabe, N.; Hirayama, R.; Kubota, N. The Chemopreventive Flavonoid Apigenin Confers Radiosensitizing Effect in Human Tumor Cells Grown as Monolayers and Spheroids. Journal of Radiation Research 2007, 48, 45–50.
- Engelmann, C.; Blot, E.; Panis, Y.; Bauer, S.; Trochon, V.; Nagy, H.J.; Lu, H.; Soria, C. Apigenin–Strong Cytostatic and Anti-Angiogenic Action in Vitro Contrasted by Lack of Efficacy in Vivo. Phytomedicine 2002, 9, 489–495.
- Piantelli, M.; Rossi, C.; Iezzi, M.; La, Sorda, R.; Iacobelli, S.; Alberti, S.; Natali, P.G. Flavonoids Inhibit Melanoma Lung Metastasis by Impairing Tumor Cells Endothelium Interactions. Journal of Cellular Physiology 2006, 207, 23–29.
- Shi, M.D.; Shiao, C.K.; Lee, Y.C.; Shih, Y.W. Apigenin, a Dietary Flavonoid, Inhibits Proliferation of Human Bladder Cancer T-24 Cells Via Blocking Cell Cycle Progression and Inducing Apoptosis. Cancer Cell International 2015, 15, 33.
- Zhu, Y.; Mao, Y.; Chen, H.; Lin, Y.; Hu, Z.; Wu, J.; Xu, X.; Xu, X.; Qin, J.; Xie, L. Apigenin Promotes Apoptosis, Inhibits Invasion and Induces Cell Cycle Arrest of T24 Human Bladder Cancer Cells. Cancer Cell International 2013, 13, 54.
- Way, T.D.; Kao, M.C.; Lin, J.K. Apigenin Induces Apoptosis Through Proteasomal Degradation of HER2/neu in HER2/neu-Overexpressing Breast Cancer Cells Via the Phosphatidylinositol 3-Kinase/Akt-Dependent Pathway. Biological Chemistry 2004, 279, 4479–4489.
- Way, T.D.; Kao, M.C.; Lin, J.K. Degradation of HER2/neu by Apigenin Induces Apoptosis Through Cytochrome C Release and Caspase-3 Activation in HER2/neu-Overexpressing Breast Cancer Cells. FEBS Letters 2005, 579, 145–152.
- Choi, E.J.; Kim, G.H. Apigenin Induces Apoptosis Through Mitochondria/Caspase-Pathway in Human Breast Cancer MDA-MB-453 Cells. Journal of Clinical Biochemistry and Nutrition 2009, 44, 260–265.
- Weldon, C.B.; McKee, A.; Collins-Burow, B.M.; Melnik, L.I.; Scandurro, A.B.; McLachlan, J.A.; Burow, M.E.; Beckman, B.S. PKC-Mediated Survival Signaling in Breast Carcinoma Cells: A Role for MEK1-AP1 Signaling. International Journal of Oncology 2005, 26, 763–768.
- Choi, E.J.; Kim, G.H. Apigenin Causes G/M Arrest Associated with the Modulation of p21, Cip1 and Cdc2 and Activates p53-Dependent Apoptosis Pathway in Human Breast Cancer SK-BR-3 Cells. The Journal of Nutritional Biochemistry 2009, 20, 285–290.
- Lindenmeyer, F.; Li, H.; Menashi, S.; Soria, C.; Lu, H. Apigenin Acts on the Tumor Cell Invasion Process and Regulates Protease Production. Nutrition and Cancer 2001, 39, 139–147.
- Seo, H.S.; Ku, J.M.; Choi, H.S.; Woo, J.K.; Jang, B.H.; Shin, Y.C.; Ko, S.G. Induction of Caspase-Dependent Apoptosis by Apigenin by Inhibiting STAT3 Signaling in HER2-Overexpressing MDA-MB-453 Breast Cancer Cells. Anticancer Research 2014, 34, 2869–2882.
- Harrison, M.E.; Coombs, M.R.P.; Delaney, L.M.; Hoskin, D.W. Exposure of Breast Cancer Cells to a Subcytotoxic Dose of Apigenin Causes Growth Inhibition, Oxidative Stress, and Hypophosphorylation of Akt. Experimental and Molecular Pathology 2014, 97, 211–217.
- Seo, H.S.; Ku, J.M.; Choi, H.S.; Woo, J.K.; Shin, I.; Woo, J.K.; Park, S.Y.; Shin, Y.C.; Ko, S.K. Apigenin Induces Caspase-Dependent Apoptosis by Inhibiting Signal Transducer and Activator of Transcription 3 Signaling in HER2-Overexpressing SKBR3 Breast Cancer Cells. Molecular Medicine Reports 2015, 12, 2977–2984.
- Yin, F.; Giuliano, A.E.; Law, R.E.; Van Herle, A.J. Apigenin Inhibits Growth and Induces G2/M Arrest by Modulating Cyclin-CDK Regulators and ERK MAP Kinase Activation in Breast Carcinoma Cells. Anticancer Research 2000, 21, 413–420.
- Long, X.; Fan, M.; Bigsby, R.M.; Nephew, K.P. Apigenin Inhibits Antiestrogen-Resistant Breast Cancer Cell Growth Through Estrogen Receptor-α-Dependent and Estrogen Receptor-Α-Independent Mechanisms. Molecular Cancer Therapy 2008, 7, 2096–2108.
- Van Meeuwen, J.A.; Korthagen, N.; de Jong, P.C.; Piersma, A.H.; Van den Berg, M. Anti-estrogenic Effects of Phytochemicals on Human Primary Mammary Fibroblasts, MCF-7 Cells and Their Co-Culture. Toxicology and Applied Pharmacology 2007, 221, 372–383.
- Lee, W.J.; Chen, W.K.; Wang, C.J.; Lin, W.L.; Tseng T.H. Apigenin Inhibits HGF-Promoted Invasive Growth and Metastasis Involving Blocking PI3K/Akt Pathway and β4 Integrin Function in MDA-MB-231 Breast Cancer Cells. Toxicology and Applied Pharmacology 2008, 226, 178–191.
- Alol, L.H.; Al-Mzaien, K.A.; Hussein, S.M. The Promising Anticancer Efficacy of Parsley Seeds Flavonoid (Apigenin) in Induced Mammary Adenocarcinoma (AMN3) Mice. Journal of Physiology and Biomedical Sciences 2012, 25, 5–12.
- Menendez, J.A.; Vazquez-Martin, A.; Oliveras-Ferraros, C.; Garcia-Villalba, R.; Carrasco-Pancorbo, A.; Fernandez-Gutierrez, A.; Segura-Carretero, A. Analyzing Effects of Extra-Virgin Olive Oil Polyphenols on Breast Cancer-Associated Fatty Acid Synthase Protein Expression Using Reverse-Phase Protein Microarrays. International Journal of Molecular Medicine 2008, 22, 433.
- Zheng, P.W.; Chiang, L.C.; Lin, C.C. Apigenin Induced Apoptosis Through p53-Dependent Pathway in Human Cervical Carcinoma Cells. Life Sciences 2005, 76, 1367–1379.
- Czyż, J.; Madeja, Z.; Irmer, U.; Korohoda, W.; Hülser, D.F. Flavonoid Apigenin Inhibits Motility and Invasiveness of Carcinoma Cells in Vitro. International Journal of Cancer 2005, 114, 12–18.
- Liu, J.; Cao, X.C.; Xiao, Q.; Quan, M.F. Apigenin Inhibits HeLa Sphere-Forming Cells Through Inactivation of Casein Kinase 2α. Molecular Medicine Reports 2015, 11, 665–669.
- Wu, K.; Yuan, L.H.; Xia, W. Inhibitory Effects of Apigenin on the Growth of Gastric Carcinoma SGC-7901 Cells. World Journal of Gastroenterology 2005, 11, 4461.
- Jiang, D.; Liu, F.; Huang, Q.L. Selectively Inhibition of Cell Viability and Induction of Apoptosis in Human Gastric Cancer BGC-823 Cell Line by Apigenin. Journal of Hunan Norway University (Medical Sciences) 2010, 3, 006.
- Chen, J.; Chen, J.; Li, Z.; Liu, C.; Yin, L. The Apoptotic Effect of Apigenin on Human Gastric Carcinoma Cells Through Mitochondrial Signal Pathway. Tumor Biology 2014, 35, 7719–7726.
- Lefort, E.C.; Blay, J. Apigenin and Its Impact on Gastrointestinal Cancers. Molecular Nutrition and Food Research 2013, 57, 126–144.
- Hu, T.P.; Cao, J.G. Study on Pro-Apoptotic Effect of Apigenin on Human Gastric Cancer Cells and Its Underlying Mechanisms. International Journal of Pathology and Clinical Medicine 2007, 1, 001.
- Kuo, C.H.; Weng, B.C.; Wu, C.C.; Yang, S.F.; Wu, D.C.; Wang, Y.C. Apigenin Has Anti-Atrophic Gastritis and Anti-Gastric Cancer Progression Effects in Helicobacter Pylori Infected Mongolian Gerbils. Journal of Ethnopharmacology 2014, 151, 1031–1039.
- Yuan, L.; Xia, W.; Zhao, X.; Zhang, X. Zhang, L.; Wu, K. Inhibition of PKB/Akt Activity Involved in Apigenin-Induced Apoptosis in Human Gastric Carcinoma Cells. Chinese Science Bulletin 2007, 52, 2226–2232.
- Isoda, H.; Motojima, H.; Onaga, S.; Samet, I.; Villareal, M.O.; Han, J. Analysis of the Erythroid Differentiation Effect of Flavonoid Apigenin on K562 Human Chronic Leukemia Cells. Chemico-Biological Interactions 2014, 220, 269–277.
- Solmaz, S.; Adan, Gokbulut, A.; Cincin, B.; Ozdogu, H.; Boga, C.; Cakmakoglu, B.; Kozanoglu, I.; Baran, Y. Therapeutic Potential of Apigenin, a Plant Flavonoid, for Imatinib-Sensitive and Resistant Chronic Myeloid Leukemia Cells. Nutrition and Cancer 2014, 66, 599–612.
- Zhao, M.; Ma, J.; Zhu, H.Y.; Zhang, X.H.; Du, Z.Y.; Xu, Y.J.; Yu, X.D. Apigenin Inhibits Proliferation and Induces Apoptosis in Human Multiple Myeloma Cells Through Targeting The Trinity of CK2, Cdc37 and Hsp90. Molecular Research 2011, 10, 104.
- Liao, Y.; Shen, W.; Kong, G.; Lv, H.; Tao, W.; Bo, P. Apigenin Induces the Apoptosis And Regulates MAPK Signaling Pathways in Mouse Macrophage ANA-1 Cells. PloS One 2014, 9, 3.
- Saeed, M.; Kadioglu, O.; Khalid, H.; Sugimoto, Y.; Efferth T. Activity of the Dietary Flavonoid, Apigenin, Against Multidrug-Resistant Tumor Cells as Determined by Pharmacogenomics and Molecular Docking. The Journal of Nutritional Biochemistry 2015, 26, 44–56.
- Johnson, J.L.; Mejia, E.G. Flavonoid Apigenin Modified Gene Expression Associated with Inflammation and Cancer and Induced Apoptosis in Human Pancreatic Cancer Cells Through Inhibition of GSK‐3β/NF‐κB Signaling Cascade. Molecular Nutrition & Food Research 2013, 57, 2112–2127.
- Melstrom, L.G.; Salabat, M.R.; Ding, X.Z.; Milam, B.M.; Strouch, M.; Pelling, J.C.; Bentrem D.J. Apigenin Inhibits the GLUT-1 Glucose Transporter and the Phosphoinositide 3-Kinase/Akt Pathway in Human Pancreatic Cancer Cells. Pancreas 2008, 37, 426–431.
- Lee, S.H.; Ryu, J.K.; Lee, K.Y.; Woo, S.M.; Park, J.K.; Yoo, J.W.; Kim, Y.T.; Yoon, Y.B. Enhanced Anti-Tumor Effect of Combination Therapy with Gemcitabine and Apigenin in Pancreatic Cancer. Cancer Letters 2008, 259, 39–49.
- Wu, D.G.; Yu, P.; Li, J.W.; Jiang, P.; Sun, J.; Wang, H.Z.; Zhang, L.D.; Wen, M.B.; Bie, P. Apigenin Potentiates the Growth Inhibitory Effects by IKK-β-Mediated NF-κB Activation in Pancreatic Cancer Cells. Toxicology Letters 2014, 224, 157–164.
- Mrazek, A.A.; Porro, L.J.; Bhatia, V.; Falzon, M.; Spratt, H.; Zhou J.; Chao, C.; Hellmich, M.R. Apigenin Inhibits Pancreatic Stellate Cell Activity in Pancreatitis. Journal of Surgical Research 2015, 196, 8–16.
- Chen, R.; Hollborn, M.; Grosche, A.; Reichenbach, A.; Wiedemann, P.; Bringmann, A.; Kohen, L. Effects of the Vegetable Polyphenols Epigallocatechin-3-Gallate, Luteolin, Apigenin, Myricetin, Quercetin, and Cyanidin in Primary Cultures of Human Retinal Pigment Epithelial Cells. Molecular Vision 2014, 20, 242.
- Mandery, K.; Bujok, K.; Schmidt, I.; Keiser, M.; Siegmund, W.; Balk, B.; König, J.; Fromm, M.F.; Glaeser, H. Influence of the Flavonoids Apigenin; Kaempferol and Quercetin on the Function of Organic Anion Transporting Polypeptides 1A2 and 2B1. Biochemical Pharmacology 2010, 80, 1746–1753.
- Xu, Y.Y.; Wu, T.T.; Zhou, S.H.; Bao, Y.Y.; Wang, Q.Y.; Fan, J.; Huang, Y.P. Apigenin Suppresses GLUT-1 and p-AKT Expression to Enhance the Chemosensitivity to Cisplatin Of Laryngeal Carcinoma Hep-2 Cells: An in Vitro Study. International Journal of Clinical and Experimental Pathology 2014, 7, 3938.
- Arango, D.; Morohashi, K.; Yilmaz, A.; Kuramochi, K.; Parihar, A.; Brahimaj, B.; Grotewold, E.; Doseff, A.I. Molecular Basis for the Action of a Dietary Flavonoid Revealed by the Comprehensive Identification of Apigenin Human Targets. Procedeeingsof the National Academy of Sciencesof the United States of America 2013, 110, E2153–E2162.
- Vanić, Ž. Phospholipid Vesicles for Enhanced Drug Delivery in Dermatology. Journal of Drug Discovery and Developmental Delivery 2015, 2, 1–9.
- Zhai, Y.; Guo, S.; Liu, C.; Yang, C.; Dou, J.; Li, L.; Zhai, G. Preparation and in Vitro Evaluation of Apigenin-Loaded Polymeric Micelles. Colloids and Surfaces A: Physicochemical and Engineering Aspects 2013, 429, 24–30.
- Zhao, L.; Zhang, L.; Meng, L.; Wang, J.; Zhai, G. Design and Evaluation of a Self-Micro Emulsifying Drug Delivery System for Apigenin. Drug Development and Industrial Pharmacy 2013, 39, 662–669.
- Sen, K.; Banerjee, S.; Mandal, M. Reversal of Warburg Effect by Apigenin and 5-Fluorouracil Loaded Dual Drug Liposomes Result in Enhanced Colorectal Chemotherapy. Cancer Research 2014, 74, 4594–4594.
- Ding, S.M.; Zhang, Z.H.; Song, J.; Cheng, X.D.; Jiang, J.; Jia, X.B. Enhanced Bioavailability of Apigenin Via Preparation of a Carbon Nanopowder Solid Dispersion. International Journal of Nanomedicine 2014, 9, 2.